- 1College of Plant Protection, China Agricultural University, Beijing, China
- 2Key Laboratory of Surveillance and Management for Plant Quarantine Pests, Ministry of Agriculture and Rural Affairs, Beijing, China
- 3Institute of Sanya, China Agricultural University, Sanya, China
Objective: Bactrocera dorsalis (Hendel) has a wide host range. It has been the most important quarantine pest in many countries or regions. Currently, chemical control and bait trapping are mainly used in the monitoring, prevention, and control of B. dorsalis. However, chemical control will cause pollution of the environment and drug resistance of insects. Methyl eugenol, the main attractant currently used, can only attract males of B. dorsalis.
Methods: This study focused on the attractant function and active substances of one key intestinal bacterium, Enterobacter cloacae, which was isolated from B. dorsalis.
Results: First, the attraction of the E. cloacae autoclaved supernatant to male and female adults of 0, 6, and 15 days post-emergence was confirmed using a Y-type olfactometer. Subsequently, through metabolome sequencing and bioassays, L-prolinamide was identified and confirmed as the most effective attractant for B. dorsalis. Finally, the synergistic effect of L-prolinamide with the sex attractant ME was validated through field experiments. This study confirmed the attraction effect of E. cloacae on B. dorsalis and also proved the attraction effect of L-prolinamide, the metabolite of E. cloacae, on B. dorsalis. This laid a theoretical foundation for the development of a new attractant and safe, green, and efficient prevention and control technology of B. dorsalis.
1 Introduction
Bactrocera dorsalis (Hendel) belongs to the Diptera (Tephritidae) family. Due to the direct damage it causes to fruits and vegetables and its negative effect on imports and exports, B. dorsalis has become one of the world’s most destructive agricultural pests and a major impediment to international fresh commodity trade (Qin et al., 2018). It has caused massive economic losses to agricultural production in tropical and subtropical countries or regions. Bactrocera dorsalis can damage over 40 families and 250 genera of fruits and vegetables due to its extensive host range (Liu et al., 2017). It causes fruit rot and premature fruit drop due to female adults laying eggs in the fruits and larvae eating the fruits before pupating (Qin et al., 2018; Verghese et al., 2012; Chen and Ye, 2007). Therefore, the prevention and management of this notorious pest are of great significance. At present, chemical, biological, and agricultural methods are employed, with chemical control prevailing due to its higher efficiency. Trapping males using methyl eugenol (ME), the main sexual attractant used currently, is also an effective method for population control. However, chemical control can also cause pollution to the environment and induce a high level of drug resistance in insects. Moreover, attractants can only attract males. Therefore, it is necessary to explore new environmentally friendly control methods and new efficient attractants.
Many microbes play a critical role in the growth and evolution of insects (Genta et al., 2006; Wang et al., 2011). Many studies have shown that four major phyla of intestinal bacteria, namely, Bacteroidetes, Actinobacteria, Firmicutes, and Proteobacteria, were found in the intestine of B. dorsalis (Hadapad et al., 2019; Colman et al., 2012; Liu et al., 2016). Several gut microbes have an attractive effect on fruit flies and play an essential role in the chemical exchange between individuals. Wang et al. (2014) have identified the intestinal bacteria of B. dorsalis adults by the PCR-DGGE method and confirmed the attraction effect of these bacteria on B. dorsalis. Their results indicated that Enterobacteriaceae, Enterococcaceae, and Bacillariophyceae had attraction effects on B. dorsalis, among which Enterobacter cloacae had a better attraction for B. dorsalis. It has been reported that the volatile substance 2-butanone produced by Klebsiella pneumoniae, Citrobacter fumigatus, and E. cloacae can attract B. dorsalis (Jang and Nishijima, 1990). It has also been found that metabolites of K. pneumoniae, K. oxytoca, and Raoultella terrigena isolated from the reproductive system of female B. dorsalis had an attraction effect on B. dorsalis, but the active substance responsible has not yet been confirmed (Shi et al., 2012; Shi et al., 2012).
Using attractants to control and prevent fruit flies can reduce the use of chemicals and, hence, alleviate the problems caused by pesticide resistance. Based on different attracting targets, attractants include male, female, and bisexual attractants. Most of the current male attractants are plant-derived volatiles or paraphylactones, which are usually used to attract males in the field or to monitor populations (Vargas et al., 2010), among which ME, ME analogs, Capilure, trimedlure, Zingerone [4-(4-hydroxy-3-methoxyphenyl)-2-butanone], and cuelure (CL) are the main known species (Zhang, 1991; Park et al., 2020). Until now, ME can be obtained from more than 450 kinds of plant releases, and it has been the most widely used B. dorsalis sex attractant (Tan et al., 2011). More than 80 species of fruit fly males, including B. carambolae, B. correcta, B. invadens, and B. dorsalis, are strongly attracted to ME (Tan et al., 2011; Shelly, 2010). A study has shown that the ME ingested by males can be converted into several metabolites, which are stored in their glands and act as components of the male sex pheromone (Nishida et al., 1988). In addition, ME plays an important role in enhancing the mating ability and total protein content of B. dorsalis males (Ji et al., 2013; Reyes-Hernández et al., 2019). Intake of ME by B. carambolae males will strengthen the sexual selection of poplar fruit flies (Wee et al., 2007). CL was initially noted to have a significant attraction effect on Zeugodacus cucurbitae, and the subsequent test revealed that raspberry ketone was not as effective as CL (Beroza et al., 1960; Keiser et al., 1973; Drew, 2010). However, ME and CL poorly attract some fruit fly males, such as B. xanthodes (Broun) and B. halfordiae (Royer et al., 2019a; Royer et al., 2019b). It was also demonstrated that some eugenol analogs (isoeugenol, ME, and dihydroeugenol) and zinc ketone were more effective male attractants than CL or ME for some fruit flies, with ME showing an effective attraction more than 50 times higher than ME in attracting Bactrocera xanthodes (Royer et al., 2019b; Royer et al., 2018). The United States Department of Agriculture has developed trimedlure (TML), which has been widely used as an attractant for Ceratitis capitata. TML is an isomeric mixture of 4-(and 5-) chloro-2-methylcyclo-hexane-1-carboxylate. Among them, (1R,2R,4S) tert-butyl 4-chloro-2-methylcyclohexane-1-carboxylate was considered to be the most effective attractant (Jang et al., 2005), and its analog ceralure has also been found to be effective in attracting C. capitata. However, due to the difficulty of its synthesis, the most widely used attractant for Mediterranean fruit fly is still TML (Jang et al., 2005). However, most of these attractants are male attractants and can only attract males. There are also some female or bisexual attractants that can attract females or both males and females. These attractants are usually intraspecific pheromones, host volatiles (Fein et al., 1982), food source attractants (Heath et al., 1995), associated bacterial ferments, and secondary metabolites (Drew et al., 1983). Most of the reported intraspecific pheromones with female attraction potential were sex pheromones, which were obtained by extracting rectal gland isolates and were usually composed of pheromones released by male adults (Zhang et al., 2019). The role of female fruit flies is critical for population growth. Hence, despite the effectiveness of ME in attracting male fruit flies, a need exists for attractants that can target both sexes and reduce the environmental impact. Enterobacter cloacae and its metabolites may offer advantages in the attractant market by providing a stronger and longer-lasting attractant effect with lower environmental impact and production costs. Additionally, the natural sources and unique mechanisms of action of these metabolites could make them an effective and sustainable alternative. Thus, research on female or bisexual attractants is very important for the development of new green environment-friendly control technology of fruit flies.
In this study, a laboratory-reared population was utilized to investigate the attraction effect of E. cloacae, a key intestinal bacterium isolated from B. dorsalis, on host fruit flies at various developmental stages. Active substances with attraction properties were screened through metabolome analysis of the supernatant from E. cloacae. Synergistic effect of the active substances on ME was also investigated through a field experiment. This study reported for the first time that L-prolinamide has an attraction effect on B. dorsalis, and it has a synergistic effect on the sexual attractant named ME, which is important for further development of green and efficient attractants.
2 Materials and methods
2.1 Insects
Adults of B. dorsalis were initially collected from Guangzhou, Guangdong province, and the populations have been reared in the laboratory for more than 15 generations. Larvae were fed with artificial diets following the description of Bai et al. (2019). The adults were fed with a powdered diet (peptone: sucrose = 1: 3) after emergence and provided with deionized water using moist cotton balls. The larvae (4-day-old) and adult males and females (0, 6, and 15 days post-emergence) of laboratory-reared B. dorsalis were collected for this experiment.
