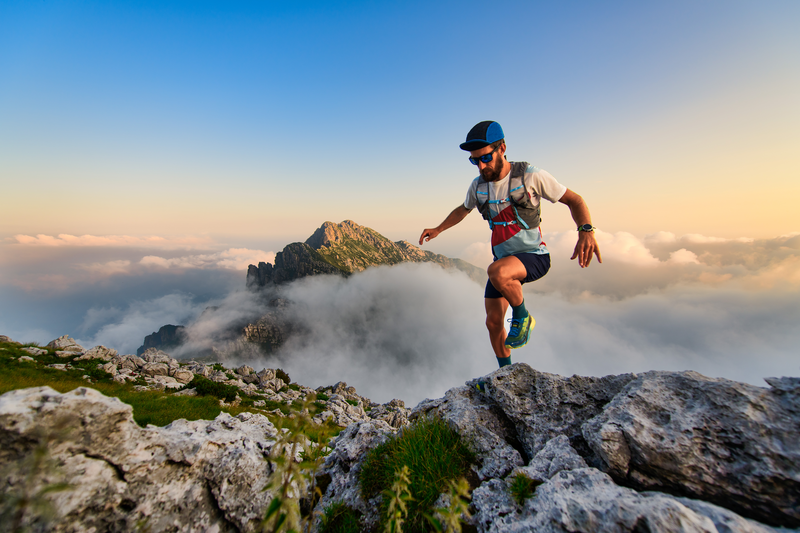
95% of researchers rate our articles as excellent or good
Learn more about the work of our research integrity team to safeguard the quality of each article we publish.
Find out more
ORIGINAL RESEARCH article
Front. Physiol. , 05 November 2024
Sec. Vascular Physiology
Volume 15 - 2024 | https://doi.org/10.3389/fphys.2024.1471272
This article is part of the Research Topic Decoding Vascular Aging: Unraveling the Enigma of Pathological Conditions & Pre-mature Vascular Aging View all 7 articles
Elevated plasma levels of oxidized low-density lipoprotein (oxLDL) are a risk factor and key component that accelerates and worsens cardiovascular disease fueling inflammation, plaque buildup and vascular damage. OxLDL can elicit its detrimental action via lectin-like oxLDL receptor 1 (LOX-1). In this study, we determined whether oxLDL, via LOX-1, alters aortic vascular reactivity and determined if sex and age differences exist. Thoracic aortic endothelium-intact or -denuded ring segments were isolated from 7 to 12 months old intact C57BL/6J female and male mice and pre-incubated with oxLDL ex vivo (50ug/dL; 2 h). Using wire myography, cumulative concentration-response curves to phenylephrine (PE) were generated to determine contractile responses. From these curves, the EC50 was determined and used to contract rings to assess acetylcholine (ACh) dependent relaxation. Calculated aortic stiffness and remodeling were also assessed. BI-0115 (10
Elevated oxidized low-density lipoprotein (oxLDL) is a risk factor and key factor in the development of vascular pathogenesis associated with atherosclerosis and stroke (Trpkovic et al., 2015; Xu et al., 2022). The rising prevalence of dyslipidemia, which affects not only aged populations (Liu et al., 2023) but also younger cohorts due to increased prevalence of poor diet and lack of exercise, has sparked interest in better understanding the molecular and functional effects of elevated oxLDL plasma levels on the vascular wall. One of the preceding indications of development of oxLDL-induced pathology is augmented endothelial dysfunction which can ultimately predispose vessels to structural wall changes (Gimbrone and García-Cardeña, 2016; Ross, 1993). A defining feature of oxLDL-mediated processes, closely linked to endothelial dysfunction, is oxidative stress (Valente et al., 2014). Mechanisms associated with the complex regulation of redox balance by oxLDL has emerged as a pivotal focus in the study of cardiovascular diseases. This is further convoluted by the addition of inflammation which has also been investigated in the context of atherosclerosis, aneurysmal formation, and stroke, and demonstrates the intricate mechanisms through which oxLDL may impact vascular function and health.
OxLDL is a byproduct of lipid peroxidation and exerts its effects on a number of cell types including endothelial cells, platelets, macrophages, fibroblasts, and smooth muscle cells through lectin-like oxLDL receptor 1 (LOX-1) (Pirillo et al., 2013; Truthe et al., 2024). LOX-1 is described as a type II integral membrane glycoprotein receptor that was initially cloned from bovine aortic endothelial cells and human lung by Sawamura et al. (1997). In addition to oxidative stress and inflammation, oxLDL/LOX-1 receptor activation has been shown to elicit the upregulation of signaling pathways that can impact vascular reactivity. (Blair et al., 1999; Cominacini et al., 2001; Ryoo et al., 2006; Ryoo et al., 2011; Xu et al., 2007; Li and Mehta, 2000; Ou et al., 1985; Li and Renier, 2009). For example, oxLDL/LOX-1 receptor activation can contribute to endothelin-1-mediated vasoconstriction. Binding of oxLDL to LOX-1 can also trigger the impairment of endothelial dependent relaxation through decreased nitric oxide (NO) bioavailability and increased reactive oxygen species production [Reviewed by Pirillo et al. (2013)] Ryoo et al. (2006) and Gradinaru et al. (2015). NO is crucial for maintaining vascular tone, and reduction in NO production and/or bioavailability leads to impaired vascular relaxation and contributes to increased vascular resistance leading to endothelial dysfunction (Ryoo et al., 2011; Jiang et al., 2001; Pandey et al., 2014; Tanaka et al., 2009). Together this indicates a critical link between oxLDL, and possibly LOX-1, and vascular pathologies (Ryoo et al., 2011; Jiang et al., 2001; Pandey et al., 2014; Tanaka et al., 2009) which can in turn predispose vessels to structural wall modifications (Ross, 1993). These cascades of events are central to the development of vascular pathologies such as atherosclerosis, aortic aneurysms and the involvement in the acute response to ischemic injury such as stroke.
The oxLDL/LOX-1 pathway plays a complex multimodal role in regulating not only vascular tone and reactivity, but vessel wall integrity and structure. LOX-1 deficiency has been associated with the thinning of adventitial collagen, potentially contributing to increased susceptibility to ruptured aortic aneurysms (Takahashi et al., 2023). It has also been found that LOX-1 plays a key role in the proliferation of cardiac fibroblasts leading to cardiac remodeling (Liu et al., 2016), which has concomitantly been suggested to occur within the vasculature as oxLDL contributes to endothelial dysfunction (Galle et al., 2006). Although these studies provide evidence that LOX-1 plays a pivotal role in altering vessel structural integrity, they underscore the need for further investigation into the mechanisms driving aortic reactivity, stiffness, and remodeling in response to oxLDL-mediated LOX-1 signaling during both physiological and pathological conditions.
