- 1Department of Cellular and Integrative Physiology, University of Nebraska Medical Center, Omaha, NE, United States
- 2Department of Anesthesiology, University of Nebraska Medical Center, Omaha, NE, United States
Introduction: Sex-specific patterns in respiratory conditions, such as asthma, COPD, cystic fibrosis, obstructive sleep apnea, and idiopathic pulmonary fibrosis, have been previously documented. Animal models of acute lung injury (ALI) have offered insights into sex differences, with male mice exhibiting distinct lung edema and vascular leakage compared to female mice. Our lab has provided evidence that the chemoreflex is sensitized in male rats during the recovery from bleomycin-induced ALI, but whether sex-based chemoreflex changes occur post-ALI is not known. To bridge this gap, the current study employed the bleomycin-induced ALI animal model to investigate sex-based differences in chemoreflex activation during the recovery from ALI.
Methods: ALI was induced using a single intra-tracheal instillation of bleomycin (bleo, 2.5 mg/Kg) (day 1). Resting respiratory frequency (fR) was measured at 1-2 days pre-bleo, day 7 (D7) post-bleo, and 1 month (1 mth) post-bleo. The chemoreflex responses to hypoxia (10% O2, 0% CO2) and normoxic-hypercapnia (21% O2, 5% CO2) were measured before bleo administration (pre-bleo) and 1 mth post-bleo using whole-body plethysmography. The apnea-hypopnea Index (AHI), post-sigh apneas, and sighs were measured at each time point.
Results: There were no significant differences in resting fR between male and female rats at the pre-bleo time point or in the increase in resting fR at D7 post-bleo. At 1 mth post-bleo, the resting fR was partially restored in both sexes but the recovery towards normal ranges of resting fR was significantly lower in male rats. The AHI, post-sigh apneas, and sighs were not different between male and female rats pre-bleo and 1 mth post-bleo. However, at D7 post-bleo, the male rats exhibited a higher AHI than female rats. Both male and female rats exhibited a sensitized chemoreflex in response to hypoxia and normoxic-hypercapnia with no significant differences between sexes.
Conclusion: A sex difference in resting ventilatory parameters occurs post ALI with a prolonged increase in resting fR and larger AHI in male rats. On the other hand, we did not find any sex differences in the chemoreflex sensitization that occurs at 1 mth post-bleo. This work contributes to a better understanding of sex-based variations in lung disorders.
1 Introduction
In studies involving vertebrate animals and humans, the consideration of “sex” as a critical biological variable is essential throughout the design, analysis, and reporting of research (Lee, 2018). Sex-related differences in lung development are apparent throughout life, affected by factors like variations in lung growth and maturity, as well as the influence of sex hormones (Carey et al., 2007; Silveyra et al., 2021). Pulmonary diseases such as respiratory distress syndrome, bronchiolitis, pneumonia, chronic obstructive pulmonary disease (COPD), obstructive sleep apnea (OSA), and the SARS-CoV-2 epidemic have reported sex differences (Silveyra et al., 2021).
Acute lung injury (ALI) and its severe form, acute respiratory distress syndrome (ARDS) caused by the disruption of the normal capillary endothelial barrier leads to impacting ventilatory control (Young et al., 2019). Acute respiratory failure, responsible for 10% of ICU admissions with significant mortality and morbidity, affects approximately 200,000 new cases annually in the US alone (Mowery et al., 2020). Epidemiological research on sex differences in all-cause ARDS presents contradictory findings, with some studies indicating a substantial correlation between ARDS incidence, mortality, and sex (Moss and Mannino, 2002; Agarwal et al., 2006; Phua et al., 2009; Lemos-Filho et al., 2013; Chen et al., 2015). Structural and functional variations in lung and airway development, influenced by genetic, epigenetic, hormonal, and environmental factors, contribute to these disparities (Silveyra et al., 2021). Notably, pre-term birth in males is associated with a greater disadvantage (Bancalari and Jain, 2019). Sex hormones, particularly testosterone and estrogen, play a role in influencing immune-related cells, macrophage polarization, airway smooth muscle cells, and inflammation (Becerra-Díaz et al., 2018). Clinical studies show that male infants are more susceptible to lower respiratory tract infections, bronchiolitis, respiratory distress syndrome, and bronchopulmonary dysplasia (Liptzin et al., 2015). In contrast, male children are more prone to asthma, while women exhibit a higher exacerbation risk in chronic obstructive pulmonary disease (COPD) and a lower likelihood of obstructive sleep apnea (OSA) compared to men (Han et al., 2018). Sex differences have also been studied in different models of ALI by several groups. Most importantly, all those studies focused on lung pathology post-ALI. A potential sex difference in the neural control of breathing post-ALI has not been investigated.
Carotid bodies (CBs), peripheral chemoreceptors found at the common carotid artery bifurcation, are stimulated by hypoxemia brought on by ALI. As a basic defensive response to return blood gas concentrations to normal, these chemoreceptors detect changes in pH and blood gas levels. The enhancement of sympathetic drive by acute or chronic CB activation is a well-established phenomenon. Excessive sympathetic output can cause cardiac arrhythmias, cardio-renal syndrome, metabolic syndrome, Type 2 diabetes, and impaired cardiac activity (Mark, 1995; Marcus et al., 2014; Moreira et al., 2015; Li, 2022). Previously, our laboratory has provided evidence of increased chemosensitivity during recovery from ALI in male rats (Kamra et al., 2022). Nevertheless, there remains a knowledge gap regarding the comparisons of male vs. female chemoreflex changes during the recovery from ALI. The current study utilizes a bleomycin-induced ALI animal model to investigate sex-based differences in chemoreflex activation under ALI conditions.
2 Methods
2.1 Ethical approval
Animals were housed in a temperature-controlled environment (22°C–25°C) with a 12 h light-dark cycle and ad libitum access to food and water, by standards set by the National Institutes of Health Guidelines for the Care and Use of Laboratory Animals. All experimental protocols were approved by the Institutional Animal Care and Use Committee (IACUC) of the University of Nebraska Medical Center (protocol ID no. 17-006-03 FC).