2.2 Bacteria
Bactrocera dorsalis adults at 10 days post-eclosion were selected and washed with sterile enzyme-free water to clean their body surfaces. Sequentially, surface disinfection was performed using the 1% sodium hypochlorite solution and 75% ethanol solution, each for 1 min, followed by three washes with enzyme-free sterile water to remove residual solutions. The flies were then placed on ice for storage. In a sterile Petri dish, PBS buffer solution was added, and the midgut was dissected using dissecting forceps. The gut and remaining tissues were placed in separate sterile centrifuge tubes, with five guts combined as one biological replicate. After grinding in liquid nitrogen, PBS was added to create a grinding solution. Subsequently, 100 μL of the gut-grinding solution was pipetted onto NA culture medium and spread evenly. Following incubation at 37°C for 2 days, single bacterial colonies on the plates were purified. After two rounds of purification, single colonies were selected and cultured in the NB liquid medium. Bacterial DNA was extracted using a bacterial genome DNA extraction kit (Tiangen, Beijing, China), amplified using universal bacterial 16S rDNA primers (Table 1), and aligned with NCBI sequences. Physiological and biochemical identification kits specific to Enterobacteriaceae (SHBG08, Qingdao Hope Bio-Technology Co., Ltd., China) confirmed the strain as E. cloacae. Enterobacter cloacae was mixed with glycerin and stored at −80°C until reused. The strain can be revived using LB liquid medium before being used. The bacterial solution was obtained after being cultured for 48 h under the culture condition of 37°C and 220 r/min.
2.3 Attracting ability of E. cloacae on larvae of B. dorsalis
Four kinds of artificial food were used for this experiment, including food which has been eaten by the same group of larvae (A), food which has been eaten by another group of larvae (B), fresh food with the E. cloacae supernatant added (C), and fresh food with nothing added as control (D). The formula and the condition of the food are listed in Table 2. A, B, and C are the experimental groups, and D is the control group. Five grams of food from the experiment group (A, B, or C) and control group (D) were put in the two sides of a 9-cm culture dish, respectively. Five 4-day-old larvae were placed at the mid-point between the two kinds of food each time, while their feeding choices were recorded. The condition that the larvae arrive at one group and feed, without secondary selection, can be recorded as a valid selection. Ten replicates were set up for each group (Figure 1).
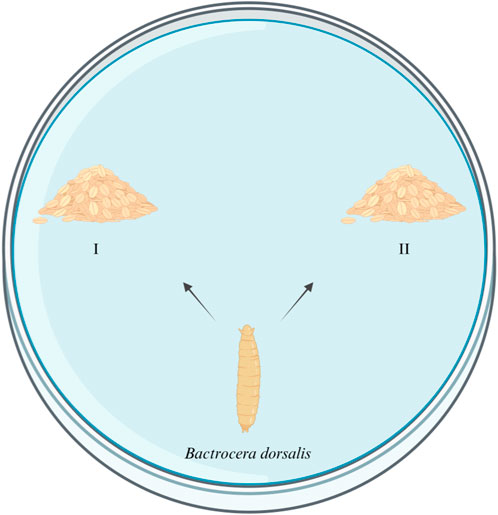
Figure 1. Attraction test of E. cloacae on the larvae of B. dorsalis. I: experimental group. II: control group.
2.4 Attracting ability of E. cloacae on adults of B. dorsalis with different developing periods
Two kinds of attractant and double-distilled water were used for this test, including the E. cloacae supernatant (A), autoclave sterilized E. cloacae supernatant (B), and double-distilled water as control (C). The detailed composition of these attractants are listed in Table 3. A self-made Y-type olfactometer was used to test the attraction effect on male and female B. dorsalis at 0, 6, and 15 days post-emergence. The self-made Y-type olfactory instrument is composed of an air pump, a pressure regulator tank (Outstanding, China), a gas flowmeter (Shuanghuan, China), an activated carbon tube, a sample bottle, and a Y-type tube (Crystal Technical, China). The Y-type tube has a handle length of 20 cm, an arm length of 10 cm, and an angle of 60°. The two arms of the Y-type tube are equipped with fluorescent lamps. It was ensured that both arms have the same light intensity. The protocol is shown in Figure 2, and the airflow rate was maintained at 100 mL/min. The condition that the adult entered the Y-tube for more than 1 cm and stayed for more than 15 s was recorded as a valid selection. After 30 valid selections in each group, the device was disassembled and rinsed with anhydrous ethanol and 75% alcohol and dried. Then, the sample bottles were switched, and the test was repeated. There were three replicates recorded for each developing period and sex. This part of the study involved the use of larvae (4-day-old) and adult males and females (0, 6, and 15 days post-emergence) of B. dorsalis. Each group had 30 valid samples.
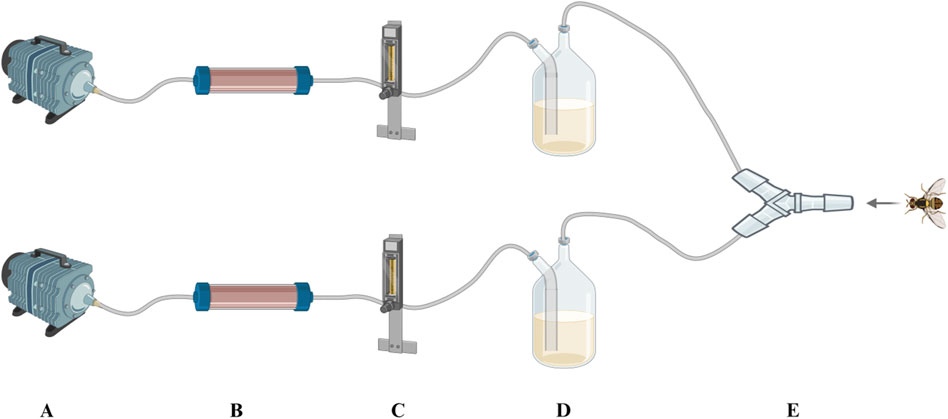
Figure 2. Self-made Y-type olfactometer. (A) Air pump, (B) active carbon filter, (C) gas flowmeter, (D) reagent bottle, and (E) Y-tube.
2.5 Preparation of metabolome samples of E. cloacae
The bacteria solution, after being cultured for 48 h (37°C, 220 r/min) using LB liquid media, was centrifuged at 10,000 r/min for 15 min, and 200 mL of the supernatant was used as sample 1. An additional 200 mL of the supernatant was autoclaved at 121°C for 20 min and used as sample 2. There were six replicates for each sample. Finally, the processed samples were used for the LC–MS non-targeted metabolomics study (Shanghai Majorbio Bio-Pharm Technology Co., Ltd.). A measure of 25 mg of solid sample was added to a 2-mL centrifuge tube, and a 6-mm-diameter grinding bead was added. An amount of 400 μL of the extraction solution [methanol: water = 4:1 (v:v)] containing 0.02 mg/mL of the internal standard (L-2-chlorophenylalanine) was used for metabolite extraction. Samples were ground by the Wonbio-96c (Shanghai Wanbo biotechnology co., Ltd.) frozen tissue grinder for 6 min (−10°C, 50 Hz), followed by low-temperature ultrasonic extraction for 30 min (5°C, 40 kHz). The samples were left at −20°C for 30 min, centrifuged for 15 min (4°C, 13,000 g), and the supernatant was transferred to the injection vial for LC–MS/MS analysis. An amount of 20 μL of the supernatant was removed from each sample and mixed as a quality control sample (QC) with the same volume as the sample. The QC samples were disposed and tested in the same manner as the analytic samples. It helped represent the whole sample set, which would be injected at regular intervals (every 5–15 samples) in order to monitor the stability of the analysis.
2.6 Analysis of the metabolome of Enterobacter cloacae
2.6.1 Liquid chromatography (LC)–mass spectrometry (MS)/MS analysis
The LC–MS/MS analysis of the sample was conducted on a Thermo UHPLC-Q Exactive HF-X system equipped with an ACQUITY HSS T3 column (100 mm × 2.1 mm i.d., 1.8 μm; Waters, United States) at Majorbio Bio-Pharm Technology Co. Ltd. (Shanghai, China). The mobile phases consisted of 0.1% formic acid in water: acetonitrile (95:5, v/v) (solvent A) and 0.1% formic acid in acetonitrile: isopropanol: water (47.5:47.5, v/v) (solvent B). The flow rate was 0.40 mL/min, and the column temperature was 40°C. The injection volume was 3 μL.
The mass spectrometric data were collected using a Thermo UHPLC-Q Exactive HF-X Mass Spectrometer equipped with an electrospray ionization (ESI) source operating in the positive mode and negative mode. The optimal conditions were set as follows: aux gas heating temperature at 425°C; capillary temp at 325°C; sheath gas flow rate at 50 psi; aux gas flow rate at 13 psi; ion-spray voltage floating (ISVF) at −3,500 V in negative mode and 3,500 V in positive mode; normalized collision energy, 20–40–60 eV rolling for MS/MS. The full MS resolution was 60,000, and the MS/MS resolution was 7,500. Data acquisition was performed in the data-dependent acquisition (DDA) mode. The detection was carried out over a mass range of 70–1,050 m/z.
2.6.2 Substance identification and analysis
The UHPLC-MS raw data were converted into the common format by Progenesis QI software (Waters, Milford, United States) through baseline filtering, peak identification, peak integral, retention time correction, and peak alignment. Then, the data matrix containing sample names, m/z, retention time, and peak intensities was exported for further analyses. At the same time, the metabolites were identified by searching the databases, and the main databases were the HMDB (http://www.hmdb.ca/), Metlin (https://metlin.scripps.edu/), and the self-compiled Majorbio Database (MJDB) of Majorbio Biotechnology Co., Ltd. (Shanghai, China).
