- 1School of Medicine and Dentistry, Griffith University, Gold Coast, QLD, Australia
- 2Spaceport Australia, Moree, NSW, Australia
- 3Faculty of Dentistry, The British University in Egypt, Cairo, Egypt
The health of astronauts during and after the return from long-haul space missions is paramount. There is plethora of research in the literature about the medical side of astronauts’ health, however, the dental and oral health of the space crew seem to be overlooked with limited information in the literature about the effects of the space environment and microgravity on the oral and dental tissues. In this article, we shed some light on the latest available research related to space dentistry and provide some hypotheses that could guide the directions of future research and help maintain the oral health of space crews. We also promote for the importance of regenerative medicine and dentistry as well highlight the opportunities available in the expanding field of bioprinting/biomanufacturing through utilizing the effects of microgravity on stem cells culture techniques. Finally, we provide recommendations for adopting a multidisciplinary approach for oral healthcare during long-haul space flights.
Introduction
Space flights have a significant effect on different tissues of the body (Iwase et al., 2020) and the oral cavity (Mednieks and Hand, 2019) including the nervous system (Onorato et al., 2020), musculoskeletal system (Juhl IV et al., 2021); bone (Stavnichuk et al., 2020), teeth (Moussa et al., 2023), saliva (Sun et al., 2022) and skin (Caswell and Eshelby, 2022). The majority of the changes are related to the changes in microgravity, increased exposure to radiation in particular the galactic cosmic rays (GCR), using dry washing technique, variations in air quality and changes in microbe replication and growth parameters which could be related to immunosuppression (Onorato et al., 2020; Stavnichuk et al., 2020; Juhl IV et al., 2021; Caswell and Eshelby, 2022; Sun et al., 2022). Furthermore, the interaction between microgravity and the exposure to ionizing radiation could have a substantial effect on physiological events and cellular responses (Yatagai et al., 2019).
Dental emergencies have a substantial impact on space missions to the extent that it has been classified as one of the top five essential conditions that need to be predicted before launching a space mission (Menon et al., 2012). As a result, The Space Medicine Exploration Medical Condition List included a number of dental conditions not limited to toothache, pulpitis, avulsion, tooth loss, crown replacement, and temporary fillings (Watkins et al., 2011; Johnston et al., 2014; Blue et al., 2019). More recent, a new medical conditions list was developed for space exploration flights based on reviewing information from nine previous lists in a systematic way by subject matter experts based on their history of occurrence in previous flights and citation in previous lists (Kreykes et al., 2023). It would not be a surprise to mention that dental conditions were a priority on the list. The above work related to medical conditions list relevant to space flights would help in reduction of the number of dental events. However, it should be taken into consideration that other physiological changes in the body due to the influence of microgravity (bone loss as an example), could indirectly result in dental disease and/or a relevant dental event during space flights as a result of upsetting the balance within other oral anatomical structures, saliva or oral microbiome (Lloro et al., 2020).
The effect of space flights on dental caries
Factors affecting dental caries progression in space
There are several factors that contribute to the development of relevant dental events in space. These factors include, increase in salivary immunogolbulins (Brown et al., 1974; Brown et al., 1976) and increase of salivary Mycoplasms (Brown et al., 1974). In longer space flights, anaerobic bacteria populations increased even in studies with simulated microgravity (Brown et al., 1976). Changes in the oral microbiome could be related to the lack of a balanced diet during space exploration missions (Tang et al., 2021) as well as the stress that astronauts have to endure. This highlights the importance of establishing sustainable food production systems (Tang et al., 2021; Pandith et al., 2023) and the mental wellbeing with regards to psychological support to astronauts (Arone et al., 2021; Oluwafemi et al., 2021). Furthermore, there is growing evidence that cosmic radiation during space travel alters gut microbiota including the Lactobacillus and Bacteroides families (Li et al., 2022). We hypothesize that the oral microbiome is also affected by cosmic radiation which could lead to accelerating the progression of dental caries and other forms of oral diseases through a number of mechanisms including the alteration of the immune response and mutation accumulation. The changes in the oral microbiome should not be taken lightly as it would lead to a state of chronic inflammation that could be a risk factor for increasing the chances of the incidence of other chronic conditions such as asthma, arthritis and ischemic heart disease (Lloro et al., 2020). Furthermore, the state of chronic inflammation in the oral cavity, increases the permeability of mucous membranes that could act as potential entry routes for microbes through the oral cavity, eyes, nose and respiratory tracts (Szot et al., 2017; Gomes et al., 2018).
Possible mechanisms of dental caries development and progression during space flights
There have been cases of dental pain related to caries progression during space flights reported with no success of identifying the origin of the problem (Gontcharov et al., 2005; Menon, 2012). It is understandable that progression of the caries would be related to the increase in anaerobic bacteria as described above and the elevated Streptococcus mutants count (Orsini et al., 2017) as well as the variations/evolution of S. mutants species that alters their survival and pathogenic properties (Fernander et al., 2022; Fernander et al., 2023). However, the origin of the carious lesion could have been an area of pre-existing demineralization or arrested caries. This raises the point of carefully selecting astronauts during the recruitment process and ensuring that all pre-existing lesions are treated as well as preventative measures applied to avoid caries progression. It could be argued that the increase in IgA and IgG discussed above could have contributed to increasing the adhesion of cariogenic substances and microbes to the salivary biofilm on the teeth surfaces (Costalonga and Herzberg, 2014). In addition to the above, studies have shown increased levels of glucosyltransferase enzymes during microgravity adding strength to the virulence of S. mutants through facilitating the synthesis of glucans from sucrose and mediating the attachment of micro-organisms to tooth surfaces (Omar et al., 2012).
Effects of microgravity on dental caries progression
Further investigating the hypothesis of increased virulence, gene expression and antibiotic resistance of pathogens in simulated microgravity (Taylor, 2015; Senatore et al., 2020) is not only important for astronauts but also is significant for understanding and exposing potential alternative mechanisms of disease progression that could be applied to clinical practice on earth (Salavatifar et al., 2023). In simulated microgravity, increased pain scores around the lower jaw especially around the submandibular and sublingual areas during mandibular movements could be related to the lack of resistance from gravity and the potential loss of muscle tone/strength during long term space flights which could eventually lead to changes in occlusion and/or teeth clashing in occlusal positions that are less ideal secondary to the muscular changes. Furthermore, the sleep disturbances and unregulated sleep patterns of astronauts could also contribute to the oral and dental issues described above as sleep deprivation is linked to higher levels of blood cortisol and elevated levels of stress which would result in physiological changes to the symbiosis of the oral cavity (Pavy Le-Traon and Roussel, 1993; Wu et al., 2018).
The effect of space flights on dental hard tissues
Effect of microgavity on craniofacial structures
Understanding the effects of microgravity on craniofacial structures is crucial to predict the risks during long-haul space flights in order to develop comprehensive plans for prevention and management of dental emergencies (Moussa et al., 2023). However, it has been noted that there is not a sufficient number of studies that discuss the effects of the space environment on many areas of the craniofacial complex such as the maxilla, temporomandibular joint, molar, premolar, and canine teeth, as well as small sample sizes for the studies of the mandible and incisors (Moussa et al., 2023). There are indications that space flights might induce changes in the teeth/dentition, for example, it was shown that the pulpal size increased in space flight animals which indicates deficiency in dentin formation and/or quality (Maupin et al., 2019). Furthermore, there were contradictory results related to incisor calcium (Ca) and phosphorus (P) content between studies conducted during the Cosmos-1887 mission (Simmons et al., 1990) and the Cosmos-1129 mission (Rosenberg et al., 1984). In the first study, the Ca and P content remained relatively unchanged (Simmons et al., 1990), while in the second study, the overall Ca and P content in the entire dentin was greater in space flights compared to ground crew samples but in the inner half of dentin (formed during the space flight) was relatively deficient (Rosenberg et al., 1984). It should be noted that according to our knowledge, no studies were conducted so far on the effect of cosmic radiation on dental hard tissue. We hypothesize that the exposure to higher radiation doses during long-haul space flights will negatively impact dental hard tissues based on the previous knowledge related to the development of radiation caries after the exposure to high doses of radiation during radiotherapy for cancer treatment which could change the mechanical properties, ultrastructure, appearance, crystal properties, and the chemical composition of dental hard tissue (Lu et al., 2019).
Directions for future research on the effect of space flights on teeth
With the limited data available on the effect of space flights on teeth and dental hard tissues, the results remain unconclusive. This is due to the absence of consistency between different studies where different factors were not standardized nor considered such as the duration of the space flight, diet, age and sex of the samples in each study (Moussa et al., 2023). Furthermore, most of the studies conducted on the effect of space flights on teeth/dental hard tissues used rodents which are completely different from humans that their incisors continuously erupt, the tooth enamel and dentin are constantly deposited by ameloblasts and odontoblasts (Goldberg et al., 2014). This makes the translation of results into human subjects inaccurate and unreliable. However, rodents’ molar teeth show limited continuous eruption and renewal of their dental tissues which can be a more accurate comparison to human teeth in response to environmental factors and space conditions including microgravity (Goldberg et al., 2014; Moussa et al., 2023). More studies are necessary to develop valid conclusions with regards to the effects of long-haul space flights on dental hard tissue and teeth in order to fill the current gaps in the literature and knowledge.
The effect of space flights on the alveolar bone
Effect of microgravity on bone loss
Cosmonauts experience three stages of physiologic adaptation resulting from altering gravity during space flights: 1) alterations upon entering weightless (initial adaptation), 2) modifications during extended time in weightlessness, and 3) re-adaptation to Earth’s gravity (Iwase et al., 2020). The immuno-haematological, bone metabolic, musculoskeletal, cardiovascular and neurovestibular systems of the body are each affected by weightlessness; alterations to these systems occur during these adaptation periods (Frippiat et al., 2016; Iwase et al., 2020; Tesei et al., 2022).
Loss of bone due to exposure to microgravity is a serious problem for the wellbeing of crew members on exploration flights (Oganov et al., 1991; Leblanc et al., 2000; Vico et al., 2000; Smith and Heer, 2002; Smith et al., 2005). Exposure to weightless causes bones’ fragility, which can affect them even after their return (Planel, 2004; Iwase et al., 2020). Although the exact process of loss of bone mineral during space missions is unknown, obviously it is multi-factorial (Zwart et al., 2018). Microgravity has significant negative consequences such as muscular atrophy and, more crucially, loss of bone. Throughout a mission to space, individuals lose a mean of between 0.5 and 2 percent of bone mass each month, or six to twenty-four percent annually (Hodkinson et al., 2017; Lloro et al., 2020; Stavnichuk et al., 2020; Moroni et al., 2022). Plenty of research indicate that weightless can induce osteoclastogenesis while increasing resorption of bones, either during long-haul space flights or in a simulated microgravity setting (Lloro et al., 2020).
