- 1College of Martial Arts and Traditional Ethnic Sports, Jilin Sport University, Changchun, China
- 2Department of Physical Education, Kyungpook National University, Daegu, Republic of Korea
- 3School of Physical Education and Sports Science, Soochow University, Suzhou, China
- 4School of Educational Studies, Universiti Sains Malaysia, Penang, Malaysia
Introduction: This study aimed to evaluate the effects of varied resistance training modalities on physical fitness components, body composition, maximal strength assessed by one-repetition maximum (1RM), isokinetic muscle functions of the shoulder and knee joints, and biomechanical properties of core muscles.
Methods: Forty participants were randomly assigned to four groups: control group (CG, n = 10), compound set training group (CSG, n = 10), pyramid set training group (PSG, n = 10), and superset training group (SSG, n = 10). Excluding the CG, the other three groups underwent an 8-week resistance training program, three sessions per week, at 60%–80% of 1RM intensity for 60–90 min per session. Assessments included body composition, physical fitness components, 1RM, isokinetic muscle functions, and biomechanical properties (muscle frequency, stiffness, etc.) of the rectus abdominis and external oblique muscles.
Results: The PSG demonstrated the most significant improvement in relative peak torque during isokinetic testing of the shoulder and knee joints. Compared to the CG, all exercise groups exhibited positive effects on back strength, sprint performance, 1RM, and core muscle biomechanics. Notably, the PSG showed superior enhancement in external oblique stiffness. However, no significant differences were observed among the exercise groups for rectus abdominis biomechanical properties.
Discussion: Structured resistance training effectively improved maximal strength, functional performance, and core muscle biomechanics. The pyramidal training modality conferred specific benefits for isokinetic muscle functions and external oblique stiffness, suggesting its efficacy in enhancing force production capabilities and core stability.
1 Introduction
Resistance training is an effective exercise modality that can significantly enhance muscular strength, facilitate muscular hypertrophy, and improve overall physical fitness (Schoenfeld, 2010). This training approach elicits physiological stimuli, such as mechanical tension, muscle damage, and metabolic stress, through the manipulation of external loads, thereby initiating adaptive responses within the musculature (Florini, 1987). The primary variables in resistance training include training volume, intensity, rest intervals, and exercise order (Haff and Triplett, 2016). By strategically adjusting and combining these variables, various innovative training modalities can be derived. Pyramid training involves alternating between lighter and heavier intensities, such as a repetition scheme of 3-5-7-5-3, gradually increasing and subsequently decreasing the training load (Fleck and Kraemer, 2014; Cuevas-Aburto et al., 2020). Superset training entails performing two or more exercises targeting the same muscle group consecutively, such as pairing seated rows with pull-ups, augmenting metabolic stress and muscular fatigue (Robbins et al., 2010; Iversen et al., 2021). Compound training adheres to the traditional approach of training muscle groups in succession, selecting a primary exercise as the core and supplementing it with related assistance exercises, such as utilizing squats as the primary lower body exercise, complemented by calf raises and hip extensions (Haff and Triplett, 2016; Kraemer et al., 2002). Through various combinations of variables, these diverse training modalities can produce different outcomes, such as altering training stimuli and metabolic stress, thereby providing athletes with a range of training options. Judicious utilization of these modalities can help maintain novelty and sustained efficacy (Robbins et al., 2010; Pareja-Blanco et al., 2017).
Extant research indicates that different training modalities exert varying effects on strength development, physical fitness, and muscular characteristics. Certain studies have found that compound training can enhance one-repetition maximum (1RM) and overall muscular strength (Baz-Valle et al., 2019; Paoli et al., 2017), while pyramid training is more conducive to improving isometric muscular power (Mangine et al., 2015), and superset training can improve flexibility and reduce body fat percentage (Schoenfeld et al., 2014). However, the impacts of these training modalities on improving fundamental physical fitness, 1RM levels, and isometric muscular function remain contentious.
Non-invasive assessments of alterations in superficial muscle stiffness and tension can elucidate the pathological consequences of exercise and the efficacy of interventions (Dahmane et al., 2001; Marusiak et al., 2012). Previous investigations have substantiated the reliability of employing myotonometry (Myoton) to measure skeletal muscle stiffness and tension in diverse populations, such as healthy adults, individuals with Parkinson’s disease, and stroke survivors (Mullix et al., 2012; Marusiak et al., 2012; Chuang et al., 2013). Nevertheless, extant research has not comprehensively explored the effects of these training modalities on muscular mechanical properties, including tension, stiffness, and shape recovery ability.
Consequently, the present study endeavors to systematically compare prevalent resistance training modalities (pyramid sets, supersets, and compound sets) and comprehensively evaluate their respective impacts on physical fitness, isometric joint strength, and biomechanical properties of core muscle groups. The study will assess participants’ 1RM levels, isometric strength performance of limb joints, and employ non-invasive myotonometry techniques to quantify alterations in mechanical properties, such as muscle tension, stiffness, and shape recovery capacity, of core muscle groups. This research will not only augment existing knowledge but also provide empirical evidence to facilitate the development of individualized, goal-oriented training programs, optimizing training efficacy for diverse populations and objectives, thereby advancing theoretical and practical developments within related domains.
