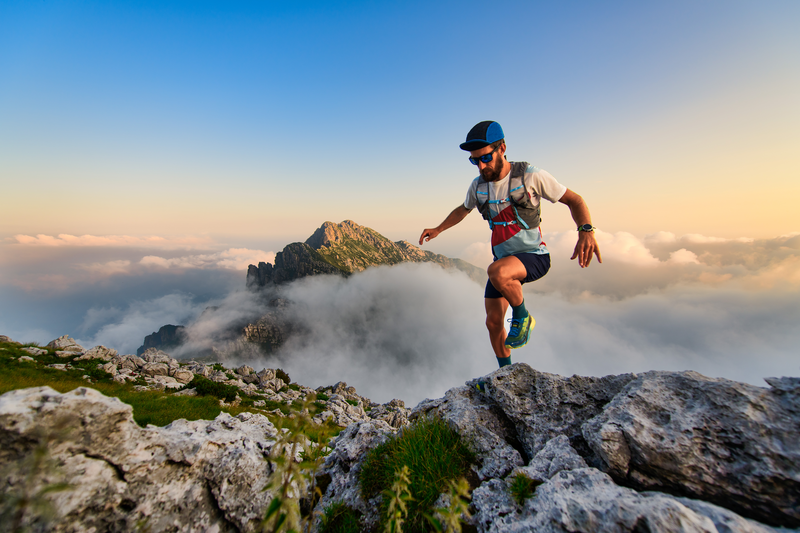
95% of researchers rate our articles as excellent or good
Learn more about the work of our research integrity team to safeguard the quality of each article we publish.
Find out more
ORIGINAL RESEARCH article
Front. Physiol. , 13 December 2024
Sec. Striated Muscle Physiology
Volume 15 - 2024 | https://doi.org/10.3389/fphys.2024.1489439
This article is part of the Research Topic Physiology of Human Myopathies View all 5 articles
Introduction: Adrenergic activation of protein kinase A (PKA) in cardiac muscle targets the sarcolemma, sarcoplasmic reticulum, and contractile apparatus to increase contractile force and heart rate. In the thin filaments of the contractile apparatus, cardiac troponin I (cTnI) Ser22 and Ser23 in the cardiac-specific N-terminal peptide (NcTnI: residues 1 to 32) are the targets for PKA phosphorylation. Phosphorylation causes a 2-3 fold decrease of affinity of cTn for Ca2+ associated with a higher rate of Ca2+ dissociation from cTnC leading to a faster relaxation rate of the cardiac muscle (lusitropy). Cardiomyopathy-linked mutations primarily affect Ca2+ regulation or the PKA-dependent modulatory system, such that Ca2+-sensitivity becomes independent of phosphorylation level (uncoupling) and this could be sufficient to induce cardiomyopathy. A drug that could restore the phosphorylation-dependent modulation of Ca2+-sensitivity could have potential for treatment of these pathologies. We have found that a number of small molecules, including silybin B, resveratrol and EGCG, can restore coupling in single filament assays.
Methods: We did molecular dynamics simulations (5x1500ns for each condition) of the unphosphorylated and phosphorylated cardiac troponin core with the G159D DCM mutation in the presence of the 5 ligands and analysed the effects on several dynamic parameters. We also studied the effect of the ligands on the contractility of cardiac muscle myocytes with ACTC E99K and TNNT2 R92Q mutations in response to dobutamine.
Results: Silybin B, EGCG and resveratrol restored the phosphorylation-induced change in molecular dynamics to wild-type values, whilst silybin A, an inactive isomer of silybin B, and Epicatechin gallate, an EGCG analogue that does not recouple, did not. We analysed the atomic-level changes induced by ligand binding to explain recoupling. Mutations ACTC E99K and TNNT2 R92Q blunt the increased relaxation speed response to β1 adrenergic stimulation of cardiac myocytes and we found that resveratrol, EGCG and silybin B could restore the β1 adrenergic response, whereas silybin A did not.
Discussion: The uncoupling phenomenon caused by cardiomyopathy-related mutations and the ability of small molecules to restore coupling in vitro and lusitropy in myocytes is observed at the cellular, molecular and atomistic levels therefore, restoring lusitropy is a suitable target for treatment. Further research on compounds that restore lusitropy is thus indicated as treatments for genetic cardiomyopathies. Further molecular dynamics simulations could define the specific properties needed for recoupling and allow for the prediction and design of potential new drugs.
In cardiac muscle, contractility is controlled by changes in the concentration of intracellular Ca2+ ion that binds to troponin, a component of the thin filaments, to activate the cyclic contractile interaction of thick and thin filaments. In addition to this Ca2+ switch, cardiac muscle possesses a unique modulatory mechanism that allows the heart to meet increased oxygen demand during exercise.
Release of adrenaline and noradrenaline activates β-1 receptors in cardiac myocytes and leads to activation of adenylyl cyclase via stimulatory G-protein (Gs). The resulting increase of the cytosolic cyclic adenosine monophosphate (cAMP) levels leads to activation of protein kinase A (PKA), which phosphorylates a number of targets in the sarcolemma, sarcoplasmic reticulum, and contractile apparatus to increase contractile force and heart rate. In the thin filaments of the contractile apparatus, cardiac troponin I (cTnI) is the target for PKA phosphorylation and is phosphorylated at residues Ser22 and Ser23 in the cardiac-specific N-terminal peptide (NcTnI: residues 1 to 32). Phosphorylation is coupled to a 2-3 fold decrease of affinity of troponin for Ca2+ due to altered cTnC-cTnI interactions, linked to a higher rate of Ca2+ dissociation from cardiac troponin C (cTnC) (Robertson et al., 1982; Dong et al., 2007; Baryshnikova et al., 2008; Little et al., 2012). The consequent faster relaxation rate of the cardiac muscle due to TnI phosphorylation (lusitropy) is essential for shortening the cardiac muscle relaxation phase of the contraction-relaxation cycle allowing for efficient contraction at a faster heart rate.
In vitro studies have demonstrated that mutations in thin filament proteins that are associated with inherited cardiomyopathies (hypertrophic cardiomyopathy, HCM, and dilated cardiomyopathy, DCM) often abolish the relationship between Ca2+-sensitivity and TnI phosphorylation by PKA and that the mutations also impair lusitropy in many animal models (Marston and Pinto, 2023). This trait, which we have termed ‘uncoupling’, was first noted in 2001 (Deng et al., 2001; Deng et al., 2003). In 2007-9 three publications studying the TNNC1 G159D mutation (G159D), that causes DCM, showed uncoupling with recombinant mutant troponin, with troponin extracted from a patient with the mutation and with rat trabeculae with the mutation exchanged in myocytes (Biesiadecki et al., 2007; Dong et al., 2008; Dyer et al., 2009). Subsequently, almost every thin filament mutation that was tested proved to be uncoupled including the HCM mutations TPM1 E180G, TNNT2 R92Q, and ACTC E99K used in this study (summarized in (Messer and Marston, 2014; Marston and Pinto, 2023)). It is relevant to note that in in vitro experiments, uncoupling is always complete, irrespective of the mutation and that uncoupling is associated with both HCM and DCM-causing mutations. Uncoupling results in blunting of the lusitropic response to β-1 receptor stimulation. Suppression of lusitropy is linked to heart failure phenotypes in animal models, and in clinical practice a reduced response to adrenergic stimulation is correlated with an unfavorable cardiac outcome. (Wilkinson et al., 2015; Waddingham et al., 2018; Marston and Pinto, 2023).