2.2 Animals
Eighteen adult (eight male and ten female) Sprague-Dawley rats (2 - 3 months old) were used for these experiments. They were allowed to acclimate for 3 days to their new environment before the experiment. All animal experimentation (collection of ventilatory parameters during rest and hypoxic/hypercapnic gas exposure) was performed during the day (9:00–16:00 h). Delivery of bleomycin sulfate (bleo) was performed within the Department of Comparative Medicine. At the end of the experimental protocol, all animals were humanely euthanized with an overdose of pentobarbital sodium (150 mg/kg, IV). Euthanasia was confirmed by the removal of vital organs. An experimental timeline is shown in Figure 1.
2.3 Drugs and chemicals
Bleo was purchased from Enzo Life Sciences (New York, United States). Bleo was dissolved in saline for intra-tracheal administration. This procedure was performed within the Department of Comparative Medicine.
2.4 Rat model of lung injury
Rats were randomized into two experimental groups and evaluated at three time points- 1-2 days before instillation (pre-bleo), day 7 (D7) post-bleo, and 1 month (1 mth) post-bleo instillation as follows: male bleo-treated rats (n = 8) and female bleo-treated rats (n = 10). Bleo (2.5 mg/kg) was instilled on day 0 intratracheally under 2% - 3% isoflurane anesthesia.
2.5 Breathing and ventilatory chemoreflex function
Unrestrained whole-body plethysmography was utilized to measure ventilatory parameters-respiratory rate (fR), tidal volume (Vt) and minute ventilation (V̇E) in conscious rats by using signals from a differential-pressure transducer (DLP 2.5, Harvard Apparatus), amplified, and connected to a PC via an acquisition system (PowerLab 35 Series) managed by LabChart (v8.1.5) software (ADInstruments, Colorado Springs, United States). Rats were acclimated to the plethysmograph chamber for 1 h each for two consecutive days before recordings. Respiratory parameters were not recorded during the acclimatization sessions. The plethysmography chambers used for this study were custom-made (Midwest Plastics Inc., Nebraska, United States) and were 10, 10.5, and 20 cm in height, width, and length, respectively. The volume channel (i.e., flow integration) was calibrated by pushing 5 mL of air using a syringe before the start of the recording. During recordings, a constant flow of gas at 3 L/min was maintained to avoid an increase in humidity, temperature, and CO2 levels using a manually operated flow meter (Precision Medical, Northampton, PA, United States). The body weight (in grams) of rats was recorded before each experiment. In the resting state, rats were exposed to normoxia (21% O2, 0% CO2) for baseline measurements followed by two different gas challenges-hypoxia (10% O2, 0% CO2) and normoxic hypercapnia (21% O2, 5% CO2) balanced by N2. The order of the gas challenge was randomized and was maintained for 5 minutes. The last 1-min-long segment without any artifacts was used for analysis. A normoxic exposure of a minimum of 10 min or more was used in between challenges. All resting ventilatory parameters considered for analysis were recorded when the rats were awake and stationary (no activity-related events were included in the analysis in the LabChart8 raw data file). V̇E was calculated as the product of fR and Vt. Vt and V̇E were normalized to body weight. All data values were extracted from LabChart8 raw data files. A 30-min recording without artifacts was recorded for all rats while they breathed room air. This was used to measure all resting ventilatory parameters and to manually extract apneas, hypopneas, sighs, and post-sigh apneas from LabChart 8 raw data files. Apneas were defined as the cessation of breathing for at least three respiratory cycles, as determined by the respiratory rate for the prior 10 s; hypopneas were defined as reductions in breath amplitude 50% of the average cycle amplitude of the preceding 10 s of regular breathing; post-sigh apneas were defined as the cessation of breathing for at least three respiratory cycles immediately after a sigh. Apneas and hypopneas were expressed as Apnea-Hypopnea Index (AHI, events/hour). Sighs and post-sigh apneas were also expressed as events/hour.
2.6 Statistical analysis
Data analysis in text, tables, and figures are presented as mean ± SD. Statistical evaluation was analyzed using GraphPad Prism (GraphPad Software, San Diego, CA. Version 8). For the chemoreflex comparisons, two-way ANOVA with Bonferroni corrections (male vs. Female and Pre-Bleo vs. Post-Bleo) was used with p ≤ 0.05 being statistically significant. Body weight and baseline respiratory parameters at multiple time points post-Bleo were compared by using two-way Repeated Measurement (RM) ANOVA with p ≤ 0.05 being statistically significant.
3 Results
3.1 Effect of bleomycin on body weight in male and female rats post-ALI
Body weights were measured in all rats at pre-bleo administration and post-bleo administration at D7 and 1 mth. Body weight did not change in either male or female rates during the first 7 days post-bleo (Table 1). At 1-mth post-bleo, male and female rats significantly increased body weight by 99 ± 74 g (p < 0.0001) and 56 ± 24 g, p < 0.0001), respectively (Table 1).

Table 1. Mean body weight and mean change in body weight (in grams) for male and female dose bleo rats.
3.2 Sex-based differences in baseline respiratory parameters between male and female rats post-ALI
As noted in Figures 2A, C, E, G, male and female rats showed a steady normal resting fR pattern pre-bleo with average fR similar between groups (male rats = 95 ± 15 bpm; female rats = 86 ± 11 bpm (Table 2). However, as expected, consistent with our previously published data, both male (280 ± 70 bpm, p < 0.0001) and female (240 ± 43 bpm, p < 0.0001) rats exhibited a significant increase in resting fR post-bleo treatment at D7 after receiving bleo compared to the pre-bleo time point (Table 2). This increase in fR was partially restored at 1 mth post-bleo treatment in both groups (male = 141 ± 44 bpm (p = 0.0004, D7 vs. 1 mth post-bleo) and female = 104 ± 23 bpm (p < 0.0001, D7 vs. 1 mth post-bleo) (Table 2; Figures 2B, D, F, H). The comparison of fR between male and female rats at 1 mth post-bleo time point was significantly different (p = 0.03) with male rats having a higher resting fR than female rats (male vs. female: 141 ± 44 bpm vs. 104 ± 23 bpm). In male rats, the Vt and VE were significant differences in D7 post-bleo when compared to pre-bleo time points. The female group also showed no significant differences in Vt, however, VE was significantly increased at D7 post-bleo and 1 mth-post-bleo when compared to pre-bleo (p < 0.0001, female rats: pre-bleo vs. D7 post-bleo).