2 Materials and methods
2.1 Participants
This study employed a convenience sampling approach to recruit 40 male participants aged 18–30 years, each with a minimum of 6 months’ experience in resistance training. The participants were randomly assigned to four groups: Control Group (CG), Compound Set Group (CSG), Pyramid Set Group (PSG), and Superset Group (SSG), with 10 individuals in each group. Quality assurance measures were implemented to ensure participants met the following criteria: a) aged between 18 and 30 years, b) no positive response on the Physical Activity Readiness Questionnaire (PAR-Q), c) abstinence from substances that could influence the results (e.g., caffeine, creatine, steroids), and d) absence of musculoskeletal issues that might impede resistance exercise execution. This study received approval from the Institutional Review Board of Jeju National University (JJNU-IRB-2023-004). G*Power software (G*Power 3.1.9.7, University of Kiel, Kiel, Germany) was used to calculate the appropriate sample size. Additionally, the reference reported main effect size of 1.38 (Karasu et al., 2018), including a significance level α of 0.05 and a power 1-β of 0.95, calculated a sample size of 10 for each group. Table 1 presents the physical characteristics of the participants.
2.2 Exercise protocol
The 8-week resistance training intervention comprised three sessions per week, incorporating compound sets, pyramid sets, and superset routines. The initial 3 weeks served as an intensity adaptation phase, with training intensities individualized based on one 1RM test results. Each training session followed a structured protocol:
• Dynamic stretching: A Rated Perceived Exertion (RPE) scale of 9–11 was utilized to prepare the body for subsequent training.
• Running at 50%–70% of maximum heart rate: Lasted for 10 min to elevate heart rate and prepare the body for resistance training.
• Resistance training: including:
1. Compound sets: Two exercises targeting the same muscle group performed consecutively, with a 1-min rest interval between sets.
2. Pyramid sets: Traditional pyramid-style training, with progressive increments in weight and decrements in repetitions across sets.
3. Superset: Two or more exercises targeting the same muscle group performed consecutively, without rest intervals.
Refer to the revised Table 2 for specific exercise details and loading parameters.
• Static stretching: Static stretching was performed for 5 min with an RPE rating of 6–7 as a cooldown exercise to alleviate muscle tension and minimize post-training discomfort.
2.2.1 Resistance training variables
The reporting of resistance training variables adhered to the recommendations by Coratella, G. (2022). The specific resistance training variables for each group were as follows:
• Control Group (CG): Performed traditional straight sets with three sets of eight to twelve repetitions at 60%–70% of 1RM for all exercises.
• Compound Set Group (CSG): Weeks 1–3: Three sets of eight to twelve repetitions at 60%–70% 1RM. Weeks 4–6: Three sets of six to eight repetitions at 70% 1RM. Weeks 7–8: Three sets of four to six repetitions at 80% 1RM. One-minute rest between compound sets.
• Pyramid Set Group (PSG): Day 1: One set at 60% 1RM, one set at 70% 1RM, one set at 80% 1RM. Day 2: One set at 80% 1RM, one set at 70% 1RM, one set at 60% 1RM. Ninety-second rest between pyramid sets.
• Superset Group (SSG): Weeks 1–3: Three sets of eight to twelve repetitions at 60-70% 1RM. Weeks 4–6: Three sets of six to eight repetitions at 70% 1RM. Weeks 7–8: Three sets of four to six repetitions at 80% 1RM. No rest between superset exercises.
2.3 Measurement procedures
2.3.1 Body composition
The InBody 770 body composition analyzer (InBody, Seoul, South Korea) was utilized to measure height, weight, skeletal muscle mass, fat mass, body mass index (BMI), and body fat percentage. Participants refrained from food and fluid intake for 8 h before the measurement, wore light, form-fitting clothing, and removed any metallic objects. Body fat percentage was estimated using bioelectrical impedance analysis. BMI was calculated as weight (kg) divided by height (m) squared, while body fat percentage was estimated based on bioelectrical impedance analysis.
2.3.2 Basic fitness tests
2.3.2.1 Flexibility
Measure flexibility using the Takei TKK5111 sit-and-reach test. The participant sits with feet no more than 5 cm apart, then reaches forward with both hands toward the end of the device. Hold the position for 3 s and perform 2 trials, recording the maximum distance in centimeters (cm).
2.3.2.2 Muscle strength
Assess dominant hand grip strength using the Takei TKK5101 digital grip strength meter and back strength using the Takei TKK5402 digital strength meter. Perform 2 trials and record the best score in kilograms (kg).
2.3.2.3 40 m sprint
Measure time using the Casio OST-30W stopwatch. After one practice run, provide adequate rest. From the starting line, the participant runs at maximum speed to the 40 m mark, and the time is recorded when the chest crosses the finish line, to the nearest 0.01 s.
2.3.2.4 Illinois agility test
Use the Casio OST-30W stopwatch to measure time on a standard 10 m × 5 m course. The participant starts on the signal, runs to the finish, and the best time of 2 trials is recorded in 0.01 s.
2.3.2.5 Standing long jump
Stand with feet as close to the starting line as possible, then jump as far as possible. Record the distance from the start line to the nearest heel after 1 trial, with approximately 1 min of rest between the 2 measured jumps. Record the maximum distance in meters (m).
2.3.3 One repetition maximum (1RM) of bench press, deadlift, and squat
The (1RM for the bench press, deadlift, and squat exercises was measured to assess the participants’ maximal strength. This assessment was conducted following the protocol recommended by the National Strength and Conditioning Association (NSCA) (Haff and Triplett, 2015). To ensure participant safety, at least two trained assistants were present to serve as spotters during the testing. Prior to the 1RM measurements, participants performed a standardized warm-up routine to prepare their musculoskeletal system and reduce the risk of injury. After the warm-up, participants attempted to lift the maximum weight they could for a single repetition on each of the three lifts. If a participant failed to complete a lift, the weight was immediately reduced by 5%, and the participant was given adequate rest before retrying the lift. This process continued until the participant’s 1RM was successfully determined for each exercise.