The data matrix obtained by searching databases was uploaded to the Majorbio cloud platform (https://cloud.majorbio.com) for data analysis. First, the data matrix was pre-processed as follows: at least 80% of the metabolic features detected in any set of samples was retained. After filtering, the minimum value in the data matrix was selected to fill the missing value, and each metabolic signature was normalized to the sum. To reduce the errors caused by sample preparation and instrument instability, the response intensities of the sample mass spectrometry peaks were normalized using the sum normalization method to obtain the normalized data matrix. Meanwhile, the variables of QC samples with relative standard deviation (RSD) > 30% were excluded and log10 logarithmicized to obtain the final data matrix for subsequent analysis. Then, through R software ropls tools, the data matrix of input samples was used for PCA analysis, which generally reflected the metabolic differences among samples in each group and the variation degree among samples in the group (Cala et al., 2018). OPLS-DA analysis was used to eliminate noise information that is not relevant to classification and also obtain relevant metabolite information that causes significant differences between the two groups (Cala et al., 2018). To visualize the content of metabolites, metabolites were clustered according to their content in each sample. At the same time, metabolites were classified according to their participation in the pathway or the function they perform using the KEGG database. The metabolically concentrated metabolites are displayed on the KEGG pathway map (Li et al., 2018).
2.7 Field experiment
The field experiment was conducted in Yazhou, Sanya City, Hainan Province, from October to November of 2023. A total of eight traps, where four traps were the control group and another four traps were the treatment group, were randomly hung on one of the branches in the sunshade side of the green canopy. The hanging height was between 1 m and 1.5 m from the ground. For control groups, only the absorbent cotton, in which 3 mL ME was added, was placed in the small tank. However, for the treatment group, in addition to absorbent cotton with 3 mL ME, the bottom was filled with 100 mL L-prolinamide solution with a final concentration of 3%. The traps were hung on the same branch, and the distance between the control and treatment group was approximately 0.5 m. The numbers, species, and the sex ratio of flies were investigated every 7 days, and the traps were changed. There were four biological replications for this experiment, and the experiment was repeated four times.
2.8 Statistical analysis
SPSS statistical analysis software was used to analyze the relevant experimental data using nonparametric tests. S was used as the selection rate, and the calculation method is explained herein. The term “average number of B. dorsalis attracted” refers to the number of adults who enter the Y-tube side for more than 1 cm and stay for more than 15 s. The term “total number of B. dorsalis” refers to the 30 valid selections of the adult in each group.
3 Results
3.1 Response of 4-day-old B. dorsalis larvae to various types of artificial food
As shown in Figure 3, compared to CK, the 4-day-old larvae preferred to feed on treated artificial food. The food that has been fed by other insects for 4 days showed the highest selectivity (88.00%, P < 0.01), followed by the fresh food prepared with E. cloacae solution (72%, P < 0.01) and the food that has been fed by themselves for 4 days (66.00%, p = 0.015 < 0.05).
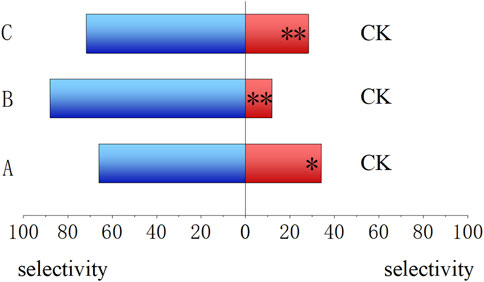
Figure 3. Feeding trend of Bactrocera dorsalis larvae for different larval feeds. (A) Food that has been fed by themselves for 4 days. (B) Food that has been fed by the larvae other than themselves for 4 days. (C) Fresh food prepared using the Enterobacter cloacae solution. (CK) Fresh food prepared using ddH2O. *: P < 0.05; **: P < 0.01.
3.2 Attraction effect of E. cloacae on adult B. dorsalis
Compared to double-distilled water and the E. cloacae supernatant, the autoclaved supernatant of E. cloacae showed a significantly increased attraction ability for adults of B. dorsalis at 0, 6, and 15 days post-emergence, including females (Figures 4A, E) and males (Figures 4B, F). Additionally, the number of B. dorsalis attracted by double-distilled water was significantly higher than that attracted by the supernatant of bacteria, except for 6-day-old females (Figures 4C, D).
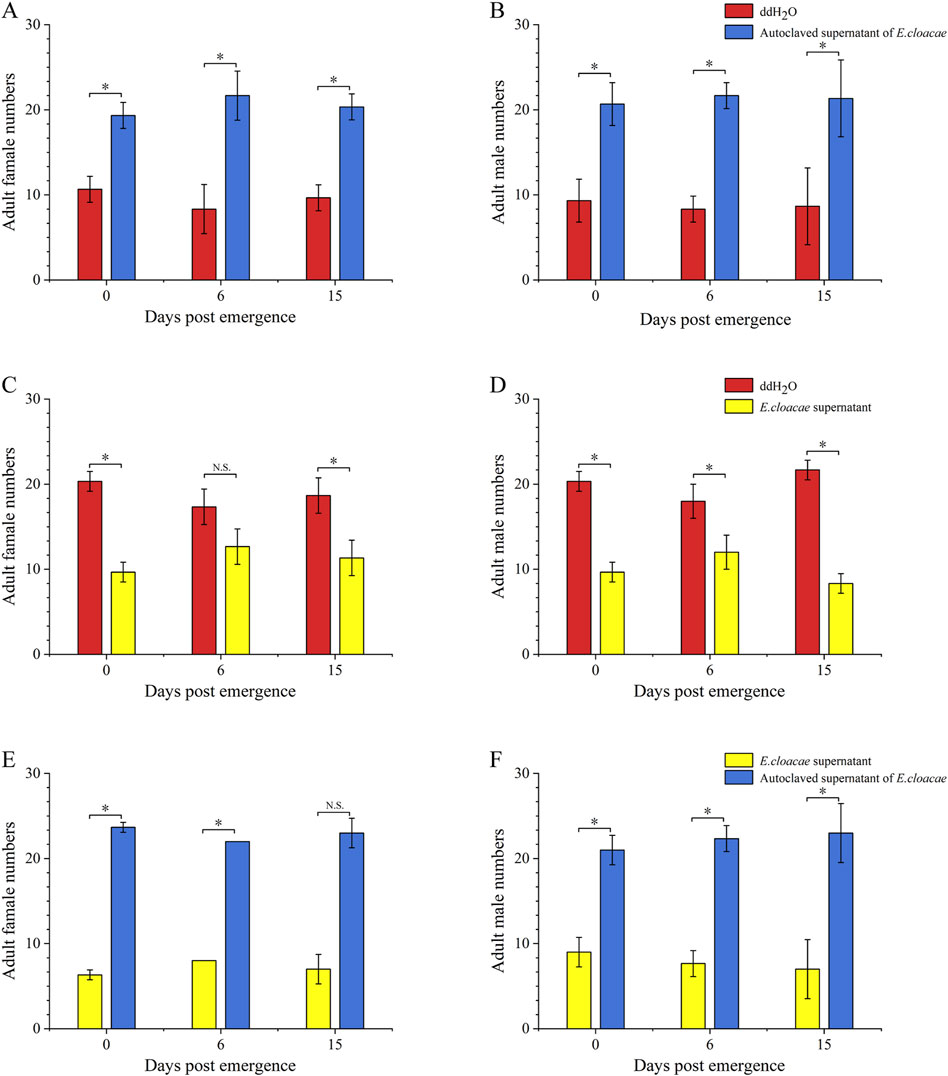
Figure 4. Attractant of Enterobacter cloacae to adults of Bactrocera dorsalis. Red: ddH2O; yellow: supernatant of Enterobacter cloacae; blue: autoclaved supernatant of E. cloacae. (A): attraction of Enterobacter cloacae supernatant which has been autoclave sterilized to female B. dorsalis. (B): attraction of E. cloacae supernatant which has been autoclave sterilized to male B. dorsalis. (C): attraction of E. cloacae supernatant to female B. dorsalis. (D): attraction of E. cloacae supernatant male B. dorsalis. (E): attraction of E. cloacae supernatant which has been autoclave sterilized and E. cloacae supernatant to female B. dorsalis. (F): attraction of E. cloacae supernatant which has been autoclave sterilized and E. cloacae supernatant to male of B. dorsalis. *: P < 0.05; **: P < 0.01.
3.3 Comparative metabolome analysis of the supernatant and autoclaved supernatant of E. cloacae
3.3.1 PCA and orthogonal partial least-squares discrimination (OPLS-DA) analysis
As shown in Supplementary Figure S1, the total ion overlap chromatograms of the quality control samples in the positive and negative ion modes had good peak shapes and relatively uniform distribution, which represent a high quality of the metabolome. The PCA analysis allows us to observe the separation trend between groups and the presence of outliers in the experimental model and reflects the inter- and intra-group variability from the raw data. The treated group was farther apart from the control, and the biological repetitions in the treated or control group are closer together (Figure 5A).
