- 1State Key Laboratory for Biology of Plant Diseases and Insect Pests, Key Laboratory of Natural Enemy Insects of Ministry of Agriculture and Rural Affairs, Institute of Plant Protection, Chinese Academy of Agricultural Sciences, Beijing, China
- 2School of Horticulture and Gardening, Tianjin Agricultural University, Tianjin, China
- 3Key Laboratory of Animal Biosafety Risk Prevention and Control (North) of Ministry of Agriculture and Rural Affairs, Shanghai Veterinary Research Institute, Chinese Academy of Agricultural Sciences, Shanghai, China
Thioredoxin (Trx), an important part of thioredoxin systems, plays crucial role in maintaining the intracellular redox balance by scavenging reactive oxygen species (ROS). However, few Trxs have been functionally characterized in Arma chinensis, especially in diapause. In this study, diapause induction condition promoted hydrogen peroxide accumulation and increased CAT enzymatic activity and ascorbate content, suggesting that A. chinensis was exposed to high level of ROS. Therefore, we identified AcTrx2 and AcTrx-like, and investigated the relationship with antioxidant defense. It was found that AcTrx2 expression was significantly induced, whereas AcTrx-like expression was the highest on day 10 under diapause conditions. The expression of AcTrx2 and AcTrx-like in fat body, a central metabolic organ of resisting oxidative stress, was significantly increased under diapause conditions, and was significantly improved by 5/15°C (diapause temperature). We investigated the knockdown of AcTrx2 and AcTrx-like in A. chinensis and found that some selected antioxidant genes were upregulated, indicating that the upregulated genes may be functional compensation for AcTrx2 and AcTrx-like silencing. We also found that the enzymatic activities of SOD and CAT, and the metabolite contents of hydrogen peroxide, ascorbate increased after AcTrx2 and AcTrx-like knockdown. These results suggested the AcTrx2 and AcTrx-like may play critical roles in antioxidant defense of A. chinensis diapause.
1 Introduction
Diapause is a developmental arrest stage in which insects respond to harsh environmental conditions, such as high summer temperatures, winter cold conditions, food limitation, or crowding (Guo et al., 2021a; Guo et al., 2021b). Lower temperature is usually used by insects to suppress their metabolism, conserve energy stores and gain cold resistance during winter diapause (Uzelac et al., 2024). However, lower temperatures can induce excessive reactive oxygen species (ROS), and high concentration of ROS has destructive effects on cells, including lipids, membranes, proteins, and nucleic acids (i.e., peroxide pressure) (Poli et al., 2004). In order to avoid the damage to cells caused by the toxicity of ROS, organisms have developed antioxidant enzymatic systems, including superoxide dismutase (SOD), thioredoxin peroxidase (TPx), peroxiredoxin (Prx) and thioredoxin (Trx) to maintain the intracellular ROS at proper levels (Kobayashi-Miura et al., 2007). Among these enzymes, Trx, a natural electron donor, is an important part of thioredoxin systems and plays essential roles in redox-regulatory processes (Kondo et al., 2006; Yoshioka et al., 2006; Lu and Holmgren, 2014).
Thioredoxin, a member of the small molecule reductase family, contains the typical disulfide bond active site Cys-Gly-Pro-Cys (CGPC), which is widely distributed from Archaea to human (Powis and Montfort, 2001; Lu and Holmgren, 2014). Since the first Trx is identified from Escherichia coli as a hydrogen donor for ribonucleotide reductase (Laurent et al., 1964), thioredoxin family genes have been identified and functionally studied in other living organisms, such as Drosophila melanogaster (Kanzok et al., 2001), Spodoptera litura (Kang et al., 2015), Helicoverpa armigera (Zhang et al., 2015), etc. Moreover, thioredoxin in mammals is mainly divided into two types, Trx1 and Trx2, which are located in the cytoplasm and mitochondria, respectively (Lu and Holmgren, 2014). Further studies have found that thioredoxin exerts antioxidant effects mainly through two aspects: one is as an electron donor of peroxidase, and the other is as a disulfide reductase of intracellular proteins (Kalinina et al., 2008).
Functionally, thioredoxin not only has the function of scavenging free radicals and protecting cells from oxidative stress (Chen et al., 2002; Revathy et al., 2012), but also has many physiological activities such as reducing hydrogen peroxide and regulating growth (Damdimopoulos et al., 2002). Studies on thioredoxin protecting organisms from ROS damage have focused on mammals, plants and bacteria (Benitez-Alfonso et al., 2009; Serata et al., 2012; Lee et al., 2013), but have been less studied in insects. In D. melanogaster, Trx1, Trx2, and TrxT have been cloned and identified (Bauer et al., 2002; Svensson et al., 2003), of which Trx2 has been shown to be involved in regulating redox response and modulating the lifespan of flies (Bauer et al., 2002; Svensson and Larsson, 2007). Similarly, in Apis cerana, three Trx genes (AccTrx-like1, AccTrx1, and AccTrx2) have been demonstrated to play an important role in antioxidant defense (Lu et al., 2012; Yao et al., 2013; Yao et al., 2014). HaTrx2, in H. armigera, can reduce ROS production and larvae death caused by nucleopolyhedrovirus (NPV) (Zhang et al., 2015), while GmTrx2 and GmTrx-like1 from Grapholita molesta can resist oxidative stress caused by emamectin benzoate (Shen et al., 2018). Although the functional researches of Trx have been extended from model insects such as D. melanogaster to lepidoptera insects, there are few reports about its role in insect diapause.
Arma chinensis is the dominant natural enemy of many agricultural and forestry pests, and has strong predation ability on more than 40 kinds of pests, such as Lepidoptera, Coleoptera, Hemiptera and Hymenoptera (Meng et al., 2022). Unlike the photoperiod induced diapause of Colaphellus bowringi (Guo et al., 2021a), the diapause of A. chinensis dependes on low temperature. According to previous studies, characteristic active-site motifs of Trx are highly conserved and involved resistance to low-temperature induced oxidative stress in mammals and other species (Lu and Holmgren, 2014). Therefore, we predicted that Trxs may play an important role in diapause. In this study, we examined antioxidant enzyme activities and metabolite amounts of A. chinensis under diapause conditions, and have identified AcTrx2 and AcTrx-like. Moreover, we further examined the temporal and spatial expression patterns of AcTrx2 and AcTrx-like under diapause and non-diapause conditions and the differences in expression at low temperatures. Finally, we used RNA interference (RNAi) to detect the function of AcTrx2 and AcTrx-like in resisting oxidative stress by detecting antioxidant enzyme activities, metabolite amounts, and the expression levels of other antioxidant genes. Our results provide a new perspective on the resistance mechanisms of AcTrx2 and AcTrx-like to oxidative stress in A. chinensis diapause and help to develop strategies to improve survival during diapause of natural enemy insects.