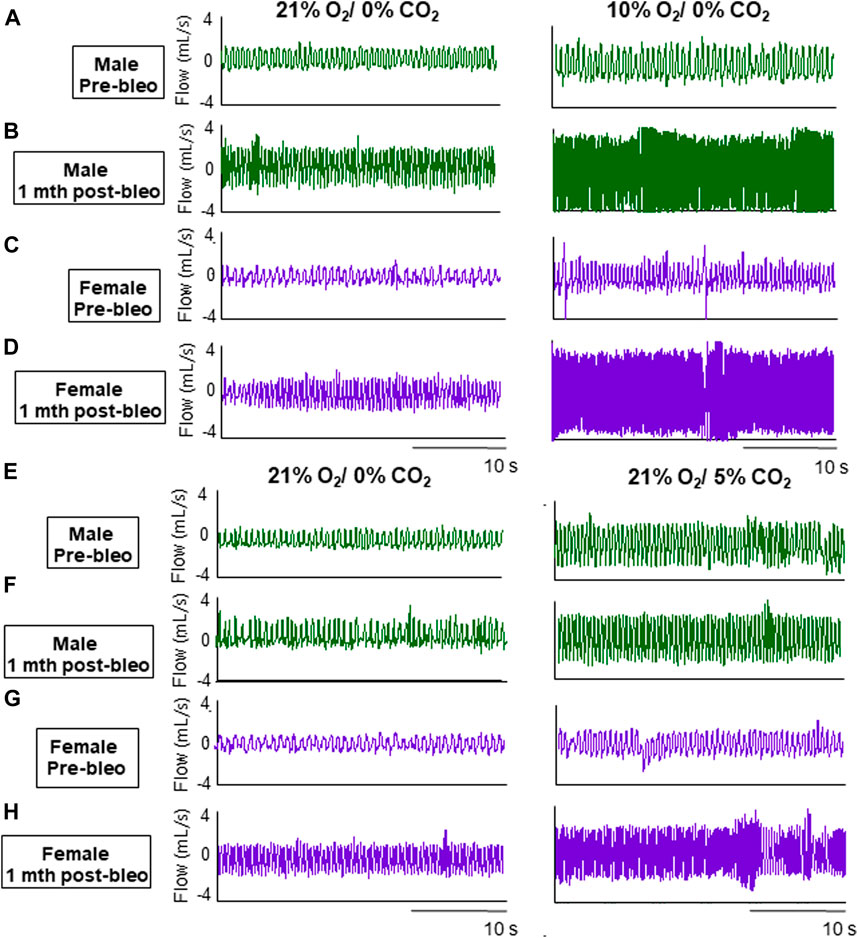
Figure 2. Representative ventilator tracings at normoxia, 10% hypoxia, and 5% normoxic-hypercapnia were obtained in a male and a female rat.
3.3 Sex-based differences in the occurrence of apneic events between male and female rats post ALI
The 30-minute-raw data recorded during normoxic gas exposure were utilized to manually extract apneas from male and female rats at three time points-pre-bleo, D7 post-bleo, and 1 mth post-bleo. Male and female rats showed a significant difference (p = 0.02) in the occurrence of apneas throughout the experimental timeline. The changes pre- and post-bleo were also seen to be significantly different (p = 0.002) (Figure 3A).
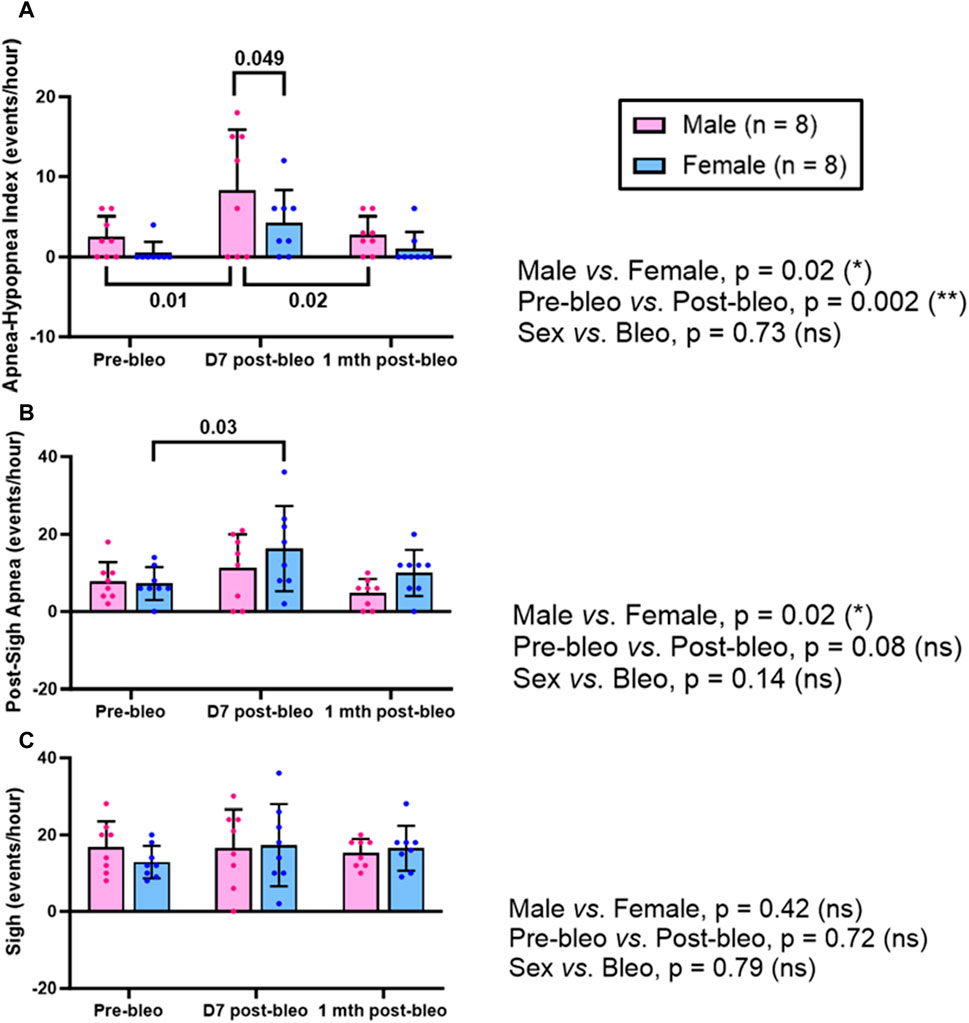
Figure 3. Effects of sex, bleomycin (pre-vs. post-bleo) and the interaction between the sex and treatment for (A) Apnea-hypopnea index (AHI), (B) Post-sigh apnea occurrence, and (C) Sigh occurrence in male (n = 8) and female (n = 8) rats. Two-way ANOVA, repeated measures, Values are mean ± SD.