OxLDL induced endothelial dysfunction can be precipitated by factors including diet, biological sex, and aging. OxLDL/LOX-1 activation leads to increased oxidative stress and inflammation, significant contributors to vascular dysfunction and aging making LOX-1 a potential therapeutic strategy for the prevention and treatment of CVD (Barreto et al., 2021; Munno et al., 2024). Although fewer studies have examined the influence of sex on LOX-1 expression and regulation under physiological and pathological conditions compared to aging studies (Ogola et al., 2022), its posited that vascular oxLDL/LOX-1 levels or activity may be affected by biological sex. Long-standing studies have reported that estrogen exerts a protective effect against oxidative stress [Reviewed by Khalil (2005)] which could potentially explain the lower oxLDL levels in premenopausal women compared to men. However, as women transition into menopause and ovarian production of 17-beta estradiol decreases, their oxLDL levels tend to increase (Paik et al., 2013) contributing to diminished cardiovascular protective advantages they once enjoyed during the premenopausal years. In terms of vascular reactivity, sex differences in endothelium-dependent responses have been thoroughly investigated [Reviewed by Costa et al. (2023)] Kauser and Rubanyi (1995); Riedel et al. (2019). Endogenous estrogen levels contribute to mechanisms linked to vasodilation (e.g., enhanced NO and prostacyclin production) while androgens are more linked to augmented vasoconstrictor pathways (e.g., enhanced thromboxane synthase activity) (Li et al., 2004) (Krause et al., 2011; Gonzales et al., 2005). Interestingly, at the molecular level, a candidate gene study revealed sex-specific association between the OLR1 gene, the gene that codes for LOX-1, and carotid plaque formation (Wang et al., 2011). A study by Akhmedov et al. (2014) reported that overexpression of endothelial LOX-1 increases plaque formation and atherosclerosis in male mice. This, combined with other findings demonstrating higher LOX-1 expression in male aortas compared to female aortas (Kim and Hamblin, 2016), suggests that oxLDL/LOX-1 signaling may be influenced by biological sex. However, what specific role oxLDL/LOX-1 plays on vascular function including the consideration of sex-specific responses remains an area of investigation. Therefore, in this study we addressed whether oxLDL alters vascular reactivity, stiffness, and remodeling across age and sex in thoracic aortic ring preparations isolated from gonadally intact 7 and 12 month old male and female mice and determined whether oxLDL mediated actions are LOX-1 dependent.
All mice experiments were approved by the Institutional Animal Care and Use Committee at the University of Arizona (IACUC 16-079, PI: RJG). Male and female mice (C57BL/6J; Jackson Laboratory Bar Harbor, ME) were non-apolipoprotein-E deficient mice to minimize confounding variable of prior exposure to elevated serum levels of oxLDL (4.5 ng/dL) previously found to develop in apolipoprotein-E deficient mice by 10 weeks of age (Kato et al., 2009).
Thoracic aortas were isolated from 7-month (n = 19/male; n = 14/female) and 12-month (n = 15/male; n = 18/female) C57BL/6J intact female (not cycled) and male mice. Thoracic aortas were placed in ice-cold PSS bicarbonate buffer (PSS: 118.99 mM NaCl, 4.69 mM KCl, 1.17 mM MgSO4*7H2O, 1.18 mM KH2PO4, 1.60 mM CaCl2-2H2O, 25.00 mM NaHCO3, 0.03 mM EDTA, 5.50 mM glucose) bubbled continuously with 21% O2 and 5% CO2 and cleaned of fat and connective tissue and cut into 1 mm rings for isometric contractile force measurements. Next, endothelium-intact or -denuded rings were mounted on tungsten wires and immersed in PSS at 37°C with constant gassing (21% O2 and 5% CO2) in a wire myography chamber (DMT 610) and incubated ex vivo with oxLDL (50
Thoracic aortic stiffness was determined as previously described by assessing the slope of the stress-strain curve (Del Campo et al., 2019). Incremental recordings of both the force tension and internal diameter were taken during mechanical aortic ring stretching within the tissue bath. The force measurements were recorded by a force transducer and plotted against the corresponding diameter of the aortic rings. Once plotted, a linear regression analysis was performed for each treatment group to ascertain the slope of force over diameter. As described by Del Campo et al. (2019), an increased slope value of the tension over diameter relationship corresponds to an increased stiffness of the aortic ring segment. The remodeling endpoint was determined using the second extrapolated value from the linear regression analysis of the tension over diameter plots in combination with the LaPlace equation. Del Campo et al. (2019) previously described this methodology of estimating the vessel diameter, from which decreased vessel diameter at 100 mmHg would indicate inward remodeling. With regards to the specific protocol, diameter–tension relationships were determined following 2 h incubation, but prior to KCL wakeup by a stepwise stretching of the tissue increasing its passive diameter by increasing the distance between the tungsten wires that were passed through the lumen of the aortic segment. Both the force and internal circumference of each aortic vessel segment was recorded and transformed into vessel diameter. The estimated diameter at 100 mmHg of pressure was determined utilizing the DMT normalization module (LabChart software, ADInstruments) which applies the obtained diameter-tension relationship and Laplace equation. Linear regression of each diameter-tension relationship was utilized to determine passive aortic stiffness and remodeling, corresponding to the steepness of the slope and diameter of vessel at zero pressure in relation to estimated diameter at 100 mmHg. Stress strain curves were also generated to visualize the mechanical elasticity and stiffness changes within the isolated aortic segments from each experimental/drug treatment cohort. Stress was expressed at force (mN)/area (mm2) and strain calculated as the ratio of (initial diameter–final diameter)/final diameter.
To investigate potential molecular mechanisms underlying oxLDL-mediated changes in vasoreactivity, stiffness, and remodeling during aging, we examined the levels of relevant mediators in thoracic aortas from 1 month old and a small cohort of 16 month old aged male mice. Quantitative real time PCR (qRT-PCR) was utilized to measure changes in mRNA levels of LOX-1, CD36, ET-1, ET-1R
All reagents were purchased from Sigma Aldrich (St. Louis, MO) unless otherwise noted.
For each treatment group, an n ≥ 3 was used to achieve an acceptable power for statistical analysis. Experiments were repeated for statistical analysis and data graphed using Prism 9.3.0 for Windows, GraphPad Software, United States, www.graphpad.com (GraphPad Software). Shapiro-Wilk and F-tests were performed to confirm if data sets achieved a normal distribution and equal variance among groups. Direct comparisons between two groups were made using an unpaired t-test. Comparisons between three or more groups were made using a two-way ANOVA with Tukey’s multiple comparisons post hoc test. p < 0.05 was considered significant. Values are expressed as means ± SEM.
It has been previously shown that 26 month male C57BL/6 mice exhibited increased aortic contraction compared to younger 5 month mice in response to PE (De Moudt et al., 2022). Similarly, in this study PE-induced thoracic aortic contraction was increased in both 12 month female and male mice relative to 7 month counterparts (Figures 1A, B); and this increase was most pronounced in the males (×4.4) as compared to the females (×1.8). When comparing males to females there was a notable sex dependent increase in the efficacy of PE-induced contraction in the females compared to males at 7-month-old (Figure 1C). That was not observed in 12-month-old mice (Figure 1D). While there were notable effects in age and sex on PE-induced contraction efficacy, we did not observe alterations to the potency of PE (Figure 1E).
Figure 1. PE-Induced Contraction of Thoracic Aortic Rings. Concentration response curves (A–D) to PE in 1 mm thoracic aortic rings from 7- and 12-month-old male and female mice. Graph in figure (E) represents LogEC50 of PE obtained from concentration response curves in male 7- (n = 6) and 12 month (n = 5) and female 7- (n = 4–5) and 12-month-old (n = 6) mice. Individual values are shown are transparent data points. Grouped data are represented as means ± SEM. Two-Way ANOVA with Tukey’s post hoc test *p < 0.05, ****p < 0.0001.
From the PE cumulative concentration-response curves we derived the EC50 for each animal to next assess ACh-mediated relaxation. Counter to our observations of age-dependent differences in PE-induced aortic contraction, there were no differences in 7 and 12 month female (Figure 2A) and 7 and 12 month male ACh mediated relaxation (Figure 2B). However, in alignment with previous observations made in 5 month male and female Sprague–Dawley rats (Akther et al., 2021), ACh-induced relaxation was greater in 7 month female mice than males (Figure 2C). This sex difference in response to ACh was diminished in 12 month male and female mice (Figure 2D). Similar to PE-induced contraction of the thoracic aortas, there was no changes in the potency of ACh-mediated relaxation in the 7 and 12 month male and female mice (Figure 2E).
Figure 2. ACh-Induced Relaxation PE Pre-Contracted Thoracic Aortic Rings. Concentration response curves (A–D) to ACh (PE-precontracted) in 1 mm thoracic aortic rings from 7- and 12-month-old male and female mice. Figure (E) illustrates LogIC50 of ACh obtained from concentration response curves in male 7- (n = 6) and 12 month (n = 5) and female 7- (n = 4–5) and 12-month-old (n = 6) mice. Individual values are shown are transparent data points. Grouped data are represented as means ± SEM. Two-Way ANOVA with Tukey’s post hoc test **p < 0.01.