2 Materials and methods
2.1 Insects
Arma chinensis were reared in our laboratory with Antheraea pernyi pupa at a constant temperature of 26°C± 1°C, under 70 %± 5% RH and a 16L: 8 D photoperiod. Diapause condition: the female adults within 24 h of emergence were placed at light ratio of 8L:16 D with transitions of temperatures 5/15°C, 70% ± 5% RH.
2.2 Difference of redox levels of A. chinensis under non-diapause or diapause condition
To detect oxidation and antioxidant levels in A. chinensis under two conditions, enzymatic capacity of superoxide dismutase (SOD) and catalase (CAT), and metabolite content of hydrogen peroxide (H2O2), and ascorbate were measured. Samples were collected from adults reared under diapause conditions for 10 days or non-diapause conditions for 10 days. The BCA Protein Assay Kit (Nanjing Jiancheng Bioengineering Institute, Nanjing, China) were used to extract and quantify the protein. According to the instructions, the catalase test kit, superoxide dismutase test kit, hydrogen peroxide test kit, ascorbate test kit (Nanjing Jiancheng Bioengineering Institute, Nanjing, China) were used to assay SOD enzymatic capacity, CAT enzymatic capacity, H2O2 content, and ascorbate content, respectively.
2.3 Gene identification and sequence analysis
The thioredoxin sequences were derived from previous transcriptome data of Arma chinensis in our laboratory. The open reading frames (ORFs) of thioredoxin 2 (Trx2, PP820916) and thioredoxin-like (Trx-like, PP820915) were amplified using special primers (Supplementary Table S1) and then cloned into the pMD18-T vector (Takara Bio, Otsu, Japan) for sequencing (TSINGKE Bio, Beijing, China). Homologous protein sequences of AcTrx2 and AcTrx-like from other species were obtained from National Center for Biotechnology Information (NCBI) (Supplementary Tables S2, S3), and analyzed using DNAman 6.0.3 software. Conserved domains in AcTrx2 and AcTrx-like were predicted by NCBI services. Finally, MEGA v6.0 software was used to perform the phylogenetic analysis with the neighbor-joining method (1,000 bootstrap replicates).
2.4 Assays of RT-qPCR for temporal and spatial expression patterns
To detect the difference of temporal and spatial expression of AcTrx2 and AcTrx-like in diapause condition and non-diapause condition, adults reared for 0, 10, 20, 30, 40 days under diapause conditions and adults reared for 0, 3, 6, 9, 12 days under non-diapause conditions were collected, respectively. Three biological replicates were taken at each time point and each treatment contained five adults. Samples of different tissues were collected from adults reared under diapause conditions for 10 days or non-diapause conditions for 10 days, including head, fat body, midgut and ovary. Each sample was repeated 3 times and each tissue was dissected from at least eight adults.
Total RNA was extracted by using TRIzol reagent (Takara, Kyoto, Japan), and the first-strand of cDNA was synthesized with PrimeScript RT reagent kit with gDNA Eraser (Takara, Kyoto, Japan). According to the manufacturer’s instructions, RT-qPCR was performed on LightCycler® 96 Instrument (Roche (China) Holding Ltd., Shanghai, China) using cDNA and TB Green™ Premix Ex Taq™ (Takara, Kyoto, Japan) in a 20 µL reaction system. The primers used for RT-qPCR in this study were shown in Supplementary Table S1, and RPL27 gene was selected as endogenous reference gene. The results of qPCR were analyzed using the 2−ΔΔCT method (Livak and Schmittgen, 2001).
2.5 Effect of temperature on AcTrx2 and AcTrx-like expression
To determine the role of AcTrx2 and AcTrx-like in resistance to low temperature during diapause, we examined the expression of genes at 5°C, 15°C and 5/15°C (diapause temperature). Samples were collected from adults exposed to 5°C, 15°C and 5/15°C for 24, 48, and 72 h, and the adults exposed to 26°C were as controls. Each treatment contained three biological replicates and each replicate contained six adults. All samples were immediately stored at −80°C for total RNA extraction, cDNA synthesis and qPCR detection.
2.6 dsRNA synthesis and injection of AcTrx2 and AcTrx-like
To detect the function of AcTrx2 and AcTrx-like, the gene expression levels were knocked down using RNA interference (RNAi). Double-stranded RNAs (dsRNAs) of GFP, AcTrx2, and AcTrx-like were synthesized by using MEGAscript RNAi kit (Ambion, California, United States) and the purified target sequences, which were amplified by RT-PCR using cDNA and special primers containing T7 polymerase promoter sequence (Supplementary Table S1). The NanoDrop™ 2000 spectrophotometer and agarose gel electrophoresis were used to detect the concentration and quality of dsRNA.
For RNAi of AcTrx2 and AcTrx-like, 2-day postemergence adults were injected with dsRNA from each gene (2 μg/individual) by using a microinjector from the second and third abdominal segments. Samples were collected at 48 h after dsRNA injection. Adults were injected with dsGFP as a negative control. Biological replicates were performed 3 times in each treatment with at least 10 adults in each replicate. All samples were used for total RNA extraction, cDNA synthesis and RT-qPCR detection to examining gene interference efficiency.
2.7 Transcriptional levels of other antioxidant genes after knockdown of AcTrx2 and AcTrx-like
After AcTrx2 and AcTrx-like knockdown, three other antioxidant genes, including glutaredoxin 5 (Grx5), glutaredoxin domain-containing cysteine-rich protein (GrxCR), protein disulfide isomerase (PDI) were examined. RT-qPCR was used to detect the expression levels of Trx-like, GrxCR, Grx5, and PDI after AcTrx2 knockdown. Expression levels of Trx, GrxCR, Grx5, and PDI were determined following AcTrx-like silencing.