Pharmacological approaches for a favourable bone response during space flights
As the density of bones is adequate before the commencement of flight, the best approach to pharmacotherapy preventing loss of bone is to avoid loss of bone rather than accelerating its production once stress is reduced throughout flight. Multiple medications have been suggested to assist with avoiding loss of bone in weightless (Iwase et al., 2020). Bisphosphonates possess two phosphonate groups as well as are structurally identical to pyrophosphate. They attach to hydroxyapatite in the bone matrix, preventing loss of bone by blocking osteoclastic resorption of bone. In addition, these medications have been shown to be beneficial for inhibiting the loss of bones throughout bedrest trials (Thompson et al., 1990; Grigoriev et al., 1992; Rodan and Fleisch, 1996; Iwase et al., 2020). Despite the inherent risk of bisphosphonate related osteonecrosis of the jaw (BRONJ) that is associated with bisphosphonate treatment, the risk is minimal during space travel due to the strict requirements and screening that astronauts go through before being accepted in the space crew. This minimizes the chances of needing invasive procedures that involve manipulation of the jawbone during space missions.
Amongst numerous forms of bisphosphonates, pamidronate has been proved to slow bones’ minerals loss and avoid the production of renal stones throughout bed rest trials (Watanabe et al., 2004; Iwase et al., 2020). In summary, the majority of the bone degradation seen in the astronauts who took part in this investigation may have non-reversible characteristics. Even though bisphosphonates, medications that prevent resorption of bone and are employed in conjunction with physical activity, have been demonstrated to be effective in maintaining DXA-BMD at the spine and hip levels, the reported variability in bone response pattern could result in unexpected treatment outcomes (LeBlanc et al., 2013). Although bisphosphonates and nutrition were regarded as therapeutic options to reduce loss of bone, exercising proved as the most effective (LeBlanc et al., 2002; LeBlanc et al., 2013; Hodkinson et al., 2017). As such, resistance exercise, particularly, is considered to promote osteogenesis (Guadalupe-Grau et al., 2009; Hodkinson et al., 2017).
Effects of microgravity and space travel on the mandible
Previous research investigated the implications of space travel and simulated microgravity on the mandible. In rats flown aboard Cosmos 1129 for 18.5 days, periosteal osteogenesis decreased in non-muscle-covered parts of the mandibular bone (molar area) (Simmons et al., 1990). The mesial portion of the first molar had less alveolar bone formation, suggesting a delay of mouse molars’ usual distal drift. There was also a reduction in alveolar bone mineral and collagen in the most matured parts, but elevated levels in the most undeveloped parts, indicating a delay in maturity (Simmons et al., 1990). The lower jaws of rats that travelled for a 12-day period aboard Cosmos 1887 exhibited comparatively elevated calcium and magnesium levels, even though their hydroxyapatite crystals were smaller (Simmons et al., 1990; Mednieks and Hand, 2019). Changes in bone architecture and blood vessels are less pronounced in the lower jaw than in the vertebra and the lower extremities’ long bone. In the Bion-M1 flying and habitat control mice, the reduction in blood vessels observed was probably attributable their consumption of soft paste food. Flight mice have a greater percentage of sclerostin-positive osteoclasts and osteocytes in their lower jaws, indicating that weightlessness might have a role (Mednieks and Hand, 2019).
Other animal and human investigations that extended multiple weeks indicated that reducing mastication and hence occlusal pressures influenced all tissues, such as bones and musculature (Philippou et al., 2015; Treffel et al., 2016). A different possible worry is osteonecrosis of the maxilla and mandible, but the likelihood is limited (Woo et al., 2005; Gill et al., 2009; Iwase et al., 2020). It should be noted that the issue of possible osteonecrosis is not related to space flights or microgravity but rather as a result of the side effects of the use of bisphosphonates. These osteolytic or osteonecrotic occurrences consistently coincide with tooth infection, iatrogenic trauma (tooth extraction/denture damage), or physiological strain (mastication) (Ruggiero et al., 2004; Ruggiero and Woo, 2008; Iwase et al., 2020). It is difficult to hypothesize based on the available animal studies (Ghosh et al., 2016; Moussa et al., 2023) that the alveolar is bone would be significantly impacted at a similar capacity in long-haul space flights as other bones in the body. Therefore, further research is deemed necessary to confirm the extent of the well-established negative effects of microgravity on skeletal bones and its correlation with the maxillary and mandibular alveolar bone.
The effect of space flights on the oral mucosa
Effect of salivary changes on the oral mucosa during space flights
Oral mucosa is the protective intra-oral layer that acts as barrier against oral infection as well as contributing to wound healing. It is always laminated by a thin film of saliva that keeps it wet. It has been demonstrated in literature that the salivary composition changes under simulated microgravity conditions (Sun et al., 2022). Furthermore, changes in the size of salivary glands, reduction in masticatory activity and changes in the expression of salivary proteins are also evident (Mednieks and Hand, 2019). To be more specific, it has been noted that simulated microgravity results in the shift in the salivary microbiome from oral health related bacteria to oral disease related bacteria with a trend towards reduction of the salivary PH which still remained alkaline (Sun et al., 2022). Another study revealed that salivary microbiome changes during space flights are correlated with viral reactivation of certain conditions that affect the oral mucosa (Urbaniak et al., 2020).
Oral mucosal conditions during space travel and their effect on the overall health
Multiple oral mucosa related issues have been associated with long-haul space flights and prolonged exposure to microgravity including sarcopenia (Runfeldt et al., 2019), peripheral nerves alterations, neuromotor plaque in the muscles of mastication, labial, lingual and buccal weakness, nociplastic pain in oral mucosal diseases, soft tissue changes and pathologies related to chewing, corticomotor neuroplasticity of tongue, and swallowing biomechanics (Dugan et al., 2023). Various modern techniques including transcranial electrical and magnetic stimulation (tES and TMS) which are non-invasive brain simulation techniques that are used in pain control and could be helpful in space flights (Ekhtiari et al., 2019). Furthermore, it has been proven that the space flights conditions had an impact on the overall microbiome of astronauts and the host-microbe interactions (Tesei et al., 2022). Probiotics have been suggested as promising countermeasures for health issues during long-haul space flights and we propose that this could be applied for oral diseases and infections (Bharindwal et al., 2023). Further studies are deemed necessary to validate this proposal.
Preventative measures against soft tissue infections during space flights
Due to the potential of an immune system drop (Sonnenfeld, 2005; Crucian et al., 2008) and delayed wound healing (Davidson et al., 1998) during long-haul space flights, every effort should be made to minimize the chances of infection in human space travel. Exercise (Shearer et al., 2009), vitamin D supplements (Martineau et al., 2011), maintaining a healthy microbiological status (Ilyin, 2005), waterless hand hygiene products, personal protective equipment, chlorhexidine mouth rinses, gamma irradiation of selected foods and ultraviolet light for disinfecting surfaces (Mermel, 2013) are highly important due to the increased chances of opportunistic infections in space. An interesting finding from a recent study showed that Bacillus horneckiae, that was first identified within the Kennedy Space Centre was present in the impression disinfection solution in a university clinic (Chukwu et al., 2024). This bacterial species was not killed with the industry standard impression disinfectant solution and its pathogenicity is still unknown (Chukwu et al., 2024). This highlights the high variability in bacterial species during long-haul space flights and the need for strict infection control/prevention measures during space flights.
The effect of space flights on periodontal disease
Microgravity as a risk factor for periodontal disease
The revelation that periodontal issues are the next most prevalent dental disease in prolonged solitary settings implies that such long duration has an impact on oral hygiene, however, it is reported that the increased risk for periodontal disease in long-haul space flights is mainly related to less than optimal oral care, environmental factors as the dry and cold air in spaceships as well as the lack of pre-mission visits to the dentist for regular check-ups and regular professional dental care (Kupper et al., 2014). That scenario might be readily resolved by teaching the aboard medical staff on dental hygiene using an ultrasonic scaler and demanding everyone onboard to get regular professional hygiene (Lloro et al., 2019). In addition, low salivary flow and dry mouth increase the risk of caries and periodontal disorders; dry mouth can be caused by inhaling dry compressed gases in an aeroplane (Fontana and Zero, 2006; Pathak, 2015).
Microgravity and low-shear stress can cause an imbalance in microbial communities and alter bacterial physiological processes (Nickerson et al., 2000; Nickerson et al., 2004; Wilson et al., 2007; Castro-Wallace et al., 2017; Fernander et al., 2022). Oral occurrences reported from lengthy as well as weightless operations included a rise in streptococcal and anaerobic components (Streptococcus mutans) in saliva and dental plaque microorganisms in addition to a growth in IgA in saliva as well as demonstrating an elevated prevalence over brief durations (Lloro et al., 2020). The gastrointestinal tract is the most complex community followed by the human mouth which has over 1000 distinct species (Dewhirst et al., 2010; Human Microbiome Project Consortium, 2012; Fernander et al., 2022). Studies have demonstrated that microorganisms can create biofilms, produce extracellular polysaccharides, grow faster, change their pathogenic stress response and virulence, acquire new resistance, and produce more secondary metabolites when exposed to simulated microgravity (Fang et al., 1997; Nickerson et al., 2000; McLean et al., 2001; Brown et al., 2002; Lynch et al., 2004; Allen et al., 2008; Crabbé et al., 2008; Mauclaire and Egli, 2010; Castro et al., 2011; Gilbert et al., 2020; Fernander et al., 2022).
Biofilm considerations for periodontal disease during long-haul space flights
Despite environmental stresses such as eating habits, pH, temperature, and flow of saliva, bacterial communities may maintain the density of cells of up to 100 CFU mL (Evaldson et al., 1982; Kolenbrander et al., 2006; Palmer et al., 2006; Kreth et al., 2008; Fernander et al., 2022). Oral microorganisms contribute to both systemic and oral health because bacteria live on all of the oral cavity’s surfaces (teeth, tongue, gums, etc.), blocking pathogen colonization. (Shroff et al., 1995; Hooper et al., 2012; Fernander et al., 2022). Nevertheless, tooth caries is still amid the most common disorders among people (Takahashi and Nyvad, 2011; Fernander et al., 2022). The phenotypes of the bacteria causing periodontal disease on earth are extensively known, and treatment options are more predictable, but this may not be the case during long-term space flight (Marsh, 1999; Schachtele et al., 2007; Gross et al., 2010; Fernander et al., 2022). A simulated study of a Skylab mission found that secretory immunoglobulin A levels steadily rose in chamber confinement, peaking at the 55th day of monitoring. Though the alterations in enteric bacilli and mycoplasma were statistically noteworthy, there was not a spike in Streptococcus mutans in saliva. Nevertheless, a study showed a rise in Streptococcus mutans in plaque on teeth, which was linked to a diet high in sugar (Brown et al., 1974; Lloro et al., 2020).