2.3.4 Isokinetic shoulder and knee joint functions
To obtain accurate and reliable isokinetic peak torque data for the shoulder and knee joints, this study employed the Humac Norm isokinetic dynamometer (Humac Norm 776, CSMI, Boston, United States) and its Trunk Extension and Flexion (TEF) module. The isokinetic function of the shoulder and knee joints was assessed at an angular velocity of 60 degrees per second.
Prior to testing, participants performed three familiarization trials to acquaint themselves with the procedure and mitigate risks. Gravity correction was implemented during testing to eliminate the effect of gravity. The range of motion for shoulder testing was 30° internal rotation to 90° external rotation, measuring the isokinetic peak torque of the internal and external rotator muscle groups at 60°/s. The range of motion for knee testing was 0°–100° flexion-extension, measuring the isokinetic peak torque of the flexor and extensor muscle groups at 60°/s.
Each joint was tested six times bilaterally, with the average of the middle four results taken to enhance data reliability. To mitigate the influence of muscle fatigue, a 2-min interval was implemented between familiarization trials and formal testing, a 1-min interval between single sides, and a 2-min interval between both sides, with a 60-s recovery period after each test. The testing procedure was optimized to obtain high-quality data while minimizing the risk of harm to the participants.
2.3.5 Measurement of muscle biomechanical properties
To evaluate the biomechanical performance of the trunk muscles, this study utilized the MyotonPRO (Myoton AS, Tallinn, Estonia) non-invasive contact soft tissue measurement device. This device is equipped with a three-axis digital accelerometer that can simultaneously obtain biomechanical parameters such as muscle tension and viscoelasticity by measuring the frequency decay and graphing the acceleration of natural tissue oscillations.
The measurements were conducted under controlled environmental conditions (limited resonant effects from noise and vibration, temperature 22°C–24°C, relative humidity 45%–60%). To ensure data accuracy, participants were instructed to remain relaxed during the measurement process to avoid producing unnecessary muscle tension. During measurement, the polycarbonate probe (3 mm diameter) was vertically applied to the center of the muscle belly to induce resonant vibrations. Muscle tension was represented by frequency (Hz), reflecting the intrinsic oscillation state without active contraction during baseline electromyography. The biomechanical parameters measured included dynamic stiffness (N/m) reflecting dynamic rigidity, logarithmic decrement reflecting vibration damping, stress relaxation time (ms), and creep reflecting viscoelasticity.
A multi-scan mode was employed, with the rectus abdominis (RA) and external oblique (EO) muscles measured to assess changes in the mechanical properties of the core muscles. The number of repetitions was set to five, with a tap time of 15 ms and a damping period of 0.8 s to ensure internal consistency. To improve the reliability of repeated measurements, all measurements before and after were performed by the same examiner. Prior to measurement, participants rested in a supine position for 20 min. During measurement, participants breathed naturally and maintained a stationary state without external force. Measurements were taken in the supine position, with the coefficient of variation (CV) observed on the MyotonPRO LCD monitor. Each site was measured twice; if CV > 3%, an additional measurement was taken, and the average of two measurements with CV ≤ 3% was used as the final result.
2.4 Statistical analysis
Statistical analyses were performed using IBM SPSS Statistics 22.0 (IBM Co., Armonk, NY, United States). Participants were randomly assigned to a control group (CG), compound set group (CSG), pyramid set group (PSG), or superset group (SSG). Pre-intervention and post-intervention data were collected to evaluate the effects of each training modality. All variables, encompassing pre-intervention and post-intervention values, were measured and recorded using SPSS 22.0. Means ± standard deviations (SDs) were calculated for each variable to describe the central tendency and dispersion of the data. The Shapiro-Wilk test (Razali, 2011) as employed to verify the normality of the dependent variables before and after the intervention, followed by Levene’s test to assess the homogeneity of variance.
Partial eta-squared (ηp2) was utilized as the effect size measure to quantify the magnitude of between-group differences. A two-way repeated measure analysis of variance (ANOVA) was conducted to evaluate the interaction between groups and time points for each variable, with post hoc analyses using Tukey’s correction. To compare the effects of different training programs on between-group differences, we employed ANOVA instead of an analysis of covariance (ANCOVA) with baseline as a covariate. This approach was chosen because the primary focus of this study was to assess the impact of different training programs on the changes in these variables, rather than accounting for individual differences. A significance level of p ≤ 0.05 was set to determine the statistical significance of the results.
3 Results
3.1 Body composition
The comparison of body composition among different resistance exercise sets (Table 3) revealed significant interactions between groups and time for weight, skeletal muscle mass, fat mass, and body fat percentage. However, according to post hoc tests, there were no significant differences among the groups in weight (F = 1.089, p = .366, ηp2 = 0.035), skeletal muscle mass (F = 1.192, p = .327, ηp2 = 0.038), fat mass (F = .676, p = .573, ηp2 = 0.038), and body fat percentage (F = .724, p = .544, ηp2 = 0.024).