A drug that could restore the phosphorylation-dependent modulation of Ca2+-sensitivity could have potential for treatment of these pathologies. We have investigated small molecules that can specifically reverse these abnormalities in vitro (‘recoupling’). Based on our lead compound, epigallocatechin-3-gallate (EGCG) (Tadano et al., 2010; Papadaki et al., 2015) that is both a desensitiser and a recoupler, we examined 40 compounds and found 23 compounds that reversed the uncoupling. Many of these are pure recouplers (i.e., have no desensitising activity) whilst three of the compounds desensitized but did not recouple and the rest had no effect (Sheehan et al., 2018). Thus recoupling and desensitisation are independent processes. Importantly, recoupling was complete and independent of the causative mutation and the nature of the compound. Small molecules that can act as desensitisers or recouplers have the potential for treatments of HCM or DCM. However we do not know the molecular mechanism of the recoupling phenomenon, nor do we know whether the recoupling compounds we investigated in vitro are effective in intact muscle. In this paper, we aim to answer these questions in vitro, in vivo, and in silico.
Understanding how phosphorylation modulates troponin function is not straightforward since troponin contains several disordered regions that are crucial to regulation and are not accessible to methodologies such as X-ray diffraction or cryoelectron microscopy that only resolve static structures. We recently used molecular dynamics (MD) simulation to determine the atomistic changes that occur when Ser22, 23 of cTnI is phosphorylated in the regulatory core of troponin. These studies showed that phosphorylation induced no significant stable structural changes but rather phosphorylation changed the dynamics of the transitions between a small number of states (Zamora et al., 2016; Yang et al., 2023). We established metrics characteristic of the Ser22/23 phosphorylation transition: changes in the distribution of the angle between helices A and B of troponin C and the angle between the NcTnC domain and the ITC domain that were consistent with phosphorylation destabilising the open state of TnC. Importantly, the introduction of the TNNC1 G159D mutation profoundly changed these metrics. Unphosphorylated G159D tended to have values equivalent to phosphorylated wild-type and upon phosphorylation the values for G159D moved in the opposite direction to wild-type (Yang et al., 2023).
The analysis of MD simulations of G159D troponin clearly shows that the mutation abrogates the effects of phosphorylation on structure and dynamics and thus explains its uncoupling activity. We may also use these metrics to determine the effects of small molecules on the system and to test whether recoupling in vitro corresponds to the restoration of wild-type dynamics in silico.
We analysed molecular dynamics simulations of unphosphorylated and phosphorylated cardiac Troponin core with the G159D mutation in the presence of two pure recouplers, silybin B (SB) and resveratrol, and epigallocatechin-3 gallate (EGCG), a mixed recoupler and desensitiser. These were compared with silybin A (SA), the stereoisomer of silybin B and epicatechin gallate (ECG), closely related to EGCG, that have no measurable recoupling activity in vitro as controls. We found that silybin B, EGCG, and resveratrol restored most key metrics towards wild-type values, whilst silybin A and ECG did not. EGCG gave a complex response consistent with its dual function as recoupler and desensitiser.
In parallel, we have extended our studies to intact cardiomyocytes. Both HCM and DCM-causing mutations that uncouple in vitro blunt the response to β1 adrenergic stimulation in intact cardiomyocytes and cardiac tissue (Marston and Pinto, 2023). We have therefore tested the effects of small molecules, that recouple in vitro, on the response to a β1 adrenergic agonist in myocytes with ACTC E99K and TNNT2 R92Q mutations. We found that resveratrol, EGCG, and silybin B could restore the β1 adrenergic response whilst silybin A did not; in the case of EGCG, the response was partly confounded by off-target effects.
Reagents were obtained from Sigma-Aldrich, except for optically pure silybin A and silybin B prepared by Prof. Vladimir Kren and Dr. David Biedermann (Monti et al., 2010).
The movement of synthetic thin filaments over immobilised myosin was measured by in vitro motility assay as described by (Messer et al., 2016). The fraction motile parameter is plotted here. We measured Ca2+ activation curves for the wild type. TPM1 E180G and TNNC1 G159D troponin-containing thin filaments in the unphosphorylated and phosphorylated states obtained by phosphatase and kinase treatments. A single Ca2+-concentration assay was used as a rapid screen for coupling and recoupling activity. [Ca2+] was 0.0.73µM and [ligand] was 100μM; the method is described in Supplementary Material 1.
Mouse cardiomyocyte isolation protocol and measurement of cell shortening was based on previously described methods using the Ionoptix system (Ionoptix, Milton, MA). Cell contraction was measured using a charge-coupled device video camera (MyoCam-S, IonOptix) connected to a personal computer running IonWizard software (Rowlands et al., 2017). Guinea pig myocyte contractility was measured in the Cytocypher instrument as described by (Wright et al., 2020) using transfected guinea-pig myocytes prepared as described by (Robinson et al., 2018). Incubation medium was Krebs-Hensleit buffer with added 1mM CaCl2, oxygenated with 95% oxygen/5% CO2. The myocytes were incubated at 37° and continuously stimulated at 1Hz. 10 s of contractility was collected and analysed for each cell. Contractility in each dish of myocytes was measured in the absence and then the presence of 0.4 µM dobutamine and 50 nM of the specific β2 antagonist, ICI 118,551. Changes were analysed by a paired t-test.
MD simulations of troponin were performed as described by Yang et al. (Yang et al., 2023). The cTn model used for simulations in this project was based on that constructed and extensively studied in the group (Zamora et al., 2016; Yang et al., 2023). The phosphorylated systems and G159D mutation were created from this model by modifying Ser22, Ser23 of the cTnI protein, and/or Gly159 of cTnC by manually editing the residues. The computational model was prepared in two different phosphorylation wild-type apo states: uP (no phosphorylation) and SEP (cTnI S22/S23 phosphorylated with net charge −2 each). All simulations started at the same structure. Ligands were added to the solvent 10Å away from the troponin surface.
Parameterisation of the ligands in this study was based on a general AMBER force field (GAFF) which is suitable for small organic molecules (Wang et al., 2004). Ligand partial charges were derived from the ground state structures which were found by following conformational search, geometry optimisation, and thermal correction procedures, as detailed below: An ensemble of conformers was generated for each ligand using the ETKDG algorithm as implemented by RDKit (Riniker and Landrum, 2015).
Each conformer was optimised to a local minimum. Each optimised structure was subjected to thermal correction with frequency to calculate the Gibbs free energy (Grimme, 2012; Li et al., 2015; Luchini et al., 2020). The structure with the lowest ground-state energy was picked as the ground-state structure.
From the ground state structures, restrained electrostatic potential (RESP) calculations were used to obtain the charge distribution on the molecule (Bayly et al., 1993). Gaussian16 (Frisch, 2016) was used for both geometry optimisation and RESP calculation. Geometry optimisation was done with B3LYP functional with cc-pVDZ basis set and the polarisable continuum model (PCM) in water as an implicit solvent (Miertuš et al., 1981; Lee et al., 1988; Dunning, 1989; Becke, 1993). RESP calculation was done with the Hartree-Fock method and 6-31G* basis set in a vacuum. (Ditchfield et al., 1971). AmberTools was then used to generate the charge parameters for each atom.
Representative structures, binding energy estimation by MMBPSA, interhelical angle distribution and hinge angle analysis were performed as described by (Yang et al., 2023). CPPTraj was used to determine the contact/interactions. Pytraj (Hai et al., 2016), a Python package binding to cppraj program (Roe and Cheatham, 2013), was used for the distance measurements in this work. The ligand was deemed to be in contact with a residue when the minimal distance between any atom of the ligand and a residue was lower than 2.5 Å. The same was applied to ligand atom interactions where a ligand atom was deemed to be in contact with the protein when the minimal distance between atoms of the ligand and any part of the protein was lower than 2.5 Å.
Ideally, we should measure the effects of small molecule recouplers using the same uncoupling mutation in vitro, in vivo, and in silico. Unfortunately, there is no animal model of the TNNC1 G159D DCM-related mutation that we have used for the molecular dynamics simulations. We, therefore, used two alternative uncoupling mutations for the myocyte experiments: TNNT2 R92Q and ACTC E99K.