We next assessed the impact of acute oxLDL (50
Figure 3. Male Thoracic Aortic Ring Contraction of 7- and 12-Month-Old Mice Following oxLDL Exposure, LOX-1 Inhibition, and oxLDL + LOX-1 Inhibition. Concentration response curves (A, B) to PE in 1 mm thoracic aortic rings from 7- and 12-month-old male mice following exposure to either vehicle (7 month: n = 5–6; 12 month: n = 5), oxLDL (50
Following the PE-induced contractility studies, we next assessed the effects of oxLDL/LOX-1 on ACh-mediated relaxation in 7 month and 12 month aortic rings pre-contracted with PE (predetermined EC50 dose) (Figure 4). In both the 7 month (Figure 4A) and 12 month (Figure 4B) male thoracic aortic rings, incubation with oxLDL ex vivo significantly attenuated ACh-mediated relaxation compared to vehicle treated rings. We further observed that LOX-1 inhibition with BI-0115 (LOX-1 inhibitor) attenuated this response in the rings collected from 7 month old male mice however BI-0115 had no effect on ACh-mediated relaxation in oxLDL treated aortic rings from 12 month old male mice suggesting an age dependent response to ACh relaxation following exposure oxLDL. When the aortic rings were denuded, ACh-mediated relaxation was nearly abolished and the effect of oxLDL nullified when compared to vehicle (Supplementary Figure S1B). We further observed that BI-0115 alone and BI -0115 plus oxLDL elicited a nominal increase in contraction in response to concentration response curve to ACh perhaps suggesting a smooth muscle LOX-1 dependent contractile response independent of oxLDL (Supplementary Figure S1B). Although changes in the efficacy of the ACh response curve following either oxLDL, BI-0115 alone, or oxLDL plus BI-0115 were observed, there were no changes in the potency of ACh-mediated relaxation (Figure 4C). Together, these data suggest that although oxLDL attenuates endothelial dependent relaxation, involvement of LOX-1 activation is age dependent between aortas from both 7- and 12-month-old male mice.
Figure 4. Male Thoracic Aortic Ring Relaxation of 7- and 12-Month-Old Mice Following oxLDL Exposure, LOX-1 Inhibition, and oxLDL + LOX-1 Inhibition. Concentration response curves (A, B) to ACh (PE-precontracted) in 1 mm thoracic aortic rings from 7- and 12-month-old male mice following exposure to either vehicle (7 month: n = 5–6; 12 month: n = 5), oxLDL (50
We first assessed thoracic aortic contractility via PE in both 7 and 12 month old. females and observed a decrease in contractility following exposure to either oxLDL, LOX-1 inhibition, or oxLDL plus LOX-1 inhibition (Figures 5A, B). These results are similar to our previous findings in 12-month-old male thoracic aortas; however, they contrast with the contractility responses observed in 7-month-old male aortas. When aortic rings were denuded, as observed previously in our male denuded preparations (Supplementary Figure S1A), the effect of oxLDL was nullified in denuded female aortic rings (Supplementary Figure S1C). And similar to that observed in males, we did not observe a change in the potency of PE-mediated contraction in females regardless of age or treatment (Figure 5C).
Figure 5. Female Thoracic Aortic Ring Contraction of 7- and 12-Month-Old Mice Following oxLDL Exposure, LOX-1 Inhibition, and oxLDL + LOX-1 Inhibition. Concentration response curves to (A, B) PE in 1 mm thoracic aortic rings from 7- and 12-month-old female mice following exposure to either vehicle (7 month: n = 4–5; 12 month: n = 5), oxLDL (50
When assessing the impact of oxLDL on endothelial dependent relaxation in the female mice, we observed that oxLDL attenuated ACh induced relaxation in both 7- and 12-month-old female aortic rings (Figures 6A, B). This attenuative oxLDL response on the ACh induced relaxation appeared to be less prominent in both the 7 month (51.5
Figure 6. Female Thoracic Aortic Ring Relaxation of 7- and 12-Month-Old Mice Following oxLDL Exposure, LOX-1 Inhibition, and oxLDL + LOX-1 Inhibition. Concentration response curves (A, B) to ACh (PE-precontracted) in 1 mm thoracic aortic rings from 7- and 12-month-old female mice following exposure to either vehicle (7 month: n = 4–5; 12 month: n = 5), oxLDL (50
We next examined how acute oxLDL exposure impacts the thoracic aorta functionally in terms of stiffness (Figure 7). Utilizing the diameter-tension curves from both 7- and 12-month-old male and female aortic ring studies, calculated measurements resulted in an overall effect of both sex and age on stiffness (Figure 7A). These data indicate that 7 month thoracic aortic rings were stiffer in comparison to 12 month rings and that male mice had increased stiffness compared to females. Additionally, oxLDL as well as the selective LOX-1 inhibitor, BI-0115, in the presence or absence of oxLDL increased stiffness in 7 month male aortas in an endothelial dependent manner (Figure 7B). Whereas oxLDL did not influence the stiffness of 12 month males, or 7- and 12-month-old females. Interestingly, the selective LOX-1 inhibitor alone or in the presence of oxLDL decreased 7 month female aortic stiffness however this response was not endothelial dependent (Figure 7C). While there was an overall effect of age on aortic stiffness, we did not observe an effect of oxLDL, LOX-1 inhibition, or oxLDL plus LOX-1 inhibition on 12 month male and female aortic stiffness (Figure 7A). Accompanying stress strain curves are included in Supplementary Figures S3A–D (7-month-old male and female endothelium intact and denuded) and in Supplementary Figures S4A, B (12-month-old male and female endothelium intact only).
Figure 7. OxLDL Differentially Alters Thoracic Aortic Stiffness. Graphs depicting wire myography mediated (A–C) linear regression analysis of diameter–tension relationships from 1 mm thoracic aortic vessels obtained from 7- and 12-month-old male and female mice following exposure to either vehicle (Male: 7 month: n = 6; 12 month: n = 5; Female: 7 month: n = 4–5; 12 month: n = 6), oxLDL (50
By collecting aortic ring force and internal circumference measurements, we transformed these data recordings to calculate vessel diameter (see Section 2 for details). In terms of the finding, although we did not observe an overall effect of aging 5 months in mice on inward aortic remodeling we did however, observe a significant impact of sex suggesting that female thoracic aortas are smaller in diameter compared to males (Figure 8). Upon further examination addressing the effects of oxLDL, dissimilar to our observations of altered stiffness, we did not observe an effect of oxLDL on 7 month male and female remodeling. However, we did observe that in denuded 7 month male aortic rings, oxLDL, BI-0115, and oxLDL plus BI-0115 increased inward remodeling (Supplementary Figure S2A). We also observed that endothelial removal resulted in inward remodeling of 7 month female aortic rings (Supplementary Figure S2B). The LOX-1 inhibitor in plus or minus oxLDL had no effect. In the older cohorts, oxLDL induced a significant inward remodeling in both endothelial intact 12 month male and female aortic rings, and these responses were LOX-1 dependent (Figure 8). From this we hypothesized that perhaps 12 month mice may be potentially metabolizing or up taking oxLDL at a greater rate compared to the 7-month-old mice resulting in a smaller diameter at 100 mmHg. In efforts to elucidate potential molecular mechanisms of oxLDL mediated altered vasoreactivity, stiffness, and remodeling in aging, we assessed levels of possible involved mediators within the thoracic aortas from 1-month-old and a small cohort of 16-month-old aged male mice (Supplementary Figure S5). We observed that oxLDL increased mRNA levels of LOX-1, endothelin-1, endothelin-1-receptors alpha and beta, and interleukin-6. Intriguingly however, we did not observe an increase in CD36 expression following oxLDL exposure but did observe an increase in CD36 expression with aging. Moreover, we also observed that levels of LOX-1, endothelin-1-receptor beta, and interleukin-6 were increased with age, but not endothelin-1 and endothelin-1-receptor alpha. Together these data suggest that increased age may potentiate a preferential increase in both LOX-1 and CD36-mediated uptake of oxLDL, and future studies will determine if these factors play a role in increased inward remodeling that we observed in our 12-month-old mice.