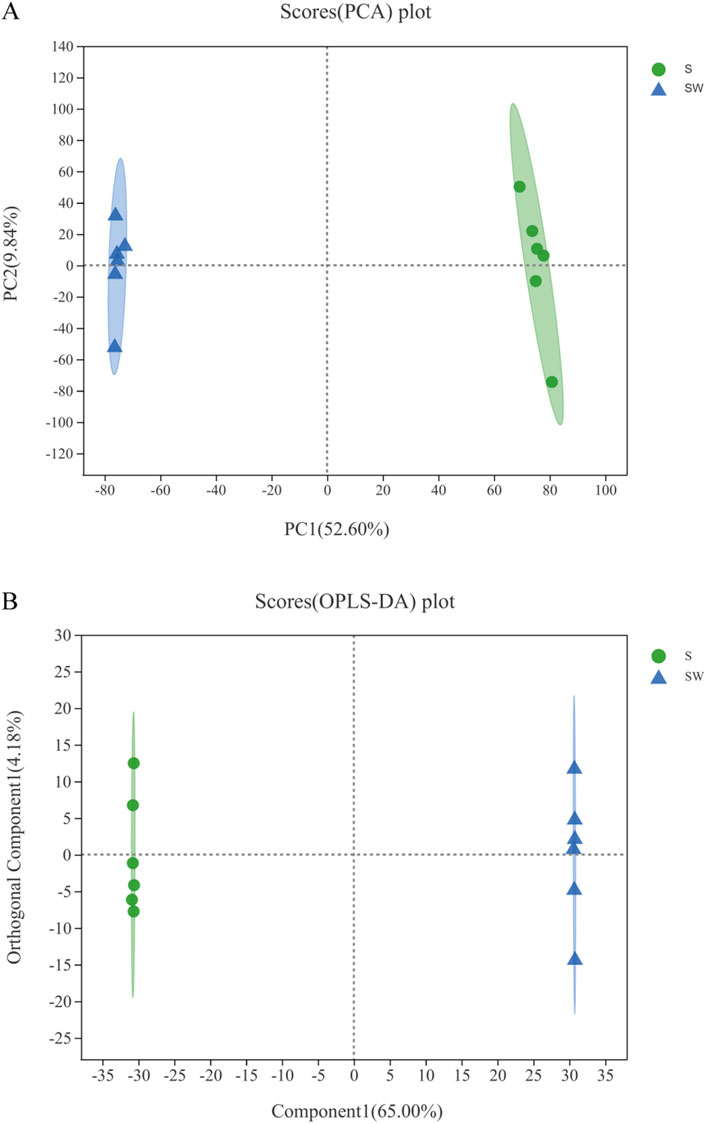
Figure 5. Scatter points of PCA (A). Dispersion points of OPLS-DA (B). (S) Experimental group: Enterobacter cloaca supernatant that has been autoclave sterilized. (SW) Control group: Enterobacter cloaca supernatant. The confidence ellipse represents the group of “true” samples with 95% confidence, and the samples beyond this region can be considered as possible abnormal samples.
The OPLS-DA method can distinguish the test group from the control group (R2X = 0.65, R2Y = 1, Q2 = 0.995) with good stability, as shown in Figure 5B. All samples in the test and control groups were within the 95% confidence interval, indicating that the accuracy of these data is relatively high. Both PCA and OPLS-DA analysis showed that the differences between the test and control groups are more pronounced and the intra-group replicates are more stable.
3.3.2 Heatmap analysis of differential metabolites
Differential metabolites between the autoclaved supernatant and supernatant of E. cloacae were screened based on univariate statistical analysis and VIP values. A total of 302 differential metabolites were screened (P < 0.05 and VIP values > 1), of which 208 were upregulated (VIP > 1 and fold change > 1) and 94 were downregulated (VIP > 1 and 0 < fold change < 1) (Figure 6A). Among them, FC > 1 and the metabolites with clear species are provided in Supplementary Table S1.
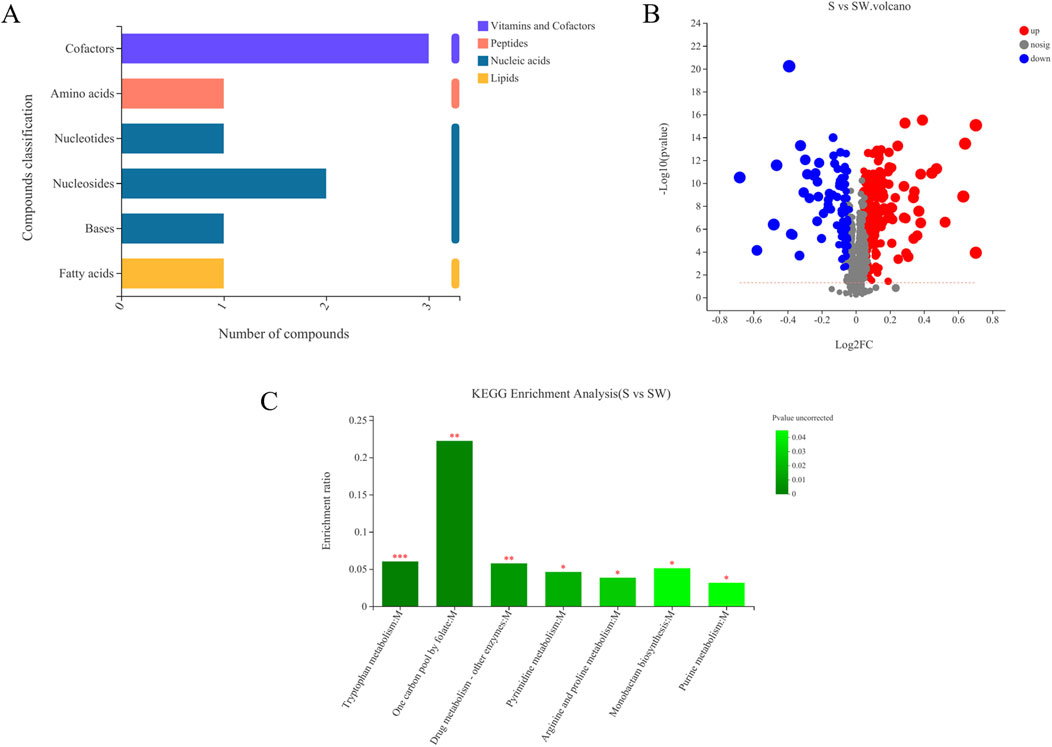
Figure 6. Volcanic map of differential metabolites in different samples (A). (S) Experimental group (Enterobacter cloaca supernatant that has been autoclave sterilized). (SW) Control group (E. cloaca supernatant). Classification of KEGG compounds (B). The ordinate is the secondary classification category of KEGG compounds. The horizontal coordinate is the number of metabolites annotated to this classification. KEGG pathway enrichment of differential metabolites in experimental group and metabolite (C). The abscissa represents the pathway name, and the ordinate represents the enrichment rate, indicating the ratio of the number of metabolites enriched in this pathway to the number of metabolites annotated into the pathway. The greater the ratio, the greater the degree of enrichment. The color gradient of the column represents the significance of enrichment, and the darker the color, the more significant the enrichment of the KEGG term. P value < 0.001 is marked with ***, P value < 0.01 is marked with **, and P value < 0.05 is marked with *.
Among the 81 upregulated metabolites, carboxylic acids and their derivatives were the most abundant, accounting for 18 species. The following were pregnenolone lipids, accounting for 14 species. The others were triterpenoids, sphingolipids, peptides, nitrogen-containing carboxylic acid derivatives, and amides, respectively (Supplementary Table S1). KEGG compound is a collection of small molecules, biopolymers, and other chemicals associated with biological systems. As shown in Figure 6B, the differential metabolites can be classified into four kinds of metabolites, including lipids, nucleic acids, peptides, vitamins, and cofactors. Myristic acid was present in the lipids. There were four nucleic acids, among which were thymidine, deoxyuridine, 5′-CMP, and (2R,3R,4S,5R)-2-(6-aminopurin-9-yl)-5-(hydroxymethyl) oxolane-3,4-diol. There was one peptide, L-tyrosine. There were three vitamins and coenzyme factors, namely, S-adenosylmethionine, pyrroloquinoline quinone, and tetrahydrofolate (Figure 7; Supplementary Table S2).
3.3.3 KEGG enrichment analysis of differential metabolites
Based on the KEGG enrichment analysis, it was found that the different metabolites in the test and control groups were mainly enriched in the following pathways: tryptophan metabolism, one carbon pool by folate, drug metabolism—other enzymes (pyrimidine metabolism), purine metabolism, monobactam biosynthesis, and arginine and proline metabolism pathways. The one carbon pool by folate pathway was the most enriched (Figure 6C).
3.3.4 Heatmap analysis of differential metabolites
Heatmaps constructed based on differential metabolites between the supernatant and autoclaved supernatant of E. cloacae showed a clear clustering (Supplementary Figure S2). The autoclaved supernatant showed a higher attracting ability than the E. cloacae supernatant, which helped us focus on the upregulated differential metabolite. Figure 6D shows the top 30 differential metabolites, which contain L-prolinamide.