In vitro motility measurements of thin filaments containing these mutations have demonstrated that TNNC1 G159D, TNNT2 R92Q, and ACTC E99K, together with several other mutations in thin filament proteins, are uncoupled and that coupling can be restored to all of them by EGCG, silybin B, and resveratrol but not by silybin A, ECG and EGCG (Table 1, Supplementary Material 2; Sheehan et al., 2018). Thus it is reasonable to conclude that the recoupling effect of small molecules, silybin B, EGCG, and resveratrol is independent of the causative mutation and that a full restoration of the effects of troponin I phosphorylation is achieved by all recouplers (Papadaki et al., 2015; Sheehan et al., 2018) summarized in Supplementary Material 2.
Table 1. Effects of TnI PKA phosphorylation on EC50 of wild-type and mutant thin filaments and its modulation by small molecules.
In addition, there is substantial evidence that mutations that uncouple in vitro also cause an impaired response to adrenergic stimulation in intact cardiomyocytes or in the intact heart (Table 2 in Marston and Pinto, 2023) including the three mutations studied here. We therefore propose that TNNC1 G159D, TNNT2 R92Q, and ACTC E99K are functionally equivalent in our assays.
Table 2. Quantification of the effects of phosphorylation and small molecules on A/B angle metrics. Ticks indicate where the wild-type changes in phosphorylation are restored by the small molecules.
In order to assess the effects of EGCG, silybin A, and silybin B on uncoupling in intact cells, we isolated cardiomyocytes from wild-type and two well-characterised models of hypertrophic cardiomyopathy that are uncoupled in vitro due to mutations in a thin filament protein. The models were ACTC E99K transgenic mouse myocytes and guinea-pig cardiomyocytes with the TNNT2 (TnT) R92Q mutation replacing the wild-type protein, introduced by transfection (Song et al., 2011; Messer et al., 2016; Robinson et al., 2018).
The contractility of wild-type and mutant myocytes was measured. We studied three main parameters that are modulated by β1 adrenergic activation: the contractile amplitude (% change in cell length, %L0), the time of contraction (time to 90% peak, ttp90), and the time of relaxation (time to 90% baseline, ttb90), see Figure 1A and Supplementary Material 3. Whilst the contraction amplitude of non-transgenic mouse and guinea-pig myocytes was similar, ttp90 and ttb90 in guinea pig were about double that of mouse, indicating slower kinetics.
Figure 1. (A) Effects of dobutamine on key parameters of cardiac myocyte contractility. Data from experiments where wild-type and mutant cells were measured together. Shortening amplitude, ttp90 and ttb90 parameters are shown before and after addition of dobutamine; mean and Standard error are shown for 40–60 myocytes. Blue- WT and E99K mouse compared. Purple- WT and R92Q guinea-pig compared. The Incubation medium was Krebs-Hensleit buffer with added 1mM CaCl2, oxygenated with 95% oxygen/5% CO2. The myocytes were incubated at 37° and continuously stimulated a 1Hz. 10 s of contractility was collected and analysed for each cell. *, ** indicate significant differences due to adding dobutamine at 0.05 and 0.01 levels respectively using paired t-test. For numerical data and statistical analysis see Supplementary Material 3. (B) Lusitropy and the effect of small molecules measured in cardiomyocytes. Lusitropy is defined as the fractional change of ttb90 due to dobutamine [ 1-(ttb90+Dob/ttb90-Dob)]. Lusitropy (shorter relaxation time) corresponds to a decrease in this parameter. Blue, mouse experiments, Purple, Guinea-pig experiments. Mean, sem and significance in paired t-test is shown as for (A). Lusitropy is lost in the mutant myocytes but is restored by EGCG, Silybin B and resveratrol but not by Silybin A. Numerical data and statistical analysis are presented in Supplementary Material 4.
Cardiomyopathic mutations affected the baseline contractility of cardiomyocytes as previously noted (Song et al., 2011; Robinson et al., 2018). Both ttp90 and ttb90 were greater in ACTC E99K mouse cardiac cells compared to WT cells by 41% and 46% respectively, indicating slower contraction and relaxation whilst contractile amplitude was not different. In the guinea-pig TNNT2 R92Q cells, ttp90 was the same but ttb90 was 35% greater than the wild type (mean of all cells tested) indicating slower relaxation due to the mutation.
The normal response to β1 receptor activation is increased amplitude of shortening and shorter contraction and relaxation times (lusitropy). In contrast, mutations that are observed to be uncoupled in vitro have a blunted response to β1 adrenergic stimulation in intact cardiomyocytes and cardiac tissue (Marston and Pinto, 2023). We confirmed this finding in our preparations (Figure 1A).
We treated cells with the β1-selective agonist dobutamine in the presence of the highly selective β2 receptor antagonist, ICI-118,551. In NTG mouse cells, adding dobutamine increased the amplitude of shortening by 47% and decreased ttb90 by 21%, thus indicating both inotropic and lusitropic effects. In guinea-pig cells, dobutamine did not affect amplitude but ttb90 was decreased by 21% indicating normal lusitropy.
In the mutant cells, we did not observe any significant lusitropy, indicating that β1 adrenergic stimulation and the consequent troponin I phosphorylation were uncoupled from its effect on the rate of relaxation (Supplementary Material 3, Figure 1A). We then tested the ability of small molecules to restore ttb90 to WT levels in the presence of dobutamine.
EGCG, silybin A, silybin B, and resveratrol were examined as potential recoupling compounds. Mouse E99K or guinea-pig R92Q myocytes were incubated in the presence of the small molecules and contractility was measured before and after adding 0.4µM dobutamine. Preliminary titrations indicated that maximal effects of these molecules were achieved with micromolar concentrations, much lower than the concentrations needed for the in vitro motility assays as previously noted (Papadaki et al., 2015; Friedrich et al., 2016). We quantified lusitropy as the fractional change of ttb90 due to dobutamine [ 1- (ttb90 +Dob/ttb90 -dob)]. The baseline lusitropy in NTG mouse or guinea pig was a 20%-24% decrease whilst in the mutant myocytes dobutamine induced a non-significant increase in ttb90 (12% in mouse, 9% in guinea pig).
In the presence of the known recoupling molecules, there was a clear restoration of the lusitropic effect (Figure 1B). In the presence of silybin B, dobutamine reduced ttb90 by 25% in the E99K mouse and 13% in the R92Q guinea pig. In the presence of resveratrol, dobutamine reduced ttb90 by 41% in the E99K mouse and 17% in the R92Q guinea pig. With EGCG, dobutamine reduced ttb90 by 22% in the E99K mouse and reduced ttb90 by 17% in the R92Q guinea pig. In contrast, silybin A, which does not recouple in vitro, did not restore lusitropy; we observed a non-significant 4% increase in ttb90 in E99K mouse and an 18% increase in R92Q guinea pig myocytes which is similar to the effect of dobutamine in the absence of small molecules (9% increase), see Figure 1B and Supplementary Material 4. ECG was not tested in this system. Thus, the small molecules that were shown to be recouplers in vitro also restored the blunted lusitropic response to β1 adrenergic stimulation in intact mutant myocytes. The specificity of this effect is confirmed by the inability of silybin A to restore lusitropy.
To understand how the small molecules modulated troponin behaviour at the atomic level we performed molecular dynamics simulations (5 × 1500 ns) on phosphorylated and unphosphorylated wild-type and TnC G159D DCM mutant troponin in the presence of silybin A, silybin B, EGCG, ECG, and resveratrol (RVL).
In previous molecular dynamics simulations, we demonstrated that phosphorylation of troponin I does not significantly alter the structure of the troponin core. However, phosphorylation does change the dynamics of troponin and the uncoupling mutant TnC G159D. We have derived several metrics that are characteristic of the phosphorylated and unphosphorylated states. In particular, the distribution of the angle between cTnC helices A and B and the angle between quasi-rigid troponin domains (NcTnC and the ITC domain) may be used since the effect of TnI phosphorylation on the metrics of the uncoupling TnC G159D mutation are strikingly different from wild-type with phosphorylation-dependent changes in G159D being mostly in the opposite direction to those in wild-type (Yang et al., 2023). Figure 2B shows the angle distributions comparing WT and G159D troponin whilst Figure 2C shows the effect of small molecules and phosphorylation on the angle distributions for G159D. Corresponding data for ECG is in Supplementary Material 6.