Figure 8. OxLDL Effect Remodeling in Thoracic Aortic Rings in a Sex, Endothelial, and LOX-1 Dependent Manner. Estimated diameter at 100 mmHg from 1 mm thoracic aortic rings was calculated in 7- and 12-month-old male and female mice to predict inward remodeling following exposure to either vehicle (Male: 7 month: n = 6; 12 month: n = 5; Female: 7 month: n = 4–5; 12 month: n = 6), oxLDL (50
In the present study we employed an ex vivo thoracic aortic ring preparation to evaluate the impact of the oxLDL/LOX-1 axis on vasoreactivity, stiffness, remodeling, and transcription in the context of sex and age to further elucidate the detrimental role oxLDL/LOX-1 plays in altering vascular function and health. Additionally, we evaluated the role of LOX-1 receptor dependance on thoracic aortic vascular reactivity and endothelial function. We have for the first time, demonstrated the influence of sex, age, endothelium dependence, and LOX-1 activation following 2 h ex vivo oxLDL exposure in mediated alterations in thoracic aortic vasoreactivity, stiffness, and remodeling. In brief, oxLDL exposure 1) increased contractility of 7 month old male thoracic aortas in response to PE in an endothelial and LOX-1 dependent manner, 2) decreased contractility in 12 month old males as well as 7 and 12 months old females, 3) induced altered ACh dependent relaxation (indirect assessment of endothelial dysfunction) in all cohorts regardless of age or sex, but was most pronounced and LOX-1 dependent in the 7 month old males compared to 12 month old males and 7 and 12 months old females, 4) increased aortic stiffness in 7 month males in a LOX-1 independent manner, and 5) increased inward remodeling of 12 month old male and female thoracic aortas in a LOX-1 dependent manner.
Elucidating the sexual dimorphism in response to cardiovascular pathology such as elevated levels of oxLDL is significant however the impact of oxLDL on vascular reactively has not been thoroughly investigated. Thus, we considered sex as a critical factor in mediating outcomes due to the burgeoning and substantial amount of prior published work demonstrating sex differences during cardiovascular disease. In agreement with other published reports, our data demonstrate that oxLDL via LOX-1 can play a significant role in modulating thoracic aortic vascular reactivity, in part by mediating endothelial dysfunction. Not yet reported by others, our findings suggest that alterations in thoracic aortic vascular reactivity to acute exposure of oxLDL is sex dependent and may potentially contribute to the variations and susceptibility to vascular diseases observed between men and women clinically. Although in this study we did not address vascular LOX-1 expression levels in male verses female, Matilla et al. (2022) demonstrated higher levels of LOX-1 expression cardiac valvular tissues from males verses females. This heightened expression of LOX-1 in males may contribute to a greater predisposition to oxidative stress and inflammation, key drivers of impaired vascular reactivity. In terms of sex differences associated with vascular wall integrity, clinically aortic dissection and rupture have been reported to be higher amongst males compared to females (Olsson et al., 2006). However, this finding has been convoluted by more recent studies demonstrating that thoracic aortic aneurysm mediated death and acute symptoms are higher in women than men (Boczar et al., 2019). Boczar et al. (2019) reported that aneurysmal growth was more than twice as fast in female as it was in male patients and that aortic stiffness was associated with this increase in growth in females but not males. Prior studies examining potential mechanisms underlying these clinical sex differences have found that in experimental aortic aneurysms, female mice exhibited higher levels of estrogen receptor alpha as well as lower matrix metalloproteinase 9 and 2 activity compared to males which could be contributing to increased vasoprotective effects and decreased vascular inflammation in females (Laser et al., 2014). It has also been found in the International Registry of Acute Aortic Dissection, that females presenting with acute aortic dissection were older than males (Nienaber et al., 2004), further suggesting sex differences potentially due to the loss of vasoprotective effects of estrogen upon menopause (Gavin et al., 2009). Understanding these sex differences is crucial for tailoring medical interventions and improving outcomes for both men and women diagnosed with thoracic aortic pathology.
In addition to sex differences, aging is linked to various structural, mechanical, and functional alterations in the vasculature, which are marked by increased arterial stiffness, diminished elasticity, endothelial dysfunction, and changes in vascular tone [Reviewed by Harvey et al. (2016)]. Additionally, the effect of age has been shown to play a role in vascular function at the proteomic and genomic level (Tyrrell et al., 2022; Wan et al., 2023), highlighting the detrimental impact that age plays in the progression and continuation of vascular disease. It has been previously found that aging transforms the human aortic proteome from healthy to a pathological state (thoracic aortic aneurysms), leading to a differential regulation of biological processes (Tyrrell et al., 2022). Several of these processes found to be downregulated with aging include extracellular matrix organization, receptor-mediated endocytosis, and golgi vesicle transport which play all play a role in vasoreactivity and function (Mammoto et al., 2022; Cui et al., 2020; Lee et al., 2009). These proteomic changes may play a crucial role in the deterioration of thoracic aortic function with age. Additionally, a cross-sectional study indicated that the normal aging process within the aorta is associated with gradual aortic elongation and a notable change in aortic geometry (Adriaans et al., 2018). This structural alteration may contribute to age-related changes in aortic function and will be examined in our future studies as it relates to oxLDL/LOX-1. This is further substantiated by a separate study which revealed age-related unfolding of the aortic arch is linked to increased proximal aortic stiffness even in humans free of overt cardiovascular disease, reflecting the interplay of age-related changes that could prompt pathology (Redheuil et al., 2011). Morphology of the human aorta undergoes age-related changes, affecting its diameter, length, and other key aspects such as atherosclerosis (Greenwald, 2007; da Silva et al., 1999; Sawabe et al., 2011; Collins et al., 2014; Fritze et al., 2012; Yamada et al., 2015). These morphological alterations are likely to contribute to the overall decline in thoracic aortic function as the vasculature ages. The aging process is multifaceted and exerts effects on thoracic aortic function through structural changes, alterations in aortic proteome regulation, and modifications in aortic morphology and geometry. Further understanding these mechanisms, especially under pathology such as hyperlipidemia, is crucial for developing targeted interventions to mitigate age-related thoracic aortic dysfunction.
In this study we observed that oxLDL exposure induced endothelial dysfunction resulting in endothelial dependent differentially altered vasoreactivity across a modest span of aging and sex which appear to be in part LOX-1 receptor dependent. It has been previously found that oxLDL mediates an upregulation of endothelin-1, a potent vasoconstrictor, in endothelial cells (Niemann et al., 2005), which corresponds to our findings at the mRNA level (Supplementary Figure S5) and could in part play a role in our observations of increased PE induced contractility in 7 month males (Figure 1). This is further perpetuated by findings demonstrating that blockade of ET-1R
Intriguingly, we observed a concomitant increase in basal expression of ET-1Rβ in 16 month male thoracic aortas but not alpha. This may suggest an increase in ET-1Rβ mediated increase within the endothelium and subsequential increase in ET-1Rβ -mediated relaxation in the 12 month male mice. In turn, this can result in attenuated contractility in response to PE as well as greater a ACh-mediated relaxation, potentially due to previously demonstrated expression of ET-1Rβ within the endothelium (Khimji and Rockey, 2010; Sakurai et al., 1990; Levin, 1995) and an ET-1Rβ-mediated relaxation in rat thoracic aortic vessels (Tykocki et al., 2009). Expression of endothelin-1 and its receptors merits further investigation across the sexes as it has been previously observed that in human internal mammary arteries, exposure to 17β-estradiol resulted in downregulation of ET-1Rα/β expression (Haas et al., 2007) suggesting that females may respond differentially along the endothelin-1/receptor axis compared to males and may in part delineate the decreased contractility observed in 7 and 12 months female aortic rings exposed to oxLDL.
