- 1Research Division, Dynamical Business and Science Society–DBSS International SAS, Bogotá, Colombia
- 2Research Group in Physical Activity, Sports and Health Sciences (GICAFS), Universidad de Córdoba, Montería, Colombia
- 3Hologenomiks Research Group, Department of Genetics, Physical Anthropology and Animal Physiology, University of the Basque Country (UPV/EHU), Leioa, Spain
- 4Physiology of Work and Exercise Response (POWER) Laboratory, Institute of Exercise Physiology and Rehabilitation Science, University of Central Florida, Orlando, FL, United States
- 5Faculty of Kinesiology and Health Studies, University of Regina, Regina, SK, Canada
- 6Department of Health Sciences, Faculty of Health Sciences, University of Jaén, Jaén, Spain
- 7Facultad de Deportes, Universidad Autónoma de Baja California, Tijuana, Mexico
- 8Facultad de Medicina y Psicología, Universidad Autónoma de Baja California, Tijuana, Mexico
- 9Department of Physical Education Studies, Brandon University, Brandon, MB, Canada
- 10Applied Bioenergetics Lab, Faculty of Sport and Physical Education, University of Novi Sad, Novi Sad, Serbia
- 11Department of Nutrition and Public Health, University of Agder, Kristiansand, Norway
- 12Faculty of Health Sciences, University of Pecs, Pécs, Hungary
- 13Physical Education and Sport, Faculty of Medicine, University of Málaga, Málaga, Spain
- 14Exercise and Sport Nutrition Laboratory, Human Clinical Research Facility, Texas A&M University, College Station, TX, United States
Introduction
Sarcopenia is generally defined as an age-related musculoskeletal disease characterized by the reductions in muscle strength, lean/muscle mass (quality) and functional ability leading to various adverse health outcomes, including impaired physical function and reduced quality of life (Kirk et al., 2024). Sarcopenia is also associated with cognitive decline in older adults (Arosio et al., 2023). As sarcopenia is a potentially reversible condition whose prevalence increases with age, several non-pharmacological strategies have been developed to counteract its progression in older adults (Cannataro et al., 2021; Cannataro et al., 2022).
Sedentary and physically inactive older adults exhibit a reduced myofibrillar protein-synthesis response to dietary protein intake, which significantly accelerates the progression of sarcopenia (Moore, 2014). This issue is further exacerbated in obese elderly populations. Age-related muscle ‘anabolic resistance’ becomes particularly pronounced in response to low or moderate protein intake, a dietary pattern commonly observed in older individuals (Aragon et al., 2023). It is now recognized that a daily intake of at least 1.0 g of protein per kg/day, rich in essential amino acids (primarily leucine) is crucial to maintaining positive protein balance in musculoskeletal tissue. While we acknowledge the importance of high protein intake in older adults, we will not elaborate on this topic, given the extensive international and governmental efforts already in place. For example, the ESPEN Expert Group (Deutz et al., 2014) and the PROT-AGE Study Group (Bauer et al., 2013) provide international guidelines, while governmental initiatives such as the World Health Organization’s ‘Keep fit for life: meeting the nutritional needs of older persons’ (Organization WH and Policy TU-SoNS, 2002) and the European Commission’s ‘Dietary recommendations for protein intake for adults and older adults’ (Commission) address this issue comprehensively.
In this opinion article, we advocate for the combination of creatine monohydrate supplementation and resistance training as a safe and effective non-pharmacological strategy to prevent and treat sarcopenia that should be internationally recognized by health practitioners and public health organizations.
Creatine is a conditionally essential nutrient for lifelong vitality
Creatine is derived from reactions involving amino acids in the liver and brain. Approximately 95% of the creatine pool in the body is found in skeletal muscle, while 5% is found in other tissues with high energy demands such as cardiomyocytes, hepatocytes, kidney cells, inner ear cells, enterocytes, spermatozoa, and photoreceptor cells. Creatine in its phosphorylated form, phosphocreatine (PCr), plays a pivotal role in sustaining adenosine triphosphate (ATP) in these cells (Bonilla et al., 2021a).
Creatine is naturally present in meat, fish, and poultry with an estimated daily requirement of approximately 2 g·day−1 for a 70-kg male to maintain normal creatine levels in the human body (Kreider and Stout, 2021; Wallimann et al., 2011). Notwithstanding, research across diverse populations has demonstrated that endogenous creatine synthesis may be insufficient under numerous physiological and pathological conditions (Ostojic and Forbes, 2022).
Following the groundbreaking research of Dr. Roger Harris in 1992 (Harris et al., 1992), creatine monohydrate became widely available as a dietary supplement in the United States and Europe, fully compliant with the U.S. Food and Drug Administration (FDA) regulations. Given its natural presence in food and its availability as a supplement before 15 October 1994, creatine was grandfathered under the Dietary Supplement Health and Education Act (DSHEA) as a legal dietary supplement in the U.S. Recently, the FDA expressed no objections to a Generally Recognized as Safe (GRAS) application, permitting the inclusion of creatine monohydrate as a food additive in various food products (GRAS Notice 931) (USFaD and Inventories, 2020). Further, after consultation on novel food status under Regulation (EU) 2015/2283, creatine is not considered a novel food as creatine monohydrate has been used for human consumption to a significant degree in the European Union before 15 May 1997 (Safety EHaF, 2023). Creatine is approved for inclusion in dietary supplements and/or food products in numerous countries, including Canada, Australia, the European Union, Japan, South Korea, and Brazil, among others (Jager et al., 2011). Extensive research, including randomized, double-blind, placebo-controlled clinical trials, have consistently demonstrated that creatine monohydrate supplementation is both safe and effective in humans (Kreider and Stout, 2021; Kreider et al., 2017; Kreider et al., 2022), including older adults (Candow et al., 2022; Candow and Moriarty, 2024). Thus, there is a strong consensus within the scientific community that creatine monohydrate supplementation (e.g., 20 g/day or 0.3 g/kg/day for 5–7 days; 3–5 g/day or 0.03 g/kg/day; or 0.1–0.14 g/kg/day) can safely and effectively enhance exercise performance capacity and training adaptations in both untrained and trained individuals, regardless of exercise interventions, biological sex or age (Kreider and Stout, 2021; Kreider et al., 2017; Burke et al., 2019; Candow et al., 2019; Forbes et al., 2021a; Kley et al., 2013; Maughan et al., 2018; Bakian et al., 2020; Korovljev et al., 2021a; Korovljev et al., 2021b; Korovljev et al., 2021c; Ostojic, 2021a; Ostojic, 2021b; Ostojic, 2021c; Ostojic et al., 2021a; Todorovic et al., 2023; Ostojic et al., 2024a; Ostojic et al., 2024b).