Figure 2. The distribution of a helix A/B and Interdomain angles determined by molecular dynamics simulations. (A) Left:Model defining the location of the hinge between N-terminal and C-terminal domains of troponin C. Right: Model showing the orientation of helices A and B in the N-terminus of troponin C. (B) The effects of the G159D mutation and phosphorylation on the distribution of the interdomain angle and the helix A/B angle. Unphosphorylated, uP, phosphorylated, SEP. (Yang et al., 2023). (C) The effects of phosphorylation and small molecules on the distribution of the interdomain angle (left) and the helix A/B angle (right) For G159D. Tables 2, 3 show the quantification of the distributions. Distribution plots for troponin in the presence of ECG are shown in Supplementary Material 6.
The A/B helix angle may be used as a metric for the opening and closing of the hydrophobic patch (Table 2). In WT, the effect of phosphorylation is a decrease of the mean A/B angle, from 101.8° to 96.1° (∆ = - 5.6°). For the G159D mutant, we observe the complete inverse behaviour: upon phosphorylation, the A/B angle increases from 97.1° to 99.0° (∆ = +1.9°). Comparing the WT and G159D, unphosphorylated A/B angles we see that the WT angle is significantly higher than G159D.
We determined the effect of the small molecule ligands on the interhelix angle distribution in G159D and wild-type troponin (Figure 2; Table 2). In wild-type the ligands tended to have no effect or to increase A/B angle in both unphosphorylated and phosphorylated states, however, the decrease in mean angle upon phosphorylation, associated with a normal response to phosphorylation was preserved. In G159D the effects of the small molecules were different, the three recouplers restored the decrease in A/B angle on phosphorylation (SB, −3.1°, EGCG, −13.3°, RVL, −11.1° compared with −5.6° in the wild-type) whereas, with silybin A, which has no recoupling activity in vitro, the change resembled that of G159D (SA, +4.88°, ECG, −0.2° compared with ApoG159D +1.9°). This pattern of results was also clear when considering the percentage of the area under the curve for the A/B angle above 110°, corresponding to the boundary of the open state of troponin C. With G159D this parameter increased from 1% to 7% on phosphorylation. It increased from 11% to 24% in the presence of silybin A and from 4% to 6% with ECG but with the three recoupling molecules the mean angle decreased (SB, 14 > 9%, EGCG, 11 > 3%, RVL 25 > 1% compared with 18 > 1% in wild-type troponin). Thus the three small molecules that are physiological recouplers restore the phosphorylation-dependent changes in the disease-causing, uncoupled, G159D mutation at the atomic level and silybin A and ECG that are not recouplers, do not restore the A/B angle change.
The principal motion of troponin is a hinge-like motion between the two quasi-rigid domains of troponin, NcTnC, and the ITC domain. The distribution of hinge angles in wild-type troponin shows an increase in the mean angle upon phosphorylation and a lengthening of the TnC peptide link between the domains. In contrast, phosphorylation of G159D shifts the inter-domain angle distribution towards a lower mean angle. Thus the wild-type response to phosphorylation is disrupted by the G159D mutation at the atomic level (Figure 2C; Tables 3, 4).
Table 3. Quantification of the effect of phosphorylation and small molecules on interdomain hinge angle metrics. Ticks indicate where the wild-type changes in phosphorylation are restored by the small molecules.
Table 4. Quantification of the effect of phosphorylation and small molecules on interdomain distance and on the interdomain ionic bond between TnC G/D159 and R83. Ticks indicate where the wild-type changes on phosphorylation are restored by the small molecules.
When the recoupling molecules SB and RVL were added to the G159D simulation, the increase in mean angle upon phosphorylation was restored toward wild-type (SB, +3.58°, RVL, +9.28° compared with WT, +1.65°). In contrast, adding silybin A or ECG to G159D did not reverse the hinge angle change and resembled untreated G159D (SA, −6.4°, ECG -8.3° compared with apoG159D. −5.3°). The effect of EGCG on the G159D hinge angle was anomalous: the mean angle decreased by 7.0° despite EGCG being a recoupling molecule. However, The EGCG angle distribution is bimodal or tri-modal suggesting multiple actions. If just the prominent peak centred on 103° is considered, EGCG may behave like the other recouplers. EGCG is both a recoupler and a desensitiser of actomyosin and this second property, not shared with SB or RVL, may be responsible for the occupancy below 80°. This is supported by the observation that the distribution plot for ECG which is a pure desensitizer is simpler and lacks the 80° component (Supplementary Material 6).
It is also notable that phosphorylation and mutations affect the broadness of the distribution of hinge angles which is reduced on phosphorylation of wild-type but not with G159D. G159D is substantially more rigid than wild type and it was proposed by Yang et al. that the strong bond between TnC R83 and D159 across the hinge played a part here (Yang et al., 2023). It is thus noteworthy that this interaction is substantially weakened by all the ligands binding even though there is no evidence for any ligand binding close to this site, implying an allosteric effect (Table 5).
Table 5. Quantification of the effect of phosphorylation and small molecules on switch peptide of TnI binding to troponin C and ligand binding to troponin. Ligand binding parameters in kcal/mole.
The molecular dynamics simulations allowed us to determine how the small molecules interact with G159D troponin and how this modifies the response to phosphorylation. Figure 3 and Supplementary Material 5 illustrate the preferred solution conformation of the ligands considered in this paper. Silybin B and A are approximately flat; they are stereoisomers with the C ring in opposite orientations. The A, C, and D rings of EGCG have considerable homology with silybin but the orientation of the B ring gives the molecule a propellor-like shape ECG and EGCG have very similar conformations. Resveratrol likewise has some structural homology with silybin and EGCG.
Figure 3. Parameterised solution configurations Silybin A (magenta) silybin B (green), EGCG (yellow) and resveratrol (blue). A and D rings of silybin A and B and A and C rings of EGCG are aligned. See Supplementary Material 5 for identification of the atoms and rings.
MMPBSA was used to calculate ∆G for ligand binding (Table 5). ∆G was in the range 25.5-26.2 kJ/mol for EGCG, ECG, silybin A, and silybin B. ∆G for resveratrol appeared to be lower, consistent with its radically different binding footprint on troponin compared with the other recouplers. For binding to G159D, ∆G did not change much with phosphorylation except for silybin A where binding became significantly weaker on phosphorylation.
We identified the most probable contacts on troponin using the CPPTraj procedures. The plot in Supplementary Material 7A plots the probability of interactions between the ligand and each amino acid of the troponin core. Although it is quantitative, the plot is one-dimensional and gives no idea of the stereochemistry of the interactions. In every case, discrete contact patterns were observed in unphosphorylated G159D that were different for each of the four small molecules and that changed upon phosphorylation as would be expected if they influence the phosphorylation-dependent structural and dynamic changes we have demonstrated.
One way of visualizing the interaction hotspots is to plot all contacts above a threshold (in this case interactions present >10% of the time) on representative models of the troponin core structure, see Supplementary Material 7B, C. Taken together these plots show that ligand binding is phosphorylation-dependent. In general, hotspots tended to be more dispersed after phosphorylation indicating that ligands explore a wider range of interactions compared with unphosphorylated although overall ∆G was not changed. Many of the phosphorylation-dependent hotspots are near the NcTnI-NcTnC interface or the interdomain interface. It is also apparent that ligand interaction can change the conformation of the troponin core, consistent with the angle changes discussed above. Apparent contacts with the tail of troponin (around the TnI helix 1-helix 2 turns and the linker before TnT helix1) are likely to be artefactual since these parts of troponin will be in contact with the rest of the thin filament in vivo (Yamada et al., 2020; Risi et al., 2024).
In these plots there appear to be multiple discrete hotspots; an example of this is silybin B binding to unphosphorylated G159D where the hotspot appears to extend over a large area of the NcTnC surface which would not be possible for a single site given the size of silybin B and, in EGCG binding to unphosphorylated G159D, there are two hotspots on opposite sides of the troponin again not possible to be occupied by EGCG simultaneously. This is most likely due to the time-averaged nature of the CPPTraj calculations whereas, in reality, ligand binding is a dynamic process. The ligand may occupy a number of locations at different times all of which appear in the model.