2.8 Analysis of enzymatic activity and metabolite content after knockdown of AcTrx2 and AcTrx-like
To determine the role of AcTrx2 and AcTrx-like in antioxidant function, we examined the enzymatic activities of superoxide dismutase (SOD) and catalase (CAT) and metabolite content of H2O2, and ascorbate after genes knockdown. Protein samples were collected at 48 h after dsRNA injection and were extracted and quantified with the BCA Protein Assay Kit (Nanjing Jiancheng Bioengineering Institute, Nanjing, China). Then, according to the instructions, a catalase test kit and a superoxide dismutase test kit (Nanjing Jiancheng Bioengineering Institute, Nanjing, China) were used to assay enzymatic capacity of SOD and CAT, respectively. Similarly, the metabolite content of H2O2, and ascorbate were quantified using hydrogen peroxide, ascorbate test kit (Nanjing Jiancheng Bioengineering Institute, Nanjing, China), respectively, referring to the instructions.
2.9 Statistical analysis
The SPSS 21 software was used to perform statistical analysis of experimental datas and values were represented as the means ± stand error of mean (SEM) with at least three independent repetitions. Gene expression in different tissues were analyzed using ANOVA, followed by a Turkey’s HSD multiple comparison test, with a and b indicating significant differences at p < 0.05. Other RT-qPCR results and the results of enzymatic activity and metabolite content were analyzed by using the pair-wise Student’s t-test, and significance levels were denoted by * (0.01 < p < 0.05) and ** (p < 0.01).
3 Results
3.1 Difference of oxidation level of A. chinensis between non-diapause and dipause conditions
To determine the oxidation level of A. chinensis under diapause conditions, the content of hydrogen peroxide (H2O2) and ascorbate, and the enzymatic activities of superoxide dismutase (SOD) and catalase (CAT) were examined. As shown in Figure 1, compared with no-diapause group, the H2O2 and ascorbate contents were significantly increased (Figures 1A,B), and the enzymatic activity of CAT was also significantly enhanced (Figure 1D), but there was no difference in SOD capacity (Figure 1C). The above results suggested that A. chinensis was exposed to ROS under diapause condition.
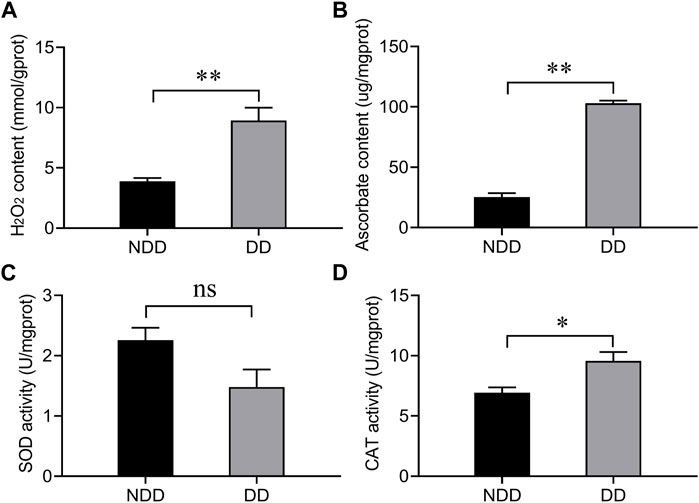
Figure 1. Determination of (A) hydrogen peroxide, (B) ascorbate, and the enzymatic activity of antioxidants (C) SOD, and (D) CAT under no-diapause and diapause conditions. Adults were collected at 10 days after emergence under no-diapause and diapause conditions, respectively. NDD: non-diapause-destined female refers to the adults were raised for 10 days in reproduction conditions; DD: diapause-destined female refers to the adults were raised for 10 days in diapause conditions. Each value represents the mean ± stand error of mean (SEM). Asterisks indicate significant differences between NDD and DD using Student’s t-test (*, 0.01 < p < 0.05; **, p < 0.01).
3.2 Cloning and sequence analysis of AcTrx2 and AcTrx-like
Two distinct cDNA fragments were identified from the transcriptome database of A. chinensis, and the two gene sequences were analyzed with different molecular characteristics using the NCBI-ORF Finder and NCBI-BLAST, subsequently named AcTrx2 and AcTrx-like. Sequence analysis showed that the open reading frame (ORF) of AcTrx2 and AcTrx-like was 324 bp and 381 bp, encoding 107 amino acids and 126 amino acids, respectively (Supplementary Figure S1, S2). Multiple sequence alignment revealed AcTrx2 was 57%–88% sequence identity with homologous amino acid sequences from other species and possessed a highly conserved CGPC active-site motif (Figure 2A). Low amino acid identity (10%–58%) between AcTrx-like and other Trx-like sequences including Halyomorpha halys, Nezara viridula, and Zeugodacus cucurbitae, and an active site sequence ALPC was found in the A. chinensis (Figure 2B). Phylogenetic analysis revealed that AcTrx2 was most closely related to the HhTrx2 homologue (Figure 3A), and AcTrx-like was the most closely related to HhTrx-like than other selected species (Figure 3B). These results were consistent with the predictive evolutionary relationship of multiple alignments of amino acid sequences.
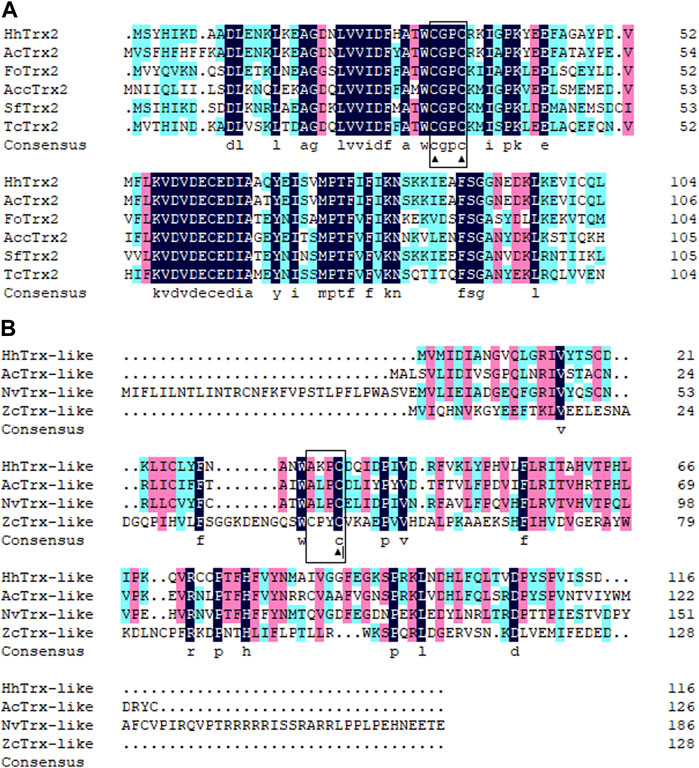
Figure 2. Multiple alignments of (A) AcTrx2 and (B) AcTrx-like with homologs from other insect species. The conserved CGPC motif and ALPC motif were boxed and the active sites were marked by ▲. The protein names and accession numbers were listed in Supplementary Table S2.