In addition to increased interest regarding vasoreactivity, there has been a growing interest in elucidating the relationship between oxLDL and aortic stiffness as well as remodeling. Previous work such as the pivotal “Health ABC Study” (Brinkley et al., 2009), has contributed substantially to this line of interest. Wherein the authors explored the correlation between plasma oxLDL levels and arterial stiffness in older adults, revealing via pulse wave velocity measurements a significantly increased incidence of high arterial stiffness with increased oxLDL levels. Moreover, in a separate study, it was demonstrated that LOX-1 was associated with arterial stiffness in both middle-aged and elderly men and women (Otsuki et al., 2015). Others have demonstrated that in the aging human aorta, the degradation of the extracellular matrix is exacerbated by increased levels and activity of the inflammatory enzyme, MMP-2 (McNulty et al., 2005; Tayebjee et al., 2005). Intriguingly, in this study we observed a paradoxical decrease in aortic stiffness in the 12 month compared to 7 month mice. Counter to the previous report which demonstrated that male C57BL/6 mice thoracic aortas progressively increase in stiffness at 12 month compared to 4 month (De Moudt et al., 2022). We hypothesize that our observations could be due to a retention of elastic properties as we did not observe an age dependent change in luminal diameter as these have been described as the most consistent well-reported changes resulting in aortic stiffness (Lee and Oh, 2010). Further investigation into the underlying mechanisms of this observation is warranted but falls outside the scope of this study.
While we observed no effect of oxLDL aortic stiffness in 12 month male or female, we did observe an increase in stiffness in 7 month male thoracic aortic rings following oxLDL (Figure 7). Moreover, we observed a sex-dependent response of LOX-1 inhibition which resulted in increased 7 month male stiffness but decreased age-matched female aortic stiffness in the presence or absence of oxLDL in an endothelial dependent manner. Together these data suggest that endothelial LOX-1 may play a differential role in the maintenance of 7 month male and female thoracic aortic stiffness but diminishes with age, in our case middle age. We could hypothesize that this could be a function of pathophysiologic aging-mediated upregulation of CD36 expression as observed in this study but warrants further elucidation. It has been reported that CD36 plays a critical role in oxLDL accumulation and internalization in macrophages (Nagy et al., 1998; Yang et al., 2018) from which we hypothesize that the upregulation of CD36 with age could also in part be responsible for the age dependent effects of oxLDL/LOX-1-mediated increases inward remodeling observed in the 12 month male and females, but not 7 month mice. Moreover, due to the previously noted LOX-1-mediated vascular remodeling (Hofmann et al., 2017), we theorize that CD36 is competing for oxLDL binding resulting in increased efficacy of LOX-1 inhibition to attenuate oxLDL/LOX-1-mediated inward remodeling in 12 month mice compared to 7 month mice. Together suggesting a differential role of LOX-1 in the progression of oxLDL-mediated thoracic aortic pathology in terms of stiffness and remodeling within males and female mice across age.
We acknowledge that the complex cascade of oxLDL/LOX-1 within the thoracic aorta cannot be exactly modeled in an ex vivo setting. Moreover, we recognize that isolated aortic ring segments do not fully replicate the complex architecture of an intact vessel, particularly the integrated endothelial and smooth muscle layers, nor do they capture the pulsatile dynamics observed in vivo. However, ex vivo studies enable the pharmacologic evaluation of vascular reactivity. Although this method may only offer an indirect assessment of specific cellular and molecular mechanisms under oxLDL exposure, the findings can still provide insights that reflect potential observations in pathological conditions like hyperlipidemia and dyslipidemia in vivo.
In conclusion, our ex vivo study has significantly contributed to the further elucidation of the intricate relationship between the oxLDL/LOX-1 axis and mouse thoracic aortic physiology, particularly within the contexts of biological sex and the more mature aging vasculature. We have successfully demonstrated the impact of acute ex vivo oxLDL exposure on distinct alterations in aortic vasoreactivity, stiffness, and remodeling, revealing differential responses across age and sexes. Importantly, our findings highlight the pivotal role of the endothelium and LOX-1 in coordinating these responses. Our results also further point towards the interplay between oxLDL and endothelin-1, contextualizing potential contributions to observed vasoreactivity changes. Furthermore, our paradoxical observation of decreased aortic stiffness in the older cohort of mice (12 month old) studied warrants deeper exploration to uncover the underlying mechanisms of oxLDL and its role in regulating vascular reactivity during middle age. While we observed varying effects of LOX-1 inhibition on stiffness between age-matched male and female mice, the LOX-1 receptors potential relationship with CD36-mediated processes adds yet another layer of complexity to the multifaceted nature of oxLDL-mediated effects. Future studies will be aimed at further elucidation into the mechanisms linking oxLDL, LOX-1, endothelin-1, and CD36, while also considering the broader implications for age and sex differences in vascular health. This study lays a strong foundation for unraveling the elaborate molecular pathways that contribute to oxLDL/LOX-1-mediated alterations in aortic physiology, with potential implications for therapeutic interventions in cardiovascular health.
The original contributions presented in the study are included in the article/Supplementary Material, further inquiries can be directed to the corresponding author.
The animal study was approved by the Institutional Animal Care and Use Committee at the University of Arizona. The study was conducted in accordance with the local legislation and institutional requirements.
TW: Conceptualization, Data curation, Formal Analysis, Funding acquisition, Investigation, Methodology, Software, Validation, Visualization, Writing–original draft, Writing–review and editing. SA: Conceptualization, Writing–review and editing. RG: Conceptualization, Data curation, Formal Analysis, Funding acquisition, Investigation, Methodology, Project administration, Resources, Software, Supervision, Validation, Visualization, Writing–original draft, Writing–review and editing.
The author(s) declare that financial support was received for the research, authorship, and/or publication of this article. This research was funded American Heart Association, grant number 19AIREA34480018 (RG); Valley Research Partnership, grant numbers VRP37 P2 (RG) and VRP55 P1a (TW and RG).
We would like to thank Dr. Taben Hale for providing us access to and utilization of the DMT 610 myography rig.
The authors declare that the research was conducted in the absence of any commercial or financial relationships that could be construed as a potential conflict of interest.
All claims expressed in this article are solely those of the authors and do not necessarily represent those of their affiliated organizations, or those of the publisher, the editors and the reviewers. Any product that may be evaluated in this article, or claim that may be made by its manufacturer, is not guaranteed or endorsed by the publisher.