Bacteroides species, Veillonella species, Fusobacterium species, Neisseria species, and Streptococcus mutans appear in larger quantities in periodontitis when compared to the healthy status of the oral cavity (Costalonga and Herzberg, 2014). These bacterial species were present and proliferated in the oral microbiome of the astronauts over three Skylab missions (Lloro et al., 2020). Veillonella species in plaque might account for as much as 45 percent throughout the early phases of periodontal or gingival inflammation. In addition to other risk factors, Fusobacterium species is a direct bacterial pathogen that contributes to the development of several periodontal disorders (Ardila et al., 2010; Lloro et al., 2020). L. salivarius, P. denticolens, S. mutans and S. wiggsiae were nearly entirely detected in plaques derived from dentinal caries. However, in infants, S. sanguinis and some species of Neisseria and Leptotrichia were regularly discovered in healthy tooth’s plaque (Richards et al., 2017). Similar bacterial strains were evident under the effect of microgravity, but the question remains on whether the changes in the oral microbiome is solely related to the effect of microgravity or other factors as radiation, isolation, stress which also affect oral and systemic health in space (Lloro et al., 2020). The significance of the development of periodontitis after bacterial proliferation is clear given that, in an individual suffering from a mild periodontal inflammation, the portion of the periodontal bag directly contacting the bacterial plaque is around 72 cm2, which is roughly the human palm’s size (Page, 1998; Lloro et al., 2020). Table 1 summarizes the bacterial species found during space missions and their role in development of dental diseases on earth.
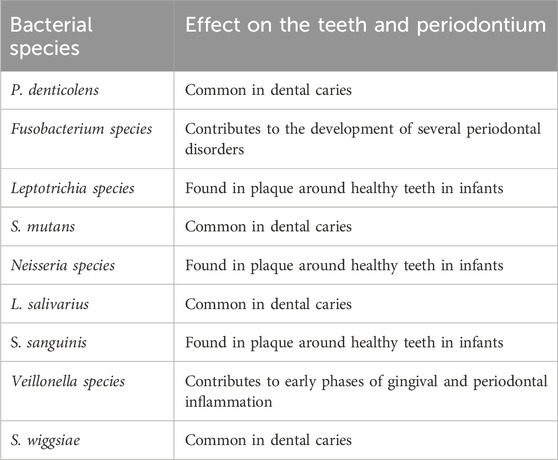
Table 1. A summary of the bacterial phenotypes isolated from different space missions and their origin as well as role in dental and/or periodontal disease on earth.
Mechanism of development and progression of periodontal disease in space travellers
A comprehensive evaluation of the influence of periodontal condition on cortisol levels in saliva demonstrated its rise proportional with the advancement and extent of periodontitis (Botelho et al., 2018; Lloro et al., 2020). Cortisol is an oxidative damage mediator; hence it could be contributory to these variations as oxidative stress appears to play a significant role in advanced periodontal inflammation (Aschbacher et al., 2013; Acquier et al., 2017; Lloro et al., 2020). Based on these findings, a study determined that adding cortisol to the culture media at 12 and 24 hours drastically boosted the development rate of Porphyromonas gingivalis dose-independently. This study indicates that cortisol could have a particular influence on the development of Porphyromonas gingivalis (Akcali et al., 2014; Lloro et al., 2020). This aligns with recent literature indicating that elevated cortisol levels have a significant effect on the immune system’s response in particular T-cells (Miranda et al., 2023) and antimicrobial salivary proteins (Agha et al., 2020). However, it should be noted that other studies indicated that different space flight stressors (rather than cortisol alone) may interact and produce adverse health effects amongst space travellers (Radstake et al., 2022). This area of research needs further exploration as cortisol levels fluctuate during space flights as a result of altered sleep patterns (Gemignani et al., 2014). We propose that further space environment simulation studies are necessary to understand the full effect of different stressors (including cortisol) on the overall physical and mental health of astronauts. We recommend that collecting salivary samples to measure cortisol levels would be a convenient tool for sample collection.
Considering how alterations in cosmonaut physiological processes impact periodontal state, it is critical to emphasize its biologic link with systemic illnesses such as obstetric difficulties, cardiovascular disease, diabetes, chronic respiratory diseases, and cancer (Akcali et al., 2014; Bui et al., 2019; Lloro et al., 2020). The research extensively reports a link amongst periodontitis, aging, and chronic non-communicable illnesses (Bui et al., 2019). A consistent theme that arose was the effects on low-grade systemic inflammation caused by periodontal bacteraemia/endotoxemia caused by daily activities such as eating and teeth brushing via acute-phase (C-reactive protein, CRP) and neutrophil oxidative stress responses (Sanz et al., 2018; Lloro et al., 2020).
Preventive measures and prior investigations significantly reduce dental incidents. This underscores the need for specialized dental staff and supplies during unique situations, particularly in upcoming long-term space trips (Lloro et al., 2019). To minimize the chance of contracting infections in space, both the team and the spacecraft are subjected to extensive microbial screening before the mission. However, this precautionary measure might not avoid new evolving characteristics from emerging within the member’s microflora because of being subjected to the new conditions found during long-haul space flights (Barratt and Pool, 2008; Fernander et al., 2022).
There are limited studies that discuss the effect of space flights on periodontal disease. However, several studies have shown that motility and chemotaxis of some bacterial strains increased under microgravity conditions (Acres et al., 2021). Furthermore, it was reported that other environmental and genetic host-related factors increased the chances of biofilm formation (Rossi et al., 2018), affected oxygen availability (Marteyn et al., 2011), increased adhesion and invasion of epithelial cells (Lee and Falkow, 1990). The above changes were attributed to the downtrend of the regulation of expression of hfq which is an important post-transcriptional factor that facilitates the pairing of small RNAs with their target mRNAs with an important role in bacteria (Valentin-Hansen et al., 2004; Gottesman and Storz, 2011; Vogel and Luisi, 2011). Decreased hfq expression was evident in simulated microgravity studies and consistent with stress response studies (Gangaiah et al., 2014; Kim et al., 2015; Wang et al., 2018). We cannot exclude the possibility of ionizing/cosmic radiation affecting the microbiome via mutation accumulation. One of the under-rated biological hazards of ionizing radiation during space travel is microbiome-mediated pathophysiology (Casero et al., 2017), which warrants further investigations to establish the chronic effects of radiation on the oral health. Most of the available literature is related to gut microbiota rather than the oral microbiome and is mainly based on animal models (Fernandes et al., 2023).
In addition to the bacterial-related factors, we believe that the changes in bone and salivary microbiome discussed above as well as the potential drop in immunity as result of prolonged exposure to space radiation and microgravity (Cao, 2022) could contribute to the development of periodontal disease during long-haul space flights. It has been reported that simulated microgravity increased the salivary levels of Actinomyces species which has a high affinity to sticking on the tooth and root surfaces as well as high association with periodontal disease and apical periodontitis (Do et al., 2017; Sun et al., 2022). Furthermore, it has been shown that the salivary microbiome changes during space flights with Streptococcus mutants being the most abundant species contributing to 8% of the total micro-organisms detected (Urbaniak et al., 2020). This supports our hypothesis that the incidence of periodontal disease would tend to increase during long-haul space flights. Furthermore, the reason behind the scarcity of studies around periodontal health in space could be related to the fact that all astronauts undergo a rigorous dental check-up before embarking on space flights to ensure that their dental and periodontal tissues are in a meticulous condition to avoid the need for emergency treatment during long-haul space flights. As a result, all astronauts are selected with no existing ongoing chronic dental and/or periodontal conditions.
Regenerative medicine and dentistry and space flights
Challenges and advancements in bioengineering research in space
The lengthy space flights could result in progressive tissue degradation and an increased susceptibility to injury. Therefore, the field of regenerative dentistry, medicine, surgical repair and advancement of wound healing is highly important (Iordachescu et al., 2023). Due to isolation, distance, and the absence of a possible emergency evacuation plan in space, the design of facilities, infrastructure and the specialized equipment required remain a challenging task to the rapidly developing field of regenerative bioengineering (Iordachescu et al., 2023). In addition to the above, the exposure to extensive cosmic radiation complicates the requirements for development of research facilities in during space missions (Röstel et al., 2020).
Despite the obstacles mentioned in the previous paragraph, developments are ongoing in the fields of research of stem cells (Blaber et al., 2014), spheroids - a form of spherical agglomerates of cells (Pietsch et al., 2017), organs-onchips (Low and Giulianotti, 2019) and the implementation of biomanufacturing/bioprinting (Cubo-Mateo et al., 2020; Sharma et al., 2022). Examples of the progress in the field of stem cell research on earth are some FDA-approved therapeutic products including cultured epidermal autografts (Epicell®) that allows treating deep dermal burns (Brockmann et al., 2018), or the autologous cultured chondrocytes on porcine collagen membranes (MACI®) for fixing cartilage defects (Bliley et al., 2022). There are limitless applications for biofabrication techniques in space so that space flights become self-sufficient (Moroni et al., 2022).
Tissue engineering and space travel
While microgravity could be viewed as a hurdle for tissue engineering, however, it is a valuable tool that can be utilized in cell culture techniques especially the stem cell culture environment for cell-based therapy (Imura et al., 2019). Furthermore, bone marrow stromal (BMSCs) and bone-derived mesenchymal stem cells that were cultured under simulated microgravity environments had great success in treating different spinal cord and brain injuries through its neuroprotective properties (Yuge et al., 2011; Mitsuhara et al., 2013; Otsuka et al., 2018). In addition to the above, differentiation and growth of dental pulp stem cells was enhanced in animal model that used simulated microgravity (Li et al., 2017) and the 3D growth and architecture of ameloblast-like cells as well as periodontal ligament cells engineering were improved under the microgravity conditions (Pandya et al., 2021). This could open the horizon for rapid developments in the field of regenerative dentistry and tooth vitality. Finally, in order to fully understand best possible ways to overcome the effect of microgravity and galactic cosmic rays (GCRs) on different tissues including the nervous system, the development of animal experimental models could be an alternative method to overcome the challenges described above and accelerate the development of knowledge in the field of regenerative medicine/dentistry and bioengineering in space missions (Onorato et al., 2020). The outcomes from human research conducted in space are of extreme scientific/biomedical importance and translate to medical care on Earth (Shelhamer et al., 2020).