In order to understand ligand binding it is necessary to study it in 4 dimensions-this can only be done by directly observing the binding trajectories (Figure 4 and Supplementary Material 8). Observation confirms the stochastic nature of ligand binding. A ligand can bind at several discrete locations as was suggested for silybin B and EGCG. In the five 1500 ns runs, the initial locations can be different. Often the ligand goes to one location and stays there for prolonged periods, however, the ligand can move during a run, either by ‘creeping’ across the troponin or more often by dissociation and rebinding. In some cases, the ligand is free for a substantial part of the simulation, most notably with resveratrol (See Table 5).
Figure 4. Ligands bound to unphosphorylated G!59D troponin in their most probable state. (A) Stick representation of the ligand interacting with TnC(light grey) and TnI(blue). TnT is light brown. The ligand is red The molecules are orientated to give the best view of the interaction. For EGCG and SilybinB parts of TnC not involved in ligation are hidden for clarity. (B) Whole troponin surface representations. TnI is blue, TnC is grey and TnT is light blue or cyan. The ligand is red. Single frames are selected from the full trajectory (3750 frames) to illustrate the most common positions of the ligands. Models are orientated to show the ligand binding optimally. Examples of complete trajectories are illustrated in Supplementary Material 8.
We searched for a pattern of contacts that was associated with recoupling. Supplementary Material 8 and Figure 4 show the analysis of selected whole trajectories and highlight those positions where the ligand is often found. Observation suggests for silybin B there are two common positions: at the end of cTroponin, intercalated between the NcTnI peptide and NcTnC A and B helices (site A) and at the ‘top’ of the hinge, interacting with CcTnT and the ‘inhibitory peptide’ (137-148) (site B). Silybin B is bound nearly all the time to unphosphorylated G159D and tends to go to one site or the other. Therefore the time average in Supplementary Material 7B is overwhelmingly a mixture of two separate hotspots. Figure 4 shows a frame from one run where Silybin B occupies site A for most of the 1500ns run. When phosphorylated (SEP), silybin B is almost never at site A. It is often in parts of the tail and CcTnC and sometimes also at the top of hinge like Silybin B site B.
Silybin A also binds primarily near the N terminus of TnI and the helix A-B loop of EF I (Figure 4 and Supplementary Material 8). Unlike Silybin B, Silybin A does not appear to be intercalated between NcTnI and NcTnC but sits on the surface with only the A ring engaged. A secondary site is at the ‘top’ of the interdomain hinge region. All of these contacts are lost on phosphorylation.
In unphosphorylated G159D, EGCG occupies the site intercalated between NcTnI and NcTnC (site A) identified in silybin B part of the time, although it does not use the same set of contacts and there is additional hydrogen bonding to the switch peptide, perhaps associated with its desensitising property (Figure 4 and Supplementary Material 8). None of these sites are occupied when troponin is phosphorylated.
In contrast, ECG primarily occupies a site on the opposite side of NcTnC where it sits on the surface approximately flat with the B ring extending away from troponin (Supplementary Material 5).
Resveratrol binds less frequently to troponin than the others (it was free for 45% of the MD runs see Table 3B) and most often makes contacts with a completely different region of troponin, inserted into the C-terminal domain of troponin C helix E, close to the interdomain interface (Figure 4, Supplementary Material 7, 8).
Lusitropy, the increase in the rate of cardiac muscle relaxation, is an essential component of the heart’s response to adrenergic activation (Marston and Pinto, 2023). It is predominantly mediated by PKA phosphorylation of cardiac troponin I (Kentish et al., 2001; Layland et al., 2005). However in genetic cardiomyopathies due to mutations in thin filament proteins, lusitropy is much reduced and in vitro PKA phosphorylation does not alter Ca2+-sensitivity, the usual measure of the effect of phosphorylation (Messer and Marston, 2014; Marston and Pinto, 2023). Basic and animal studies indicate that specific suppression of lusitropy can induce symptoms of heart failure under stress and clinical studies have shown that lack of response to adrenergic activation is associated with increased adverse cardiac events (Waddingham et al., 2018; Marston and Pinto, 2023). Thus suppression of lusitropy by mutations may be a significant disease mechanism for cardiomyopathy.
Restoration of the lusitropic response is therefore a suitable target for therapy. We have investigated a series of small molecules, acting on troponin, that can restore the phosphorylation-dependent modulation of Ca2+-sensitivity (recoupling) at the single myofilament level as potential treatments (Sheehan et al., 2018; Papadaki et al., 2015). These molecules appeared to be promising since they all gave total restoration in vitro that was independent of the mutation that caused the dysfunction in lusitropy (summarised in Supplementary Material 2). However, it was not established whether these small molecules had any effect on intact muscle and the molecular mechanism by which many different small molecules could cause a single outcome, recoupling, was unknown.
In this study, we have addressed these questions for five small molecules with a range of functions and structures. Silybin B and resveratrol are structurally distinct yet both have a pure recoupling activity in vitro, whilst EGCG is bifunctional, being both a recoupler and a desensitiser. As negative controls, we studied silybin A, the stereoisomer of silybin B, and ECG, a close analogue of EGCG, that has no recoupling activity in vitro.
We tested these small molecules for their ability to restore lusitropy in two previously studied mutant systems. Myocytes from ACTC E99K mice and guinea-pig myocytes transfected with the TNNT2 R92Q mutation were challenged with the β1 specific adrenergic agent dobutamine (Song et al., 2011; Robinson et al., 2018). The results correlated with in vitro results: the mutant myocytes had suppressed lusitropy but wild-type lusitropy could be restored by silybin B, EGCG, and Resveratrol but not by silybin A. This result confirms that recouplers studied in vitro do restore lusitropy, meaning that these small molecules have therapeutic potential.
To understand the molecular mechanism by which silybin B, EGCG, and resveratrol restore lusitropy we extended our molecular dynamics studies of the structure and dynamics of the troponin core that have defined how phosphorylation at cTnI Ser22 and 23 modulates the dynamics of troponin and how the DCM-associated mutation, G159D interferes with this modulation (Zamora, 2019; Yang et al., 2023). Several phosphorylation-dependent metrics may be derived from the MD simulations; the most useful for this study are the change in the distribution of the angle between the A and B helices of cTnC (a measure of the opening and closing of the hydrophobic patch) and changes in the hinge angle between the two quasi rigid domains of troponin (NcTnC and cTnI 1-33 vs. CcTnC/TnT/TnI helices H1 and H2 see (Marston and Zamora, 2020)).
Phosphorylation, in wild-type, causes an increase in the mean A/B helix angle and a reduction in the range of motion (FWHM); phosphorylation decreases the mean hinge angle whilst also reducing the FWHM parameter.
In contrast, phosphorylation of G159D troponin has an opposite effect on most of the metrics compared with wild-type. The G159D mutant troponin has an intrinsically lower A/B helix angle and FWHM than the wild-type and phosphorylation causes an increased mean helix A/B angle whilst there is a decrease in interdomain hinge angle when G159D is phosphorylated. We have proposed that these abnormalities seen in G159D dynamics are causative of the uncoupling effect of the mutation (Yang et al., 2023) and therefore could be used to test for recoupling.
In the presence of the three recoupling compounds the parameters for the A/B helix angle and the interdomain hinge angle of G159D troponin are largely restored to wild-type values (See Figure 5 for a summary), thus recoupling involves restoration of wild-type dynamic behaviour in troponin at the atomic level. It is perhaps relevant that the strong ionic and H bonding between the mutant amino acid cTnC D159 and R83 across the interdomain interface thought to be responsible for the reduced range of hinge motion in the mutant, is much reduced by ligand binding. It has been noted that ligands may occupy multiple sites only one of which may be relevant to recoupling and also that the ligand is not always bound, especially with resveratrol (Table 3). These factors may obscure the full effects of the ligands on the MD metrics.