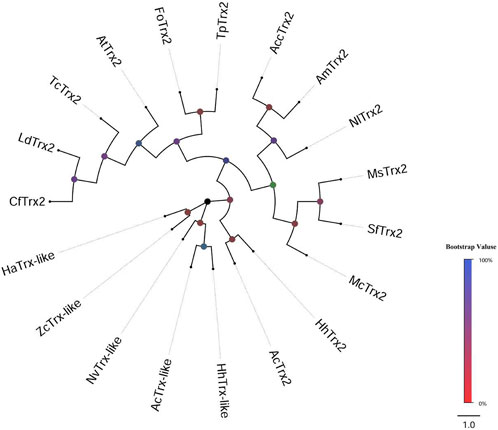
Figure 3. Phylogenetic analysis of AcTrx2 and AcTrx-like with homologs from other insect species. The phylogenetic tree was constructed based on amino acid sequences of AcTrx2 and AcTrx-like using the MEGA-based neighbor-joining method with 1,000 bootstraps. The protein names and accession numbers were listed in Supplementary Table S3.
3.3 Spatio-temporal expression profiles of AcTrx2 and AcTrx-like
RT-qPCR was used to investigate the transcriptional expression of AcTrx2 and AcTrx-like under non-diapause and diapause conditions at various developmental stages, and in different tissues of A. chinensis. The results of temporal expression profile showed that the expression of AcTrx2, in non-diapause conditions, decreased with the development time, and the highest expression appeared at 0 days of emergence (Figure 4A), while in diapause conditions, the expression of AcTrx2 increased gradually with developmental time, and the lowest expression was at 0 days of emergence (Figure 4C). AcTrx-like was highly expressed in 6, 9, and 12-day postemergence adults in non-diapause conditions (Figure 4B). However, under diapause conditions, the expression levels of AcTrx-like showed an overall upward trend, and the highest expression was at 10 days after A. chinensis emergence (Figure 4D). For spatial expression, AcTrx2 was the most highly expressed in head from A. chinensis adults in non-diapause conditions (Figure 4E), and the expression of AcTrx2 in the head and fat body was the highest in diapause condition, followed by the ovary (Figure 4G). AcTrx-like transcriptional expression was the highest in the ovary and the lowest in the head under non-diapause conditions (Figure 4F), whereas in diapause conditions, AcTrx-like expression was highest in ovary, fat body and midgut (Figure 4H).
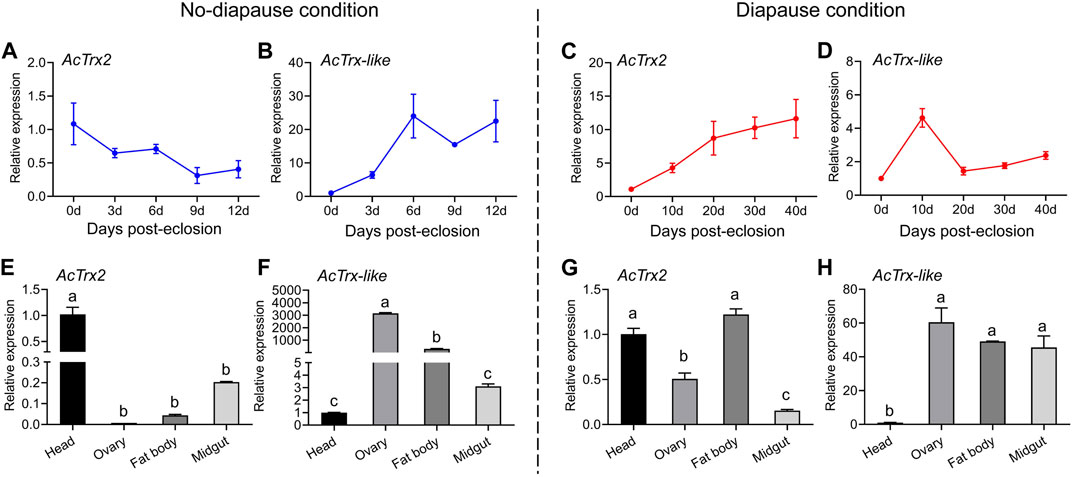
Figure 4. Expression patterns of AcTrx2 and AcTrx-like at different developmental stages and in different tissues. (A–D) Temporal expression profiles of AcTrx2 and AcTrx-like under non-diapause and diapause conditions. (E–H) Spatial transcript expression analysis of AcTrx2 and AcTrx-like under non-diapause and diapause conditions. Data represent mean ± stand error of mean (SEM). Different letters indicated statistically significant differences between different tissues using a one-way analysis of variance (ANOVA) with Tukey’s multiple comparisons test, p < 0.05.
3.4 Expression profiles of AcTrx2 and AcTrx-like at different temperatures
To determine the effect of low temperature on AcTrx2 and AcTrx-like expression, we examined genes expression at 5°C, 15°C, and 5/15°C. RT-qPCR results showed that AcTrx2 expression was significantly induced after A. chinensis exposure to three low temperatures for 24, 48, and 72 h, and AcTrx2 was most sensitive to the 5/15°C, which is the diapause temperature (Figure 5A). Under stress of 5/15°C, AcTrx-like was all upregulated at 24, 48, and 72 h, while at 15°C, AcTrx-like expression was induced only at 72 h after treatment (Figure 5B). In the 5°C treatment, the expression of AcTrx-like was significantly inhibited at 24 and 72 h, but there was no significant difference at 48 h (Figure 5B).