A multidisciplinary approach to maintaining oral health during space flights
The importance of treatment planning in aerospace dentistry
Dentists are increasingly concerned about dental issues in solitary and confined groups, particularly those who are isolated or have no access to healthcare. This is especially important given the growth of manned space programs, Antarctica research, and submarine operations. Financial expenses for treatment, specialized dental staff, evacuation, and transportation to dental institutions vary based on the frequency of acute dental occurrences and the dental care provided (Lloro et al., 2019). Therefore, dental planning is crucial for maintaining aircrews’ operational health, especially at higher altitudes, and ensuring oral health to enable uninterrupted tasks (Pathak, 2015; Pathak et al., 2015; Lloro et al., 2019). Aerospace dentistry focuses on enhancing the dental and oral hygiene of crew members, particularly in preventing illnesses caused by changes in atmospheric pressure (Pathak, 2015; Pathak et al., 2015). High-altitude fluctuations can cause discomfort, pain, and organ malfunction like periodontitis, dental abscesses, and deep carious lesions, and for those reasons, a proper assessment could help in preventing them (Pathak, 2015; Pathak et al., 2015).
NASA’s mission to establish human settlement on Mars is a growing concern due to potential risks to mission goals; one of which is that crew members face microbiological issues during long-haul space flights, potentially limiting their production and the ships’ integrity. To minimize infection, extensive microbial screening is conducted (Fernander et al., 2022) The implementation of prevention strategies and prior screenings significantly reduce oral issues rates of occurrence (Lloro et al., 2019). However, the crew’s microflora may develop unique evolutionary traits due to their exposure (Fernander et al., 2022; Fernander et al., 2023). NASA has developed tight requirements for cosmonaut choosing, continuation, as well as prior to flight oral examinations for particular space flights, plus a rigid clinical regimen. Astronauts undergo rigorous screenings 24 weeks before departure, including dental care at least 12 weeks before missions.
Dental emergencies during long-haul space flights
The Space Medicine Exploration Medical Condition List states that basic dental treatments and diagnoses will be accessible (Blue et al., 2019); furthermore, dental emergencies were forecasted to be one of the leading circumstances influencing subsequent mission goals by the Integrated Mathematical Medical Model (Fernander et al., 2022). As time progresses, space medicine is achieving more recognition as a speciality that is involved in different phases of the space flight from the crew selection, training and the space flight itself to post-flight rehabilitation and long-term health of astronauts (Hodkinson et al., 2017). Table 2 summarizes the possible dental emergencies that can occur during as well as before and after space flights. This is extremely important given the complexity of the nature risk factors that astronauts are exposed to during long-haul space flights (Figure 1).
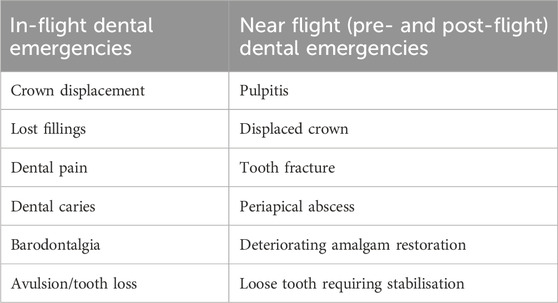
Table 2. Presenting a summary of the possible dental emergencies that require medical attention during as well as before and after space flights.
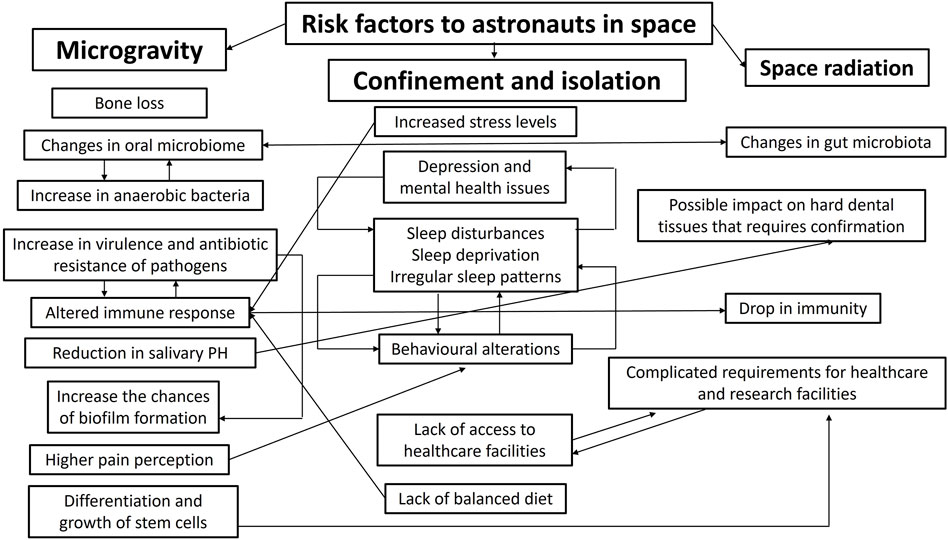
Figure 1. Summarizes the possible correlations between risk factors to astronauts in space and illustrates the complex nature of the health-related challenges in long-haul space flights.
A dentist would be crucial during long-term space trips to Mars, as full dental treatment, radiographs, and root canals are unavailable in space. Aeronautic dentistry is a relatively new field that has received little attention (Pathak, 2015; Pathak et al., 2015). It is crucial that the aviation sector promotes treatment protocols and diagnostic tools to safeguard the health of its crew, as clinical aeronautic dentistry aims to restore crew members to full wellness after oral emergencies (Pathak, 2015; Pathak et al., 2015), in addition, dental professionals and flight crews should take advantage of any opportunity to incorporate dental and oral health into crews’ physiological prerequisites in order to boost their overall health (Pathak, 2015; Pathak et al., 2015).
Special precautions are necessary for aircrew patients during restorative, endodontic, oral and maxillofacial surgical, and prosthodontics treatments to prevent onboard incapabilities and potential major problems (Pathak, 2015; Pathak et al., 2015; Lloro et al., 2019). Excavating dental decays and restoring them must be performed ahead of flight departure, also leaking fillings ought to get replaced after which an extensive inspection of the cavity floor is necessary to avoid pulp chamber penetration, and a protective cavity liner like glass-ionomer cement is applied. For successful multi-visit root canal therapy, the interim restoration should be appropriately positioned (Pathak, 2015; Pathak et al., 2015). Although, in 1995, root canal therapy for a suspicious pulp chamber invasion in a crew member was needed in order to avoid silent pulp necrosis and sub-acute pulpitis, and their pressure related complications; moreover, when not properly addressed, endodontic infections might result in leaking of diseased tissue into the peri-radicular tissues and subcutaneous emphysema (Niazi and Bakhsh, 2022).
Preventative measures against dental problems before and during space flights
Cuspal covering indirect restorations can serve as preventative dentistry. Moreover, resin cements are recommended for cementation of indirect restoration due to their superior retention and decreased porosity (Lyons et al., 1997; Pathak, 2015; Pathak et al., 2015). Eating sweets or chewing gum during missions can increases salivary output hence prevent dry mouth; noting that dry mouth is correlated with periodontal diseases and high caries incidence. Once a maxillary tooth is extracted, oral surgeons should check for oro-antral communication, which may cause sinusitis upon exposure to a fluctuating pressure; if present, an oro-antral communication ought to be sutured. Furthermore, osseointegrated dental implants may enhance edentulous denture retention (Pathak, 2015; Pathak et al., 2015).
NASA does not verify an individual onboard prevention technique without considering different bone-loss strategies for each crew. Moreover, pharmaceutical therapies may prevent bone loss during space flights, but prospective studies should be meticulously designed to yield unambiguous results (Orwoll et al., 2013). Finally, in addition to the medical and dental considerations discussed above, it is essential to understand that a holistic approach needs to be taken into account while planning for the health, safety and wellbeing of the astronauts in long-haul space flights. This should take into account the psychological aspects of the astronauts’ health through promotion of positive emotions, subjective resilience and the prevention of the development of emotional disorders to allow adaptation to extreme environments (Gatti et al., 2022). Finally, Table 3 summarises the recommended directions for future research that are deemed necessary to advance the field of aerospace dentistry and provide a holistic approach to the health and wellbeing of astronauts during long-haul space flights.
Conclusion
This review summarizes the available research with regards to the effects of microgravity and long-haul space flights on oral and dental tissues. We provide a few hypotheses that could contribute to expanding the current available knowledge in this field. Dentistry in space travel is best incorporated within a medical team that provides a holistic and multidisciplinary healthcare approach to the astronauts. Furthermore, the most significant evidence-based changes within the oral cavity that are related to the space environment are changes in the salivary components and oral microbiology. Finally, we highlight the promising potentials for space travel in regenerative medicine and dentistry, especially with regards to the utilization of stem cell culture and bioengineering/biomanufacturing. The information presented in the current review helps provide directions for future research as well as recommendations for maintaining the health of astronauts and preventing the long-term negative effects of space travel and microgravity.
Author contributions
MB: Conceptualization, Funding acquisition, Supervision, Writing–review and editing, Data curation, Formal Analysis, Investigation, Methodology, Project administration, Resources, Software, Validation, Visualization, Writing–original draft. GC: Conceptualization, Funding acquisition, Supervision, Writing–review and editing. HH: Conceptualization, Data curation, Formal Analysis, Funding acquisition, Investigation, Methodology, Project administration, Resources, Software, Supervision, Validation, Visualization, Writing–original draft, Writing–review and editing. MS: Conceptualization, Data curation, Formal Analysis, Funding acquisition, Investigation, Methodology, Project administration, Resources, Software, Supervision, Validation, Visualization, Writing–original draft, Writing–review and editing. MA-A: Conceptualization, Data curation, Formal Analysis, Funding acquisition, Investigation, Methodology, Project administration, Resources, Software, Supervision, Validation, Visualization, Writing–original draft, Writing–review and editing.
Funding
The authors declare that no financial support was received for the research, authorship, and/or publication of this article.
Conflict of interest
Author GC was employed by the company Spaceport Australia.
The remaining authors declare that the research was conducted in the absence of any commercial or financial relationships that could be construed as a potential conflict of interest.