3.4 Screening and identification of the attractive active substance of E. cloacae against B. dorsalis
Based on previous studies, most of the substances found to have attractive activity are pyrazines, amines, ammonia, alcohols, ketones, acids, and phenols. Three different metabolites, namely, 3-indoleacetic acid, 2-hydroxycinnamic acid, and L-prolinamide, were selected for the attractive ability test. These three substances had similar functional groups to methyl salicylate, ME, and N-(3-methylbutyl) acetamide (Table 4), whose attractive ability to B. dorsalis has been confirmed.
Compared to untreated fresh food, 3-indoleacetic acid-treated food and L-prolinamide-treated food showed a significantly lower attractive ability to the 4-day-old larvae, with an attraction rate of 35.00% (P < 0.01) and 31.77% (P < 0.01), respectively. The selection rate of 2-hydroxycinnamic acid-treated food (53.33%) and untreated fresh food (46.67%) by 4-day-old larvae had no significant difference (Figure 8). Meanwhile, for the attraction ability of these three substances on female and male adults, L-prolinamide was effective in attracting both newly emerged, 6-day, and 15-day-old males and females, and the number of B. dorsalis attracted in the treatment group was significantly higher than that in the control group (Figures 9A, B). 3-Indoleacetic acid showed attractive ability to 15-day-old males (Figures 9C, D), while 2-hydroxycinnamic acid only showed attractive ability to 6-day-old females (Figures 9E, F).
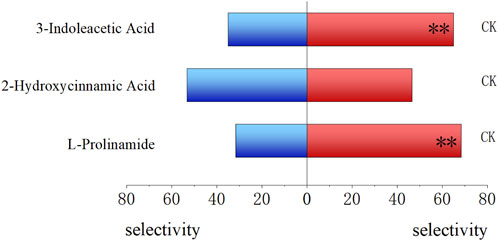
Figure 8. Attraction ability of L-prolinamide, 2-hydroxycinnamic acid, and 3-indoleacetic acid on the larvae of Bactrocera dorsalis. Red: control group, where larvae feed on the food normally added with ddH2O. Blue: experiment group, where larvae feed on the food added with L-prolinamide, 2-hydroxycinnamic acid, and 3-indoleacetic acid, respectively. *: P < 0.05; **: P < 0.01.
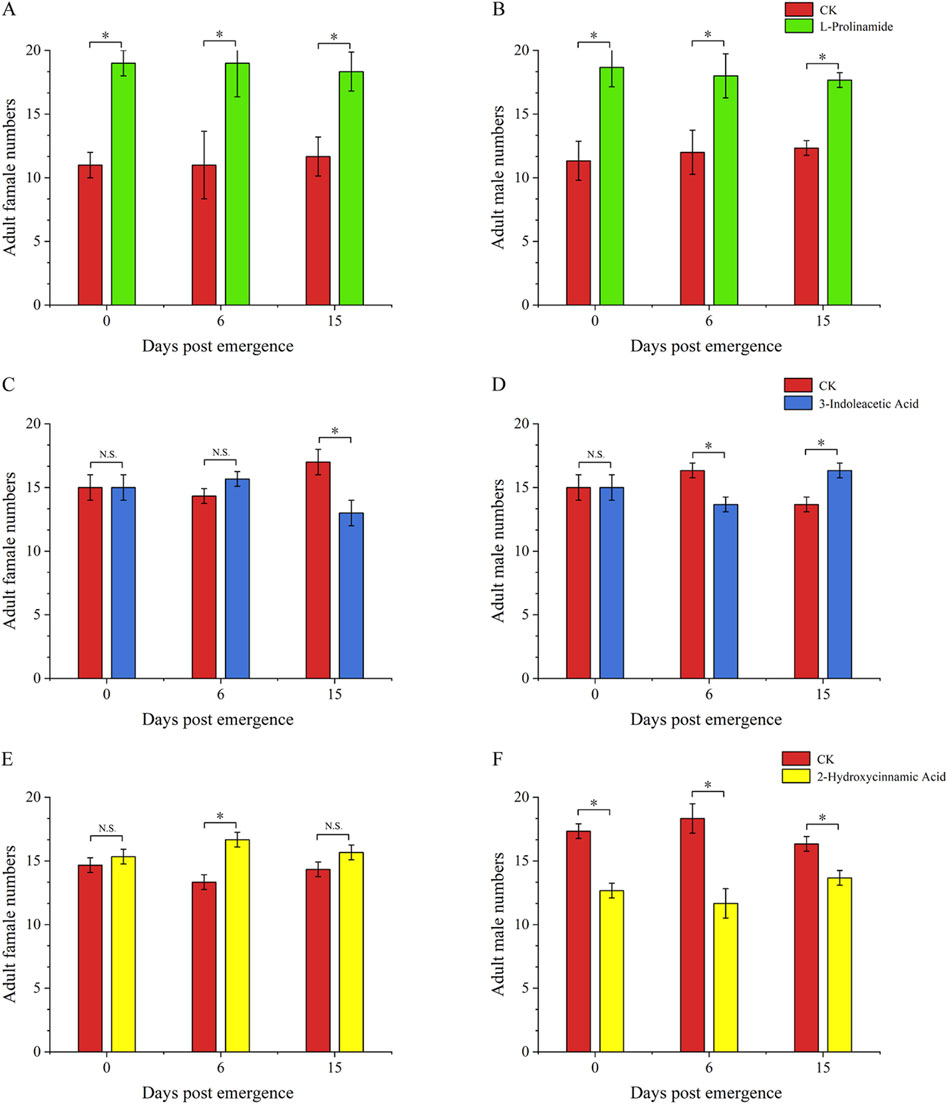
Figure 9. Attraction ability of L-prolinamide, 2-hydroxycinnamic acid, and 3-indoleacetic acid to adult Bactrocera dorsalis. Red: control group, where larvae feed on the food normally added with ddH2O. Blue: experiment group, where larvae feed on the food normally added with 3-indoleacetic acid. Green: experiment group, where larvae feed on the food normally added with L-prolinamide. Yellow: experiment group, where larvae feed on the food normally added with 2-hydroxycinnamic acid. (A) Attraction results of L-Prolinamide to female Bactrocera dorsalis at different ages; (B) Attraction results of L-Prolinamide to male Bactrocera dorsalis at different ages; (C) Attraction ability of 3-Indoleacetic Acid to female Bactrocera dorsalis at different; (D) Attraction ability of 3-Indoleacetic Acid to male Bactrocera dorsalis at different; (E) Attraction ability of 2-Hydroxycinnamic acid to female Bactrocera dorsalis at different ages; (F) Attraction ability of 2-Hydroxycinnamic acid to male Bactrocera dorsalis at different ages. *: P < 0.05. **: P < 0.01.
3.5 Potential use of L-prolinamide as the synergistic agents of sexual attractants in the field
As shown in Figure 10, the trapping rate of L-prolinamide with a concentration of 3% was significantly higher than that of the control group (n = 16, P < 0.05). Therefore, L-prolinamide with 3% concentration had a synergistic effect on ME for the attraction of B. dorsalis. A few females were trapped in the treatment group for our pre-experiment, which was constructed in Sanya, Hainan province, in July 2022. However, only 2–7 females were trapped, and it did not display a significant difference.
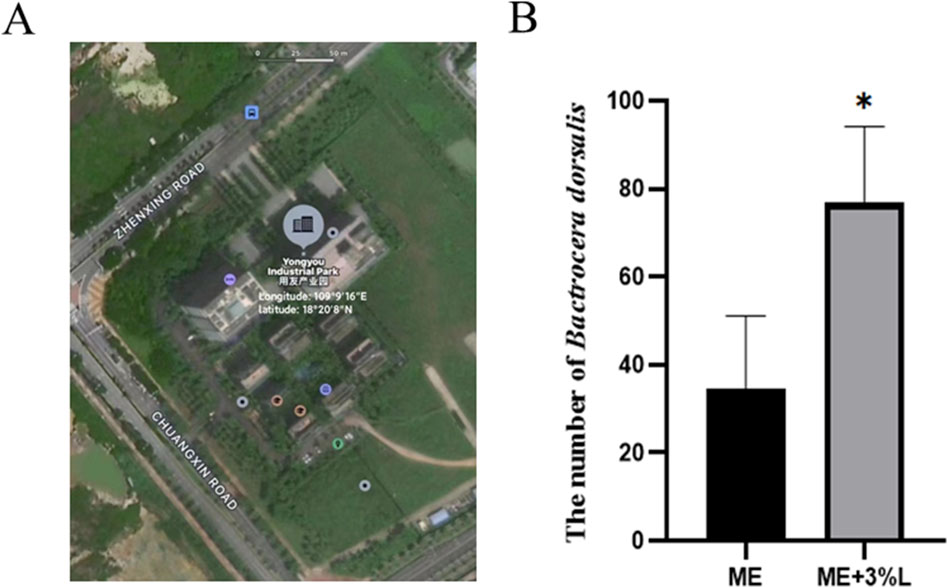
Figure 10. Synergistic effect of L-prolinamide on ME. (A) Geographical distribution of the site for field experiment: Yongyou Industrial Park (Longitude:109°9′16″E; latitude: 18°20′8″N); (B): the number of B. dorsalis that were trapped using ME and ME mixed with L-prolinamide at a concentration of 3%, respectively. ME, methyl eugenol. ME, control group, only the absorbent cotton, in which 3 mL ME was added, was placed in the small tank for trapping. ME + 3% L: treatment group, besides absorbent cotton with 3 mL ME, the bottom was filled with 100 mL L-prolinamide solution with a final concentration of 3%. The traps were hung on the same branch, and the distance between the control and treatment group was approximately 15 m. N = 16. *: P < 0.05.