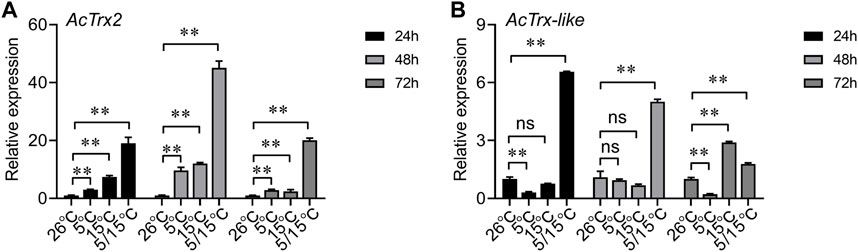
Figure 5. Expression patterns of (A) AcTrx2 and (B) AcTrx-like in adults after exposure to different temperatures. Total RNA was harvested from adults of A. chinensis samples following temperature challenges, including 5°C, 15°C, and 5/15°C. Data represent mean ± stand error of mean (SEM). Asterisks indicate significant differences between different temperatures using Student’s t-test (*, 0.01 < p < 0.05; **, p < 0.01), ns: no significance.
3.5 Expression profiles of other antioxidant genes after knockdown of AcTrx2 and AcTrx-like
RT-qPCR was used to detect changes in the expression of other antioxidant genes after AcTrx2 and AcTrx-like silencing to reveal the relationship between these two genes and other antioxidant genes. When the expression of AcTrx2 decreased by 67.9%, the expression of AcGrxCR and AcGrx5 increased significantly, while the expression of AcTrx-like decreased, and the expression of AcPDI did not change (Figure 6A). Compared to the GFP groups, AcPDI and AcGrxCR were upregulated after AcTrx-like suppressed by 75.9%, but AcTrx2 expression was downregulated, and AcGrx5 had no difference in expression (Figure 6B).
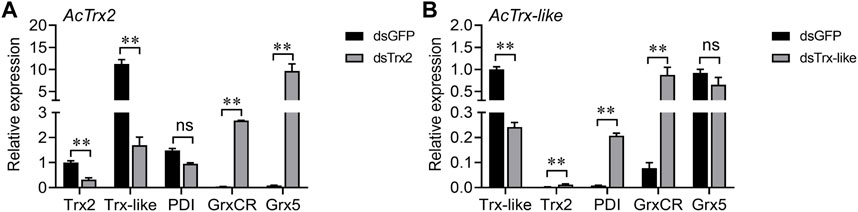
Figure 6. Expression analysis of other antioxidant genes after the knockdown of (A) AcTrx2 and (B) AcTrx-like. The samples were collected at 48 h after dsRNA injection of GFP, Trx2 and Trx-like and then subjected to total RNA extraction and qRT-PCR analysis. Grx5, glutaredoxin 5; GrxCR, glutaredoxin domain-containing cysteine-rich protein; PDI, protein disulfide isomerase. Data represent mean ± stand error of mean (SEM). Asterisks indicate significant differences between different temperatures using Student’s t-test (*, 0.01 < p < 0.05; **, p < 0.01), ns: no significance.
3.6 Effects of AcTrx2 and AcTrx-like knockdown on antioxidant enzyme activities and metabolite contents
To elucidate the function of AcTrx2 and AcTrx-like in antioxidant defense in A. chinensis, superoxide dismutase (SOD) capacity, catalase (CAT) capacity, hydrogen peroxide (H2O2) content, and ascorbate content of A. chinensis were examined. As shown in Figure 7, compared with the control group, when AcTrx2 and AcTrx-like were silenced, the H2O2 and ascorbate contents were significantly increased (Figures 7A,B), and the enzymatic activity of CAT was also significantly enhanced (Figure 7D). However, SOD capacity decreased after AcTrx2 was suppressed, and improved after AcTrx-like knockdown (Figure 7C). All the above results indicated that these two Trx genes may be involved in antioxidant defense in A. chinensis.
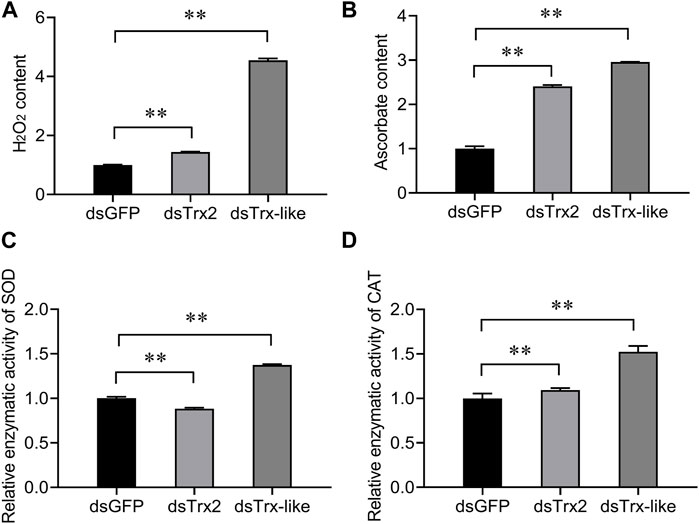
Figure 7. Effects of AcTrx2 and AcTrx-like knockdown on metabolite contents of (A) hydrogen peroxide and (B) ascorbate, and enzymatic activity of (C) SOD and (D) CAT. Whole adults were collected at 48 h after GFP, AcTrx2, and AcTrx-like dsRNA injection and then analyzed using the corresponding kits according to the manufacturer’s protocol. Data represent mean ± stand error of mean (SEM). Asterisks indicate significant differences between different temperatures using Student’s t-test (*, 0.01 < p < 0.05; **, p < 0.01).
4 Discussion
Diapause is a characteristic of insects to avoid harsh environment, and it is an important mean to extend the shelf life of natural enemy products to realize commercialization (Denlinger, 2008). Large number of previous studies mainly focused on the mechanism of diapause regulation, such as the role of juvenile hormone (JH), insulin, etc., in diapause (Tian et al., 2021; Chen et al., 2024). However, there are few studies on insect resistance to low temperature, which is usually used to induce insect winter diapause and causes an increase in reactive oxygen species (ROS) leading to oxidative damage (El-Saadi et al., 2023). In this study, we found that the content of hydrogen peroxide and ascorbate, and the enzymatic activity of CAT increased in diapause condition, indicating that A. chinensis was exposed to ROS and used antioxidant enzymes and antioxidant substances to resist oxidative damage. In addition to these, there may be other antioxidant genes involved in eliminating ROS. As far as we know, Trxs can maintain cellular redox homeostasis and participate in resistance to oxidative damage caused by ROS (Holmgren, 1985; Arnér and Holmgren, 2000; Myers et al., 2008). Hence, we hypothesized that Trxs regulates oxidation levels during diapause induction of A. chinensis.