Publisher’s note
All claims expressed in this article are solely those of the authors and do not necessarily represent those of their affiliated organizations, or those of the publisher, the editors and the reviewers. Any product that may be evaluated in this article, or claim that may be made by its manufacturer, is not guaranteed or endorsed by the publisher.
References
Acquier A. B., De Couto Pita A. K., Busch L., Sánchez G. A. (2017). Parameters of oxidative stress in saliva from patients with aggressive and chronic periodontitis. Redox Rep. 22 (3), 119–126. doi:10.1080/13510002.2016.1198104
Acres J. M., Youngapelian M. J., Nadeau J. (2021). The influence of space flight and simulated microgravity on bacterial motility and chemotaxis. npj Microgravity 400 (1), 7. doi:10.1038/s41526-021-00135-x
Agha N. H., Baker F. L., Kunz H. E., Spielmann G., Mylabathula P. L., Rooney B. V., et al. (2020). Salivary antimicrobial proteins and stress biomarkers are elevated during a 6-month mission to the International Space Station. J. Appl. Physiology 128 (2), 264–275. doi:10.1152/japplphysiol.00560.2019
Akcalı A., Huck O., Buduneli N., Davideau J. L., Köse T., Tenenbaum H. (2014). Exposure of Porphyromonas gingivalis to cortisol increases bacterial growth. Archives Oral Biol. 59 (1), 30–34. doi:10.1016/j.archoralbio.2013.09.003
Allen C. A., Niesel D. W., Torres A. G. (2008). The effects of low-shear stress on Adherent-invasive Escherichia coli. Environ. Microbiol. 10 (6), 1512–1525. doi:10.1111/j.1462-2920.2008.01567.x
Ardila C. M., Granada M. I., Guzmán I. C. (2010). Antibiotic resistance of subgingival species in chronic periodontitis patients. J. Periodontal Res. 409 (4), 557–563. doi:10.1111/j.1600-0765.2010.01274.x
Arone A., Ivaldi T., Loganovsky K., Palermo S., Parra E., Flamini W., et al. (2021). The burden of space exploration on the mental health of astronauts: a narrative review. Clin. Neuropsychiatry 18 (5), 237–246. doi:10.36131/cnfioritieditore20210502
Aschbacher K., O'Donovan A., Wolkowitz O. M., Dhabhar F. S., Su Y., Epel E. (2013). Good stress, bad stress and oxidative stress: insights from anticipatory cortisol reactivity. Psychoneuroendocrinology 38 (9), 1698–1708. doi:10.1016/j.psyneuen.2013.02.004
Barratt M. R., Pool S. L. (2008). Principles of Clinical Medicine for Space Flight. First Edition (New York City: Springer), 27–57.
Bharindwal S., Goswami N., Jha P., Pandey S., Jobby R. (2023). Prospective use of probiotics to maintain astronaut health during space flight. Life 13 (3), 727. doi:10.3390/life13030727
Blaber E., Sato K., Almeida E. A. (2014). Stem cell health and tissue regeneration in microgravity. Stem Cells Dev. 23, 73–78. doi:10.1089/scd.2014.0408
Bliley J. M., Shiwarski D. J., Feinberg A. W. (2022). 3D-bioprinted human tissue and the path toward clinical translation. Sci. Transl. Med. 14 (666). Article eabo7047. doi:10.1126/scitranslmed.abo7047
Blue R., Nusbaum D., Antonsen E. (2019). Development of an accepted medical condition list for exploration medical capability scoping. Johns. Space Cent. Houst. Tex.
Botelho J., Machado V., Mascarenhas P., Rua J., Alves R., Cavacas M. A., et al. (2018). Stress, salivary cortisol, and periodontitis: a systematic review and meta-analysis of observational studies. Archives Oral Biol. 96, 58–65. doi:10.1016/j.archoralbio.2018.08.016
Brockmann I., Ehrenpfordt J., Sturmheit T., Brandenburger M., Kruse C., Zille M., et al. (2018). Skin-derived stem cells for wound treatment using cultured epidermal autografts: clinical applications and challenges. Stem Cells Int. 2018, 4623615. Article 4623615. doi:10.1155/2018/4623615
Brown L. R., Frome W. J., Handler S. F., Wheatcroft M. G., Johnston D. A. (1976). Effect of Skylab missions on clinical and microbiologic aspects of oral health. J. Am. Dent. Assoc. 93, 357–363. doi:10.14219/jada.archive.1976.0502
Brown L. R., Wheatcroft M. G., Frome W. J., Rider L. J. (1974). Effects of a simulated Skylab mission on the oral health of astronauts. J. Dent. Res. 53, 1268–1275. doi:10.1177/00220345740530053201
Brown R. B., Klaus D., Todd P. (2002). Effects of space flight, clinorotation, and centrifugation on the substrate utilization efficiency of E. coli. Microgravity Sci. Technol. 13 (4), 24–29. doi:10.1007/BF02881678
Bui F. Q., Almeida-da-Silva C. L. C., Huynh B., Trinh A., Liu J., Woodward J., et al. (2019). Association between periodontal pathogens and systemic disease. Biomed. J. 42 (1), 27–35. doi:10.1016/j.bj.2018.12.001
Cao X. (2022). Research progress on the effects of microgravity and space radiation on astronauts’ health and nursing measures. Open Astron. 31, 300–309. doi:10.1515/astro-2022-0038
Casero D., Gill K., Sridharan V., Koturbash I., Nelson G., Hauer-Jensen M., et al. (2017). Space-type radiation induces multimodal responses in the mouse gut microbiome and metabolome. Microbiome 5 (1), 105. doi:10.1186/s40168-017-0325-z
Castro S. L., Nelman-Gonzalez M., Nickerson C. A., Ott C. M. (2011). Induction of attachment-independent biofilm formation and repression of Hfq expression by low-fluid-shear culture of Staphylococcus aureus. Appl. Environ. Microbiol. 77 (18), 6368–6378. doi:10.1128/AEM.00175-11
Castro-Wallace S. L., Chiu C. Y., John K. K., Stahl S. E., Rubins K. H., McIntyre A. B. R, et al. (2017). Nanopore DNA sequencing and genome assembly on the international space station. Sci. Rep. 7 (1), 18022. doi:10.1038/s41598-017-18364-0
Caswell G., Eshelby B. (2022). Skin microbiome considerations for long haul space flights. Front. Cell Dev. Biol. 10. Article 956432. doi:10.3389/fcell.2022.956432
Chukwu S., Munn A., Wilson J. C., Ibrahim H., Gosling D., Love R. M., et al. (2024). Efficacy of an impression disinfectant solution after repeated use: an in vitro study. Heliyon 10 (1), e23792. Article e23792. doi:10.1016/j.heliyon.2023.e23792
Costalonga M., Herzberg M. C. (2014). The oral microbiome and the immunobiology of periodontal disease and caries. Immunol. Lett. 162 (2 Part A), 22–38. doi:10.1016/j.imlet.2014.08.017
Crabbé A., De Boever P., Van Houdt R., Moors H., Mergeay M., Cornelis P. (2008). Use of the rotating wall vessel technology to study the effect of shear stress on growth behaviour of Pseudomonas aeruginosa PA01. Environ. Microbiol. 10 (8), 2098–2110. doi:10.1111/j.1462-2920.2008.01631.x
Crucian B. E., Stowe R. P., Pierson D. L., Sams C. F. (2008). Immune system dysregulation following short-vs long-duration space flight. Aviat. Space, Environ. Med. 79, 835–843. doi:10.3357/asem.2276.2008
Cubo-Mateo N., Podhajsky S., Knickmann D., Slenzka K., Ghidini T., Gelinsky M. (2020). Can 3D bioprinting be a key for exploratory missions and human settlements on the Moon and Mars? Biofabrication 12 (4), 043001. doi:10.1088/17585090/abb53a
Davidson J. M., Aquino A. M., Woodward S. C., Wilfinger W. W. (1998). Sustained microgravity reduces intrinsic wound healing and growth factor responses in the rat. FASEB J. 13, 325–329. doi:10.1096/fasebj.13.2.325
Dewhirst F. E., Chen T., Izard J., Paster B. J., Tanner A. C., Yu W. H., et al. (2010). The human oral microbiome. J. Bacteriol. 192 (19), 5002–5017. doi:10.1128/JB.00542-10
Do T., Damé-Teixeira N., Naginyte M., Marsh P. D. (2017). Root surface biofilms and caries. Monogr. Oral Sci. 26, 26–34. doi:10.1159/000479304
Dugan C., Parlatescu I., Popescu B. O., Pop C. S., Marin M., Dinculescu A., et al. (2023). Applications for oral research in microgravity - lessons learned from burning mouth syndrome and ageing studies. J. Med. Life 16 (3), 381–386. doi:10.25122/jml-2022-0285
Ekhtiari H., Tavakoli H., Addolorato G., Baeken C., Bonci A., Campanella S., et al. (2019). Transcranial electrical and magnetic stimulation (tES and TMS) for addiction medicine: a consensus paper on the present state of the science and the road ahead. Neurosci. Biobehav. Rev. 104, 118–140. doi:10.1016/j.neubiorev.2019.06.007
Evaldson G., Heimdahl A., Kager L., Nord C. E. (1982). The normal human anaerobic microflora. Scand. J. Infect. Dis. Suppl. 35, 9–15.