3.2 Fundamental physical fitness
The analysis of fundamental physical fitness among various resistance exercise set compositions (Table 4) showed significant interactions between groups and time for sit-and-reach, grip strength, abdominal muscle strength, 40-m sprint, Illinois agility test, and standing long jump. However, significant differences were found in abdominal muscle strength (F = 19.284, p = .001, ηp2 = 0.395) and 40-m sprint (F = 9.130, p = .001, ηp2 = 0.245) among the groups in post hoc tests, while sit-and-reach (F = .062, p = .980, ηp2 = 0.002), grip strength (F = 1.209, p = .320, ηp2 = 0.039), Illinois agility test (F = .478, p = .669, ηp2 = 0.016) and standing long jump (F = .177, p = .912, ηp2 = 0.006) showed no significant differences. Post hoc tests indicated significant increases in abdominal muscle strength and 40-m sprint in CSG, PSG, and SSG, with no significant differences among the exercise groups.
3.3 1RM of bench press, deadlift, and squat
The evaluation of the 1RM for bench press, deadlift, and squat among resistance exercise set compositions (Table 5) demonstrated significant interactions between groups and time for these exercises. Post-hoc tests revealed significant differences in bench press (F = 17.024, p = .001, ηp2 = 0.374), deadlift (F = 23.523, p = .001, ηp2 = 0.447), and squat (F = 19.170, p = .001, ηp2 = 0.393) among the groups. Notably, there were significant increases in bench press, deadlift, and squat 1RM in CSG, PSG, and SSG, with no significant differences among the exercise groups.
3.4 Changes in shoulder stability muscle strength
Examination of changes in shoulder stability muscle strength among resistance exercise set compositions (Table 6) revealed significant interactions between groups and time for the relative values of left and right shoulder abduction and adduction muscles. However, there were no significant differences in shoulder abduction/adduction ratios over time or between groups. Post-hoc tests identified significant differences in the relative values of left shoulder abduction (F = 12.171, p = .001, ηp2 = 0.295), left shoulder adduction (F = 9.244, p = .001, ηp2 = 0.242), right shoulder abduction (F = 12.289, p = .001, ηp2 = 0.297), and right shoulder adduction (F = 16.707, p = .001, ηp2 = 0.363) among the groups. Specifically, CSG and PSG exhibited significantly higher relative values of left shoulder abduction compared to CG and SSG, and PSG showed significantly higher relative values of left shoulder adduction and right shoulder abduction compared to CG. Additionally, PSG demonstrated significantly higher relative values of right shoulder abduction compared to the other groups.
3.5 Changes in knee stability muscle strength
Analysis of changes in knee stability and muscle strength among resistance exercise set compositions (Table 7) indicated significant interactions between groups and time for the relative values of left and right knee flexion and extension muscles. However, there were no significant differences in knee flexion/extension ratios between groups or over time. Post-hoc tests revealed significant differences in the relative values of left knee flexion (F = 7.169, p = .001, ηp2 = 0.196), left knee extension (F = 6.471, p = .00, ηp2 = 0.179), right knee flexion (F = 6.576, p = .001, ηp2 = 0.182), and right knee extension (F = 8.191, p = .001, ηp2 = 0.182) among the groups. Notably, CSG and PSG exhibited significantly higher relative values of left knee flexion compared to CG, while no significant differences were observed in left knee extension.
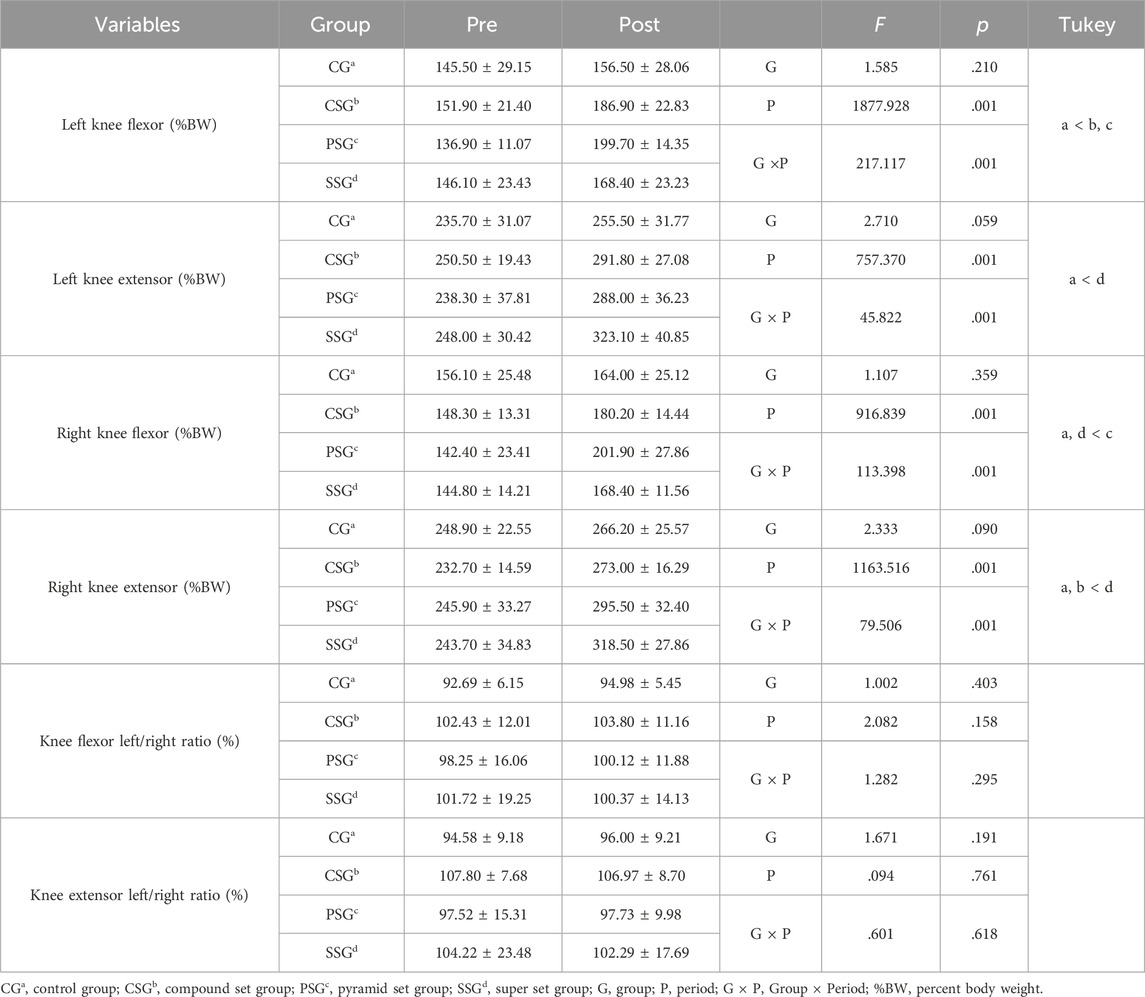
Table 7. Changes in isokinetic knee (60°/sec) muscle strength according to the resistance training structure.