In previous studies, AcTrx2 and AcTrx-like were identified and elucidated their role in the reproductive diapause of A. chinensis. AcTrx2 had high amino acid identity (57%–88%) with other insect counterparts, and contained highly conserved active sites motif-CGPC. The highly conserved redox-active dithiol group in the form of CXXC is essential for catalytic activity of Trxs (Kallis and Holmgren, 1980; Powis and Montfort, 2001). AcTrx-like sequence contained ALPC-active site sequence. These results demonstrated that AcTrx2 and AcTrx-like possessed cysteine residues of redox active sites to maintain the intracellular redox balance. AcTrx2 gene was expressed at higher levels in the head, in accordance with other insects, including H. armigera (Zhang et al., 2015), A. cerana (Yao et al., 2013), while AcTrx-like was highest expressed in the ovaries. Temporal expression profiles showed that the expression of AcTrx2 was lower in non-diapause conditions, but significantly increased in diapause conditions, while AcTrx-like was highly expressed in non-diapause conditions at the spawning stage, and the expression was the highest at 10 days under diapause conditions. Moreover, the expressions of AcTrx2 and AcTrx-like in fat body, which is the central metabolic organ that plays an important role in detoxifification/degradation of xenobiotics and resisting oxidative stress (Enayati et al., 2005; Arrese and Soulages, 2010), were significantly improved under diapause conditions. Therefore, we speculated that AcTrx2 and AcTrx-like played an important role in diapause.
Previous studies have shown that many environmental factors, including abnormal temperatures, can cause oxidative stress (Lushchak, 2011). Low temperature has been shown to induce Trxs expression in insects, such as H. armigera (Zhang et al., 2015), G. molesta (Shen et al., 2018), and A. cerana (Yao et al., 2013; 2014). In the present study, AcTrx2 expression was significantly induced by low temperature (5°C, 15°C, 5/15°C), especially diapause temperature of 5/15°C. Similarly, AcTrx-like transcription levels were also significantly increased in 5/15°C, although its expression was suppressed in 5°C. These results further demonstrated that both AcTrx2 and AcTrx-like were involved in resistance to oxidative stress induced by low-temperature during diapause.
High levels of ROS lead to DNA damage, lipid peroxidation, formation of protein disulfide bond, and protein carbonylation (Kalinina et al., 2014). Antioxidant enzymes such as CAT, SOD and antioxidant substances (ascorbate, total GSH) are used by cells to remove excessive ROS and resist oxidative damage (Meister, 1994; Corona and Robinson, 2006). In Drosophila, the loss of Trx2 promotes the expression of other antioxidant genes and exacerbates oxidative stress-dependent phenotypes (Tsuda et al., 2021). In A. cerana, the enzymatic activities of CAT and POD, and the metabolite content of H2O2, carbonyls, and ascorbate were increased after AccTrx1 was knocked down, and the expression levels of AccCAT, AccGSTS4, AccMsrA, and AccTrx2 were elevated (Yao et al., 2014). In this study, after AcTrx2 and AcTrx-like knockdown, H2O2 content, ascorbate content and CAT capacity were significantly increased, and the enzymatic activity of SOD was also enhanced after AcTrx-like silencing, revealing that A. chinensis was exposed to oxidative stress, and CAT, SOD, and ascorbate may be involved in scavenging ROS. In addition, AcGrxCR and AcGrx5 expression levels were enhanced after AcTrx2 knockdown, while AcPDI and AcGrxCR expression levels were significantly induced when AcTrx-like was silenced, revealing that all above induced genes were involved in compensating for the knockdown of AcTrx2 and AcTrx-like to resist oxidative damage.
In conclusion, the diapause induced process of A. chinensis increased reactive oxygen species content and altered antioxidant capacity. Moreover, AcTrx2 and AcTrx-like from A. chinensis were successfully identified and possessed conserved functional domains of the Trx superfamilies. The expression levels of AcTrx2 and AcTrx-like were significantly improved under diapause conditions and induced by low temperature, especially 5/15°C, implying that AcTrx2 and AcTrx-like may play critical roles in scavenging excess ROS and in antioxidant defense. After RNAi mediated knockdown of AcTrx2 and AcTrx-like, the expression levels of some other antioxidant genes (such as GrxCR, Grx5, etc.) were upregulated, the enzymatic activities of SOD and CAT increased, and metabolites (hydrogen peroxide and ascorbate) also increased. Our study verified the antioxidant roles of AcTrx2 and AcTrx-like in A. chinensis diapause, providing new insights into the mechanism of diapause in insects.
Data availability statement
The datasets presented in this study can be found in online repositories. The names of the repository/repositories and accession number(s) can be found in the article/Supplementary Material.
Ethics statement
The manuscript presents research on animals that do not require ethical approval for their study.
Author contributions
ZS: Conceptualization, Data curation, Formal Analysis, Supervision, Validation, Visualization, Writing–original draft, Writing–review and editing. QL: Data curation, Investigation, Project administration, Writing–original draft. JM: Resources, Supervision, Writing–review and editing. Y-YL: Writing–review and editing, Supervision. MW: Writing–review and editing, Resources. LZ: Funding acquisition, Resources, Supervision, Writing–review and editing.
Funding
The author(s) declare that financial support was received for the research, authorship, and/or publication of this article. This research was supported by the National Key R&D Program of China (2023YFD1400600), Major Projects of China National Tobacco Corporation [110202201022 (LS-06)], and International Science & Technology Innovation Program of Chinese Academy of Agricultural Sciences (CAAS-ZDRW202203, CAAS-ZDRW202108).
Acknowledgments
The authors thank Fang Liu of Key Laboratory of Natural Enemy Insects in Institute of Plant Protection, Chinese Academy of Agricultural Sciences, for providing the A. chinensis.
Conflict of interest
The authors declare that the research was conducted in the absence of any commercial or financial relationships that could be construed as a potential conflict of interest.
Publisher’s note
All claims expressed in this article are solely those of the authors and do not necessarily represent those of their affiliated organizations, or those of the publisher, the editors and the reviewers. Any product that may be evaluated in this article, or claim that may be made by its manufacturer, is not guaranteed or endorsed by the publisher.