Consequently, an adequate intake of dietary creatine is likely conditionally essential for maintaining optimal health and promoting growth (Ostojic and Forbes, 2022). Due to the energy and mechanical optimization of cells (Bonilla et al., 2021a), increasing intracellular creatine levels through nutritional supplementation reduces protein degradation (Parise et al., 1985), promotes activation of satellite cells (Olsen et al., 2006), and increases whole-body lean tissue mass (Pashayee-Khamene et al., 2024). The cellular bioenergetics improvements after creatine supplementation offer potential benefits beyond musculoskeletal tissue, such as in the brain, the heart, vascular health, immune system, among others (Roschel et al., 2021; Clarke et al., 2021; Solis et al., 2021; Balestrino, 2021), especially in older adults (Candow et al., 2019; Gielen et al., 2021). In the context of this opinion article, several studies show that creatine monohydrate supplementation can augment muscle mass, physical and cognitive function in older adults (Kreider et al., 2017; Candow et al., 2021; Forbes et al., 2021b), with more clinically significant effects when combined with resistance training (Delpino et al., 2022).
Resistance training offsets sarcopenia
Strength training involves contracting skeletal muscles to work against an external force. Resistance training, a form of strength training, involves different types of applied external forces, such as body weight, free weights, or resistance bands. In this article, we focus on resistance training, emphasizing its role in increasing muscle mass and strength, which are linked to improved health outcomes. Resistance training offers several physical benefits, including enhanced muscle strength, endurance, and power, along with increased bone mineral density and connective tissue remodeling. Clinically, it contributes to cardiometabolic health, helps prevent neurodegenerative disorders and mental health issues, and improves functionality, leading to reduced frailty and a lower risk of falls (American College of Sports et al., 2009; Cunha et al., 2024). Based on the available evidence, resistance training constitutes a key component of physical conditioning programs aimed at improving activities of daily living, self-care, and quality of life while reducing all-cause mortality in older adults (Cannataro et al., 2022; Kraschnewski et al., 2016).
The resistance exercise recommendations provided by the American College of Sports Medicine (ACSM) in collaboration with the American Heart Association (AHA) emphasize a training frequency of at least 2 days per week. The recommended intensity ranges from moderate (Moore, 2014; Aragon et al., 2023) to vigorous (Deutz et al., 2014; Bauer et al., 2013) on a scale of 0–10. The program should include progressive weight training with 8–10 exercises targeting major muscle groups, performing 8–12 repetitions per exercise (American College of Sports et al., 2009). Additionally, the National Strength and Conditioning Association (NSCA) suggests a resistance training regimen for older adults that involves 8–10 different free-weight or machine-based exercises, focusing on multi-joint movements and power/explosive training. This regimen includes 1-3 sets per exercise per muscle group, with 8–12 or 10–15 repetitions at 70%–85% of 1-RM, performed 2–3 days per week (per muscle group) (Fragala et al., 2019). Recent clinical evidence indicates that both low and high-frequency resistance training effectively enhances muscular strength, skeletal muscle mass, and muscle quality in older women with sarcopenia (Dos Santos et al., 2024). Cluster-set resistance training is an alternative approach that warrants further investigation in older adults, given its potential effectiveness in achieving superior results with less overall effort (Cannataro et al., 2022).
Before starting a resistance training program for older adults, it is advisable to use the ACSM’s exercise preparticipation screening tool for risk stratification (available at the ACSM Resource Library) and to establish goals based on a periodization plan. Assessing perceived exertion and pain levels are well-established methods for monitoring internal load and pain thresholds in different populations, including those at risk of frailty like older adults (Izquierdo et al., 2021). These straightforward techniques are effective for accurately tracking training intensity and making necessary adjustments (Falk Neto et al., 2020; Yu et al., 2021). Our recent findings suggest that using rating of perceived exertion (RPE) scales and perceived movement velocity is a valid, cost-effective, and practical method for assessing resistance training load progression and complementing other training metrics. Exercise professionals should ensure that participants are familiar with RPE scales and consider factors that may influence perceived exertion, such as training status, motivation, and environmental conditions (Petro et al., 2024).
We have previously outlined a comprehensive framework for understanding the etiology of resistance training-related injuries and provided detailed recommendations for exercise professionals, clinical exercise physiologists, and health practitioners to consider when designing exercise programs for older adults (Bonilla et al., 2022). Accordingly, it is essential to include sessions dedicated to familiarizing individuals with proper exercise techniques, irrespective of their experience or the type of resistance used (e.g., free weights, machine-based, or bodyweight exercises). These sessions should focus on teaching, observing, and correcting exercise techniques. Adhering to the safety principle in resistance training, it is crucial to select exercises that do not impact participants’ wellbeing. It is advisable to begin with simpler exercises (using machines or light weights) and gradually progress to more complex exercises (such as free weights with moderate-to-high loads) that demand greater control.
To enhance the health, functional capacity, and quality of life for older adults, it is recommended to implement community-based exercise programs that focus on moderate-intensity activities, such as circuit resistance training. Meta-analytic evidence demonstrates that these programs are more effective than standard care in improving functional capacity and health-related quality of life (Desveaux et al., 2014). Social media interventions targeting lifestyle behaviors—such as online communities, interactive web platforms, online education, and healthcare resources—have been well-received and can effectively promote physical activity among older adults (McKeon et al., 2022). Furthermore, promoting exercise programs that incorporate prescribed home-based therapy is also beneficial (Ricke et al., 2023). A notable example of these applications is the Curves Women’s Health and Fitness Initiative at the Exercise and Sport Nutrition Laboratory (Texas A&M University). This initiative has highlighted several benefits of circuit-style resistance training programs and the use of technology for health applications in women living with aging conditions (e.g., type-2 diabetes, obesity, etc.), including older females (Kerksick et al., 2009; Kreider et al., 2011; Magrans-Courtney et al., 2011; Galbreath et al., 2018; Kerksick et al., 2020).