The post-sigh apneas were significantly different between male vs. female rats (p = 0.02). These differences however were not seen to be affected by bleo treatment (pre-vs. post-bleo, p = 0.08) (Figure 3B). The occurrence of sighs did not change significantly at all three time points for either sex and for pre-vs. post-bleo, revealing no sex differences for sighs during the acute and recovery phase of ALI (Figure 3C).
3.4 Sex-based differences in the chemoreflex sensitivity between male and female rats during the recovery from ALI
The chemoreflex responses to 10% hypoxia and 5% normoxic-hypercapnia were assessed by measuring the absolute difference between 21% O2/0% CO2 and 10% O2/0% CO2 or 21% O2/5% CO2-induced responses. At pre-bleo, both male and female rats exhibited similar increases in fR and VE in response to 10% hypoxia (Figures 4A, C, E). At 1 mth post-bleo, there was a significant increase in chemosensitivity (Figures 4B, F) with no differences in chemoreflex activation between male vs. female groups of rats (Figures 4B, F). The changes in Vt in response to 10% hypoxia were not statistically significant for either male vs. female groups of rats (Figures 4C, D).
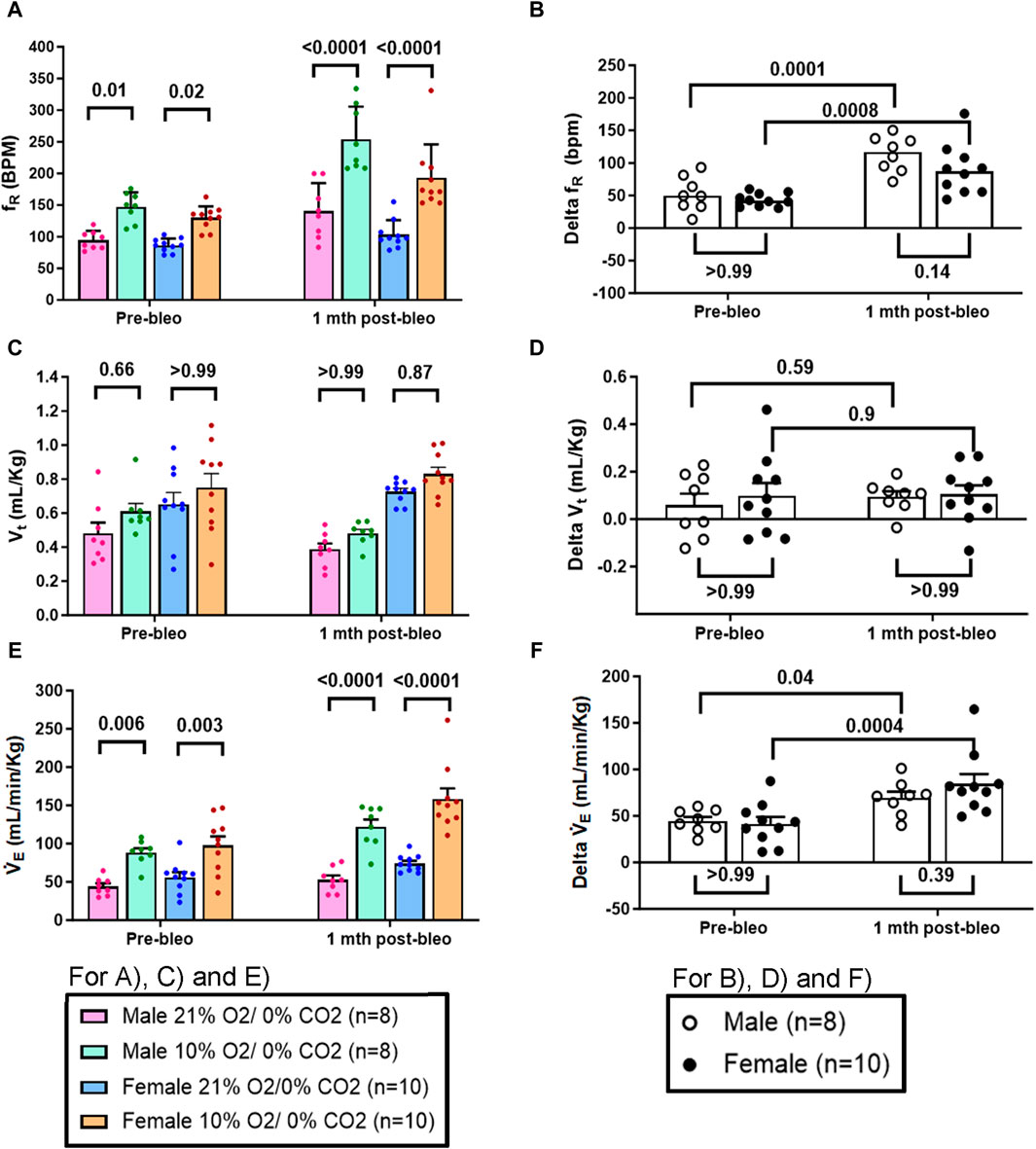
Figure 4. Effect of bleomycin on ventilatory parameters in male (n = 8) and female (n = 10) rats in response to chemoreflex activation with 10% O2/0% CO2. Two-way ANOVA, Values are mean ± SD: (A) Respiratory rate (fR) at normoxia vs. hypoxia; (B) Delta fR (Delta is the absolute difference between the response at normoxia and hypoxia); (C) Tidal volume (Vt) at normoxia vs. hypoxia; (D) Delta Vt; (E) Minute ventilation (V̇E) at normoxia vs. hypoxia; (F) Delta V̇E.