Supplementary material
The Supplementary Material for this article can be found online at: https://www.frontiersin.org/articles/10.3389/fphys.2024.1440531/full#supplementary-material
References
Arnér E. S., Holmgren A. (2000). Physiological functions of thioredoxin and thioredoxin reductase. Eur. J. Biochem. 267, 6102–6109. doi:10.1046/j.1432-1327.2000.01701.x
Arrese E. L., Soulages J. L. (2010). Insect fat body: energy, metabolism, and regulation. Annu. Rev. Entomol. 55, 207–225. doi:10.1146/annurev-ento-112408-085356
Bauer H., Kanzok S. M., Schirmer R. H. (2002). Thioredoxin-2 but not thioredoxin-1 is a substrate of thioredoxin peroxidase-1 from Drosophila melanogaster: isolation and characterization of a second thioredoxin in D. Melanogaster and evidence for distinct biological functions of Trx-1 and Trx-2. J. Biol. Chem. 277, 17457–17463. doi:10.1074/jbc.M200636200
Benitez-Alfonso Y., Cilia M., San Roman A., Thomas C., Maule A., Hearn S., et al. (2009). Control of Arabidopsis meristem development by thioredoxin-dependent regulation of intercellular transport. Proc. Natl. Acad. Sci. U. S. A. 106, 3615–3620. doi:10.1073/pnas.0808717106
Chen J. J., Liu X. X., Guo P. H., Teets N. M., Zhou J. C., Chen W. B., et al. (2024). Regulation of forkhead box O transcription factor by insulin signaling pathway controls the reproductive diapause of the lady beetle, Coccinella Septempunctata. Int. J. Biol. Macromol. 258, 128104. doi:10.1016/j.ijbiomac.2023.128104
Chen Y., Cai J., Murphy T. J., Jones D. P. (2002). Overexpressed human mitochondrial thioredoxin confers resistance to oxidant-induced apoptosis in human osteosarcoma cells. J. Biol. Chem. 277, 33242–33248. doi:10.1074/jbc.M202026200
Corona M., Robinson G. E. (2006). Genes of the antioxidant system of the honey bee: annotation and phylogeny. Insect Mol. Biol. 15, 687–701. doi:10.1111/j.1365-2583.2006.00695.x
Damdimopoulos A. E., Miranda-Vizuete A., Pelto-Huikko M., Gustafsson J. A., Spyrou G. (2002). Human mitochondrial thioredoxin. Involvement in mitochondrial membrane potential and cell death. J. Biol. Chem. 277, 33249–33257. doi:10.1074/jbc.M203036200
Denlinger D. L. (2008). Why study diapause? Entomol. Res. 38, 1–9. doi:10.1111/j.1748-5967.2008.00139.x
El-Saadi M. I., MacMillan H. A., Ferguson L. V. (2023). Cold-induced immune activation in chill-susceptible insects. Curr. Opin. Insect Sci. 58, 101054. doi:10.1016/j.cois.2023.101054
Enayati A. A., Ranson H., Hemingway J. (2005). Insect glutathione transferases and insecticide resistance. Insect Mol. Biol. 14, 3–8. doi:10.1111/j.1365-2583.2004.00529.x
Guo S., Tian Z., Wu Q. W., King-Jones K., Liu W., Zhu F., et al. (2021a). Steroid hormone ecdysone deficiency stimulates preparation for photoperiodic reproductive diapause. PLoS Genet. 17, e1009352. doi:10.1371/journal.pgen.1009352
Guo S., Wu Q. W., Tian Z., Zhu L., King-Jones K., Zhu F., et al. (2021b). Krüppel homolog 1 regulates photoperiodic reproductive plasticity in the cabbage beetle Colaphellus bowringi. Insect Biochem. Mol. Biol. 134, 103582. doi:10.1016/j.ibmb.2021.103582
Holmgren A. (1985). Thioredoxin. Annu. Rev. Biochem. 54, 237–271. doi:10.1146/annurev.bi.54.070185.001321
Kalinina E. V., Chernov N. N., Novichkova M. D. (2014). Role of glutathione, glutathione transferase, and glutaredoxin in regulation of redox-dependent processes. Biochem. (Mosc). 79, 1562–1583. doi:10.1134/S0006297914130082
Kalinina E. V., Chernov N. N., Saprin A. N. (2008). Involvement of thio-peroxi-and glutaredoxins in cellular redox-dependent processes. Biochem. (Mosc). 73, 1493–1510. doi:10.1134/s0006297908130099
Kallis G. B., Holmgren A. (1980). Differential reactivity of the functional sulfhydryl groups of cysteine-32 and cysteine-35 present in the reduced form of thioredoxin from Escherichia coli. J. Biol. Chem. 255, 10261–10265. doi:10.1016/S0021-9258(19)70458-X
Kang T., Wan H., Zhang Y., Shakeel M., Lu Y., You H., et al. (2015). Comparative study of two thioredoxins from common cutworm (Spodoptera litura): cloning, expression, and functional characterization. Comp. Biochem. Physiol. B Biochem. Mol. Biol. 182, 47–54. doi:10.1016/j.cbpb.2014.12.004
Kanzok S. M., Fechner A., Bauer H., Ulschmid J. K., Müller H. M., Botella-Munoz J., et al. (2001). Substitution of the thioredoxin system for glutathione reductase in Drosophila Melanogaster. Science. 291, 643–646. doi:10.1126/science.291.5504.643
Kobayashi-Miura M., Shioji K., Hoshino Y., Masutani H., Nakamura H., Yodoi J. (2007). Oxygen sensing and redox signaling: the role of thioredoxin in embryonic development and cardiac diseases. Am. J. Physiol. Heart Circ. Physiol. 292, H2040–H2050. doi:10.1152/ajpheart.01316.2006
Kondo N., Nakamura H., Masutani H., Yodoi J. (2006). Redox regulation of human thioredoxin network. Antioxid. Redox. Signal 8, 1881–1890. doi:10.1089/ars.2006.8.1881
Laurent T. C., Moore E. C., Reichard P. (1964). Enzymatic synthesis of deoxyribonucleotides. IV. Isolation and characterization of thioredoxin, the hydrogen donor from Escherichia coli B. J. Biol. Chem. 239, 3436–3444. doi:10.1016/S0021-9258(18)97742-2
Lee S., Kim S. M., Lee R. T. (2013). Thioredoxin and thioredoxin target proteins: from molecular mechanisms to functional significance. Antioxid. Redox. Signal 18, 1165–1207. doi:10.1089/ars.2011.4322