Finally, creating and sustaining active environments that offer safe and equitable access to resistance exercise opportunities is crucial for older adults. This involves ensuring that cities and communities provide spaces and facilities that accommodate individuals of all ages and abilities, facilitating regular engagement in resistance training. For recommendations detailing the characteristics of resistance training protocols prescribed for older adults and a description of program variables, readers should refer to the Position Statement from the National Strength and Conditioning Association (Fragala et al., 2019) and the recent scoping review on the topic by da Silva et al. (2023).
The benefits of creatine supplementation plus resistance training in older adults
Creatine monohydrate supplementation (≥5 g/day; 0.1–0.14 g/kg/day) during a resistance training program has the potential to preserve mental and physical abilities and mitigate sarcopenia and its associated risks. Table 1 shows large-scale epidemiological, meta-analytic and regulatory evidence supporting the importance of creatine intake and its positive effects when combined with resistance training to enhance cognitive function and physical vitality in older adults.
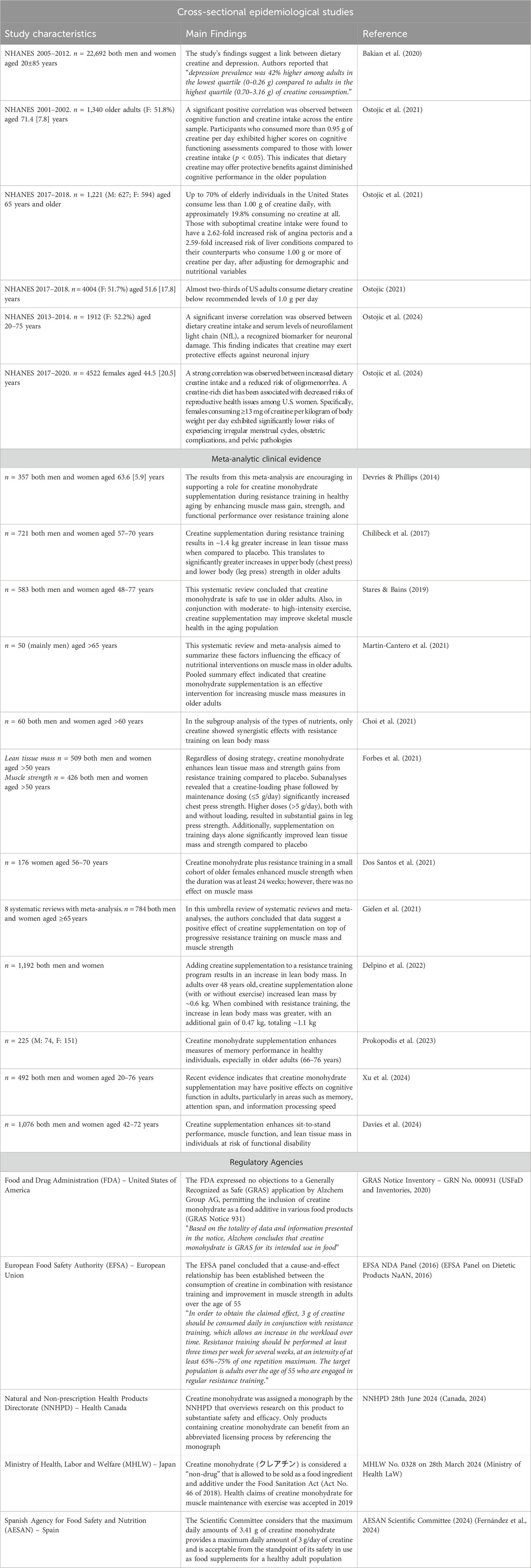
Table 1. Epidemiological, meta-analytic and regulatory evidence supporting the intake of creatine alone or combined with resistance training in older populations.
The understanding of creatine’s potential cognitive benefits has advanced with recent studies, yet significant research is still needed to clarify the underlying mechanisms. Previously, we have described plausible biological regulators mediating effects of creatine supplementation using convergent functional genomics (Bonilla et al., 2021b) and have also found that cellular allostasis relies heavily on the creatine kinase/phosphocreatine system, which contributes to the intricate balance of subcellular energy production and cellular mechanics (Bonilla et al., 2021a). This reliance has been clinically evidenced by improvements in cerebral high-energy phosphates, cognitive performance, and processing speed, as reported by Gordji-Nejad et al. (2024) following a high single dose of creatine monohydrate (0.35 g/kg) during sleep deprivation. Furthermore, Bian et al. (2023) provided valuable insights into the potential role of creatine as a neurotransmitter, presenting strong evidence of creatine’s presence within synaptic vesicles. Although promising, these cognitive improvements require standardized measurement approaches and further investigation into their clinical implications for older adults at risk of neurodegenerative diseases. To address these gaps, we invite the scientific community to contribute their data and insights to our research topic “Exploring Creatine Supplementation: Enhancing Physical and Cognitive Health in Older Adults”, where the latest findings, next research directions, and collaborative efforts on creatine for health and disease will be collected. For example, in addition to what is listed in Table 1, more clinical research on creatine benefits in preventing the development of several other aging long terms conditions are needed (e.g., diabetes, cardiovascular disease, orthopaedical function, frailty, etc.).
Overall, creatine supplementation in combination with resistance training serves as a safe and effective approach to counteract the progression of sarcopenia (Figure 1). It is clear that creatine monohydrate supplementation—regardless of creatine loading, maintenance dosage, or frequency of ingestion—during a resistance training program increases lean tissue mass and strength compared to a placebo and resistance training alone in older adults (Forbes et al., 2021b). We advocate for the prompt implementation of public health initiatives that promote the inclusion of creatine-rich foods in human nutrition (www.creatine.global) and invite practitioners to visit www.creatineforhealth.com, an initiative that brings together creatine researchers worldwide to accelerate awareness about the role of creatine supplementation for health and in clinical diseases.