Fang A., Pierson D. L., Koenig D. W., Mishra S. K., Demain A. L. (1997). Effect of simulated microgravity and shear stress on microcin B17 production by Escherichia coli and on its excretion into the medium. Appl. Environ. Microbiol. 63 (10), 4090–4092. doi:10.1128/AEM.63.10.4090-4092.1997
Fernander M. C., Parsons P. K., Khaled B., Bradley A., Graves J. L., Thomas M. D. (2022). Adaptation to simulated microgravity in Streptococcus mutans. NPJ Microgravity 8 (1), 17. doi:10.1038/s41526-022-00205-8
Fernander M. C., Spurgeon K., Graves J., Guess W., Miller J., Mangum C., et al. (2023). Co-adaptation of Streptococcus mutans to simulated microgravity and silver nitrate. Front. Astronomy Space Sci. 10, 1183867. doi:10.3389/fspas.2023.1183867
Fernandes A., Oliveira A., Soares R., Barata P. (2023). The effects of ionizing radiation on gut microbiota: what can animal models tell us? A systematic review. Curr. Issues Mol. Biol. 45 (5), 3877–3910. doi:10.3390/cimb45050249
Fontana M., Zero D. T. (2006). Assessing patients’ caries risk. J. Am. Dent. Assoc. 137 (9), 1231–1239. doi:10.14219/jada.archive.2006.0380
Frippiat J. P., Crucian B. E., de Quervain D. J., Grimm D., Montano N., Praun S., et al. (2016). Towards human exploration of space: the THESEUS review series on immunology research priorities. NPJ Microgravity 2, 16040. doi:10.1038/npjmgrav.2016.40
Gangaiah D., Labandeira-Rey M., Zhang X., Fortney K. R., Ellinger S., Zwickl B., et al. (2014). Haemophilus ducreyi Hfq contributes to virulence gene regulation as cells enter stationary phase. mBio 5 (1), 010811–e1113. doi:10.1128/mBio.01081-13
Gatti M., Palumbo R., Di Domenico A., Mammarella N. (2022). Affective health and countermeasures in long-duration space exploration. Heliyon 8 (5), e09414. doi:10.1016/j.heliyon.2022.e09414
Gemignani A., Piarulli A., Menicucci D., Laurino M., Rota G., Mastorci F., et al. (2014). How stressful are 105 days of isolation? Sleep EEG patterns and tonic cortisol in healthy volunteers simulating manned flight to Mars. Int. J. Psychophysiol. 93 (2), 211–219. doi:10.1016/j.ijpsycho.2014.04.008
Ghosh P., Stabley J. N., Behnke B. J., Allen M. R., Delp M. D. (2016). Effects of space flight on the murine mandible: Possible factors mediating skeletal changes in non-weight bearing bones of the head. Bone 83, 156–161. doi:10.1016/j.bone.2015.11.001
Gilbert R., Torres M., Clemens R., Hateley S., Hosamani R., Wade W., et al. (2020). Space flight and simulated microgravity conditions increase virulence of Serratia marcescens in the Drosophila melanogaster infection model. NPJ Microgravity 6, 4. doi:10.1038/s41526-019-0091-2
Gill S. B., Valencia M. P., Sabino M. L., Heideman G. M., Michel M. A. (2009). Bisphosphonate-related osteonecrosis of the mandible and maxilla: clinical and imaging features. J. Comput. Assisted Tomogr. 33 (3), 449–454. doi:10.1097/RCT.0b013e318186b179
Goldberg M., Kellermann O., Dimitrova-Nakov S., Harichane Y., Baudry A. (2014). Comparative studies between mice molars and incisors are required to draw an overview of enamel structural complexity. Front. Physiology 5, 359. doi:10.3389/fphys.2014.00359
Gomes C., Martinho F. C., Barbosa D. S., Antunes L. S., Póvoa H. C. C., Baltus T. H. L., et al. (2018). Increased root canal endotoxin levels are associated with chronic apical periodontitis, increased oxidative and nitrosative stress, major depression, severity of depression, and a lowered quality of life. Mol. Neurobiol. 55 (4), 2814–2827. doi:10.1007/s12035-017-0545-z
Gontcharov I. B., Kovachevich I. V., Pool S. L., Navinkov O. L., Barratt M. R., Bogomolov V. V., et al. (2005). In-flight medical incidents in the NASA-Mir program. Aviat. Space, Environ. Med. 76 (7), 692–696.
Gottesman S., Storz G. (2011). Bacterial small RNA regulators: versatile roles and rapidly evolving variations. Cold Spring Harb. Perspect. Biol. 3 (12), a003798. Article a003798. doi:10.1101/cshperspect.a003798
Grigoriev A. I., Morukov B. V., Oganov V. S., Rakhmanov A. S., Buravkova L. B. (1992). Effect of exercise and bisphosphonate on mineral balance and bone density during 360 day antiorthostatic hypokinesia. J. Bone Mineral Res. 7 (Suppl. 2), S449–S455. doi:10.1002/jbmr.5650071416
Gross E. L., Leys E. J., Gasparovich S. R., Firestone N. D., Schwartzbaum J. A., Janies D. A., et al. (2010). Bacterial 16S sequence analysis of severe caries in young permanent teeth. J. Clin. Microbiol. 48 (11), 4121–4128. doi:10.1128/JCM.01232-10
Guadalupe-Grau A., Fuentes T., Guerra B., Calbet J. A. (2009). Exercise and bone mass in adults. Sports Med. 39 (6), 439–468. doi:10.2165/00007256200939060-00002
Hodkinson P. D., Anderton R. A., Posselt B. N., Fong K. J. (2017). An overview of space medicine. Br. J. Anaesth. 119 (Suppl. l_1), i143–i153. doi:10.1093/bja/aex336
Hooper L. V., Littman D. R., Macpherson A. J. (2012). Interactions between the microbiota and the immune system. Science 336 (6086), 1268–1273. doi:10.1126/science.1223490
Human Microbiome Project Consortium (2012). Structure, function and diversity of the healthy human microbiome. Nature 486 (7402), 207–214. doi:10.1038/nature11234
Ilyin V. K. (2005). Microbiological status of cosmonauts during orbital spaceflights on Salyut and Mir orbital stations. Acta Astronaut. 56, 839–850. doi:10.1016/j.actaastro.2005.01.009
Imura T., Otsuka T., Kawahara Y., Yuge L. (2019). Microgravity" as a unique and useful stem cell culture environment for cell-based therapy. Regen. Ther. 12, 2–5. doi:10.1016/j.reth.2019.03.001
Iordachescu A., Eisenstein N., Appleby-Thomas G. (2023). Space habitats for bioengineering and surgical repair: addressing the requirement for reconstructive and research tissues during deep-space missions. NPJ Microgravity 9 (1), 23. doi:10.1038/s41526-023-00266-3
Iwase S., Nishimura N., Tanaka K., Mano T. (2020). “Effects of microgravity on human physiology,” in Beyond LEO - human health Issues for deep space exploration (London, United Kingdom: InTech Open), 1–22.
Johnston S. L., Blue R. S., Jennings R. T., Tarver W. J., Gray G. W. (2014). Astronaut medical selection during the shuttle era: 1981-2011. Aviat. Space, Environ. Med. 85 (8), 823–827. doi:10.3357/ASEM.3968.2014
Juhl O. J., Buettmann E. G., Friedman M. A., DeNapoli R. C., Hoppock G. A., Donahue H. J. (2021). Update on the effects of microgravity on the musculoskeletal system. NPJ Microgravity 7 (1), 28. doi:10.1038/s41526-021-00158-4
Kim S., Hwang H., Kim K. P., Yoon H., Kang D. H., Ryu S. (2015). Hfq plays important roles in virulence and stress adaptation in Cronobacter sakazakii ATCC 29544. Infect. Immun. 83 (5), 2089–2098. doi:10.1128/IAI.03161-14
Kolenbrander P. E., Palmer R. J., Rickard A. H., Jakubovics N. S., Chalmers N. I., Diaz P. I. (2006). Bacterial interactions and successions during plaque development. Periodontology 42, 47–79. doi:10.1111/j.1600-0757.2006.00187.x
Kreth J., Zhang Y., Herzberg M. C. (2008). Streptococcal antagonism in oral biofilms: Streptococcus sanguinis and Streptococcus gordonii interference with Streptococcus mutans. J. Bacteriol. 190 (13), 4632–4640. doi:10.1128/JB.00276-08
Kreykes A. J., Suresh R., Levin D., Hilmers D. C. (2023). Selecting medical conditions relevant to exploration space flight to create the IMPACT 1.0 medical condition list. Aerosp. Med. Hum. Perform. 94 (7), 550–557. doi:10.3357/amhp.6199.2023
Kupper T., Hettlich M., Horz H. P., Lechner K., Scharfenberg C., Conrads G., et al. (2014). Dental problems and emergencies of trekkers—epidemiology and prevention. Results of the ADEMED expedition 2008. High Alt. Med. Biol. 15 (1), 39–45. doi:10.1089/ham.2013.1108
Leblanc A., Matsumoto T., Jones J., Shapiro J., Lang T., Shackelford L., et al. (2013). Bisphosphonates as a supplement to exercise to protect bone during long-duration space flight. Osteoporos. Int. 24 (7), 2105–2114. doi:10.1007/s00198-012-2243-z
LeBlanc A., Schneider V., Shackelford L., West S., Oganov V., Bakulin A., et al. (2000). Bone mineral and lean tissue loss after long duration space flight. J. Musculoskelet. Neuronal Interact. 1 (2), 157–160.
LeBlanc A. D., Driscol T. B., Shackelford L. C., Evans H. J., Rianon N. J., Smith S. M., et al. (2002). Alendronate as an effective countermeasure to disuse induced bone loss. J. Musculoskelet. Neuronal Interact. 2 (4), 335–343.