4 Discussion
Wang et al. (2014) identified the attracting ability of gut microbes in adult B. dorsalis, and the results showed that Enterobacteraceae, Enterococcaceae, and Bacillaceae have attracting effects on adult B. dorsalis, among which E. cloacae has a better effect on host fruit fly. In addition, Bacillus cereus, Enterococcus faecalis, Citrobacter fumigatus, Klebsiella oxytoca, and K. pneumoniae have been reported to be effective in attracting B. dorsalis (Jang and Nishijima, 1990; Shi et al., 2012; Shi et al., 2012). Enterobacter cloacae also has an attractive effect on other fruit flies. Lauzon et al. showed that E. cloacae, isolated from the feces of Rhagoletis pomonella, has a strong attraction effect on R. pomonella (Lauzon et al., 1998). In addition, E. cloacae has an attractive effect on Zeugodacus cucurbitae, B. papayae, and B. zonata (Narit and Anuchit, 2011; Reddy et al., 2014). Although the attraction ability of many gut bacteria has been confirmed, the difference of attraction ability among different bacteria species and the specific substances that play a role are unknown. In our research, the results of the larval feeding tendency experiment showed that the 4-day-old larvae were more likely to feed on food that has been fed by themselves or other insects for 4 days, and they also liked the food prepared by the E. cloacae solution. Therefore, we speculated that B. dorsalis larvae may secrete a substance or pheromone during feeding, which attracted other larvae or themselves to feed. For the attraction to the adult females and males at 0, 6, and 15 days post-emergence, the autoclaved supernatant of E. cloacae displayed the highest attraction efficiency. Like our study, it has been shown that E. cloacae has a strong attraction effect on 8-day-old and sexually mature South Asian fruit flies. Additionally, research has demonstrated that the autoclaved supernatant is more effective at attracting the flies than the fermentation stock (Luo, 2016).
It has been reported that ammonia, several amines, imines, pyrazines, and acetic acid in the headspace above the filtrate of the bacterial cultures were determined to be attractive to Anastrepha ludens (Robacker and Garcia, 1993; Robacker and Flath, 1995). Hereby, we speculated that the fermentation broth of E. cloacae can produce a stable secondary metabolite that has an attraction activity on B. dorsalis after being autoclaved. Meanwhile, our results showed that there was no significant difference in the attraction effect of the autoclaved supernatant of E. cloacae on female and male adults of different ages. This suggests that the active substances responsible for attraction produced by autoclaved E. cloacae are not linked to egg laying, sexual maturation, or sex pheromone synthesis. It has been demonstrated that the bacterial medium can provide the fruit flies with carbohydrates and, in turn, can affect the attractiveness of bacteria to fruit flies (Reddy et al., 2014). Meanwhile, the protein-fed or mated fruit flies respond similarly to associated bacterial odors, regardless of the presence or absence of the host fruit (Narit and Anuchit, 2011). Therefore, the attractive substances produced by E. cloacae may be some kind of protein or amino acid needed for the growth and development of B. dorsalis rather than substances related to sexual maturation and egg production. Therefore, when screening metabolites, combined with previous studies, we guessed that the attractant is the amine-containing substance. Studies of such substances provide a potential use of them as attractants or attractants synergist in the field, which can avoid the disadvantage that some attractants used now only attract males.
Related studies have indicated that most of the substances that have an attraction effect on B. dorsalis are substances containing ammonia, pyrazine, amines, alcohols, and phenols. Furthermore, sulfides, ketones, and acids have been reported to be able to attract some fruit fly’s adults (Hadapad et al., 2016). The bacterial volatile components contained in Anastrepha ludens also showed significant attraction and were confirmed to contain mainly ammonia, amines, pyrazines, and acids (Robacker and Flath, 1995; Lee et al., 1995; DeMilo et al., 1996; Robacker and Bartelt, 1997; Robacker et al., 1998). Meanwhile, a related study has suggested that the reason some bacteria can have an attraction effect on fruit flies may be due to the production of ammonia by bacterial metabolisms. Meanwhile, amide, as a derivative of ammonia, may have an attraction effect because adult B. dorsalis feeds on it as a nitrogen source. Our results were consistent with these reports and findings. Among the three substances we chose, none of them showed attractive ability on larvae, and only L-prolinamide, a kind of amide, showed significantly higher attractive ability on the adult females and males, regardless the development stage.
Although previous studies have shown that most of the substances with an attractive effect on insects are alcohols, phenols, alkanes, and ketones, L-prolinamide has been less studied. Currently, studies have focused on the attraction effect of some other amides, such as N-(3-methylbutyl) acetamide and oleamide. It was evident that N-(3-methyl-butyl) acetamide induces electroantennagraphical response in different stages and sex combinations in B. minax by measuring citrus fruit volatiles and the electroantennographical response of B. minax (Li, 2020). A study found that the compound N-(3-methylbutyl) acetamide was the main volatile component in the venom of many female vespid wasps. The attraction ability of N-(3-methylbutyl) to Polistes genera has also been verified by field tests. The acetamide attracted male and female P. aurifer and P. metricus, as well as male P. dorsalis and P. bellicosus (Elmquist e al., 2020). The endogenous components of B. carambolae males were identified and analyzed for 6-oxo-1-nonanol and N-3-methylbutylacetamide, and subsequent studies showed that these endogenous components were able to attract a large number of females when released into the air as visible smoke (Wee and Tan, 2005). It was also found that 1 ng/μL of oleamide had a strong priming effect on the Curculio chinensis and identified a regulatory effect of oleamide on its behavior (Yan et al., 2023). In addition to attracting both males and females, L-prolinamide showed a high synergistic effect on the attraction ability of ME by a field test. Its potential use as attractants will be evaluated in the future. This laid a theoretical foundation for the development of new attractants and safe, green, and efficient prevention and control technology of B. dorsalis.
Guo et al. (2021) identified the volatile compounds of Klebsiella oxytoca and found that 3-methyl-1-butanol only attracted male Drosophila suzukii. In contrast, this study has identified a bacterium and its active compound that attract both male and female flies, addressing the current limitation where most attractants only lure males. Additionally, exploring the potential mechanism of E. cloacae and L-prolinamide in attracting B. dorsalis is critical for pest control. In Drosophila melanogaster, the sexually dimorphic central neural pathways associated with the odorant receptor DmelOR67d, which detects cVA, illustrate how olfactory sensory neurons and their projection neurons shape behavior (Datta et al., 2008; Ruta et al., 2010). Zhang et al. (2024) proposed a working model in which ME enhances the attractiveness of leks in attracting females to an arena to control B. dorsalis mating behavior, providing a scientific foundation for understanding the mechanisms of the male annihilation technique and future improvements. Given that ME are currently primarily used for managing adult male pests, investigating the potential mechanisms by which E. cloacae and L-prolinamide attract female B. dorsalis can provide valuable insights for developing pest management strategies. Therefore, further research into these mechanisms could establish a theoretical basis for developing new, environmentally friendly attractants and improving overall control efficacy. In the future, integrating research on E. cloacae and its metabolites into current pest management strategies should focus on their broader ecological and pest control impacts. These metabolites demonstrate significant environmental friendliness and protect ecosystem biodiversity compared to traditional chemical attractants. Furthermore, combining these with existing attractants like ME could achieve more effective pest control measures (Cai et al., 2018).
In summary, in this study, the attraction effect of E. cloacae, a gut bacterial strain isolated from B. dorsalis, and one of its metabolites, L-prolinamide, on host fruit fly was demonstrated. It was confirmed that there was no significant difference between the attraction effect of L-prolinamide on male and female adults, which can compensate for the weakness that most of the current attractants, such as ME, can only attract males, and it can provide a new view for environmental protection and efficient control of B. dorsalis. However, among so many differential metabolites, only three substances have been selected to investigate the attraction ability; more differential metabolites should be selected for attraction effect verification, and the comparison among these substances and their synergistic effect of existing attractants still need to be confirmed. Additionally, it is necessary to further investigate the mechanism of the attraction effect of E. cloacae and its metabolites on B. dorsalis to provide a theoretical basis for the development of novel green control technologies for this notorious pest.
Data availability statement
The original contributions presented in the study are included in the article/supplementary material, further inquiries can be directed to the corresponding author.