The Supplementary Material for this article can be found online at: https://www.frontiersin.org/articles/10.3389/fphys.2024.1471272/full#supplementary-material
Adriaans B. P., Heuts S., Gerretsen S., Cheriex E. C., Vos R., Natour E., et al. (2018). Aortic elongation part I: the normal aortic ageing process. Heart 104 (21), 1772–1777. doi:10.1136/heartjnl-2017-312866
Akhmedov A., Rozenberg I., Paneni F., Camici G. G., Shi Y., Doerries C., et al. (2014). Endothelial overexpression of LOX-1 increases plaque formation and promotes atherosclerosis in vivo. Eur. Heart J. 35 (40), 2839–2848. doi:10.1093/eurheartj/eht532
Akther F., Razan M. R., Shaligram S., Graham J. L., Stanhope K. L., Allen K. N., et al. (2021). Potentiation of acetylcholine-induced relaxation of aorta in male UC davis type 2 diabetes mellitus (UCD-t2dm) rats: sex-specific responses. Front. Physiol. 12, 616317. doi:10.3389/fphys.2021.616317
Barreto J., Karathanasis S. K., Remaley A., Sposito A. C. (2021). Role of LOX-1 (Lectin-Like oxidized low-density lipoprotein receptor 1) as a cardiovascular risk predictor: mechanistic insight and potential clinical use. Arterioscler. Thromb. Vasc. Biol. 41 (1), 153–166. doi:10.1161/ATVBAHA.120.315421
Blair A., Shaul P. W., Yuhanna I. S., Conrad P. A., Smart E. J. (1999). Oxidized low density lipoprotein displaces endothelial nitric-oxide synthase (eNOS) from plasmalemmal caveolae and impairs eNOS activation. J. Biol. Chem. 274 (45), 32512–32519. doi:10.1074/jbc.274.45.32512
Boczar K. E., Cheung K., Boodhwani M., Beauchesne L., Dennie C., Nagpal S., et al. (2019). Sex differences in thoracic aortic aneurysm growth. Hypertension 73 (1), 190–196. doi:10.1161/HYPERTENSIONAHA.118.11851
Brinkley T. E., Nicklas B. J., Kanaya A. M., Satterfield S., Lakatta E. G., Simonsick E. M., et al. (2009). Plasma oxidized low-density lipoprotein levels and arterial stiffness in older adults: the health, aging, and body composition study. Hypertension 53 (5), 846–852. doi:10.1161/HYPERTENSIONAHA.108.127043
Collins J. A., Munoz J. V., Patel T. R., Loukas M., Tubbs R. S. (2014). The anatomy of the aging aorta. Clin. Anat. 27 (3), 463–466. doi:10.1002/ca.22384
Cominacini L., Rigoni A., Pasini A. F., Garbin U., Davoli A., Campagnola M., et al. (2001). The binding of oxidized low density lipoprotein (ox-LDL) to ox-LDL receptor-1 reduces the intracellular concentration of nitric oxide in endothelial cells through an increased production of superoxide. J. Biol. Chem. 276 (17), 13750–13755. doi:10.1074/jbc.M010612200
Costa T. J., Tostes R. C. (2023). “Sex differences in vascular function,” in Cardiovascular neuroendocrinology. Editors G. L. C. Yosten, and J. T. Cunningham (Cham: Springer International Publishing), 83–108.
Cui K., Dong Y., Wang B., Cowan D. B., Chan S. L., Shyy J., et al. (2020). Endocytic adaptors in cardiovascular disease. Front. Cell Dev. Biol. 8, 624159. doi:10.3389/fcell.2020.624159
da Silva E. S., Rodrigues A. J., Castro de Tolosa E. M., Bueno P. P. R., Zanoto A., Martins J. (1999). Variation of infrarenal aortic diameter: a necropsy study. J. Vasc. Surg. 29 (5), 920–927. doi:10.1016/s0741-5214(99)70220-x
Del Campo L., Sánchez-López A., Salaices M., von Kleeck R. A., Expósito E., González-Gómez C., et al. (2019). Vascular smooth muscle cell-specific progerin expression in a mouse model of Hutchinson-Gilford progeria syndrome promotes arterial stiffness: therapeutic effect of dietary nitrite. Aging Cell 18 (3), e12936. doi:10.1111/acel.12936
De Moudt S., Hendrickx J. O., Neutel C., De Munck D., Leloup A., De Meyer G. R. Y., et al. (2022). Progressive aortic stiffness in aging C57Bl/6 mice displays altered contractile behaviour and extracellular matrix changes. Commun. Biol. 5 (1), 605. doi:10.1038/s42003-022-03563-x
Fritze O., Romero B., Schleicher M., Jacob M. P., Oh D. Y., Starcher B., et al. (2012). Age-related changes in the elastic tissue of the human aorta. J. Vasc. Res. 49 (1), 77–86. doi:10.1159/000331278
Galle J., Hansen-Hagge T., Wanner C., Seibold S. (2006). Impact of oxidized low density lipoprotein on vascular cells. Atherosclerosis 185 (2), 219–226. doi:10.1016/j.atherosclerosis.2005.10.005
Gavin K. M., Seals D. R., Silver A. E., Moreau K. L. (2009). Vascular endothelial estrogen receptor alpha is modulated by estrogen status and related to endothelial function and endothelial nitric oxide synthase in healthy women. J. Clin. Endocrinol. Metab. 94 (9), 3513–3520. doi:10.1210/jc.2009-0278
Gimbrone M. A., García-Cardeña G. (2016). Endothelial cell dysfunction and the pathobiology of atherosclerosis. Circ. Res. 118 (4), 620–636. doi:10.1161/CIRCRESAHA.115.306301
Gonzales R. J., Ghaffari A. A., Duckles S. P., Krause D. N. (2005). Testosterone treatment increases thromboxane function in rat cerebral arteries. Am. J. Physiol. Heart Circ. Physiol. 289 (2), H578–H585. doi:10.1152/ajpheart.00958.2004
Gradinaru D., Borsa C., Ionescu C., Prada G. I. (2015). Oxidized LDL and NO synthesis--Biomarkers of endothelial dysfunction and ageing. Mech. Ageing Dev. 151, 101–113. doi:10.1016/j.mad.2015.03.003
Greenwald S. E. (2007). Ageing of the conduit arteries. J. Pathol. 211 (2), 157–172. doi:10.1002/path.2101
Haas E., Meyer M. R., Schurr U., Bhattacharya I., Minotti R., Nguyen H. H., et al. (2007). Differential effects of 17beta-estradiol on function and expression of estrogen receptor alpha, estrogen receptor beta, and GPR30 in arteries and veins of patients with atherosclerosis. Hypertension 49 (6), 1358–1363. doi:10.1161/HYPERTENSIONAHA.107.089995
Harvey A., Montezano A. C., Lopes R. A., Rios F., Touyz R. M. (2016). Vascular fibrosis in aging and hypertension: molecular mechanisms and clinical implications. Can. J. Cardiol. 32 (5), 659–668. doi:10.1016/j.cjca.2016.02.070
Hofmann A., Brunssen C., Morawietz H. (2017). Contribution of lectin-like oxidized low-density lipoprotein receptor-1 and LOX-1 modulating compounds to vascular diseases. Vasc. Pharmacol. 107, 1–11. doi:10.1016/j.vph.2017.10.002
Jiang F., Gibson A. P., Dusting G. J. (2001). Endothelial dysfunction induced by oxidized low-density lipoproteins in isolated mouse aorta: a comparison with apolipoprotein-E deficient mice. Eur. J. Pharmacol. 424 (2), 141–149. doi:10.1016/s0014-2999(01)01140-2
Kato R., Mori C., Kitazato K., Arata S., Obama T., Mori M., et al. (2009). Transient increase in plasma oxidized LDL during the progression of atherosclerosis in apolipoprotein E knockout mice. Arterioscler. Thromb. Vasc. Biol. 29 (1), 33–39. doi:10.1161/ATVBAHA.108.164723
Kauser K., Rubanyi G. M. (1995). Gender difference in endothelial dysfunction in the aorta of spontaneously hypertensive rats. Hypertension 25 (4 Pt 1), 517–523. doi:10.1161/01.hyp.25.4.517