Similarly, in response to 5% normoxic-hypercapnia, both male and female rats showed similar changes in fR and VE at pre-bleo time points (Figures 5A, E). Like 10% hypoxia, both groups showed a significant increase in chemoreflex activation at 1 mth post-bleo with no difference between sexes (Figures 5B, F). The changes in Vt in response to 5% normoxic-hypercapnia were not statistically significant for either male vs. female groups of rats (Figures 5C, D).
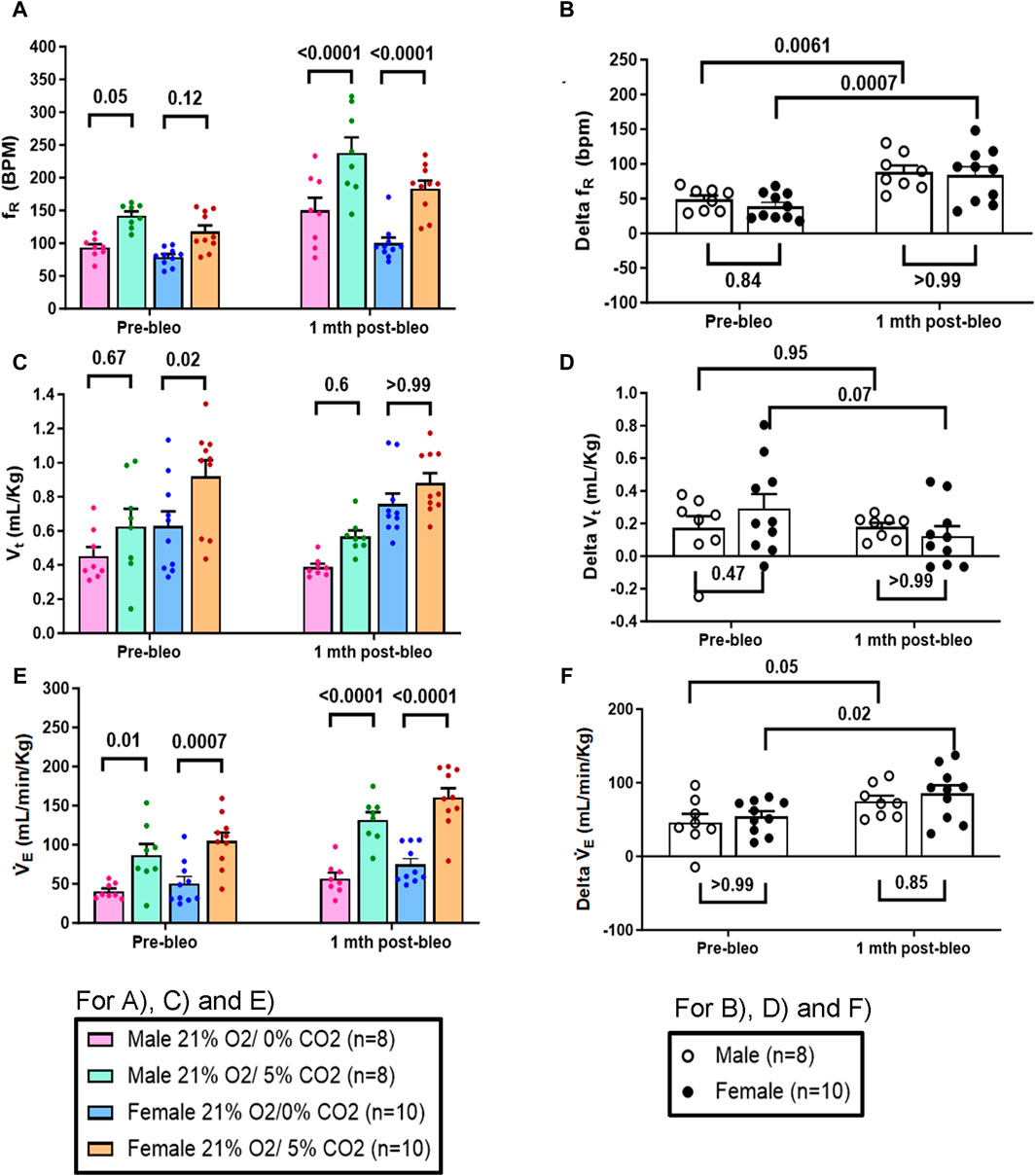
Figure 5. Effect of bleomycin on ventilatory parameters in male (n = 8) and female (n = 10) rats in response to 21% O2/5% CO2. Two-way ANOVA, Values are mean ± SD: (A) Respiratory rate (fR) at normoxia vs. normoxic-hypercapnia; (B) Delta fR (Delta is the absolute difference between the response at normoxia and normoxic-hypercapnia); (C) Tidal volume (Vt) at normoxia vs. normoxic-hypercapnia; (D) Delta Vt; (E) Minute ventilation (V̇E) at normoxia vs. normoxic-hypercapnia; (F) Delta V̇E.
4 Discussion
In this study, we examined the resting breathing parameters (fR, AHI, sighs, post sigh apneas) during both the acute phase (D7) and recovery phase (1 mth) of ALI, and the chemoreflex sensitization during the recovery from ALI in male and female rats. The key findings of this study are summarized as follows: 1) There were no significant differences in the resting breathing parameters (fR, AHI, sighs, post sigh apneas) between male and female rats. At the D7 post-bleo, resting fR was significantly increased in both male and female rats but tended to increase by a larger degree in male rats. At 1 mth post-bleo, the resting fR was partially restored in both sexes but the recovery towards normal ranges of resting fR was significantly lower in male rats. At D7 post-bleo, the males exhibited significantly more events/hour of AHI compared to females. 2) At the pre-bleo time point, both male and female groups showed similar chemoreflex responses in fR to 10% hypoxia and 5% normoxic-hypercapnia. During recovery from ALI at 1 mth post-bleo, the chemoreflex sensitivity in response to hypoxia and normoxic-hypercapnia did not exhibit sex-based differences.