Author contributions
LL: conceptualization, funding acquisition, methodology, project administration, validation, visualization, and writing–review and editing. YD: conceptualization, formal analysis, methodology, software, validation, writing–original draft, and writing–review and editing. AL: software, writing–original draft, and writing–review and editing. LZ: validation and writing–original draft. CY: validation and writing–original draft. ZL: writing–review and editing.
Funding
The authors declare financial support was received for the research, authorship, and/or publication of this article. This study received financial support from the National Key Research and Development Project (2022YFC2601500; 2022YFC2601503).
Acknowledgments
The authors would like to thank all the members of the Plant Quarantine Laboratory of China Agricultural University (CAUPQL). The data were analyzed through the free online platform of Majorbio cloud platform (http://cloud.majorbio.com). The authors also thank all reviewers for their comments on this manuscript.
Conflict of interest
The authors declare that the research was conducted in the absence of any commercial or financial relationships that could be construed as a potential conflict of interest.
Publisher’s note
All claims expressed in this article are solely those of the authors and do not necessarily represent those of their affiliated organizations, or those of the publisher, the editors, and the reviewers. Any product that may be evaluated in this article, or claim that may be made by its manufacturer, is not guaranteed or endorsed by the publisher.
Supplementary material
The Supplementary Material for this article can be found online at: https://www.frontiersin.org/articles/10.3389/fphys.2024.1465946/full#supplementary-material
References
Bai Z., Liu L., Noman M. S., Zeng L., Luo M., Li Z. (2019). The influence of antibiotics on gut bacteria diversity associated with laboratory-reared Bactrocera dorsalis. B. Entomol. Res. 5, 500–509. doi:10.1017/S0007485318000834
Beroza M., Alexander B. H., Steiner L. F., Mitchell W. T., Miyashita D. H. (1960). New synthetic lures for the male melon fly. Science 131, 1044–1045. doi:10.1126/science.131.3406.1044
Cai X. M., Li Z. Q., Pan H. S., Lu Y. H. (2018). Research and application of food-based attractants of herbivorous insect pests. Chin. J. Biol. Contr. 34, 8–35. doi:10.16409/j.cnki.2095-039x.2018.01.002
Cala M. P., Agulló-Ortuño M. T., Prieto-García E., González-Riano C., Parrilla-Rubio L., Barbas C., et al. (2018). Multiplatform plasma fingerprinting in cancer cachexia: a pilot observational and translational study. Multiplatform plasma fingerprinting cancer cachexia. J. Cachexia Sarcopeni. 9, 348–357. doi:10.1002/jcsm.12270
Chen P., Ye H. (2007). Population dynamics of Bactrocera dorsalis (Diptera: tephritidae) and analysis of factors influencing populations in Baoshanba, Yunnan, China. Entomol. Sci. 10, 141–147. doi:10.1111/j.1479-8298.2007.00208.x
Colman D. R., Toolson E. C., Takacs-Vesbach C. D. (2012). Do diet and taxonomy influence insect gut bacterial communities? Mol. Ecol. 21, 5124–5137. doi:10.1111/j.1365-294X.2012.05752.x
Datta S. R., Vasconcelos M. L., Ruta V., Luo S., Wong A., Demir E., et al. (2008). The Drosophila pheromone cVA activates a sexually dimorphic neural circuit. Nature 452, 473–477. doi:10.1038/nature06808
DeMilo A. B., Lee C. J., Moreno D. S., Martinez A. J. (1996). Identification of volatiles derived from Citrobacter freundii fermentation of a trypticase soy broth. J. Agr. Food Chem. 44, 607–612. doi:10.1021/jf950525o
Drew R. A. I. (2010). The responses of fruit fly species (Diptera: tephritidae) in the south pacific area to male attractants. Aust. J. Entomol. 13, 267–270. doi:10.1111/j.1440-6055.1974.tb.02206.x
Drew R. A. I., Courtice A. C., Teakle D. S. (1983). Bacteria as a natural source of food for adult fruit flies (Diptera: tephritidae). Oecologia 60, 279–284. doi:10.1007/BF00376839
Elmquist D. C., Landolt P. J., Cooper W. R., Reed H., Foutz J., Clepper T., et al. (2020). The venom compound N-(3-methylbutyl) acetamide attracts several Polistes (fuscopolistes) species (hymenoptera: vespidae). J. Econ. Entomol. 113, 1073–1079. doi:10.1093/jee/toaa065
Fein B. L., Reissig W. H., Roelofs W. L. (1982). Identification of apple volatiles attractive to the apple maggot, Rhagoletis pomonella. J. Chem. Ecol. 8, 1473–1487. doi:10.1007/BF00989104
Genta F. A., Dillon R. J., Terra W. R., Ferreira C. (2006). Potential role for gut microbiota in cell wall digestion and glucoside detoxification in Tenebrio molitor larvae. J. Insect Physiol. 52, 593–601. doi:10.1016/j.jinsphys.2006.02.007
Guo Y. Y., Tan D. Y., Shi H. M., Wu X. Y., Yu Y., Li T. G., et al. (2021). Attractiveness of intestinal bacterium Klebsiella oxytoca and its volatile substances to Drosophila suzukii adults. Acta Entomol. Sin. 64, 1161–1167. doi:10.16380/j.kcxb.2021.10.005
Hadapad A. B., Prabhakar C. S., Chandekar S. C., Tripathi J., Hire R. S. (2016). Diversity of bacterial communities in the midgut of Bactrocera cucurbitae (Diptera: tephritidae) populations and their potential use as attractants. Pest Manag. Sci. 72, 1222–1230. doi:10.1002/ps.4102
Hadapad A. B., Shettigar S. K., Hire R. S. (2019). Bacterial communities in the gut of wild and mass-reared Zeugodacus cucurbitae and Bactrocera dorsalis revealed by metagenomic sequencing. BMC Microbiol. 19, 282–311. doi:10.1186/s12866-019-1647-8
Heath R. R., Epsky N. D., Guzman A., Dueben B. D., Manukian A., Meyer W. L. (1995). Development of a dry plastic insect trap with food-based synthetic attractant for the Mediterranean and Mexican fruit flies (Diptera: tephritidae). J. Econ. Entomol. 88, 1307–1315. doi:10.1093/jee/88.5.1307
Jang E. B., Khrimian A., Holler T. C., Casana-Giner V., Lux S., Carvalho L. A. (2005). Field response of Mediterranean fruit fly (Diptera: tephritidae) to ceralure B1: evaluations of enantiomeric B1 ratios on fly captures. J. Econ. Entomol. 98, 1139–1143. doi:10.1603/0022-0493-98.4.1139
Jang E. B., Nishijima K. A. (1990). Identification and attractancy of bacteria associated with dacus-dorsalis (Diptera: tephritidae). Environ. Entomol. 19, 1726–1731. doi:10.1093/ee/19.6.1726
Ji Q. E., Chen J. H., McInnis D. O., Guo Q. L. (2013). The effect of methyl eugenol exposure on subsequent mating performance of sterile males of Bactrocera dorsalis. J. Appl. Entomol. 137, 238–243. doi:10.1111/j.1439-0418.2011.01686.x
Keiser I., Nakagawa S., Kobayashi R. M., Chambers D. L., Uraco T., Doolittle R. E. (1973). Attractiveness of cue-lure and the degradation product 4-(p-hydroxyphenyl)-2-butanone to male melon flies in the field in Hawaii. J. Econ. Entomol. 66, 112–114. doi:10.1093/jee/66.1.112
Lauzon C. R., Sjogren R. E., Wright S. E., Prokopy R. J. (1998). Attraction of Rhagoletis pomonella (Diptera: tephritidae) flies to odor of bacteria: apparent confinement to specialized members of Enterobacteriaceae. Environ. Entomol. 27, 853–857. doi:10.1093/ee/27.4.853
Lee C. J., DeMilo A. B., Moreno D. S., Martinez A. J. (1995). Analysis of the volatile components of a bacterial fermentation that is attractive to the mexican fruit-fly, Anastrepha ludens. J. Agr. Food Chem. 43, 1348–1351. doi:10.1021/jf00053a041
Li k. (2020). Identification of host plant volatiles and pheromones and their electroantennogram responses of Bactrocera minax. Northwest A&F University. MA Thesis.
Li Y., Fang J., Qi X., Lin M., Zhong Y., Sun L., et al. (2018). Combined analysis of the fruit metabolome and transcriptome reveals candidate genes involved in flavonoid biosynthesis in actinidia arguta. t J. Mol. Sci. 9, 1471. doi:10.3390/ijms19051471
Liu H., Zhao X. F., Fu L., Han Y. Y., Chen J., Lu Y. Y. (2017). BdorOBP2 plays an indispensable role in the perception of methyl eugenol by mature males of Bactrocera dorsalis (Hendel). Sci. Rep. 7, 15894–15914. doi:10.1038/s41598-017-15893-6
Liu L. J., Martinez-Sañudo I., Mazzon L., Prabhakar C. S., Girolami V., Deng Y. L., et al. (2016). Bacterial communities associated with invasive populations of Bactrocera dorsalis (Diptera: tephritidae) in China. B. Entomol. Res. 106, 718–728. doi:10.1017/S0007485316000390
Luo M. J. (2016). Molecular diversity analysis of the intestinal bacterial communities from adult Bactrocera tau (Walker) and their trapping effect. Thesis FAFU’s master student.