Khalil R. A. (2005). Sex hormones as potential modulators of vascular function in hypertension. Hypertension 46 (2), 249–254. doi:10.1161/01.HYP.0000172945.06681.a4
Khimji A. K., Rockey D. C. (2010). Endothelin--biology and disease. Cell Signal 22 (11), 1615–1625. doi:10.1016/j.cellsig.2010.05.002
Kim S. C., Hamblin M. H. (2016). Sex differences in the gene expression profile of LOX-1 in mouse tissues. FASEB J. 30 (S1), 738.8–8. doi:10.1096/fasebj.30.1_supplement.738.8
Krause D. N., Duckles S. P., Gonzales R. J. (2011). Local oestrogenic/androgenic balance in the cerebral vasculature. Acta Physiol. (Oxf) 203 (1), 181–186. doi:10.1111/j.1748-1716.2011.02323.x
Laser A., Ghosh A., Roelofs K., Sadiq O., McEvoy B., DiMusto P., et al. (2014). Increased estrogen receptor alpha in experimental aortic aneurysms in females compared with males. J. Surg. Res. 186 (1), 467–474. doi:10.1016/j.jss.2013.07.050
Lee H. Y., Oh B. H. (2010). Aging and arterial stiffness. Circ. J. 74 (11), 2257–2262. doi:10.1253/circj.cj-10-0910
Lee J., Reich R., Xu F., Sehgal P. B. (2009). Golgi, trafficking, and mitosis dysfunctions in pulmonary arterial endothelial cells exposed to monocrotaline pyrrole and NO scavenging. Am. J. Physiol. Lung Cell Mol. Physiol. 297 (4), L715–L728. doi:10.1152/ajplung.00086.2009
Li D., Mehta J. L. (2000). Upregulation of endothelial receptor for oxidized LDL (LOX-1) by oxidized LDL and implications in apoptosis of human coronary artery endothelial cells: evidence from use of antisense LOX-1 mRNA and chemical inhibitors. Arterioscler. Thromb. Vasc. Biol. 20 (4), 1116–1122. doi:10.1161/01.atv.20.4.1116
Li L., Renier G. (2009). The oral anti-diabetic agent, gliclazide, inhibits oxidized LDL-mediated LOX-1 expression, metalloproteinase-9 secretion and apoptosis in human aortic endothelial cells. Atherosclerosis 204 (1), 40–46. doi:10.1016/j.atherosclerosis.2008.08.008
Li X., Geary G. G., Gonzales R. J., Krause D. N., Duckles S. P. (2004). Effect of estrogen on cerebrovascular prostaglandins is amplified in mice with dysfunctional NOS. Am. J. Physiol. Heart Circ. Physiol. 287 (2), H588–H594. doi:10.1152/ajpheart.01176.2003
Liu B., Liu N. N., Liu W. H., Zhang S. W., Zhang J. Z., Li A. Q., et al. (2016). Inhibition of lectin-like oxidized low-density lipoprotein receptor-1 reduces cardiac fibroblast proliferation by suppressing GATA Binding Protein 4. Biochem. Biophys. Res. Commun. 475 (4), 329–334. doi:10.1016/j.bbrc.2016.05.095
Liu L. Y., Aimaiti X., Zheng Y. Y., Zhi X. Y., Wang Z. L., Yin X., et al. (2023). Epidemic trends of dyslipidemia in young adults: a real-world study including more than 20,000 samples. Lipids Health Dis. 22 (1), 108. doi:10.1186/s12944-023-01876-2
Mammoto A., Matus K., Mammoto T. (2022). Extracellular matrix in aging aorta. Front. Cell Dev. Biol. 10, 822561. doi:10.3389/fcell.2022.822561
Matilla L., Garaikoetxea M., Arrieta V., García-Peña A., Fernández-Celis A., Navarro A., et al. (2022). Sex-differences in aortic stenosis: mechanistic insights and clinical implications. Front. Cardiovasc Med. 9, 818371. doi:10.3389/fcvm.2022.818371
McNulty M., Spiers P., McGovern E., Feely J. (2005). Aging is associated with increased matrix metalloproteinase-2 activity in the human aorta. Am. J. Hypertens. 18 (4 Pt 1), 504–509. doi:10.1016/j.amjhyper.2004.11.011
Munno M., Mallia A., Greco A., Modafferi G., Banfi C., Eligini S. (2024). Radical oxygen species, oxidized low-density lipoproteins, and lectin-like oxidized low-density lipoprotein receptor 1: a vicious circle in atherosclerotic process. Antioxidants (Basel) 13 (5), 583. doi:10.3390/antiox13050583
Nagy L., Tontonoz P., Alvarez J. G., Chen H., Evans R. M. (1998). Oxidized LDL regulates macrophage gene expression through ligand activation of PPARgamma. Cell 93 (2), 229–240. doi:10.1016/s0092-8674(00)81574-3
Niemann B., Rohrbach S., Catar R. A., Muller G., Barton M., Morawietz H. (2005). Native and oxidized low-density lipoproteins stimulate endothelin-converting enzyme-1 expression in human endothelial cells. Biochem. Biophys. Res. Commun. 334 (3), 747–753. doi:10.1016/j.bbrc.2005.06.163
Nienaber C. A., Fattori R., Mehta R. H., Richartz B. M., Evangelista A., Petzsch M., et al. (2004). Gender-related differences in acute aortic dissection. Circulation 109 (24), 3014–3021. doi:10.1161/01.cir.0000130644.78677.2c
Ogola B. O., Abshire C. M., Visniauskas B., Kiley J. X., Horton A. C., Clark-Patterson G. L., et al. (2022). Sex differences in vascular aging and impact of GPER deletion. Am. J. Physiol. Heart Circ. Physiol. 323 (2), H336–H349. doi:10.1152/ajpheart.00238.2022
Olsson C., Thelin S., Ståhle E., Ekbom A., Granath F. (2006). Thoracic aortic aneurysm and dissection: increasing prevalence and improved outcomes reported in a nationwide population-based study of more than 14,000 cases from 1987 to 2002. Circulation 114 (24), 2611–2618. doi:10.1161/CIRCULATIONAHA.106.630400
Otsuki T., Maeda S., Mukai J., Ohki M., Nakanishi M., Yoshikawa T. (2015). Association between plasma sLOX-1 concentration and arterial stiffness in middle-aged and older individuals. J. Clin. Biochem. Nutr. 57 (2), 151–155. doi:10.3164/jcbn.15-27
Ou H. C., Song T. Y., Yeh Y. C., Huang C. Y., Yang S. F., Chiu T. H., et al. (1985)2010). EGCG protects against oxidized LDL-induced endothelial dysfunction by inhibiting LOX-1-mediated signaling. J. Appl. Physiol. 108 (6), 1745–1756. doi:10.1152/japplphysiol.00879.2009
Paik J. K., Chae J. S., Kang R., Kwon N., Lee S. H., Lee J. H. (2013). Effect of age on atherogenicity of LDL and inflammatory markers in healthy women. Nutr. Metab. Cardiovasc Dis. 23 (10), 967–972. doi:10.1016/j.numecd.2012.08.002
Pandey D., Bhunia A., Oh Y. J., Chang F., Bergman Y., Kim J. H., et al. (2014). OxLDL triggers retrograde translocation of arginase2 in aortic endothelial cells via ROCK and mitochondrial processing peptidase. Circ. Res. 115 (4), 450–459. doi:10.1161/CIRCRESAHA.115.304262
Pirillo A., Norata G. D., Catapano A. L. (2013). LOX-1, OxLDL, and atherosclerosis. Mediat. Inflamm. 2013, 152786. doi:10.1155/2013/152786
Redheuil A., Yu W. C., Mousseaux E., Harouni A. A., Kachenoura N., Wu C. O., et al. (2011). Age-related changes in aortic arch geometry: relationship with proximal aortic function and left ventricular mass and remodeling. J. Am. Coll. Cardiol. 58 (12), 1262–1270. doi:10.1016/j.jacc.2011.06.012
Riedel K., Deussen A. J., Tolkmitt J., Weber S., Schlinkert P., Zatschler B., et al. (2019). Estrogen determines sex differences in adrenergic vessel tone by regulation of endothelial β-adrenoceptor expression. Am. J. Physiol. Heart Circ. Physiol. 317 (2), H243-H254–H54. doi:10.1152/ajpheart.00456.2018
Ross R. (1993). Rous-Whipple Award Lecture. Atherosclerosis: a defense mechanism gone awry. Am. J. Pathol. 143 (4), 987–1002.