3.6 Core muscle biomechanical properties
The biomechanical parameters of the rectus abdominis (RA) muscle demonstrated statistically significant changes (P < 0.05) across all training groups after 8 weeks of intervention, including frequency, stiffness, decrement, relaxation, and creep. However, no significant differences were observed in the changes of these parameters between the different training groups (P > 0.05). For the external oblique (EO) muscle, PSG exhibited significantly greater improvements in stiffness compared to the other three groups (F = 51.310, P < 0.01, ηp2 = 0.182), while no significant differences were found among the other groups (P > 0.05). Regarding frequency, the three training groups showed significantly higher values than the CG (F = 23.240, P < 0.05, ηp2 = 0.436), but no significant differences were observed among the training groups (P > 0.05). No significant differences were detected among the groups for parameters such as decrement, relaxation, and creep (P > 0.05) (Table 8).
4 Discussion
The array of effective resistance training set combinations has expanded considerably over time, reflecting the diverse needs and preferences of contemporary individuals regarding exercise goals, time constraints, and training environments. Despite this proliferation, controversy persists regarding the optimal exercise set configurations for maximizing desired outcomes. Consequently, this study aimed to elucidate the effects of various resistance training set combinations, including compound sets, pyramid sets, and super sets, on body composition, fundamental physical fitness, and isokinetic muscle function of the shoulders and knees among healthy adults.
After an 8-week resistance training program with varied set combinations, the results demonstrated no statistically significant differences in all body composition indicators (weight, skeletal muscle mass, fat mass, body mass index, body fat percentage) compared to the control group. This finding contradicts previous research utilizing pyramid set configurations, which exhibited a decrease in body fat percentage post-exercise (Sayyah et al., 2021). However, it is essential to note that the absence of significant inter-group differences in this study may be attributable to the lack of dietary control for the subjects and the focus of the exercise protocol on analyzing the effects of non-aerobic exercise set combinations, which are believed to exert minimal impact on body composition (Willis et al., 2012; Benito et al., 2020).
The analysis of changes in fundamental physical fitness after the 8-week resistance training program revealed improvements in abdominal strength and 40-m sprint capability in all training groups compared to the control group, although the differences between each training group were not statistically significant. These findings are consistent with prior studies on the effects of different set combinations over 8–12 weeks, which reported significant increases in strength for compound sets, pyramid sets, and super sets (Prasetyo and Nasrulloh, 2017; Merrigan et al., 2019; Fink et al., 2021). Additionally, the increase in lower limb strength is closely associated with enhanced sprint performance (Delecluse, 1997; Seitz et al., 2014). However, the results of this study did not directly investigate the potential influence of neuromuscular junction factors on strength gains, which have been implicated in previous research (Aagaard et al., 2002; Folland and Williams, 2007). Consequently, future research focusing specifically on neuromuscular junction factors and their relationship with resistance training set combinations is warranted.
Comparative analysis of the differences in 1RM (one-repetition maximum) for bench press, deadlift, and squat among resistance training set combinations revealed significant increases in 1RM for all exercises compared to the control group, with no statistically significant differences between each training group. These findings are in line with previous research on 12-week set combinations, which reported significant increases in squat and bench press 1RM for compound sets and super sets (Hadi et al., 2018; Merrigan et al., 2019), respectively, while the pyramid set group exhibited significant increases in 1RM, but with no significant difference in strength gains compared to the drop set group (Angleri et al., 2017). In the 8-week resistance training program conducted in this study, free weight exercises involving technical requirements were considered closed kinetic chain exercises, which may have facilitated the development of muscular proprioception, thereby enhancing balance and coordination (Myhre, 2010; Wilson et al., 2018). However, despite the lack of observed differences between set combinations in this study, it can be inferred that compound sets, pyramid sets, and super sets are suitable for increasing 1RM demands, especially for novice individuals lacking exercise experience (Krieger, 2010; Schoenfeld et al., 2014).