Importantly, silybin A and ECG that do not recouple do not alter the phosphorylation-dependent metrics of G159D significantly, confirming the specific effect of the recouplers. It is notable that silybin B, EGCG, and resveratrol affect the parameters for unphosphorylated G159D with little effect on phosphorylated G159D in parallel with the in vitro motility measurements that also show that G159D behaves like phosphorylated wild-type independent of phosphorylation and the small molecules primarily alter the properties of unphosphorylated mutant troponin.
Figure 5. Comparison of the effect of phosphorylation of cardiac troponin on its biochemical, physiological and molecular dynamics parameters, its suppression by the TnC G159D DCM-related mutation and its restoration by small molecules. Parameters compared are lusitropy in intact myocytes (from Figure 1B), Fixed [Ca2+] screen for coupling in thin filaments measured by IVMA from (Sheehan et al., 2018) and Supplementary Material 1 and the effect of phosphorylation on EC50, (EC50P/EC50uP) in thin filaments measured by IVMA (from Table 1), Change on A/B angle on phosphorylation and change in hinge angle upon phosphorylation from Molecular dynamics simulations (from Figure 2; Tables 2, 3). The recouplers are highlighted in blue.
Understanding how the recouplers function cannot be precise because of the disordered nature of troponin in the regions where the ligands contact and the weak, stochastic binding of the ligands. Nevertheless, the ligands do satisfy the basic criterion of allosteric effectors since they bind differently to unphosphorylated and phosphorylated G159D troponin. There is no significant change in binding energetics of the ligands to unphosphorylated and phosphorylated troponin, instead, we observe that ligands are concentrated on a few ‘hotspots’ in unphosphorylated troponin, that are not occupied in phosphorylated troponin and that the ‘hotspots’ are more dispersed in phosphorylated troponin. We have concentrated our analysis on the effects of the ligands on unphosphorylated G159D troponin. The most common poses of bound silybin B and EGCG are found to be intercalated between the disordered N terminus of cTnI, adjacent to the phosphorylatable serines 22 and 23, and the helix A and B region of TnC. Since these poses are only seen in unphosphorylated troponin it is feasible that their occupancy is the cause of recoupling. Compatible with this hypothesis is the observation that silybin A binds to roughly the same region of NcTnI but is not intercalated between TnI and TnC, presumably because the stereoisomer does not fit the space. Similarly, ECG binds predominantly to the surface of cTnC at a site only rarely occupied by EGCG.
Our study included resveratrol since it was functionally identical to silybin B in single filament and myocyte assays but had a radically different chemical structure. For 45% of the MD runs resveratrol is unbound (Table 5) and its contacts with unphosphorylated troponin are also very different from silybin B and EGCG. In unphosphorylated troponin it appears that resveratrol is mostly located near helix E in the C terminal domain of cTnC near the hinge interface, possibly intercalated between the two domains. As with the other recouplers, binding is reduced and dispersed on phosphorylation. Therefore, we cannot propose a single mechanism for recoupling; a study of a wider range of recouplers would be needed to attempt to resolve the question.
A primary aim of this study was to demonstrate that the uncoupling phenomenon caused by cardiomyopathy-related mutations and the ability of small molecules to restore coupling, first found with pure thin filaments in vitro, is also relevant at the cellular and atomistic levels. Figure 5 summarises our results that support this proposition. Uncoupling is manifested at the myocyte level as an insensitivity of relaxation rate to β adrenergic stimulation and at the atomistic level as the failure of phosphorylation to increase interdomain angle or decrease A/B angle. It is evident that silybin B, resveratrol, and EGCG restore the native parameters to the mutant troponin whilst silybin A and ECG do not, as predicted.
We have argued that loss of lusitropy is a disease mechanism contributing to cardiomyopathy (Marston and Pinto, 2023) so restoring lusitropy is a suitable target for treatment. EGCG has been demonstrated to relieve symptoms of HCM in mice and paediatric patients (Quan et al., 2019). Silybin B also has the potential to play a role in safeguarding the heart against cardiomyopathy and silybin A does not compromise this activity (Sheehan et al., 2018). Racemic silybin is a readily available and safe nutraceutical, as is resveratrol (Křen and Valentová, 2022). Further research on these compounds as treatments to restore lusitropy is thus indicated.
The compounds studied here are all polyphenols that may have multiple pharmacological activities that render them unsuitable for specific treatments (Baell and Walters, 2014; Ingólfsson et al., 2014; Křen and Valentová, 2022). However, at least 23 small molecules have been shown to be recouplers. Further work on these in silico could define the specific properties needed for recoupling and allow for the prediction and design of new molecules that recouple without additional activities that could be used in the treatment of genetic cardiomyopathies.
The raw data supporting the conclusions of this article will be made available by the authors, without undue reservation.
The animal studies were approved by Guinea pig cells: The investigation was approved by the Animal Welfare and Ethical Review Board at the University of Oxford and conforms to the UK Animals (Scientific Procedures) Act, 1986, incorporating Directive 2010/63/EU of the European Parliament. Mouse cells: Experiments and animal handling were done in accordance with Imperial College guidelines. Mice were euthanized by cervical dislocation as required by Schedule I of the United Kingdom Animals (Scientific Procedures) Act 1986. The studies were conducted in accordance with the local legislation and institutional requirements. Written informed consent was obtained from the owners for the participation of their animals in this study.
ZY: Data curation, Formal Analysis, Investigation, Methodology, Software, Validation, Visualization, Writing–review and editing. AMS: Data curation, Formal Analysis, Investigation, Methodology, Validation, Visualization, Writing–review and editing. AM: Conceptualization, Data curation, Formal Analysis, Investigation, Methodology, Project administration, Supervision, Writing–review and editing. ST: Data curation, Formal Analysis, Investigation, Methodology, Writing–review and editing. AS: Data curation, Formal Analysis, Investigation, Methodology, Resources, Visualization, Writing–review and editing. CR: Conceptualization, Funding acquisition, Investigation, Methodology, Project administration, Resources, Supervision, Writing–review and editing. VK: Conceptualization, Formal Analysis, Resources, Writing–review and editing. IG: Conceptualization, Data curation, Formal Analysis, Funding acquisition, Investigation, Methodology, Project administration, Resources, Software, Supervision, Writing–original draft, Writing–review and editing. SM: Conceptualization, Data curation, Formal Analysis, Funding acquisition, Investigation, Methodology, Project administration, Resources, Supervision, Validation, Visualization, Writing–original draft, Writing–review and editing.
The author(s) declare that financial support was received for the research, authorship, and/or publication of this article. SM was supported by British Heart Foundation programme grant (RG/11/20/29266). AMS has a Ph.D. studentship funded by the BHF Centre of Research Excellence (RE/13/4/30184). ZY is supported by an awarded Ph.D. studentship from the Welcome Trust grant number 108908/Z/15/Z A 4-year PhD in theoretical systems biology and bioinformatics. VK is supported by the project of the Ministry of Education, Sports, and Youth of the Czech Republic (MEYS) “Talking microbes-understanding microbial interactions within One Health framework” (CZ.02.01.01/00/22_008/0004597) CR and AS are supported by the British Heart Foundation Grant PG/18/68/33883.
The authors declare that the research was conducted in the absence of any commercial or financial relationships that could be construed as a potential conflict of interest.
All claims expressed in this article are solely those of the authors and do not necessarily represent those of their affiliated organizations, or those of the publisher, the editors and the reviewers. Any product that may be evaluated in this article, or claim that may be made by its manufacturer, is not guaranteed or endorsed by the publisher.
The Supplementary Material for this article can be found online at: https://www.frontiersin.org/articles/10.3389/fphys.2024.1489439/full#supplementary-material
G159D, troponin with G159D mutation in troponin C; SA, SB, silybin A, silybin B; DHSA, DHSB, dehydrosilybin A, dehydrosilybin B; EGCG, epigallocatechin-3-gallate; ECG, epicatechin gallate; MD, molecular dynamics; FWHM, Full width at half maximum.