Livak K. J., Schmittgen T. D. (2001). Analysis of relative gene expression data using real-time quantitative PCR and the 2(-Delta Delta C(T)) Method. Methods 25, 402–408. doi:10.1006/meth.2001.1262
Lu J., Holmgren A. (2014). The thioredoxin antioxidant system. Free Radic. Biol. Med. 66, 75–87. doi:10.1016/j.freeradbiomed.2013.07.036
Lu W., Kang M., Liu X., Zhao X., Guo X., Xu B. (2012). Identification and antioxidant characterisation of thioredoxin-like1 from Apis cerana cerana. Apidologie 43, 737–752. doi:10.1007/s13592-012-0148-7
Lushchak V. I. (2011). Environmentally induced oxidative stress in aquatic animals. Aquat. Toxicol. 101, 13–30. doi:10.1016/j.aquatox.2010.10.006
Meister A. (1994). Glutathione-ascorbic acid antioxidant system in animals. J. Biol. Chem. 269 (13), 9397–9400. doi:10.1016/S0021-9258(17)36891-6
Meng J. Y., Yang C. L., Wang H. C., Cao Y., Zhang C. Y. (2022). Molecular characterization of six heat shock protein 70 genes from Arma chinensis and their expression patterns in response to temperature stress. Cell. Stress Chaperones 27, 659–671. doi:10.1007/s12192-022-01303-y
Myers J. M., Antholine W. E., Myers C. R. (2008). Hexavalent chromium causes the oxidation of thioredoxin in human bronchial epithelial cells. Toxicology 246, 222–233. doi:10.1016/j.tox.2008.01.017
Poli G., Leonarduzzi G., Biasi F., Chiarpotto E. (2004). Oxidative stress and cell signalling. Curr. Med. Chem. 11, 1163–1182. doi:10.2174/0929867043365323
Powis G., Montfort W. R. (2001). Properties and biological activities of thioredoxins. Annu. Rev. Biophys. Biomol. Struct. 30, 421–455. doi:10.1146/annurev.biophys.30.1.421
Revathy K. S., Umasuthan N., Lee Y., Whang I., Kim H. C., Lee J. (2012). Cytosolic thioredoxin from Ruditapes philippinarum: molecular cloning, characterization, expression and DNA protection activity of the recombinant protein. Dev. Comp. Immunol. 36, 85–92. doi:10.1016/j.dci.2011.06.006
Serata M., Iino T., Yasuda E., Sako T. (2012). Roles of thioredoxin and thioredoxin reductase in the resistance to oxidative stress in Lactobacillus casei. Microbiol. Read. 158, 953–962. doi:10.1099/mic.0.053942-0
Shen Z. J., Liu Y. J., Gao X. H., Liu X. M., Zhang S. D., Li Z., et al. (2018). Molecular identification of two thioredoxin genes from Grapholita molesta and their function in resistance to emamectin benzoate. Front. Physiol. 9, 1421. doi:10.3389/fphys.2018.01421
Svensson M. J., Chen J. D., Pirrotta V., Larsson J. (2003). The ThioredoxinT and deadhead gene pair encode testis- and ovary-specific thioredoxins in Drosophila melanogaster. Chromosoma 112, 133–143. doi:10.1007/s00412-003-0253-5
Svensson M. J., Larsson J. (2007). Thioredoxin-2 affects lifespan and oxidative stress in Drosophila. Hereditas 144, 25–32. doi:10.1111/j.2007.0018-0661.01990.x
Tian Z., Guo S., Li J. X., Zhu F., Liu W., Wang X. P. (2021). Juvenile hormone biosynthetic genes are critical for regulating reproductive diapause in the cabbage beetle. Insect Biochem. Mol. Biol. 139, 103654. doi:10.1016/j.ibmb.2021.103654
Tsuda M., Ootaka R., Ohkura C., Kishita Y., Seong K. H., Matsuo T., et al. (2021). Loss of Trx-2 enhances oxidative stress-dependent phenotypes in Drosophila. FEBS Lett. 584, 3398–3401. doi:10.1016/j.febslet.2010.06.034
Uzelac I., Avramov M., Knežić T., Tatić V., Gošić-Dondo S., Popović Ž. D. (2024). Prolonged heat stress during winter diapause alters the expression of stress-response genes in Ostrinia nubilalis (Hbn.). Int. J. Mol. Sci. 25, 3100. doi:10.3390/ijms25063100
Yao P., Chen X., Yan Y., Liu F., Zhang Y., Guo X., et al. (2014). Glutaredoxin 1, glutaredoxin 2, thioredoxin 1, and thioredoxin peroxidase 3 play important roles in antioxidant defense in Apis cerana cerana. Free Radic. Biol. Med. 68, 335–346. doi:10.1016/j.freeradbiomed.2013.12.020
Yao P., Hao L., Wang F., Chen X., Yan Y., Guo X., et al. (2013). Molecular cloning, expression and antioxidant characterisation of a typical thioredoxin gene (AccTrx2) in Apis cerana cerana. Gene 527, 33–41. doi:10.1016/j.gene.2013.05.062
Yoshioka J., Schreiter E. R., Lee R. T. (2006). Role of thioredoxin in cell growth through interactions with signaling molecules. Antioxid. Redox. Signal 8, 2143–2151. doi:10.1089/ars.2006.8.2143
Keywords: Arma chinensis, thioredoxin, diapause, oxidative stress, antioxidant defense
Citation: Shen Z, Luo Q, Mao J, Li Y, Wang M and Zhang L (2024) Molecular identification of two thioredoxin genes and their function in antioxidant defense in Arma chinensis diapause. Front. Physiol. 15:1440531. doi: 10.3389/fphys.2024.1440531
Received: 29 May 2024; Accepted: 24 June 2024;
Published: 24 July 2024.
Edited by:
Federico Sesti, Rutgers, United StatesReviewed by:
Diego Cotella, Università degli Studi del Piemonte Orientale, ItalyRahul P. Patel, University of North Carolina at Chapel Hill, United States
Copyright © 2024 Shen, Luo, Mao, Li, Wang and Zhang. This is an open-access article distributed under the terms of the Creative Commons Attribution License (CC BY). The use, distribution or reproduction in other forums is permitted, provided the original author(s) and the copyright owner(s) are credited and that the original publication in this journal is cited, in accordance with accepted academic practice. No use, distribution or reproduction is permitted which does not comply with these terms.
*Correspondence: Lisheng Zhang, zhangleesheng@163.com