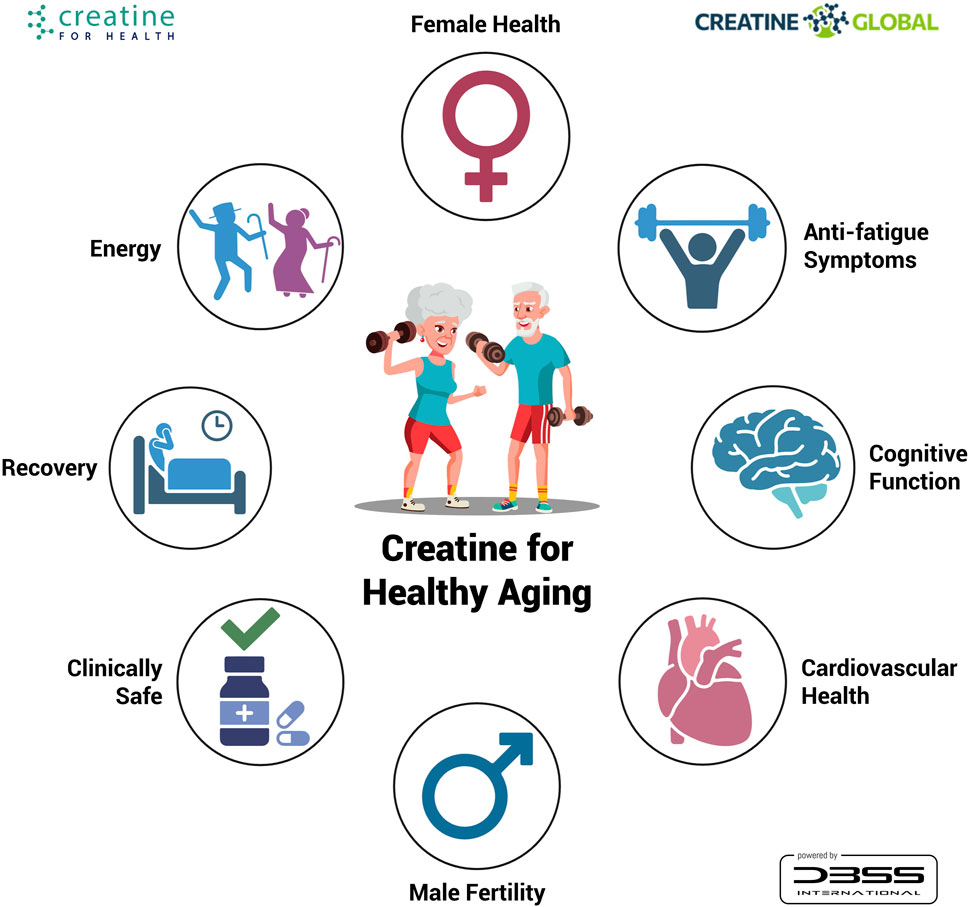
Figure 1. Benefits of creatine monohydrate supplementation plus resistance training in older adults.
Author contributions
DB: Conceptualization, Formal Analysis, Funding acquisition, Investigation, Methodology, Project administration, Software, Visualization, Writing–original draft. JS: Conceptualization, Data curation, Formal Analysis, Supervision, Validation, Writing–review and editing. DC: Data curation, Validation, Writing–review and editing. JJ-G: Data curation, Validation, Writing–review and editing. LG-M: Data curation, Formal Analysis, Validation, Writing–review and editing. MO-O: Data curation, Validation, Writing–review and editing. SF: Data curation, Validation, Writing–review and editing. SO: Data curation, Validation, Writing–review and editing. SV-M: Data curation, Validation, Writing–review and editing. RK: Conceptualization, Data curation, Formal Analysis, Supervision, Validation, Writing–review and editing.
Funding
The author(s) declare that no financial support was received for the research, authorship, and/or publication of this article.
Acknowledgments
Editors of the research topic “Cognitive Impairment and Physical Function in Older Adults” participated in this opinion article. This had no impact on the peer review process and the final decision.
Conflict of interest
DB serves as scientific and managing director of KreaFood-an R&D&I Project, has served as science product manager for MTX Corporation® in Europe, has acted as a scientific consultant for MET-Rx® and Healthy Sports in Colombia, has conducted academic-sponsored research on creatine, and receives honoraria for speaking about dietary supplements at international conferences and private courses. SO co-owns patent “Supplements Based on Liquid Creatine” at the European Patent Office (WO2019150323 A1). SO has received research support related to creatine during the past 36 months from the Ministry of Education, Science, and Technological Development; Provincial Secretariat for Higher Education and Scientific Research; Alzchem Group AG; ThermoLife International; and Hueston Hennigan LLP. DC has conducted industry-sponsored research involving creatine supplementation and received creatine donations for scientific studies and travel support for presentations involving creatine supplementation at scientific conferences. In addition, DC serves as an expert witness/consultant in legal cases involving creatine supplementation. SF has received creatine donations for scientific studies and is a scientific advisor for Bear Balanced, a company that sells creatine products. JS has conducted industry-sponsored research on sports nutrition over the past 25 years. Further, JS has also received financial support for presenting on the science of various nutraceuticals, at industry-sponsored scientific conferences. RK has conducted industry-sponsored research on creatine, received financial support for presenting on dietary supplements at industry sponsored scientific conferences, and has served as an expert witness on cases related to creatine. Finally, RK serves as chair of the “Creatine for Health” scientific advisory board sponsored by Creapure® and Creavitalis® -Alzchem Group AG while DB, JS, DC, SF and SO serve as members of this board.
The remaining authors declare that the research was conducted in the absence of any commercial or financial relationships that could be construed as a potential conflict of interest.
The author(s) declared that they were an editorial board member of Frontiers, at the time of submission. This had no impact on the peer review process and the final decision.
Publisher’s note
All claims expressed in this article are solely those of the authors and do not necessarily represent those of their affiliated organizations, or those of the publisher, the editors and the reviewers. Any product that may be evaluated in this article, or claim that may be made by its manufacturer, is not guaranteed or endorsed by the publisher.