Ryoo S., Bhunia A., Chang F., Shoukas A., Berkowitz D. E., Romer L. H. (2011). OxLDL-dependent activation of arginase II is dependent on the LOX-1 receptor and downstream RhoA signaling. Atherosclerosis 214 (2), 279–287. doi:10.1016/j.atherosclerosis.2010.10.044
Ryoo S., Lemmon C. A., Soucy K. G., Gupta G., White A. R., Nyhan D., et al. (2006). Oxidized low-density lipoprotein-dependent endothelial arginase II activation contributes to impaired nitric oxide signaling. Circ. Res. 99 (9), 951–960. doi:10.1161/01.RES.0000247034.24662.b4
Sakurai T., Yanagisawa M., Takuwa Y., Miyazaki H., Kimura S., Goto K., et al. (1990). Cloning of a cDNA encoding a non-isopeptide-selective subtype of the endothelin receptor. Nature 348 (6303), 732–735. doi:10.1038/348732a0
Sawabe M., Hamamatsu A., Chida K., Mieno M. N., Ozawa T. (2011). Age is a major pathobiological determinant of aortic dilatation: a large autopsy study of community deaths. J. Atheroscler. Thromb. 18 (2), 157–165. doi:10.5551/jat.6528
Sawamura T., Kume N., Aoyama T., Moriwaki H., Hoshikawa H., Aiba Y., et al. (1997). An endothelial receptor for oxidized low-density lipoprotein. Nature 386 (6620), 73–77. doi:10.1038/386073a0
Settergren M., Böhm F., Rydén L., Pernow J. (2008). Cholesterol lowering is more important than pleiotropic effects of statins for endothelial function in patients with dysglycaemia and coronary artery disease. Eur. Heart J. 29 (14), 1753–1760. doi:10.1093/eurheartj/ehn166
Takahashi K., Aono J., Nakao Y., Hamaguchi M., Suehiro C., Kurata M., et al. (2023). LOX-1 deficiency increases ruptured abdominal aortic aneurysm via thinning of adventitial collagen. Hypertens. Res. 46 (1), 63–74. doi:10.1038/s41440-022-01093-x
Tanaka J., Qiang L., Banks A. S., Welch C. L., Matsumoto M., Kitamura T., et al. (2009). Foxo1 links hyperglycemia to LDL oxidation and endothelial nitric oxide synthase dysfunction in vascular endothelial cells. Diabetes 58 (10), 2344–2354. doi:10.2337/db09-0167
Tayebjee M. H., Lip G. Y., Blann A. D., Macfadyen R. J. (2005). Effects of age, gender, ethnicity, diurnal variation and exercise on circulating levels of matrix metalloproteinases (MMP)-2 and -9, and their inhibitors, tissue inhibitors of matrix metalloproteinases (TIMP)-1 and -2. Thromb. Res. 115 (3), 205–210. doi:10.1016/j.thromres.2004.08.023
Trpkovic A., Resanovic I., Stanimirovic J., Radak D., Mousa S. A., Cenic-Milosevic D., et al. (2015). Oxidized low-density lipoprotein as a biomarker of cardiovascular diseases. Crit. Rev. Clin. Lab. Sci. 52 (2), 70–85. doi:10.3109/10408363.2014.992063
Truthe S., Klassert T. E., Schmelz S., Jonigk D., Blankenfeldt W., Slevogt H. (2024). Role of lectin-like oxidized low-density lipoprotein receptor-1 in inflammation and pathogen-associated interactions. J. Innate Immun. 16 (1), 105–132. doi:10.1159/000535793
Tykocki N. R., Gariepy C. E., Watts S. W. (2009). Endothelin ET(B) receptors in arteries and veins: multiple actions in the vein. J. Pharmacol. Exp. Ther. 329 (3), 875–881. doi:10.1124/jpet.108.145953
Tyrrell D. J., Chen J., Li B. Y., Wood S. C., Rosebury-Smith W., Remmer H. A., et al. (2022). Aging alters the aortic proteome in health and thoracic aortic aneurysm. Arterioscler. Thromb. Vasc. Biol. 42 (8), 1060–1076. doi:10.1161/ATVBAHA.122.317643
Valente A. J., Irimpen A. M., Siebenlist U., Chandrasekar B. (2014). OxLDL induces endothelial dysfunction and death via TRAF3IP2: inhibition by HDL3 and AMPK activators. Free Radic. Biol. Med. 70, 117–128. doi:10.1016/j.freeradbiomed.2014.02.014
Wan H., Liu D., Liu B., Sha M., Xia W., Liu C. (2023). Bioinformatics analysis of aging-related genes in thoracic aortic aneurysm and dissection. Front. Cardiovasc Med. 10, 1089312. doi:10.3389/fcvm.2023.1089312
Wang L., Yanuck D., Beecham A., Gardener H., Slifer S., Blanton S. H., et al. (2011). A candidate gene study revealed sex-specific association between the OLR1 gene and carotid plaque. Stroke 42 (3), 588–592. doi:10.1161/STROKEAHA.110.596841
Wendt T. S., Gonzales R. J. (2023). Ozanimod differentially preserves human cerebrovascular endothelial barrier proteins and attenuates matrix metalloproteinase-9 activity following in vitro acute ischemic injury. Am. J. Physiol. Cell Physiol. 325, C951–C971. doi:10.1152/ajpcell.00342.2023
Xu Q., Wu Q., Li H., Tian X., Zuo Y., Zhang Y., et al. (2022). Joint high level of oxidized low-density lipoprotein and high-sensitivity C-reactive protein are associated with recurrent stroke and poor functional outcome in minor stroke or transient ischemic attack. J. Am. Heart Assoc. 11 (20), e027665. doi:10.1161/JAHA.122.027665
Xu X., Gao X., Potter B. J., Cao J. M., Zhang C. (2007). Anti-LOX-1 rescues endothelial function in coronary arterioles in atherosclerotic ApoE knockout mice. Arterioscler. Thromb. Vasc. Biol. 27 (4), 871–877. doi:10.1161/01.ATV.0000259358.31234.37
Yamada H., Sakata N., Wada H., Tashiro T., Tayama E. (2015). Age-related distensibility and histology of the ascending aorta in elderly patients with acute aortic dissection. J. Biomech. 48 (12), 3267–3273. doi:10.1016/j.jbiomech.2015.06.025
Yang M., Kholmukhamedov A., Schulte M. L., Cooley B. C., Scoggins N. O., Wood J. P., et al. (2018). Platelet CD36 signaling through ERK5 promotes caspase-dependent procoagulant activity and fibrin deposition in vivo. Blood Adv. 2 (21), 2848–2861. doi:10.1182/bloodadvances.2018025411
Keywords: endothelium, oxidized low-density lipoprotein (OxLDL), lectin-like oxLDL receptor 1 (LOX-1), sex differences, age, vasoreactivity
Citation: Wendt TS, Ansar S and Gonzales RJ (2024) OxLDL/LOX-1 mediated sex, age, stiffness, and endothelial dependent alterations in mouse thoracic aortic vascular reactivity. Front. Physiol. 15:1471272. doi: 10.3389/fphys.2024.1471272
Received: 27 July 2024; Accepted: 03 October 2024;
Published: 05 November 2024.
Edited by:
Roshanak Rahimian, The University of the Pacific, United StatesReviewed by:
Mingyi Wang, National Institute on Aging (NIH), United StatesCopyright © 2024 Wendt, Ansar and Gonzales. This is an open-access article distributed under the terms of the Creative Commons Attribution License (CC BY). The use, distribution or reproduction in other forums is permitted, provided the original author(s) and the copyright owner(s) are credited and that the original publication in this journal is cited, in accordance with accepted academic practice. No use, distribution or reproduction is permitted which does not comply with these terms.
*Correspondence: Rayna J. Gonzales, cmpnb256YWxAYXJpem9uYS5lZHU=
Disclaimer: All claims expressed in this article are solely those of the authors and do not necessarily represent those of their affiliated organizations, or those of the publisher, the editors and the reviewers. Any product that may be evaluated in this article or claim that may be made by its manufacturer is not guaranteed or endorsed by the publisher.
Research integrity at Frontiers
Learn more about the work of our research integrity team to safeguard the quality of each article we publish.