Lee C. A., Falkow S. (1990). The ability of Salmonella to enter mammalian cells is affected by bacterial growth state. Proc. Natl. Acad. Sci. U. S. A. 87 (11), 4304–4308. doi:10.1073/pnas.87.11.4304
Li Y., He L., Pan S., Zhang L., Zhang W., Yi H., et al. (2017). Three-dimensional simulated microgravity culture improves the proliferation and odontogenic differentiation of dental pulp stem cell in PLGA scaffolds implanted in mice. Mol. Med. Rep. 15 (2), 873–878. doi:10.3892/mmr.2016.6042
Li Y., Sui L., Zhao H., Zhang W., Gao L., Hu W., et al. (2022). Differences in the establishment of gut microbiota and metabolome characteristics between balb/c and C57bl/6J mice after proton irradiation. Front. Microbiol. 13, 874702. doi:10.3389/fmicb.2022.874702
Lloro V., Giovannoni L. M., Lozano-de Luaces V., Lloro I., Manzanares M. C. (2020). Is oral health affected in long period space missions only by microgravity? A systematic review. Acta Astronaut. 167, 343–350. doi:10.1016/j.actaastro.2019.11.015
Lloro V., Lozano-de Luaces V., Lloro I., Manzanares M. C. (2019). The incidence of dental needs during isolated missions compared to non-isolated missions: a systematic review and implications for future prevention strategies. Mil. Med. 184 (3-4), e148–e155. doi:10.1093/milmed/usy364
Low L. A., Giulianotti M. A. (2019). Tissue chips in space: modeling human diseases in microgravity. Pharm. Res. 37, 8. doi:10.1007/s11095-019-2742-0
Lu H., Zhao Q., Guo J., Zeng B., Yu X., Yu D., et al. (2019). Direct radiation-induced effects on dental hard tissue. Radiat. Oncol. 14 (1), 5. doi:10.1186/s13014-019-1208-1
Lynch S. V., Brodie E. L., Matin A. (2004). Role and regulation of sigma S in general resistance conferred by low-shear simulated microgravity in Escherichia coli. J. Bacteriol. 186 (24), 8207–8212. doi:10.1128/JB.186.24.8207-8212.2004
Lyons K. M., Rodda J. C., Hood J. A. (1997). The effect of environmental pressure changes during diving on the retentive strength of different luting agents for full cast crowns. J. Prosthet. Dent. 78 (5), 522–527. doi:10.1016/s0022-3913(97)70070-2
Marsh P. D. (1999). Microbiologic aspects of dental plaque and dental caries. Dent. Clin. N. Am. 43 (4), 599–614. doi:10.1016/s0011-8532(22)00816-3
Marteyn B., Scorza F. B., Sansonetti P. J., Tang C. (2011). Breathing life into pathogens: the influence of oxygen on bacterial virulence and host responses in the gastrointestinal tract. Cell. Microbiol. 13 (2), 171–176. doi:10.1111/j.1462-5822.2010.01549.x
Martineau A. R., Timms P. M., Bothamley G. H., Hanifa Y., Islam K., Claxton A. P., et al. (2011). High-dose vitamin D(3) during intensive-phase antimicrobial treatment of pulmonary tuberculosis: a double-blind randomised controlled trial. Lancet 377 (9761), 242–250. doi:10.1016/S0140-6736(10)61889-2
Mauclaire L., Egli M. (2010). Effect of simulated microgravity on growth and production of exopolymeric substances of Micrococcus luteus space and earth isolates. FEMS Immunol. Med. Microbiol. 59 (3), 350–356. doi:10.1111/j.1574-695X.2010.00683.x
Maupin K. A., Childress P., Brinker A., Khan F., Abeysekera I., Aguilar I. N., et al. (2019). Skeletal adaptations in young male mice after 4 weeks aboard the International Space Station. NPJ Microgravity 5, 21. doi:10.1038/s41526-019-0081-4
McLean R. J., Cassanto J. M., Barnes M. B., Koo J. H. (2001). Bacterial biofilm formation under microgravity conditions. FEMS Microbiol. Lett. 195 (2), 115–119. doi:10.1111/j.1574-6968.2001.tb10507.x
Mednieks M. I., Hand A. R. (2019). “Oral tissue responses to travel in space,” in Beyond LEO - human health issues for deep space exploration (London, United Kingdom: InTech Open), 1–26.
Menon A. (2012). Review of space flight dental emergencies (NASA/TM-2012-217368). Hanover, MD: NASA CASI.
Menon A., Barsten K., Watkins S. D. (2012). Summary report (NASA/TM-2012-217367). Hanover, MD: NASA CASI.
Mermel L. A. (2013). Infection prevention and control during prolonged human space travel. Clin. Infect. Dis. 56 (1), 123–130. doi:10.1093/cid/cis861
Miranda S., Vermeesen R., Radstake W. E., Parisi A., Ivanova A., Baatout S., et al. (2023). Lost in space? Unmasking the T Cell reaction to simulated space stressors. Int. J. Mol. Sci. 24, 16943. doi:10.3390/ijms242316943
Mitsuhara T., Takeda M., Yamaguchi S., Manabe T., Matsumoto M., Kawahara Y., et al. (2013). Simulated microgravity facilitates cell migration and neuroprotection after bone marrow stromal cell transplantation in spinal cord injury. Stem Cell Res. Ther. 4 (2), 35. doi:10.1186/scrt184
Moroni L., Tabury K., Stenuit H., Grimm D., Baatout S., Mironov V. (2022). What can biofabrication do for space and what can space do for biofabrication? Trends Biotechnol. 40 (4), 398–411. doi:10.1016/j.tibtech.2021.08.008
Moussa M. S., Goldsmith M., Komarova S. V. (2023). Craniofacial bones and teeth in spacefarers: systematic review and meta-analysis. JDR Clin. Transl. Res. 8 (2), 113–122. doi:10.1177/23800844221084985
Niazi S. A., Bakhsh A. (2022). Association between endodontic infection, its treatment and systemic health: a narrative review. Med. Kaunas. 58 (7), 931. doi:10.3390/medicina58070931
Nickerson C. A., Ott C. M., Mister S. J., Morrow B. J., Burns-Keliher L., Pierson D. L. (2000). Microgravity as a novel environmental signal affecting Salmonella enterica serovar Typhimurium virulence. Infect. Immun. 68 (6), 3147–3152. doi:10.1128/iai.68.6.3147-3152.2000
Nickerson C. A., Ott C. M., Wilson J. W., Ramamurthy R., Pierson D. L. (2004). Microbial responses to microgravity and other low-shear environments. Microbiol. Mol. Biol. Rev. 68 (2), 345–361. doi:10.1128/MMBR.68.2.345-361.2004
Oganov V. S., Rakhmanov A. S., Novikov V. E., Zatsepin S. T., Rodionova S. S., Cann C. (1991). The state of human bone tissue during space flight. Acta Astronaut. 23 (C), 129–133. doi:10.1016/0094-5765(91)90109-i
Oluwafemi F. A., Abdelbaki R., Lai J. C., Mora-Almanza J. G., Afolayan E. M. (2021). A review of astronaut mental health in manned missions: potential interventions for cognitive and mental health challenges. Life Sci. Space Res. (Amsterdam) 28, 26–31. doi:10.1016/j.lssr.2020.12.002
Omar O. M., Khattab N. M., Rashed L. A. (2012). Glucosyltransferase B, immunoglobulin A, and caries experience among a group of Egyptian preschool children. J. Dent. Child. Chic. 79 (2), 63–68.
Onorato G., Di Schiavi E., Di Cunto F. (2020). Understanding the effects of deep space radiation on nervous system: the role of genetically tractable experimental models. Front. Phys. 8, 362. doi:10.3389/fphy.2020.00362
Orsini S. S., Lewis A. M., Rice K. C. (2017). Investigation of simulated microgravity effects on Streptococcus mutans physiology and global gene expression. npj Microgravity 3, 4. doi:10.1038/s41526-016-0006-4
Orwoll E. S., Adler R. A., Amin S., Binkley N., Lewiecki E. M., Petak S. M., et al. (2013). Skeletal health in long-duration astronauts: nature, assessment, and management recommendations from the NASA bone summit. J. Bone Mineral Res. 28, 1243–1255. doi:10.1002/jbmr.1948
Otsuka T., Imura T., Nakagawa K., Shrestha L., Takahashi S., Kawahara Y., et al. (2018). Simulated microgravity culture enhances the neuroprotective effects of human cranial bone-derived mesenchymal stem cells in traumatic brain injury. Stem Cells Dev. 27, 1287–1297. doi:10.1089/scd.2017.0299
Page R. C. (1998). The pathobiology of periodontal diseases may affect systemic diseases: inversion of a paradigm. Ann. Periodontology 3 (1), 108–120. doi:10.1902/annals.1998.3.1.108
Palmer R. J., Diaz P. I., Kolenbrander P. E. (2006). Rapid succession within the Veillonella population of a developing human oral biofilm in situ. J. Bacteriol. 188 (11), 4117–4124. doi:10.1128/JB.01958-05
Pandith J. A., Neekhra S., Ahmad S., Sheikh R. A. (2023). Recent developments in space food for exploration missions: a review. Life Sci. Space Res. (Amsterdam) 36, 123–134. doi:10.1016/j.lssr.2022.09.007
Pandya M., Ma W., Lyu H., Luan X., Diekwisch T. G. H. (2021). Propagation of dental and respiratory cells and organs in microgravity. J. Vis. Exp. (171). Article e62690. doi:10.3791/62690
Pathak S. (2015). Aviation dentistry: past to present. J. Dent. Res. Rev. 2, 138–140. doi:10.4103/2348-2915.167877
Pathak Y. V., dos Santos M. A., Zea L. (2015) “Handbook of space pharmaceuticals,”. Switzerland: Springer Nature.
Pavy Le-Traon A., Roussel B. (1993). Sleep in space. Acta Astronaut. 29 (12), 945–950. doi:10.1016/0094-5765(93)90014-n
Philippou A., Minozzo F. C., Spinazzola J. M., Smith L. R., Lei H., Rassier D. E., et al. (2015). Masticatory muscles of mouse do not undergo atrophy in space. FASEB J. 29 (7), 2769–2779. doi:10.1096/fj.14-267336
Pietsch J., Gass S., Nebuloni S., Echegoyen D., Riwaldt S., Baake C., et al. (2017). Three-dimensional growth of human endothelial cells in an automated cell culture experiment container during the SpaceX CRS-8 ISS space mission - the SPHEROIDS project. Biomaterials 124, 126–156. doi:10.1016/j.biomaterials.2017.02.005
Planel H. (2004). Space and life: an introduction to space biology and medicine. Boca Raton: Taylor and Francis Group.
Radstake W. E., Baselet B., Baatout S., Verslegers M. (2022). Space flight stressors and skin health. Biomedicines 10 (2), 364. doi:10.3390/biomedicines10020364
Richards V. P., Alvarez A. J., Luce A. R., Bedenbaugh M., Mitchell M. L., Burne R. A., et al. (2017). Microbiomes of site-specific dental plaques from children with different caries status. Infect. Immun. 85 (8). Article e00106-17. doi:10.1128/IAI.00106-17
Rodan G. A., Fleisch H. A. (1996). Bisphosphonates: mechanisms of action. J. Clin. Investigation 97 (12), 2692–2696. doi:10.1172/JCI118722
Rosenberg G. D., Campbell S. C., Simmons D. J. (1984). The effects of space flight on the mineralization of rat incisor dentin. Proc. Soc. Exp. Biol. Med. 175 (4), 429–437. doi:10.3181/00379727-175-41816
Rossi E., Paroni M., Landini P. (2018). Biofilm and motility in response to environmental and host-related signals in Gram-negative opportunistic pathogens. J. Appl. Microbiol. 125, 1587–1602. Article 14089. doi:10.1111/jam.14089
Röstel L., Guo J., Banjac S., Wimmer-Schweingruber R. F., Heber B. (2020). Subsurface radiation environment of Mars and its implication for shielding protection of future habitats. J. Geophys. Res. Planets 125 (3). Article e2019JE006246. doi:10.1029/2019je006246
Ruggiero S. L., Mehrotra B., Rosenberg T. J., Engroff S. L. (2004). Osteonecrosis of the jaws associated with the use of bisphosphonates: a review of 63 cases. J. Oral Maxillofac. Surg. 62 (5), 527–534. doi:10.1016/j.joms.2004.02.004
Ruggiero S. L., Woo S. B. (2008). Biophosphonate-related osteonecrosis of the jaws. Dent. Clin. N. Am. 52 (1), 111–128. doi:10.1016/j.cden.2007.09.002
Runfeldt L. C., Gunga H. C., Steinach M. (2019). Anabolic signaling and response in sarcopenia as a model for microgravity induced muscle deconditioning: a systematic review. REACH 13, 10025. doi:10.1016/j.reach.2019.100025
Salavatifar M., Ahmadi S. M., Todorov S. D., Khosravi-Darani K., Tripathy A. (2023). Impact of microgravity on virulence, antibiotic resistance, and gene expression in beneficial and pathogenic microorganisms. Mini Rev. Med. Chem. 23 (16), 1608–1622. doi:10.2174/1389557523666230109160620
Sanz M., Ceriello A., Buysschaert M., Chapple I., Demmer R. T., Graziani F., et al. (2018). Scientific evidence on the links between periodontal diseases and diabetes: consensus report and guidelines of the joint workshop on periodontal diseases and diabetes by the International Diabetes Federation and the European Federation of Periodontology. J. Clin. Periodontology 45 (2), 138–149. doi:10.1111/jcpe.12808
Schachtele C., Nobbs A., Zhang Y., Costalonga M., Herzberg M. (2007). Oral streptococci: commensals and opportunistic pathogens. Horiz. Biosci., 411–462.