References
American College of Sports M., Chodzko-Zajko W. J., Proctor D. N., Fiatarone Singh M. A., Minson C. T., Nigg C. R., et al. (2009). American College of Sports Medicine position stand. Exercise and physical activity for older adults. Med. Sci. Sports Exerc 41 (7), 1510–1530. doi:10.1249/MSS.0b013e3181a0c95c
Aragon A. A., Tipton K. D., Schoenfeld B. J. (2023). Age-related muscle anabolic resistance: inevitable or preventable? Nutr. Rev. 81 (4), 441–454. doi:10.1093/nutrit/nuac062
Arosio B., Calvani R., Ferri E., Coelho-Junior H. J., Carandina A., Campanelli F., et al. (2023). Sarcopenia and cognitive decline in older adults: targeting the muscle-brain Axis. Nutrients 15 (8), 1853. doi:10.3390/nu15081853
Bakian A. V., Huber R. S., Scholl L., Renshaw P. F., Kondo D. (2020). Dietary creatine intake and depression risk among U.S. adults. Transl. Psychiatry 10 (1), 52. doi:10.1038/s41398-020-0741-x
Balestrino M. (2021). Role of creatine in the heart: health and disease. Nutrients 13 (4), 1215. doi:10.3390/nu13041215
Bauer J., Biolo G., Cederholm T., Cesari M., Cruz-Jentoft A. J., Morley J. E., et al. (2013). Evidence-based recommendations for optimal dietary protein intake in older people: a position paper from the PROT-AGE Study Group. J. Am. Med. Dir. Assoc. 14 (8), 542–559. doi:10.1016/j.jamda.2013.05.021
Bian X., Zhu J., Jia X., Liang W., Yu S., Li Z., et al. (2023). Suggestion of creatine as a new neurotransmitter by approaches ranging from chemical analysis and biochemistry to electrophysiology. Elife 12. doi:10.7554/eLife.89317
Bonilla D. A., Cardozo L. A., Velez-Gutierrez J. M., Arevalo-Rodriguez A., Vargas-Molina S., Stout J. R., et al. (2022). Exercise selection and common injuries in fitness centers: a systematic integrative review and practical recommendations. Int. J. Environ. Res. Public Health 19 (19), 12710. doi:10.3390/ijerph191912710
Bonilla D. A., Kreider R. B., Stout J. R., Forero D. A., Kerksick C. M., Roberts M. D., et al. (2021a). Metabolic basis of creatine in health and disease: a bioinformatics-assisted review. Nutrients 13 (4), 1238. doi:10.3390/nu13041238
Bonilla D. A., Moreno Y., Rawson E. S., Forero D. A., Stout J. R., Kerksick C. M., et al. (2021b). A convergent functional genomics analysis to identify biological regulators mediating effects of creatine supplementation. Nutrients 13 (8), 2521. doi:10.3390/nu13082521
Burke L. M., Castell L. M., Casa D. J., Close G. L., Costa R. J. S., Desbrow B., et al. (2019). International association of athletics federations consensus statement 2019: nutrition for athletics. Int. J. Sport Nutr. Exerc Metab. 29 (2), 73–84. doi:10.1123/ijsnem.2019-0065
Canada H. (2024). Creatine monohydrate Canada. Vancouver, Canada: Health Canada. Available at: https://webprod.hc-sc.gc.ca/nhpid-bdipsn/atReq?atid=creatine.mono&lang=eng ([updated 28th June, 2024. Monograph on creatine monohydrate].
Candow D. G., Chilibeck P. D., Forbes S. C., Fairman C. M., Gualano B., Roschel H. (2022). Creatine supplementation for older adults: focus on sarcopenia, osteoporosis, frailty and Cachexia. Bone 162, 116467. doi:10.1016/j.bone.2022.116467
Candow D. G., Forbes S. C., Chilibeck P. D., Cornish S. M., Antonio J., Kreider R. B. (2019). Effectiveness of creatine supplementation on aging muscle and bone: focus on falls prevention and inflammation. J. Clin. Med. 8 (4), 488. doi:10.3390/jcm8040488
Candow D. G., Forbes S. C., Kirk B., Duque G. (2021). Current evidence and possible future applications of creatine supplementation for older adults. Nutrients 13 (3), 745. doi:10.3390/nu13030745
Candow D. G., Moriarty T. (2024). Effects of creatine monohydrate supplementation on muscle, bone and brain- hope or hype for older adults? Curr. Osteoporos. Rep. 23 (1), 1. doi:10.1007/s11914-024-00895-x
Cannataro R., Carbone L., Petro J. L., Cione E., Vargas S., Angulo H., et al. (2021). Sarcopenia: etiology, nutritional approaches, and miRNAs. Int. J. Mol. Sci. 22 (18), 9724. doi:10.3390/ijms22189724
Cannataro R., Cione E., Bonilla D. A., Cerullo G., Angelini F., D'Antona G. (2022). Strength training in elderly: an useful tool against sarcopenia. Front. Sports Act. Living. 4, 950949. doi:10.3389/fspor.2022.950949
Chilibeck P. D., Kaviani M., Candow D. G., Zello G. A. (2017). Effect of creatine supplementation during resistance training on lean tissue mass and muscular strength in older adults: a meta-analysis. Open Access J. Sports Med. 8, 213–226. doi:10.2147/OAJSM.S123529
Choi M., Kim H., Bae J. (2021). Does the combination of resistance training and a nutritional intervention have a synergic effect on muscle mass, strength, and physical function in older adults? A systematic review and meta-analysis. BMC Geriatr. 21 (1), 639. doi:10.1186/s12877-021-02491-5
Clarke H., Hickner R. C., Ormsbee M. J. (2021). The potential role of creatine in vascular health. Nutrients 13 (3), 857. doi:10.3390/nu13030857
Commission, E. (2021). Dietary recommendations for protein intake for adults and older adults: health promotion and disease prevention knowledge gateway. A reference point public health policy makers Reliab. Indep. up-to date Inf. Top. Relat. Promot. health well-being. Available at: https://knowledge4policy.ec.europa.eu/health-promotion-knowledge-gateway/dietary-protein-intake-adults-3_en.