Narit T., Anuchit C. (2011). Attraction of Bactrocera cucurbitae and B. papayae (Diptera: tephritidae) to the odor of the bacterium Enterobacter cloacae. Philipp. Agric. Sci. 94, 1–6. doi:10.5423/PPJ.2011.27.1.044
Nishida R., Tan K. H., Fukami H. (1988). Cis-3,4-dimethoxycinnamyl alcohol from the rectal glands of male Oriental fruit fly, Dacus dorsalis. Chem. Express 3, 207–210.
Park S. J., De Faveri S. G., Cheesman J., Hanssen B. L., Cameron D. N., Jamie I. M., et al. (2020). Zingerone in the flower of Passiflora maliformis attracts an Australian fruit fly, Bactrocera jarvisi (tryon). Molecules 25, 2877. doi:10.3390/molecules25122877
Qin Y. J., Krosch M. N., Schutze M. K., Zhang Y., Wang X. X., Prabhakar C. S., et al. (2018). Population structure of a global agricultural invasive pest, Bactrocera dorsalis (Diptera: tephritidae). Evol. Appl. 11, 1990–2003. doi:10.1111/eva.12701
Reddy K., Sharma K., Singh S. (2014). Attractancy potential of culturable bacteria from the gut of peach fruit fly, Bactrocera zonata (Saunders). Phytoparasitica 42, 691–698. doi:10.1007/s12600-014-0410-9
Reyes-Hernández M., Thimmappa R., Abraham S., Pagadala Damodaram K. J., Pérez-Staples D. (2019). Methyl eugenol effects on Bactrocera dorsalis male total body protein, reproductive organs and ejaculate. J. Appl. Entomol. 143, 177–186. doi:10.1111/jen.12576
Robacker D. C., Bartelt R. J. (1997). Chemicals attractive to Mexican fruit fly from Klebsiella pneumoniae and Citrobacter freundii cultures sampled by solid-phase microextraction. J. Chem. Ecol. 23, 2897–2915. doi:10.1023/A:1022579414233
Robacker D. C., Flath R. A. (1995). Attractants from Staphylococcus aureus cultures for Mexican fruit fly, Anastrepha ludens. J. Chem. Ecol. 21, 1861–1874. doi:10.1007/BF02033682
Robacker D. C., Garcia J. A. (1993). Effects of age, time of day, feeding history, and gamma irradiation on attraction of Mexican fruit flies (Diptera: tephritidae), to bacterial odor in laboratory experiments. Environ. Entomol. 22, 1367–1374. doi:10.1093/ee/22.6.1367
Robacker D. C., Martinez A. J., Garcia J. A., Bartelt R. J. (1998). Volatiles attractive to the Mexican fruit fly (Diptera: tephritidae) from eleven bacteria taxa. Fla. Entomol. 81, 497–508. doi:10.2307/3495948
Royer J. E., Khan M., Mayer D. G. (2018). Methyl-isoeugenol, a highly attractive male lure for the cucurbit flower pest Zeugodacus diversus (Coquillett) (syn. Bactrocera diversa) (Diptera: tephritidae: Dacinae). J. Econ. Entomol. 111, 1197–1201. doi:10.1093/jee/toy068
Royer J. E., Mille C., Cazeres S., Brinon J., Mayer D. G. (2019a). Isoeugenol, a more attractive male lure for the cue-lure-responsive pest fruit fly Bactrocera curvipennis (Diptera: tephritidae: Dacinae), and new records of species responding to zingerone in New Caledonia. J. Econ. Entomol. 112, 1502–1507. doi:10.1093/jee/toz034
Royer J. E., Teakle G. E., Ahoafi E., Mayer D. G. (2019b). Methyl-isoeugenol, a significantly more attractive male lure for the methyl eugenol-responsive Pacific fruit fly, Bactrocera xanthodes (Diptera: tephritidae). Austral. Entomol. 58, 800–804. doi:10.1111/aen.12398
Ruta V., Datta S. R., Vasconcelos M. L., Freeland J., Looger L. L., Axel R. (2010). A dimorphic pheromone circuit in Drosophila from sensory input to descending output. Nature 468, 686–690. doi:10.1038/nature09554
Shelly T. (2010). Effects of methyl eugenol and raspberry ketone/cue lure on the sexual behavior of Bactrocera species (Diptera: tephritidae). Appl. Entomol. Zool. 45, 349–361. doi:10.1303/aez.2010.349
Shi W., Kerdelhué C., Ye H. (2012a). Genetic structure and inferences on potential source areas for Bactrocera dorsalis (hendel) based on mitochondrial and microsatellite markers. Plos One 7, e37083. doi:10.1371/journal.pone.0037083
Shi Z., Wang L., Zhang H. (2012b). Low diversity bacterial community and the trapping activity of metabolites from cultivable bacteria species in the female reproductive system of the oriental fruit fly, Bactrocera dorsalis hendel (Diptera: tephritidae). Int. J. Mol. Sci. 13, 6266–6278. doi:10.3390/ijms13056266
Tan K. H., Tokushima I., Ono H., Nishida R. (2011). Comparison of phenylpropanoid volatiles in male rectal pheromone gland after methyl eugenol consumption, and molecular phylogenetic relationship of four global pest fruit fly species: Bactrocera invadens, B. dorsalis, B. correcta and B. zonata. Chemoecology 21, 25–33. doi:10.1007/s00049-010-0063-1
Vargas R. I., Shelly T. E., Leblanc L., Pinero J. C. (2010). Recent advances in methyl eugenol and cue-lure technologies for fruit fly detection, monitoring, and control in Hawaii. Vitamins Hormones 83, 575–595. doi:10.1016/S0083-6729(10)83023-7
Verghese A., Soumya C. B., Shivashankar S., Manivannan S., Krishnamurthy S. V. (2012). Phenolics as chemical barriers to female fruit fly, Bactrocera dorsalis (Hendel) in mango. Curr. Sci. 103, 563–566.
Wang H., Jin L., Peng T., Zhang H., Chen Q., Hua Y. (2014). Identification of cultivable bacteria in the intestinal tract of Bactrocera dorsalis from three different populations and determination of their attractive potential. Pest Manag. Sci. 70, 80–87. doi:10.1002/ps.3528
Wang H., Jin L., Zhang H. (2011). Comparison of the diversity of the bacterial communities in the intestinal tract of adult Bactrocera dorsalis from three different populations. J. Appl. Microbiol. 110, 1390–1401. doi:10.1111/j.1365-2672.2011.05001.x
Wee S. L., Tan K. H. (2005). Female sexual response to male rectal volatile constituents in the fruit fly, Bactrocera carambolae (Diptera: tephritidae). Appl. Entomol. Zool. 40, 365–372. doi:10.1303/aez.2005.365
Wee S. L., Tan K. H., Nishida R. (2007). Pharmacophagy of methyl eugenol by males enhances sexual selection of Bactrocera carambolae. J. Chem. Ecol. 33, 1272–1282. doi:10.1007/s10886-007-9295-0
Yan X. Z., Wang Z. Y., Ma L., Song C. F., Zhao J. Y., Wang C. Z., et al. (2023). Electroantennogram and behavioural responses of Plutella xylostella to volatiles from the non-host plant Mentha spicata. J. Appl. Entomol. 147, 511–519. doi:10.1111/jen.13144
Zhang J., Liu W., Chang H., Wang Q., Yuan J., Liu L., et al. (2024). Methyl eugenol regulates mating behavior in oriental fruit flies by enhancing lek attractiveness. Natl. Sci. Rev., nwae294. doi:10.1093/nsr/nwae294
Keywords: oriental fruit fly, gut microorganism, Enterobacter cloacae, attractant effect, L-prolinamide, synergistic effect
Citation: Duan Y, Li A, Zhang L, Yin C, Li Z and Liu L (2024) Attractant potential of Enterobacter cloacae and its metabolites to Bactrocera dorsalis (Hendel). Front. Physiol. 15:1465946. doi: 10.3389/fphys.2024.1465946
Received: 17 July 2024; Accepted: 03 September 2024;
Published: 03 October 2024.
Edited by:
Ana Claudia A. Melo, Federal University of Rio de Janeiro, BrazilReviewed by:
Junaid Ali Siddiqui, Guizhou University, ChinaBamisope Steve Bamisile, South China Agricultural University, China
Copyright © 2024 Duan, Li, Zhang, Yin, Li and Liu. This is an open-access article distributed under the terms of the Creative Commons Attribution License (CC BY). The use, distribution or reproduction in other forums is permitted, provided the original author(s) and the copyright owner(s) are credited and that the original publication in this journal is cited, in accordance with accepted academic practice. No use, distribution or reproduction is permitted which does not comply with these terms.
*Correspondence: Lijun Liu, bGpsaXVAY2F1LmVkdS5jbg==
†These authors have contributed equally to this work and share first authorship