Senatore G., Mastroleo F., Leys N., Mauriello G. (2020). Growth of Lactobacillus reuteri DSM17938 under two simulated microgravity systems: changes in reuterin production, gastrointestinal passage resistance, and stress genes expression response. Astrobiology 20 (1), 1–14. doi:10.1089/ast.2019.2082
Sharma A., Clemens R. A., Garcia O., Taylor D. L., Wagner N. L., Shepard K. A., et al. (2022). Biomanufacturing in low Earth orbit for regenerative medicine. Stem Cell Rep. 17 (1), 1–13. doi:10.1016/j.stemcr.2021.12.001
Shearer W. T., Ochs H. D., Lee B. N., Cohen E. N., Reuben J. M., Cheng I., et al. (2009). Immune responses in adult female volunteers during the bed-rest model of space flight: antibodies and cytokines. J. Allergy Clin. Immunol. 123 (4), 900–905. doi:10.1016/j.jaci.2008.12.016
Shelhamer M., Bloomberg J., LeBlanc A., Prisk G. K., Sibonga J., Smith S. M., et al. (2020). Selected discoveries from human research in space that are relevant to human health on Earth. NPJ Microgravity 6, 5. doi:10.1038/s41526-020-0095-y
Shroff K. E., Meslin K., Cebra J. J. (1995). Commensal enteric bacteria engender a self-limiting humoral mucosal immune response while permanently colonizing the gut. Infect. Immun. 63 (10), 3904–3913. doi:10.1128/IAI.63.10.3904-3913.1995
Simmons D. J., Grynpas M. D., Rosenberg G. D. (1990). Maturation of bone and dentin matrices in rats flown on the Soviet biosatellite Cosmos 1887. FASEB J. 4 (1), 29–33. doi:10.1096/fasebj.4.1.2153084
Smith S. M., Heer M. (2002). Calcium and bone metabolism during space flight. Nutrition 18 (10), 849–852. doi:10.1016/s0899-9007(02)00895-x
Smith S. M., Wastney M. E., O'Brien K. O., Morukov B. V., Larina I. M., Abrams S. A., et al. (2005). Bone markers, calcium metabolism, and calcium kinetics during extended-duration space flight on the Mir space station. J. Bone Mineral Res. 20 (2), 208–218. doi:10.1359/JBMR.041105
Sonnenfeld G. (2005). The immune system in space, including earth-based benefits of space-based research. Curr. Pharm. Biotechnol. 6, 343–349. doi:10.2174/1389201054553699
Stavnichuk M., Mikolajewicz N., Corlett T., Morris M., Komarova S. V. (2020). A systematic review and meta-analysis of bone loss in space travelers. NPJ Microgravity 6, 13. doi:10.1038/s41526-020-0103-2
Sun H., Zhou Q., Qiao P., Zhu D., Xin B., Wu B., et al. (2022). Short-term head-down bed rest microgravity simulation alters salivary microbiome in young healthy men. Front. Microbiol. 13, 1056637. doi:10.3389/fmicb.2022.1056637
Szot P., Franklin A., Figlewicz D. P., Beuca T. P., Bullock K., Hansen K., et al. (2017). Multiple lipopolysaccharide (LPS) injections alter interleukin 6 (IL-6), IL-7, IL-10 and IL-6 and IL-7 receptor mRNA in CNS and spleen. Neuroscience 355, 9–21. doi:10.1016/j.neuroscience.2017.04.028
Takahashi N., Nyvad B. (2011). The role of bacteria in the caries process: ecological perspectives. J. Dent. Res. 90 (3), 294–303. doi:10.1177/0022034510379602
Tang H., Rising H. H., Majji M., Brown R. D. (2021). Long-term space nutrition: a scoping review. Nutrients 14 (1), 194. doi:10.3390/nu14010194
Taylor P. W. (2015). Impact of space flight on bacterial virulence and antibiotic susceptibility. Infect. Drug Resist. 8, 249–262. doi:10.2147/IDR.S67275
Tesei D., Jewczynko A., Lynch A. M., Urbaniak C. (2022). Understanding the complexities and changes of the astronaut microbiome for successful long-duration space missions. Life 12 (4), 495. doi:10.3390/life12040495
Thompson D. D., Seedor J. G., Weinreb M., Rosini S., Rodan G. A. (1990). Aminohydroxybutane bisphosphonate inhibits bone loss due to immobilization in rats. J. Bone Mineral Res. 5 (3), 279–286. doi:10.1002/jbmr.5650050312
Treffel L., Dmitrieva L., Gauquelin-Koch G., Custaud M. A., Blanc S., Gharib C., et al. (2016). Craniomandibular system and postural balance after 3-day dry immersion. PLoS ONE 11 (2), e0150052. doi:10.1371/journal.pone.0150052
Urbaniak C., Lorenzi H., Thissen J., Jaing C., Crucian B., Sams C., et al. (2020). The influence of space flight on the astronaut salivary microbiome and the search for a microbiome biomarker for viral reactivation. Microbiome 8 (1), 56. doi:10.1186/s40168-020-00830-z
Valentin-Hansen P., Eriksen M., Udesen C. (2004). The bacterial Sm-like protein Hfq: a key player in RNA transactions. Mol. Microbiol. 51 (6), 1525–1533. doi:10.1111/j.1365-2958.2003.03935.x
Vico L., Collet P., Guignandon A., Lafage-Proust M. H., Thomas T., Rehaillia M., et al. (2000). Effects of long-term microgravity exposure on cancellous and cortical weight-bearing bones of cosmonauts. Lancet 355 (9215), 1607–1611. doi:10.1016/s0140-6736(00)02217-0
Vogel J., Luisi B. F. (2011). Hfq and its constellation of RNA. Nat. Rev. Microbiol. 9 (8), 578–589. doi:10.1038/nrmicro2615
Wang C., Pu T., Lou W., Wang Y., Gao Z., Hu B., et al. (2018). Hfq, a RNA chaperone, contributes to virulence by regulating plant cell wall-degrading enzyme production, type VI secretion system expression, bacterial competition, and suppressing host defense response in Pectobacterium carotovorum. Mol. Plant-Microbe Interact. 31 (11), 1166–1178. doi:10.1094/MPMI-12-17-0303-R
Watanabe Y., Ohshima H., Mizuno K., Sekiguchi C., Fukunaga M., Kohri K., et al. (2004). Intravenous pamidronate prevents femoral bone loss and renal stone formation during 90-day bed rest. J. Bone Mineral Res. 19 (11), 1771–1778. doi:10.1359/JBMR.040811
Watkins S., Barr Y., Kerstman E. (2011). The university of Texas medical branch, N.J.S.C.B. Contract. The Space Medicine Exploration Medical Condition List.
Wilson J. W., Ott C. M., Höner zu Bentrup K., Ramamurthy R., Quick L., Porwollik S., et al. (2007). Space flight alters bacterial gene expression and virulence and reveals a role for global regulator Hfq. Proc. Natl. Acad. Sci. U. S. A. 104 (41), 16299–16304. doi:10.1073/pnas.0707155104
Woo S. B., Hande K., Richardson P. G. (2005). Osteonecrosis of the jaw and bisphosphonates. N. Engl. J. Med. 353 (1), 99–102.
Wu B., Wang Y., Wu X., Liu D., Xu D., Wang F. (2018). On-orbit sleep problems of astronauts and countermeasures. Mil. Med. Res. 5 (1), 17. doi:10.1186/s40779-018-0165-6
Yatagai F., Honma M., Dohmae N., Ishioka N. (2019). Biological effects of space environmental factors: a possible interaction between space radiation and microgravity. Life Sci. Space Res. 20, 113–123. doi:10.1016/j.lssr.2018.10.004
Yuge L., Sasaki A., Kawahara Y., Wu S. L., Matsumoto M., Manabe T., et al. (2011). Simulated microgravity maintains the undifferentiated state and enhances the neural repair potential of bone marrow stromal cells. Stem Cells Dev. 20 (5), 893–900. doi:10.1089/scd.2010.0294
Keywords: bone density, oral microbiology, dental care, microgravity, space travel, Streptococcus mutants, xerostomia
Citation: Bakr MM, Caswell GM, Hussein H, Shamel M and Al-Ankily MM (2024) Considerations for oral and dental tissues in holistic care during long-haul space flights. Front. Physiol. 15:1406631. doi: 10.3389/fphys.2024.1406631
Received: 16 April 2024; Accepted: 12 June 2024;
Published: 11 July 2024.
Edited by:
Jack J. W. A. van Loon, VU Amsterdam, NetherlandsReviewed by:
Felice Strollo, IRCCS San Raffaele Pisana, ItalyRonan Padraic Murphy, Dublin City University, Ireland
Copyright © 2024 Bakr, Caswell, Hussein, Shamel and Al-Ankily. This is an open-access article distributed under the terms of the Creative Commons Attribution License (CC BY). The use, distribution or reproduction in other forums is permitted, provided the original author(s) and the copyright owner(s) are credited and that the original publication in this journal is cited, in accordance with accepted academic practice. No use, distribution or reproduction is permitted which does not comply with these terms.
*Correspondence: Gabrielle M. Caswell, ZHJjYXN3ZWxsQHNwYWNlcG9ydGF1c3RyYWxpYS5jb20uYXUmI3gwMjAwYTs=