Cunha P. M., Werneck A. O., Santos L. D., Oliveira M. D., Zou L., Schuch F. B., et al. (2024). Can resistance training improve mental health outcomes in older adults? A systematic review and meta-analysis of randomized controlled trials. Psychiatry Res. 333, 115746. doi:10.1016/j.psychres.2024.115746
da Silva L. S. L., Goncalves L. D. S., Abdalla P. P., Benjamim C. J. R., Tasinafo M. F., Venturini A. C. R., et al. (2023). Characteristics of resistance training-based protocols in older adults with sarcopenic obesity: a scoping review of training procedure recommendations. Front. Nutr. 10, 1179832. doi:10.3389/fnut.2023.1179832
Davies T. W., Watson N., Pilkington J. J., McClelland T. J., Azzopardi G., Pearse R. M., et al. (2024). Creatine supplementation for optimization of physical function in the patient at risk of functional disability: a systematic review and meta-analysis. JPEN J. Parenter. Enter. Nutr. 48 (4), 389–405. doi:10.1002/jpen.2607
Delpino F. M., Figueiredo L. M., Forbes S. C., Candow D. G., Santos H. O. (2022). Influence of age, sex, and type of exercise on the efficacy of creatine supplementation on lean body mass: a systematic review and meta-analysis of randomized clinical trials. Nutrition 103-104, 111791. doi:10.1016/j.nut.2022.111791
Desveaux L., Beauchamp M., Goldstein R., Brooks D. (2014). Community-based exercise programs as a strategy to optimize function in chronic disease: a systematic review. Med. Care 52 (3), 216–226. doi:10.1097/MLR.0000000000000065
Deutz N. E., Bauer J. M., Barazzoni R., Biolo G., Boirie Y., Bosy-Westphal A., et al. (2014). Protein intake and exercise for optimal muscle function with aging: recommendations from the ESPEN Expert Group. Clin. Nutr. 33 (6), 929–936. doi:10.1016/j.clnu.2014.04.007
Devries M. C., Phillips S. M. (2014). Creatine supplementation during resistance training in older adults-a meta-analysis. Med. Sci. Sports Exerc 46 (6), 1194–1203. doi:10.1249/MSS.0000000000000220
Dos Santos E. E. P., de Araujo R. C., Candow D. G., Forbes S. C., Guijo J. A., de Almeida Santana C. C., et al. (2021). Efficacy of creatine supplementation combined with resistance training on muscle strength and muscle mass in older females: a systematic review and meta-analysis. Nutrients 13 (11), 3757. doi:10.3390/nu13113757
Dos Santos V. R., Antunes M., Dos Santos L., Nascimento M. A., Pina F. L. C., Carneiro N. H., et al. (2024). Effects of different resistance training frequencies on body composition, muscular strength, muscle quality, and metabolic biomarkers in sarcopenic older women. J. Strength Cond. Res. 38 (9), e521–e528. doi:10.1519/JSC.0000000000004827
EFSA Panel on Dietetic Products NaAN (2016). Creatine in combination with resistance training and improvement in muscle strength: evaluation of a health claim pursuant to Article 13(5) of Regulation (EC) No 1924/2006. EFSA J. 14 (2), 4375. doi:10.2903/j.efsa.2016.4375
Falk Neto J. H., Tibana R. A., de Sousa N. M. F., Prestes J., Voltarelli F. A., Kennedy M. D. (2020). Session rating of perceived exertion is a superior method to monitor internal training loads of functional fitness training sessions performed at different intensities when compared to training impulse. Front. Physiol. 11, 919. doi:10.3389/fphys.2020.00919
Fernández Á. J. G., Izquierdo Á. G., García C. M. A., Lesmes I. B., Martínez G. N., Sánchez S. P., et al. (2024). Report of the Scientific Committee of the Spanish Agency for Food Safety and Nutrition (AESAN) on the risk associated with the consumption of food supplements that contain creatine as an ingredient. Food Risk Assess. Eur. 2 (4). doi:10.2903/fr.efsa.2024.fr-0046
Forbes S. C., Candow D. G., Ferreira L. H. B., Souza-Junior T. P. (2021a). Effects of creatine supplementation on properties of muscle, bone, and brain function in older adults: a narrative review. J. Diet. Suppl. 19, 318–335. doi:10.1080/19390211.2021.1877232
Forbes S. C., Candow D. G., Ostojic S. M., Roberts M. D., Chilibeck P. D. (2021b). Meta-Analysis examining the importance of creatine ingestion strategies on lean tissue mass and strength in older adults. Nutrients 13 (6), 1912. doi:10.3390/nu13061912
Fragala M. S., Cadore E. L., Dorgo S., Izquierdo M., Kraemer W. J., Peterson M. D., et al. (2019). Resistance training for older adults: position statement from the national strength and conditioning association. J. Strength Cond. Res. 33 (8), 2019–2052. doi:10.1519/JSC.0000000000003230
Galbreath M., Campbell B., LaBounty P., Bunn J., Dove J., Harvey T., et al. (2018). Effects of adherence to a higher protein diet on weight loss, markers of health, and functional capacity in older women participating in a resistance-based exercise program. Nutrients 10 (8), 1070. doi:10.3390/nu10081070
Gielen E., Beckwee D., Delaere A., De Breucker S., Vandewoude M., Bautmans I., et al. (2021). Nutritional interventions to improve muscle mass, muscle strength, and physical performance in older people: an umbrella review of systematic reviews and meta-analyses. Nutr. Rev. 79 (2), 121–147. doi:10.1093/nutrit/nuaa011
Gordji-Nejad A., Matusch A., Kleedorfer S., Jayeshkumar Patel H., Drzezga A., Elmenhorst D., et al. (2024). Single dose creatine improves cognitive performance and induces changes in cerebral high energy phosphates during sleep deprivation. Sci. Rep. 14 (1), 4937. doi:10.1038/s41598-024-54249-9
Harris R. C., Soderlund K., Hultman E. (1992). Elevation of creatine in resting and exercised muscle of normal subjects by creatine supplementation. Clin. Sci. (Lond). 83 (3), 367–374. doi:10.1042/cs0830367