The analysis of changes in isokinetic muscle function demonstrated significant improvements in shoulder and knee isokinetic muscle function in the pyramid set group compared to the control group. These results are consistent with previous research findings, indicating significant increases in muscle strength at 60°/sec after 8 weeks of upper limb resistance training (Alegre et al., 2015) and significant increases in knee flexor and extensor muscle strength after 12 weeks of resistance training in men (Teng et al., 2008; Sung et al., 2016). The progressive overload principle and sufficient rest intervals between sets, inherent in the pyramid set structure, may contribute to improving isokinetic muscle function (Franco et al., 2021; Mahjur and Norasteh, 2021).
Our findings diverge to some extent from previous research investigating the mechanical properties of muscles. Pintar et al. (2009) discovered that traditional isolated abdominal exercises did not significantly enhance the biomechanical performance of the abdominal muscles, while another study demonstrated that comprehensive core training could indeed increase abdominal wall activity and stability (Luo et al., 2022). This discrepancy may stem from differences in the training modalities and measurement methods employed across studies. Our research revealed that sustained pyramidal set training is advantageous for improving the stiffness and vibration frequency of the external oblique muscles, which aligns with the findings of Sundstrup et al. (2012), who reported that targeted training for the lateral abdominal muscles improved the biomechanical characteristics of those specific muscle groups. As a crucial component of the core musculature, optimal biomechanical performance of the external oblique muscles contributes to maintaining dynamic spinal stability. Notably, we did not observe significant differences in rectus abdominis biomechanical properties across different training modalities, which could be attributed to variations in training volume. Previous research has indicated that enhancing the biomechanical performance of the rectus abdominis requires a higher training volume (Lee, 2021).
However, some limitations must be acknowledged. Firstly, the study participants were restricted to a specific region, which may affect the generalizability and applicability of the study results to broader populations. Secondly, psychological factors influencing the study participants’ training performance and results, such as motivation, perceived exertion, and adherence, were not fully controlled, which could potentially impact the observed outcomes. Additionally, despite efforts to control the dietary and physiological phenomena of the study participants, some degree of uncertainty remains, which may impact the study results. For instance, variations in macronutrient intake, hydration levels, and sleep patterns could influence recovery and adaptation processes. Finally, the genetic characteristics of the study participants, which may influence their responses to training and results, were not considered in this study. Future investigations should aim to account for these factors to enhance the robustness and generalizability of the findings.
5 Conclusion
The findings of this study indicate that an 8-week resistance training program, comprising distinct combinations of training set configurations, yielded enhancements in fundamental fitness measures and 1RM performances. Notably, the pyramidal set training regimen exhibited pronounced benefits, enhancing isometric functional performance and core muscle biomechanical properties. However, further comparative and validation studies are warranted to elucidate the differential effects of various resistance training set configurations while accounting for covariates such as age, gender, and athletic proficiency. These rigorous research endeavors will provide a robust empirical foundation for tailoring resistance training interventions to specific goals and target populations.
Data availability statement
The raw data supporting the conclusions of this article will be made available by the authors, without undue reservation.
Ethics statement
The studies involving humans were approved by the Jeju National University (JJNU-IRB-2023-004). The studies were conducted in accordance with the local legislation and institutional requirements. The participants provided their written informed consent to participate in this study.
Author contributions
PL: Data curation, Formal Analysis, Writing–original draft. HY: Supervision, Writing–review and editing. YL: Conceptualization, Writing–review and editing. ZG: Validation, Writing–review and editing.
Funding
The author(s) declare that no financial support was received for the research, authorship, and/or publication of this article.
Conflict of interest
The authors declare that the research was conducted in the absence of any commercial or financial relationships that could be construed as a potential conflict of interest.
Publisher’s note
All claims expressed in this article are solely those of the authors and do not necessarily represent those of their affiliated organizations, or those of the publisher, the editors and the reviewers. Any product that may be evaluated in this article, or claim that may be made by its manufacturer, is not guaranteed or endorsed by the publisher.
References
Aagaard P., Simonsen E. B., Andersen J. L., Magnusson P., Dyhre-Poulsen P. (2002). Increased rate of force development and neural drive of human skeletal muscle following resistance training. J. Appl. Physiology 93 (4), 1318–1326. doi:10.1152/japplphysiol.00283.2002
Alegre L. M., Aguado X., Rojas-Martín D., Martín-García M., Ara I., Csapo R. (2015). Load-controlled moderate and high-intensity resistance training programs provoke similar strength gains in young women. Muscle & Nerve 51 (1), 92–101. doi:10.1002/mus.24271
Angleri V., Ugrinowitsch C., Libardi C. A. (2017). Crescent pyramid and drop-set systems do not promote greater strength gains, muscle hypertrophy, and changes on muscle architecture compared with traditional resistance training in well-trained men. Eur. J. Appl. Physiology 117, 359–369. doi:10.1007/s00421-016-3529-1
Baz-Valle E., Schoenfeld B. J., Torres-Unda J., Santos-Concejero J., Balsalobre-Fernández C. (2019). The effects of exercise variation in muscle thickness, maximal strength and motivation in resistance trained men. PloS One 14 (12), e0226989. doi:10.1371/journal.pone.0226989
Benito P. J., Cupeiro R., Ramos-Campo D. J., Alcaraz P. E., Rubio-Arias J. Á. (2020). A systematic review with meta-analysis of the effect of resistance training on whole-body muscle growth in healthy adult males. Int. J. Environ. Res. Public Health 17 (4), 1285. doi:10.3390/ijerph17041285
Chuang L. L., Lin K. C., Wu C. Y., Chang C. W., Chen H. C., Yin H. P., et al. (2013). Relative and absolute reliabilities of the myotonometric measurements of hemiparetic arms in patients with stroke. Archives Phys. Med. Rehabilitation 94 (3), 459–466. doi:10.1016/j.apmr.2012.08.212
Coratella G. (2022). Appropriate reporting of exercise variables in resistance training protocols: much more than load and number of repetitions. Sports Medicine-Open 8 (1), 99. doi:10.1186/s40798-022-00492-1
Cuevas-Aburto J., Jukic I., González-Hernández J. M., Janicijevic D., Barboza-González P., Chirosa-Ríos L. J., et al. (2020). Effect of resistance-training programs differing in set configuration on maximal strength and explosive-action performance. Int. J. Sports Physiology Perform. 16 (2), 243–249. doi:10.1123/ijspp.2019-1005
Dahmane R., Valenčič V., Knez N., Eržen I. (2001). Evaluation of the ability to make non-invasive estimation of muscle contractile properties on the basis of the muscle belly response. Med. Biol. Eng. Comput. 39, 51–5.