Baell J., Walters M. A. (2014). Chemistry: Chemical con artists foil drug discovery. Nature 513 (7519), 481–483. doi:10.1038/513481a
Baryshnikova O. K., Li M. X., Sykes B. D. (2008). Modulation of cardiac troponin C function by the cardiac-specific N-terminus of troponin I: influence of PKA phosphorylation and involvement in cardiomyopathies. J. Mol. Biol. 375 (3), 735–751. doi:10.1016/j.jmb.2007.10.062
Bayly C. I., Cieplak P., Cornell W., Kollman P. A. (1993). A well-behaved electrostatic potential based method using charge restraints for deriving atomic charges: the RESP model. J. Phys. Chem. 97 (40), 10269–10280. doi:10.1021/j100142a004
Becke A. D. (1993). Density-functional thermochemistry. III. The role of exact exchange. J. Chem. Phys. 98 (7), 5648–5652. doi:10.1063/1.464913
Biesiadecki B. J., Kobayashi T., Walker J. S., John Solaro R., de Tombe P. P. (2007). The troponin C G159D mutation blunts myofilament desensitization induced by troponin I Ser23/24 phosphorylation. Circ. Res. 100 (10), 1486–1493. doi:10.1161/01.RES.0000267744.92677.7f
Deng Y., Schmidtmann A., Kruse S., Filatov V., Heilmeyer L. M., Jaquet K., et al. (2003). Phosphorylation of human cardiac troponin I G203S and K206Q linked to familial hypertrophic cardiomyopathy affects actomyosin interaction in different ways. J. Mol. Cell Cardiol. 35 (11), 1365–1374. doi:10.1016/j.yjmcc.2003.08.003
Deng Y., Schmidtmann A., Redlich A., Westerdorf B., Jaquet K., Thieleczek R. (2001). Effects of phosphorylation and mutation R145G on human cardiac troponin I function. Biochemistry 40 (48), 14593–14602. doi:10.1021/bi0115232
Ditchfield R., Hehre W. J., Pople J. A. (1971). Self-consistent molecular-orbital methods. IX. An extended Gaussian-type basis for molecular-orbital studies of organic molecules. J. Chem. Phys. 54 (2), 724–728. doi:10.1063/1.1674902
Dong W., Xing J., Ouyang Y., An J., Cheung H. C. (2008). Structural kinetics of Cardiac troponin C mutants linked to familial hypertrophic and dilated cardiomyopathy in troponin complexes. J. Biol. Chem. 283 (6), 3424–3432. doi:10.1074/jbc.M703822200
Dong W. J., Jayasundar J. J., An J., Xing J., Cheung H. C. (2007). Effects of PKA phosphorylation of cardiac troponin I and strong crossbridge on conformational transitions of the N-domain of cardiac troponin C in regulated thin filaments. Biochemistry 46, 9752–9761. doi:10.1021/bi700574n
Dunning T. H. (1989). Gaussian basis sets for use in correlated molecular calculations. I. The atoms boron through neon and hydrogen. J. Chem. Phys. 90 (2), 1007–1023. doi:10.1063/1.456153
Dyer E., Jacques A., Hoskins A., Ward D., Gallon C., Messer A., et al. (2009). Functional analysis of a unique troponin C mutation, Gly159Asp that causes familial dilated cardiomyopathy, studied in explanted heart muscle. Circ. Heart Fail 2, 456–464. doi:10.1161/CIRCHEARTFAILURE.108.818237
Friedrich F. W., Flenner F., Nasib M., Eschenhagen T., Carrier L. (2016). Epigallocatechin-3-Gallate accelerates relaxation and Ca2+ transient decay and desensitizes myofilaments in healthy and mybpc3-targeted knock-in cardiomyopathic mice. Front. Physiol. 7, 607–212. doi:10.3389/fphys.2016.00607
Grimme S. (2012). Supramolecular binding thermodynamics by dispersion-corrected density functional theory. Chem. Eur. J. 18 (32), 9955–9964. doi:10.1002/chem.201200497
Hai N., Dr R., J S., Case D. A. (2016). PYTRAJ v1.0.0.dev1: interactive data analysis for molecular dynamics simulations. Zenodo. doi:10.5281/zenodo.44612
Ingólfsson H. I., Thakur P., Herold K. F., Hobart E. A., Ramsey N. B., Periole X., et al. (2014). Phytochemicals perturb membranes and promiscuously alter protein function. ACS Chem. Biol. 9 (8), 1788–1798. doi:10.1021/cb500086e
Kentish J. C., McCloskey D. T., Layland J., Palmer S., Leiden J. M., Martin A. F., et al. (2001). Phosphorylation of troponin I by protein kinase A accelerates relaxation and crossbridge cycle kinetics in mouse ventricular muscle. Circ. Res. 88 (10), 1059–1065. doi:10.1161/hh1001.091640
Křen V., Valentová K. (2022). Silybin and its congeners: from traditional medicine to molecular effects. Nat. Prod. Rep. 39 (6), 1264–1281. doi:10.1039/d2np00013j
Layland J., Solaro R. J., Shah A. M. (2005). Regulation of cardiac contractile function by troponin I phosphorylation. Cardiovasc Res. 66 (1), 12–21. doi:10.1016/j.cardiores.2004.12.022
Lee C., Yang W., Parr R. G. (1988). Development of the Colle-Salvetti correlation-energy formula into a functional of the electron density. Phys. Rev. B 37 (2), 785–789. doi:10.1103/physrevb.37.785
Li Y.-P., Gomes J., Sharada S. M., Bell A. T., Head-Gordon M. (2015). Improved force-field parameters for QM/MM simulations of the energies of adsorption for molecules in zeolites and a free rotor correction to the rigid rotor harmonic oscillator model for adsorption enthalpies. J. Phys. Chem. C 119 (4), 1840–1850. doi:10.1021/jp509921r
Little S. C., Biesiadecki B. J., Kilic A., Higgins R. S. D., Janssen P. M. L., Davis J. P. (2012). The rates of Ca2+ dissociation and cross-bridge detachment from ventricular myofibrils as reported by a fluorescent cardiac Troponin C. J. Biol. Chem. 287, 27930–27940. doi:10.1074/jbc.M111.337295
Luchini G., Alegre-Requena J. V., Funes-Ardoiz I., Paton R. S. (2020). GoodVibes: automated thermochemistry for heterogeneous computational chemistry data. F1000Research 9 (Chem Inf Sci), 291. doi:10.12688/f1000research.22758.1
Marston S., Pinto J. R. (2023). Suppression of lusitropy as a disease mechanism in cardiomyopathies. Front. Cardiovasc Med. 9, 1080965. doi:10.3389/fcvm.2022.1080965
Marston S., Zamora J. E. (2020). Troponin structure and function: a view of recent progress. J. Muscle Res. Cell Motil. 41 (1), 71–89. doi:10.1007/s10974-019-09513-1
Messer A., Marston S. (2014). Investigating the role of uncoupling of Troponin I phosphorylation from changes in myofibrillar Ca2+-sensitivity in the pathogenesis of Cardiomyopathy. Front. Physiol. 5, 315. doi:10.3389/fphys.2014.00315
Messer A. E., Bayliss C. R., El-Mezgueldi M., Redwood C. S., Ward D. G., Papadaki M., et al. (2016). Mutations in troponin T associated with Hypertrophic Cardiomyopathy increase Ca2+-sensitivity and suppress the modulation of Ca2+-sensitivity by troponin I phosphorylation. Arch. Biochem. Biophys. 601, 113–120. doi:10.1016/j.abb.2016.03.027
Miertuš S., Scrocco E., Tomasi J. (1981). Electrostatic interaction of a solute with a continuum. A direct utilizaion of ab initio molecular potentials for the prevision of solvent effects. Chem. Phys. 55 (1), 117–129. doi:10.1016/0301-0104(81)85090-2
Monti D., Gažák R., Marhol P., Biedermann D., Purchartová K. i., Fedrigo M., et al. (2010). Enzymatic kinetic resolution of silybin diastereoisomers. J. Nat. Prod. 73 (4), 613–619. doi:10.1021/np900758d