Izquierdo M., Merchant R. A., Morley J. E., Anker S. D., Aprahamian I., Arai H., et al. (2021). International exercise recommendations in older adults (ICFSR): expert consensus guidelines. J. Nutr. Health Aging. 25 (7), 824–853. doi:10.1007/s12603-021-1665-8
Jager R., Purpura M., Shao A., Inoue T., Kreider R. B. (2011). Analysis of the efficacy, safety, and regulatory status of novel forms of creatine. Amino Acids 40 (5), 1369–1383. doi:10.1007/s00726-011-0874-6
Kerksick C., Thomas A., Campbell B., Taylor L., Wilborn C., Marcello B., et al. (2009). Effects of a popular exercise and weight loss program on weight loss, body composition, energy expenditure and health in obese women. Nutr. Metab. (Lond). 6, 23. doi:10.1186/1743-7075-6-23
Kerksick C. M., Roberts M. D., Campbell B. I., Galbreath M. M., Taylor L. W., Wilborn C. D., et al. (2020). Differential impact of calcium and vitamin D on body composition changes in post-menopausal women following a restricted energy diet and exercise program. Nutrients 12 (3), 713. doi:10.3390/nu12030713
Kirk B., Cawthon P. M., Arai H., Avila-Funes J. A., Barazzoni R., Bhasin S., et al. (2024). The conceptual definition of sarcopenia: delphi consensus from the global leadership initiative in sarcopenia (GLIS). Age Ageing 53 (3), afae052. doi:10.1093/ageing/afae052
Kley R. A., Tarnopolsky M. A., Vorgerd M. (2013). Creatine for treating muscle disorders. Cochrane Database Syst. Rev. 2013 (6), CD004760. doi:10.1002/14651858.CD004760.pub4
Korovljev D., Stajer V., Ostojic S. M. (2021a). Relationship between dietary creatine and growth indicators in children and adolescents aged 2-19 Years: a cross-sectional study. Nutrients 13 (3), 1027. doi:10.3390/nu13031027
Korovljev D., Todorovic N., Stajer V., Ostojic S. M. (2021b). Food creatine and DXA-derived body composition in boys and girls aged 8 to 19 years. Nutr. Metab. Insights 14, 11786388211059368. doi:10.1177/11786388211059368
Korovljev D., Todorovic N., Stajer V., Ostojic S. M. (2021c). Temporal trends in dietary creatine intake from 1999 to 2018: an ecological study with 89,161 participants. J. Int. Soc. Sports Nutr. 18 (1), 53. doi:10.1186/s12970-021-00453-1
Kraschnewski J. L., Sciamanna C. N., Poger J. M., Rovniak L. S., Lehman E. B., Cooper A. B., et al. (2016). Is strength training associated with mortality benefits? A 15year cohort study of US older adults. Prev. Med. 87, 121–127. doi:10.1016/j.ypmed.2016.02.038
Kreider R. B., Jager R., Purpura M. (2022). Bioavailability, efficacy, safety, and regulatory status of creatine and related compounds: a critical review. Nutrients 14 (5), 1035. doi:10.3390/nu14051035
Kreider R. B., Kalman D. S., Antonio J., Ziegenfuss T. N., Wildman R., Collins R., et al. (2017). International Society of Sports Nutrition position stand: safety and efficacy of creatine supplementation in exercise, sport, and medicine. J. Int. Soc. Sports Nutr. 14, 18. doi:10.1186/s12970-017-0173-z
Kreider R. B., Rasmussen C., Kerksick C. M., Wilborn C., Taylor Lt, Campbell B., et al. (2011). A carbohydrate-restricted diet during resistance training promotes more favorable changes in body composition and markers of health in obese women with and without insulin resistance. Phys. Sportsmed. 39 (2), 27–40. doi:10.3810/psm.2011.05.1893
Kreider R. B., Stout J. R. (2021). Creatine in health and disease. Nutrients 13 (2), 447. doi:10.3390/nu13020447
Magrans-Courtney T., Wilborn C., Rasmussen C., Ferreira M., Greenwood L., Campbell B., et al. (2011). Effects of diet type and supplementation of glucosamine, chondroitin, and MSM on body composition, functional status, and markers of health in women with knee osteoarthritis initiating a resistance-based exercise and weight loss program. J. Int. Soc. Sports Nutr. 8 (1), 8. doi:10.1186/1550-2783-8-8
Martin-Cantero A., Reijnierse E. M., Gill B. M. T., Maier A. B. (2021). Factors influencing the efficacy of nutritional interventions on muscle mass in older adults: a systematic review and meta-analysis. Nutr. Rev. 79 (3), 315–330. doi:10.1093/nutrit/nuaa064
Maughan R. J., Burke L. M., Dvorak J., Larson-Meyer D. E., Peeling P., Phillips S. M., et al. (2018). IOC consensus statement: dietary supplements and the high-performance athlete. Int. J. Sport Nutr. Exerc Metab. 28 (2), 104–125. doi:10.1123/ijsnem.2018-0020
McKeon G., Papadopoulos E., Firth J., Joshi R., Teasdale S., Newby J., et al. (2022). Social media interventions targeting exercise and diet behaviours in people with noncommunicable diseases (NCDs): a systematic review. Internet Interv. 27, 100497. doi:10.1016/j.invent.2022.100497
Ministry of Health LaW (2024). Do not judge it as a drug unless it claims pharmaceutical efficacy. Regarding the handling of “465 ingredient essence (raw materials)” under the food sanitation law Japan. Available at: https://www.mhlw.go.jp/content/11130500/cms_standards103_240515_03.pdf.
Moore D. R. (2014). Keeping older muscle “young” through dietary protein and physical activity. Adv. Nutr. 5 (5), 599S–607S. doi:10.3945/an.113.005405
Olsen S., Aagaard P., Kadi F., Tufekovic G., Verney J., Olesen J. L., et al. (2006). Creatine supplementation augments the increase in satellite cell and myonuclei number in human skeletal muscle induced by strength training. J. Physiol. 573 (Pt 2), 525–534. doi:10.1113/jphysiol.2006.107359
Organization WH, Policy TU-SoNS (2002). Keep fit for life: meeting the nutritional needs of older persons. Geneva, Switzerland: World Health Organization.