Animal models, such as the two-hit model involving acid instillation followed by overventilation, have provided histological evidence of sex differences in ALI, with male mice exhibiting distinct lung edema and vascular leakage compared to female mice (Erfinanda et al., 2021). Another study observed higher levels of airway inflammation and hyperresponsiveness in male mice (Carey et al., 2007). Lingappan et al. investigated sex-specific differences in lung injury induced by hyperoxic exposure in neonate mice (Lingappan et al., 2016). They found that male neonate mice exhibited a greater cytokine response and pulmonary angiogenesis arrest compared to females (Lingappan et al., 2016). They observed similar patterns in adult male mice compared to females (Lingappan et al., 2016). On the other hand, several other studies from LPS-induced ALI models showed no significant sex difference in lung pathology. For instance, Nguyen et al. reported no differences in pulmonary injury and abnormal lung development between male and female mice exposed to early postnatal systemic LPS challenge (Nguyen et al., 2019). They also reported findings that suggest a similar innate response to early neonatal LPS exposure and the resulting pulmonary sequelae in male and female mice (Nguyen et al., 2019). Another study demonstrated no sex-based differences in LPS-induced TLR4 expression in the adult rat lung (Du et al., 2005).
A previous study from our lab using a bleo-induced ALI adult rat model demonstrated a significant increase in resting fR in male ALI rats at D7 post-bleo administration (Kamra et al., 2022). The present study demonstrated no significant difference in this increase in resting fR at D7 post-bleo between male and female rats. However, a trend of comparatively higher resting fR was seen in adult male ALI rats at D7 post-bleo compared to female ALI rats. More importantly, the recovery from increased resting fR in male rats at 1 mth post-bleo was significantly slower than in female rats. This may be due to the protective effects of female sex hormones (Speyer et al., 2005; Carey et al., 2007; Erfinanda et al., 2021). It is known that female neonate mice demonstrated a better antioxidant defense mechanism against hyperoxia-induced reactive oxygen species (ROS) (Vento et al., 2009; Tondreau et al., 2012). Estrogen’s antioxidant, vasodilatory, and anti-inflammatory properties contribute to a lower risk of cardiovascular diseases in females (Xiang et al., 2021).
In addition, we also documented a significant increase in the occurrence of apneas and hypopneas in male ALI rats at the D7 post-bleo time point. This increase was significantly increased in male rats at this time point compared to female bleo rats that also exhibited a trend towards higher apneic incidences. The post-sigh apneas were significantly higher at D7 post-bleo in female rats while the male rats exhibited a trend to show an increase. Consistent with our AHI data, clinical evidence also suggests that obstructive sleep apnea is documented to be higher in males than in females (Lin et al., 2008). In our study, we see this significant increase in apneic events at the D7 post-bleo time point as lung injury is at its peak during this period, after the first and only insult of bleo (Kamra et al., 2022). It is known that the occurrence of apneas and hypopneas are among many factors associated with increased sympathetic nerve activity, inflammation, and intermittent hypoxia, and may be caused by respiratory control instability (Javaheri et al., 2017).
Previously, we reported evidence of enhanced chemoreflex response to hypoxia and normoxic-hypercapnia in the recovery phase (1 mth post-bleo) in male ALI rats (Kamra et al., 2022). However, a potential sex difference in chemoreflex sensitivity during the recovery of ALI was not examined. The data from the present study in male and female rats corroborate the findings from our previous study in male ALI rats of chemoreflex sensitization with recovery from ALI. We now show no statistically significant differences in chemoreflex sensitization in male vs. female rats during the recovery of ALI.
Supportive evidence of no sex differences in chemosensitivity was presented by Usselman et al. who examined the effect of sex on chemoreflex regulation of muscle sympathetic nerve activity in young healthy men and women who were not using any form of hormonal contraception (Usselman et al., 2015). They observed similar sympathetic responses to chemoreflex activation in men and women (Usselman et al., 2015). Interestingly, in rats exposed to CIH, another study showed there are sex differences in respiratory-related sympathetic nerve discharge that characterize differences in the respiratory modulation of sympathetic activity after CIH (Souza et al., 2018).
Independent of sex hormones, these data in juvenile rats (Souza et al., 2018) demonstrate sexual dimorphism in the reconfiguration of respiratory and pre-sympathetic network interactions after CIH (Souza et al., 2018). It is interesting to note that in the current study, at 1 mth post-bleo, the recovery of resting fR was significantly faster in female bleo rats than in male bleo rats. The generation of resting fR is controlled both by intrinsic respiratory drive and thoracic neural receptors. It is possible that in the case of female bleo-rats, the female sex hormone exhibited protective effects in the reflexes controlling the resting fR generation (Behan et al., 2003; Zhang et al., 2020). However, at the same time point (1 mth post-bleo), we note that the chemoreflex activation in response to both hypoxia and normoxic-hypercapnia was not different between the sexes. The peripheral and central chemoreceptors contribute to the integrated receptor input in the pons and medulla to modify the generation of rhythmic breathing during non-normoxic conditions. The effect of sex hormones on these chemoreceptors and whether estrogen has any protective effects on the respiratory rhythm generator is not well understood. It is noteworthy that sex-based differences in chemoreflex were not measured at the D7 post-bleo timepoint in the current study. According to our earlier research, male rats with moderate ALI tend to exhibit a significantly blunted chemoreflex response to hypoxia or normoxic-hypercapnia at this timepoint, whereas those with severe ALI generally have a more sensitive chemoreflex response (Kamra et al., 2022). The reasons for this disparity in chemoreflex sensitivity between male rats with moderate and severe ALI at D7—blunted versus sensitized—remain unclear. However, we observed that during the ALI recovery period, or 1-month post-ALI, both moderate and severe ALI rats developed a sensitized chemoreflex. Given that both moderate and severe ALI rats showed consistent chemoreflex sensitization at this chronic time window, we chose to focus on the recovery period in the current study due to the complex changes in chemoreflex sensitivity between these groups at the D7 post-ALI timepoint.