Delecluse C. (1997). Influence of strength training on sprint running performance: current findings and implications for training. Sports Med. 24, 147–156. doi:10.2165/00007256-199724030-00001
Fink J., Schoenfeld B. J., Sakamaki-Sunaga M., Nakazato K. (2021). Physiological responses to agonist–antagonist superset resistance training. J. Sci. Sport Exerc. 3, 355–363. doi:10.1007/s42978-020-00092-z
Florini J. R. (1987). Hormonal control of muscle growth. Muscle & Nerve Official J. Am. Assoc. Electrodiagn. Med. 10 (7), 577–598. doi:10.1002/mus.880100702
Folland J. P., Williams A. G. (2007). The adaptations to strength training: morphological and neurological contributions to increased strength. Sports Med. 37, 145–168. doi:10.2165/00007256-200737020-00004
Franco C. M., Carneiro M. A., de Sousa J. F., Gomes G. K., Orsatti F. L. (2021). Influence of high-and low-frequency resistance training on lean body mass and muscle strength gains in untrained men. J. Strength & Cond. Res. 35 (8), 2089–2094. doi:10.1519/JSC.0000000000003145
Hadi M., Soegiyanto M., Rahayu S., Setiono H. (2018). “Effectiveness of free weight exercise and super set machine system on strength and muscle hypertrophy,” in International conference on science and education and technology 2018 (ISET 2018) (Germany: Atlantis Press), 191–195.
Haff G. G., Triplett N. T. (2016). Essentials of strength training and conditioning. 4th edition (USA: Human kinetics).
Iversen V. M., Norum M., Schoenfeld B. J., Fimland M. S. (2021). No time to lift? Designing time-efficient training programs for strength and hypertrophy: a narrative review. Sports Med. 51 (10), 2079–2095.
Karasu A. U., Batur E. B., Karataş G. K. (2018). Effectiveness of Wii-based rehabilitation in stroke: a randomized controlled study. J. Rehabilitation Med. 50 (5), 406–412. doi:10.2340/16501977-2331
Kraemer W. J., Ratamess N. A., French D. N. (2002). Resistance training for health and performance. Curr. Sports Med. Rep. 1, 165–171. doi:10.1249/00149619-200206000-00007
Krieger J. W. (2010). Single vs. multiple sets of resistance exercise for muscle hypertrophy: a meta-analysis. J. Strength & Cond. Res. 24 (4), 1150–1159. doi:10.1519/JSC.0b013e3181d4d436
Lee K. (2021). The relationship of trunk muscle activation and core stability: a biomechanical analysis of pilates-based stabilization exercise. Int. J. Environ. Res. Public Health 18 (23), 12804. doi:10.3390/ijerph182312804
Luo S., Soh K. G., Soh K. L., Sun H., Nasiruddin N. J. M., Du C., et al. (2022). Effect of core training on skill performance among athletes: a systematic review. Front. Physiology 13, 915259. doi:10.3389/fphys.2022.915259
Mahjur M., Norasteh A. A. (2021). The effect of unsupervised home-based exercise training on physical functioning outcomes in older adults: a systematic review and meta-analysis of randomized controlled trials. Biol. Res. Nurs. 23 (3), 504–512. doi:10.1177/1099800421989439
Mangine G. T., Hoffman J. R., Gonzalez A. M., Townsend J. R., Wells A. J., Jajtner A. R., et al. (2015). The effect of training volume and intensity on improvements in muscular strength and size in resistance-trained men. Physiological Reports 3 (8), e12472.
Marusiak J., Jaskólska A., Koszewicz M., Budrewicz S., Jaskólski A. (2012). Myometry revealed medication-induced decrease in resting skeletal muscle stiffness in Parkinson’s disease patients. Clin. Biomech. 27 (6), 632–5.
Merrigan J. J., Jones M. T., White J. B. (2019). A Comparison of compound set and traditional set resistance training in women: changes in muscle strength, endurance, quantity, and architecture. J. Sci. Sport Exerc. 1, 264–272. doi:10.1007/s42978-019-00030-8
Mullix J., Warner M., Stokes M. (2012). Testing muscle tone and mechanical properties of rectus femoris and biceps femoris using a novel hand held MyotonPRO device: relative ratios and reliability. Working Papers in Health Sciences 1 (1), 1–8.