Ostojic S. M. (2021a). Nutritional profiles of US adults with suboptimal dietary creatine intake. Ann. Nutr. Metab. 77 (3), 154–158. doi:10.1159/000515916
Ostojic S. M. (2021b). Dietary creatine and kidney function in adult population: NHANES 2017-2018. Food Sci. Nutr. 9 (4), 2257–2259. doi:10.1002/fsn3.2200
Ostojic S. M. (2021c). Dietary creatine intake in U.S. population: NHANES 2017-2018. Nutrition 87-88, 111207. doi:10.1016/j.nut.2021.111207
Ostojic S. M., Forbes S. C. (2022). Perspective: creatine, a conditionally essential nutrient: building the case. Adv. Nutr. 13 (1), 34–37. doi:10.1093/advances/nmab111
Ostojic S. M., Grasaas E., Baltic S., Cvejic J. (2024a). Dietary creatine is associated with lower serum neurofilament light chain levels. Appl. physiology, Nutr. metabolism = Physiologie appliquee, Nutr. metabolisme 49 (8), 1121–1123. doi:10.1139/apnm-2024-0064
Ostojic S. M., Korovljev D., Stajer V. (2021a). Dietary creatine and cognitive function in U.S. adults aged 60 years and over. Aging Clin. Exp. Res. 33 (12), 3269–3274. doi:10.1007/s40520-021-01857-4
Ostojic S. M., Korovljev D., Stajer V. (2021b). Dietary intake of creatine and risk of medical conditions in U.S. older men and women: data from the 2017-2018 National Health and Nutrition Examination Survey. Food Sci. Nutr. 9 (10), 5746–5754. doi:10.1002/fsn3.2543
Ostojic S. M., Stea T. H., Ellery S. J., Smith-Ryan A. E. (2024b). Association between dietary intake of creatine and female reproductive health: evidence from NHANES 2017-2020. Food Sci. Nutr. 12 (7), 4893–4898. doi:10.1002/fsn3.4135
Parise G., Mihic S., MacLennan D., Yarasheski K. E., Tarnopolsky M. A. (1985). Effects of acute creatine monohydrate supplementation on leucine kinetics and mixed-muscle protein synthesis. J. Appl. Physiol. 91 (3), 1041–1047. doi:10.1152/jappl.2001.91.3.1041
Pashayee-Khamene F., Heidari Z., Asbaghi O., Ashtary-Larky D., Goudarzi K., Forbes S. C., et al. (2024). Creatine supplementation protocols with or without training interventions on body composition: a GRADE-assessed systematic review and dose-response meta-analysis. J. Int. Soc. Sports Nutr. 21 (1), 2380058. doi:10.1080/15502783.2024.2380058
Petro J. L., Ferrari G., Cardozo L. A., Vargas-Molina S., Carbone L., Kreider R. B., et al. (2024). Validity of rating of perceived exertion scales in relation to movement velocity and exercise intensity during resistance-exercise: a systematic review. Sports Health. doi:10.1177/19417381241260412
Prokopidis K., Giannos P., Triantafyllidis K. K., Kechagias K. S., Forbes S. C., Candow D. G. (2023). Effects of creatine supplementation on memory in healthy individuals: a systematic review and meta-analysis of randomized controlled trials. Nutr. Rev. 81 (4), 416–427. doi:10.1093/nutrit/nuac064
Ricke E., Dijkstra A., Bakker E. W. (2023). Prognostic factors of adherence to home-based exercise therapy in patients with chronic diseases: a systematic review and meta-analysis. Front. Sports Act. Living 5, 1035023. doi:10.3389/fspor.2023.1035023
Roschel H., Gualano B., Ostojic S. M., Rawson E. S. (2021). Creatine supplementation and brain health. Nutrients 13 (2), 586. doi:10.3390/nu13020586
Safety EHaF (2023). Consultation process on novel food status: creatine monohydrate: directorate-general for health and food safety. Available at: https://food.ec.europa.eu/safety/novel-food/consultation-process-novel-food-status_en.
Solis M. Y., Artioli G. G., Gualano B. (2021). Potential of creatine in glucose management and diabetes. Nutrients 13 (2), 570. doi:10.3390/nu13020570
Stares A., Bains M. (2020). The additive effects of creatine supplementation and exercise training in an aging population: a systematic review of randomized controlled trials. J. Geriatr. Phys. Ther. 43 (2), 99–112. doi:10.1519/JPT.0000000000000222
Todorovic N., Korovljev D., Stajer V., Jorga J., Ostojic S. M. (2023). Creatine consumption and liver disease manifestations in individuals aged 12 years and over. Food Sci. Nutr. 11 (2), 1134–1141. doi:10.1002/fsn3.3151
USFaD A. (2020). “GRN No. 931 Creatine monohydrate,” in Silver spring. Editor F. I. P. Inventories (Maryland: U.S. Department of Health and Human Services), 1–133.
Wallimann T., Tokarska-Schlattner M., Schlattner U. (2011). The creatine kinase system and pleiotropic effects of creatine. Amino Acids 40 (5), 1271–1296. doi:10.1007/s00726-011-0877-3
Xu C., Bi S., Zhang W., Luo L. (2024). The effects of creatine supplementation on cognitive function in adults: a systematic review and meta-analysis. Front. Nutr. 11, 1424972. doi:10.3389/fnut.2024.1424972
Keywords: creatine monohydrate, sarcopenia, nutrition therapy, elder nutritional physiology, resistance training, skeletal muscle, creatine
Citation: Bonilla DA, Stout JR, Candow DG, Jiménez-García JD, Gómez-Miranda LM, Ortiz-Ortiz M, Forbes SC, Ostojic SM, Vargas-Molina S and Kreider RB (2024) The power of creatine plus resistance training for healthy aging: enhancing physical vitality and cognitive function. Front. Physiol. 15:1496544. doi: 10.3389/fphys.2024.1496544
Received: 17 September 2024; Accepted: 18 November 2024;
Published: 03 December 2024.
Edited by:
Ahmad Alkhatib, Birmingham City University, United KingdomReviewed by:
Massimo Negro, Università degli studi di Pavia, ItalyCopyright © 2024 Bonilla, Stout, Candow, Jiménez-García, Gómez-Miranda, Ortiz-Ortiz, Forbes, Ostojic, Vargas-Molina and Kreider. This is an open-access article distributed under the terms of the Creative Commons Attribution License (CC BY). The use, distribution or reproduction in other forums is permitted, provided the original author(s) and the copyright owner(s) are credited and that the original publication in this journal is cited, in accordance with accepted academic practice. No use, distribution or reproduction is permitted which does not comply with these terms.
*Correspondence: Diego A. Bonilla, ZGFib25pbGxhQGRic3MucHJv