5 Conclusion
In conclusion, our study highlighted noteworthy sex differences in terms of resting fR, with a pronounced increase observed in males at the 1 mth post-bleo time point. Moreover, sex-specific variations were identified in acute AHI (at the D7 post-bleo), emphasizing the importance of sex as a determinant in respiratory responses. Interestingly, the chemoreflex response exhibited consistency across sexes during both pre-bleo and recovery from ALI (1-mth post-bleo). These findings point to a need for a broader understanding of sex-based variations in lung disorders and underscore the importance of considering sex as a crucial factor in respiratory research. Further exploration of these mechanisms is warranted to advance our knowledge and refine therapeutic strategies tailored to the unique aspects of male and female respiratory physiology.
Data availability statement
The raw data supporting the conclusions of this article will be made available by the authors, without undue reservation.
Ethics statement
The animal study was approved by the Institutional Animal Care and Use Committee (IACUC) of the University of Nebraska Medical Center. The study was conducted in accordance with the local legislation and institutional requirements.
Author contributions
KK: Data curation, Investigation, Methodology, Project administration, Validation, Writing–original draft, Writing–review and editing. IZ: Conceptualization, Methodology, Supervision, Writing–review and editing. HS: Conceptualization, Methodology, Supervision, Writing–review and editing. H-JW: Conceptualization, Funding acquisition, Investigation, Methodology, Project administration, Resources, Supervision, Visualization, Writing–review and editing.
Funding
The author(s) declare that financial support was received for the research, authorship, and/or publication of this article. This study was supported by NIH grant R01 HL-152160-01 and in part, by NIH grants R01 HL171602-01, HL-169205-01 and R21 HL170127-01. HW is also supported by Margaret R. Larson Professorship in Anesthesiology. IZ is supported, in part, by the Theodore F. Hubbard Professorship for Cardiovascular Research.
Conflict of interest
The authors declare that the research was conducted in the absence of any commercial or financial relationships that could be construed as a potential conflict of interest.
The author(s) declared that they were an editorial board member of Frontiers, at the time of submission. This had no impact on the peer review process and the final decision.
Publisher’s note
All claims expressed in this article are solely those of the authors and do not necessarily represent those of their affiliated organizations, or those of the publisher, the editors and the reviewers. Any product that may be evaluated in this article, or claim that may be made by its manufacturer, is not guaranteed or endorsed by the publisher.
References
Agarwal R., Aggarwal A. N., Gupta D., Behera D., Jindal S. K. (2006). Etiology and outcomes of pulmonary and extrapulmonary acute lung injury/ARDS in a respiratory ICU in North India. Chest 130 (3), 724–729. doi:10.1378/chest.130.3.724
Bancalari E., Jain D. (2019). Bronchopulmonary dysplasia: 50 years after the original description. Neonatology 115 (4), 384–391. doi:10.1159/000497422
Becerra-Díaz M., Strickland A. B., Keselman A., Heller N. M. (2018). Androgen and androgen receptor as enhancers of M2 macrophage polarization in allergic lung inflammation. J. Immunol. 201 (10), 2923–2933. doi:10.4049/jimmunol.1800352
Behan M., Zabka A. G., Thomas C. F., Mitchell G. S. (2003). Sex steroid hormones and the neural control of breathing. Respir. physiology Neurobiol. 136 (2-3), 249–263. doi:10.1016/s1569-9048(03)00086-7
Carey M. A., Card J. W., Voltz J. W., Germolec D. R., Korach K. S., Zeldin D. C. (2007). The impact of sex and sex hormones on lung physiology and disease: lessons from animal studies. Am. J. Physiology-Lung Cell. Mol. Physiology 293 (2), L272–L278. doi:10.1152/ajplung.00174.2007
Chen W., Chen Y. Y., Tsai C. F., Chen S. C. C., Lin M. S., Ware L. B., et al. (2015). Incidence and outcomes of acute respiratory distress syndrome: a nationwide registry-based study in Taiwan, 1997 to 2011. Medicine 94 (43), e1849. doi:10.1097/MD.0000000000001849
Du X., Yao Y., Li R., Shen C., Yin H. (2005). Influence of sexual difference on expression of toll-like receptor 4 and myeloid differential protein-2 mRNA in the lung in septic rats. Zhongguo Wei Zhong Bing Ji Jiu Yi Xue 17 (12), 726–728.