Myhre S. L. (2010). Bone Geometric adaptations and functional outcomes after ACL reconstruction: cross-sectional and prospective observations.
Paoli A., Gentil P., Moro T., Marcolin G., Bianco A. (2017). Resistance training with single vs. multi-joint exercises at equal total load volume: effects on body composition, cardiorespiratory fitness, and muscle strength. Front. Physiology 8, 1105. doi:10.3389/fphys.2017.01105
Pareja-Blanco F., Rodríguez-Rosell D., Sánchez-Medina L., Sanchis-Moysi J., Dorado C., Mora-Custodio R., et al. (2017). Effects of velocity loss during resistance training on athletic performance, strength gains and muscle adaptations. Scand. J. Med. Sci. Sports 27 (7), 724–735. doi:10.1111/sms.12678
Pintar J. A., Learman K. E., Rogers R. (2009). Traditional exercises do not have a significant impact on abdominal peak force in healthy young adults. J. Strength & Cond. Res. 23 (7), 2083–2089. doi:10.1519/JSC.0b013e3181b3e121
Prasetyo Y., Nasrulloh A. (2017). Weight training with pyramid systems to increase the leg and back muscular strength, grip strength, pull, and push strength. Man in India 97 (24), 193–201.
Razali N. M., Wah Y. B. (2011). Power comparisons of shapiro-wilk, kolmogorov-smirnov, lilliefors and anderson-darling tests. JOSMA 2 (1), 21–33.
Robbins D. W., Young W. B., Behm D. G., Payne W. R. (2010). The effect of a complex agonist and antagonist resistance training protocol on volume load, power output, electromyographic responses, and efficiency. J. Strength & Cond. Res. 24 (7), 1782–1789. doi:10.1519/JSC.0b013e3181dc3a53
Sayyah A., Asghari E., Arazi H. (2021). The effects of two loading patterns of resistance training (skewed pyramid & reverse step) on some physical and physiological capabilities of non-athlete men. Turkish J. Kinesiol. 7 (4), 123–131. doi:10.31459/turkjkin.1008780
Schoenfeld B. J. (2010). The mechanisms of muscle hypertrophy and their application to resistance training. J. Strength & Cond. Res. 24 (10), 2857–2872. doi:10.1519/JSC.0b013e3181e840f3
Schoenfeld B. J., Ratamess N. A., Peterson M. D., Contreras B., Sonmez G. T., Alvar B. A. (2014). Effects of different volume-equated resistance training loading strategies on muscular adaptations in well-trained men. J. Strength & Cond. Res. 28 (10), 2909–2918. doi:10.1519/JSC.0000000000000480
Seitz L. B., Reyes A., Tran T. T., de Villarreal E. S., Haff G. G. (2014). Increases in lower-body strength transfer positively to sprint performance: a systematic review with meta-analysis. Sports Med. 44, 1693–1702. doi:10.1007/s40279-014-0227-1
Sundstrup E., Jakobsen M. D., Andersen C. H., Zebis M. K., Mortensen O. S., Andersen L. L. (2012). Muscle activation strategies during strength training with heavy loading vs. repetitions to failure. J. Strength & Cond. Res. 26 (7), 1897–1903. doi:10.1519/JSC.0b013e318239c38e
Sung D. J., Park S. J., Kim S., Kwon M. S., Lim Y. T. (2016). Effects of core and non-dominant arm strength training on drive distance in elite golfers. J. Sport Health Sci. 5 (2), 219–225. doi:10.1016/j.jshs.2014.12.006
Teng W. M., Keong C. C., Ghosh A. K., Thimurayan V. (2008). Effects of a resistance training programme on isokinetic peak torque and anaerobic power of 13–16 years old Taekwondo athletes. Int. J. Sports Sci. Eng. 2 (2), 111–121.
Willis L. H., Slentz C. A., Bateman L. A., Shields A. T., Piner L. W., Bales C. W., et al. (2012). Effects of aerobic and/or resistance training on body mass and fat mass in overweight or obese adults. J. Appl. Physiology 113, 1831–1837. doi:10.1152/japplphysiol.01370.2011
Keywords: resistance training modalities, physical fitness, isokinetic muscle contraction, muscle biomechanics, pyramid set training
Citation: Liu P, Yuan H, Lu Y and Gao Z (2024) Resistance training modalities: comparative analysis of effects on physical fitness, isokinetic muscle functions, and core muscle biomechanics. Front. Physiol. 15:1424216. doi: 10.3389/fphys.2024.1424216
Received: 27 April 2024; Accepted: 20 June 2024;
Published: 12 July 2024.
Edited by:
Emiliano Cè, University of Milan, ItalyReviewed by:
Ali Md Nadzalan, Sultan Idris University of Education, MalaysiaGiuseppe Coratella, University of Milan, Italy
Copyright © 2024 Liu, Yuan, Lu and Gao. This is an open-access article distributed under the terms of the Creative Commons Attribution License (CC BY). The use, distribution or reproduction in other forums is permitted, provided the original author(s) and the copyright owner(s) are credited and that the original publication in this journal is cited, in accordance with accepted academic practice. No use, distribution or reproduction is permitted which does not comply with these terms.
*Correspondence: Han Yuan, 2021327546@knu.ac.kr