Papadaki M. (2015). The importance of uncoupling of troponin I phosphorylation from Ca2+ sensitivity in the pathogenesis of cardiomyopathy. PhD, Imp. Coll. Lond. doi:10.25560/29755
Papadaki M., Vikhorev P. G., Marston S. B., Messer A. E. (2015). Uncoupling of myofilament Ca2+ sensitivity from troponin I phosphorylation by mutations can be reversed by epigallocatechin-3-gallate. Cardiovasc Res. 108 (1), 99–110. doi:10.1093/cvr/cvv181
Quan J., Jia Z., Lv T., Zhang L., Liu L., Pan B., et al. (2019). Green tea extract catechin improves cardiac function in pediatric cardiomyopathy patients with diastolic dysfunction. J. Biomed. Sci. 26 (1), 32. doi:10.1186/s12929-019-0528-7
Riniker S., Landrum G. A. (2015). Better informed distance geometry: using what we know to improve conformation generation. JCTC 55 (12), 2562–2574. doi:10.1021/acs.jcim.5b00654
Risi C. M., Belknap B., Atherton J., Coscarella I. L., White H. D., Chase P. B., et al. (2024). Troponin structural dynamics in the native cardiac thin filament revealed by cryo electron microscopy. J. Mol. Biol. 436 (6), 168498. doi:10.1016/j.jmb.2024.168498
Robertson S. P., Johnson J. D., Holroyde M. J., Kranias E. G., Potter J. D., Solaro R. J. (1982). The effect of troponin I phosphorylation on the Ca2+-binding properties of the Ca2+-regulatory site of bovine cardiac troponin. J. Biol. Chem. 257, 260–263. doi:10.1016/s0021-9258(19)68355-9
Robinson P., Liu X., Sparrow A., Patel S., Zhang Y. H., Casadei B., et al. (2018). Hypertrophic cardiomyopathy mutations increase myofilament Ca2+ buffering, alter intracellular Ca2+ handling, and stimulate Ca2+-dependent signaling. J. Biol. Chem. 293 (27), 10487–10499. doi:10.1074/jbc.RA118.002081
Roe D. R., Cheatham T. E. (2013). PTRAJ and CPPTRAJ: software for processing and analysis of molecular dynamics trajectory data. J. Chem. Theory Comput. 9 (7), 3084–3095. doi:10.1021/ct400341p
Rowlands C., Owen T., Lawal S., Cao S., Pandey S., Yang H. Y., et al. (2017). Age and strain related aberrant Ca(2+) release is associated with sudden cardiac death in the ACTC E99K mouse model of hypertrophic cardiomyopathy. Am. J. Physiol. Heart Circ. Physiol. 313, H1213-H1226–H1226. doi:10.1152/ajpheart.00244.2017
Sheehan A., Messer A. E., Papadaki M., Choudhry A., Kren V., Biedermann D., et al. (2018). Molecular defects in cardiac myofilament Ca2+-regulation due to cardiomyopathy-linked mutations can Be reversed by small molecules binding to troponin. Front. Physiol. 9 (243), 243–312. doi:10.3389/fphys.2018.00243
Sheehan A. M. (2019). Modulation of cardiac muscle contractility by phosphorylation, HCM and DCM causing mutations and small molecules. PhD, Imp. Coll. Lond. doi:10.25560/79512
Song W., Dyer E., Stuckey D., Copeland O., Leung M., Bayliss C., et al. (2011). Molecular mechanism of the E99K mutation in cardiac actin (ACTC Gene) that causes apical hypertrophy in man and mouse. J. Biol. Chem. 286 (31), 27582–27593. doi:10.1074/jbc.M111.252320
Tadano N., Du C., Yumoto F., Morimoto S., Ohta M., Xie M., et al. (2010). Biological actions of green tea catechins on cardiac troponin C. Brit J. Pharmacol. 161 (5), 1034–1043. doi:10.1111/j.1476-5381.2010.00942.x
Waddingham P. H., Bhattacharyya S., Zalen J. V., Lloyd G. (2018). Contractile reserve as a predictor of prognosis in patients with non-ischaemic systolic heart failure and dilated cardiomyopathy: a systematic review and meta-analysis. Echo Res. Pract. 5 (1), 1–9. doi:10.1530/ERP-17-0054
Wang J., Wolf R., Caldwell J., Kollman P., Case D. A. (2004). Development and testing of a general amber force field. J. Comput. Chem. 25 (9), 1157–1174. doi:10.1002/jcc.20035
Wilkinson R., Song W., Smoktunowicz N., Marston S. (2015). A dilated cardiomyopathy mutation blunts adrenergic response and induces contractile dysfunction under chronic angiotensin II stress. Am. J. Physiol. H&C 309 (11), H1936–H1946. doi:10.1152/ajpheart.00327.2015
Wright P. T., Tsui S. F., Francis A. J., MacLeod K. T., Marston S. B. (2020). Approaches to high-throughput analysis of cardiomyocyte contractility. Front. Physiol. 11, 612. doi:10.3389/fphys.2020.00612
Yamada Y., Namba K., Fujii T. (2020). Cardiac muscle thin filament structures reveal calcium regulatory mechanism. Nat. Comm. 11 (1), 153. doi:10.1038/s41467-019-14008-1
Yang Z., Marston S. B., Gould I. R. (2023). Modulation of structure and dynamics of cardiac troponin by phosphorylation and mutations revealed by molecular dynamics simulations. J. Phys. Chem. B 127 (41), 8736–8748. doi:10.1021/acs.jpcb.3c02337
Zamora J. E. (2019). Investigating cardiomyopathies with atomistic simulations of cardiac Troponin. Imperial College London. PhD. doi:10.25560/78681
Keywords: cardiomyopathy, lusitropy, PKA phosphorylation, troponin (cTnI), myocyte contraction, molecular dynamics simulation, EGCG, silybin
Citation: Yang Z, Sheehan AM, Messer AE, Tsui S, Sparrow A, Redwood C, Kren V, Gould IR and Marston SB (2024) Nutraceuticals silybin B, resveratrol, and epigallocatechin-3 gallate-bind to cardiac muscle troponin to restore the loss of lusitropy caused by cardiomyopathy mutations in vitro, in vivo, and in silico. Front. Physiol. 15:1489439. doi: 10.3389/fphys.2024.1489439
Received: 01 September 2024; Accepted: 06 November 2024;
Published: 13 December 2024.
Edited by:
Aldrin V. Gomes, University of California, Davis, United StatesReviewed by:
Martina Krüger, Heinrich Heine University of Düsseldorf, GermanyCopyright © 2024 Yang, Sheehan, Messer, Tsui, Sparrow, Redwood, Kren, Gould and Marston. This is an open-access article distributed under the terms of the Creative Commons Attribution License (CC BY). The use, distribution or reproduction in other forums is permitted, provided the original author(s) and the copyright owner(s) are credited and that the original publication in this journal is cited, in accordance with accepted academic practice. No use, distribution or reproduction is permitted which does not comply with these terms.
*Correspondence: Steven B. Marston, cy5tYXJzdG9uQGltcGVyaWFsLmFjLnVr
†ORCID: Alexander Sparrow, orcid.org/0000-0002-3354-3314; Charles Redwood, orcid.org/0000-0002-8673-7542; Vladimir Kren, orcid.org/0000-0002-1091-4020; Ian R. Gould, orcid.org/0000-0003-3559-0234; Steven B. Marston, orcid.org/0000-0001-6054-6070
Disclaimer: All claims expressed in this article are solely those of the authors and do not necessarily represent those of their affiliated organizations, or those of the publisher, the editors and the reviewers. Any product that may be evaluated in this article or claim that may be made by its manufacturer is not guaranteed or endorsed by the publisher.
Research integrity at Frontiers
Learn more about the work of our research integrity team to safeguard the quality of each article we publish.