Erfinanda L., Ravindran K., Kohse F., Gallo K., Preissner R., Walther T., et al. (2021). Oestrogen-mediated upregulation of the Mas receptor contributes to sex differences in acute lung injury and lung vascular barrier regulation. Eur. Respir. J. 57 (1), 2000921. doi:10.1183/13993003.00921-2020
Han M. K., Arteaga-Solis E., Blenis J., Bourjeily G., Clegg D. J., DeMeo D., et al. (2018). Female sex and gender in lung/sleep health and disease. Increased understanding of basic biological, pathophysiological, and behavioral mechanisms leading to better health for female patients with lung disease. Am. J. Respir. Crit. care Med. 198 (7), 850–858. doi:10.1164/rccm.201801-0168WS
Javaheri S., Barbe F., Campos-Rodriguez F., Dempsey J. A., Khayat R., et al. (2017). Sleep apnea: types, mechanisms, and clinical cardiovascular consequences. J. Am. Coll. Cardiol. 69 (7), 841–858. doi:10.1016/j.jacc.2016.11.069
Kamra K., Karpuk N., Adam R., Zucker I. H., Schultz H. D., Wang H. J. (2022). Time-dependent alteration in the chemoreflex post-acute lung injury. Front. Physiology 13, 2214. doi:10.3389/fphys.2022.1009607
Lee S. K. (2018). Sex as an important biological variable in biomedical research. BMB Rep. 51 (4), 167–173. doi:10.5483/bmbrep.2018.51.4.034
Lemos-Filho L. B., Mikkelsen M. E., Martin G. S., Dabbagh O., Adesanya A., Gentile N., et al. (2013). Sex, race, and the development of acute lung injury. Chest 143 (4), 901–909. doi:10.1378/chest.12-1118
Li Y.-L. (2022). Stellate ganglia and cardiac sympathetic overactivation in heart failure. Int. J. Mol. Sci. 23 (21), 13311. doi:10.3390/ijms232113311
Lin C. M., Davidson T. M., Ancoli-Israel S. (2008). Gender differences in obstructive sleep apnea and treatment implications. Sleep. Med. Rev. 12 (6), 481–496. doi:10.1016/j.smrv.2007.11.003
Lingappan K., Jiang W., Wang L., Moorthy B. (2016). Sex-specific differences in neonatal hyperoxic lung injury. Am. J. Physiology-Lung Cell. Mol. Physiology 311 (2), L481–L493. doi:10.1152/ajplung.00047.2016
Liptzin D. R., Landau L. I., Taussig L. M. (2015). Sex and the lung: observations, hypotheses, and future directions. Pediatr. Pulmonol. 50 (12), 1159–1169. doi:10.1002/ppul.23178
Marcus N. J., Del Rio R., Schultz H. D. (2014). Central role of carotid body chemoreceptors in disordered breathing and cardiorenal dysfunction in chronic heart failure. Front. physiology 5, 438. doi:10.3389/fphys.2014.00438
Mark A. Y. L. (1995). Sympathetic dysregulation in heart failure: mechanisms and therapy. Clin. Cardiol. 18 (S1), I-3-I–I8. doi:10.1002/clc.4960181303
Moreira M. C. S., Pinto I. S. J., Mourão A. A., Fajemiroye J. O., Colombari E., Reis Â. A. S., et al. (2015). Does the sympathetic nervous system contribute to the pathophysiology of metabolic syndrome? Front. physiology 6, 234. doi:10.3389/fphys.2015.00234
Moss M., Mannino D. M. (2002). Race and gender differences in acute respiratory distress syndrome deaths in the United States: an analysis of multiple-cause mortality data (1979–1996). Crit. care Med. 30 (8), 1679–1685. doi:10.1097/00003246-200208000-00001
Mowery N. T., Terzian W. H., Nelson A. C. (2020). Acute lung injury. Curr. problems Surg. 57 (5), 100777. doi:10.1016/j.cpsurg.2020.100777
Nguyen L., Castro O., De Dios R., Sandoval J., McKenna S., Wright C. J. (2019). Sex-differences in LPS-induced neonatal lung injury. Sci. Rep. 9 (1), 8514. doi:10.1038/s41598-019-44955-0
Phua J., Badia J. R., Adhikari N. K. J., Friedrich J. O., Fowler R. A., Singh J. M., et al. (2009). Has mortality from acute respiratory distress syndrome decreased over time? A systematic review. Am. J. Respir. Crit. care Med. 179 (3), 220–227. doi:10.1164/rccm.200805-722OC
Silveyra P., Fuentes N., Rodriguez Bauza D. E. (2021). Sex and gender differences in lung disease. Lung Inflamm. Health Dis. 1304, 227–258. doi:10.1007/978-3-030-68748-9_14
Souza G. M. P., Amorim M. R., Moraes D. J. A., Machado B. H. (2018). Sex differences in the respiratory-sympathetic coupling in rats exposed to chronic intermittent hypoxia. Respir. Physiology Neurobiol. 256, 109–118. doi:10.1016/j.resp.2017.09.003
Speyer C. L., Rancilio N. J., McClintock S. D., Crawford J. D., Gao H., Sarma J. V., et al. (2005). Regulatory effects of estrogen on acute lung inflammation in mice. Am. J. Physiology-Cell Physiology 288 (4), C881–C890. doi:10.1152/ajpcell.00467.2004
Tondreau M. Y., Boucher E., Simard M., Tremblay Y., Bilodeau J. F. (2012). Sex-specific perinatal expression of glutathione peroxidases during mouse lung development. Mol. Cell. Endocrinol. 355 (1), 87–95. doi:10.1016/j.mce.2012.01.022
Usselman C. W., Gimon T. I., Nielson C. A., Luchyshyn T. A., Coverdale N. S., Van Uum S. H. M., et al. (2015). Menstrual cycle and sex effects on sympathetic responses to acute chemoreflex stress. Am. J. Physiology-Heart Circulatory Physiology 308 (6), H664–H671. doi:10.1152/ajpheart.00345.2014
Vento M., Aguar M., Escobar J., Arduini A., Escrig R., Brugada M., et al. (2009). Antenatal steroids and antioxidant enzyme activity in preterm infants: influence of gender and timing. Antioxidants redox Signal. 11 (12), 2945–2955. doi:10.1089/ars.2009.2671
Xiang D., Liu Y., Zhou S., Zhou E., Wang Y. (2021). Protective effects of estrogen on cardiovascular disease mediated by oxidative stress. Oxidative Med. Cell. Longev. 2021 (1), 5523516. doi:10.1155/2021/5523516
Young B. P., Loparo K. A., Dick T. E., Jacono F. J. (2019). Ventilatory pattern variability as a biometric for severity of acute lung injury in rats. Respir. physiology Neurobiol. 265, 161–171. doi:10.1016/j.resp.2019.03.009
Keywords: acute respiratory distress syndrome, acute lung injury, bleomycin, chemoreflex, sex-based differences
Citation: Kamra K, Zucker IH, Schultz HD and Wang H-J (2024) Chemoreflex sensitization occurs in both male and female rats during recovery from acute lung injury. Front. Physiol. 15:1401774. doi: 10.3389/fphys.2024.1401774
Received: 15 March 2024; Accepted: 08 July 2024;
Published: 22 July 2024.
Edited by:
Rohit Ramchandra, The University of Auckland, New ZealandReviewed by:
Ibrahim M. Salman, Case Western Reserve University, United StatesGary C. Mouradian, Medical College of Wisconsin, United States
Copyright © 2024 Kamra, Zucker, Schultz and Wang. This is an open-access article distributed under the terms of the Creative Commons Attribution License (CC BY). The use, distribution or reproduction in other forums is permitted, provided the original author(s) and the copyright owner(s) are credited and that the original publication in this journal is cited, in accordance with accepted academic practice. No use, distribution or reproduction is permitted which does not comply with these terms.
*Correspondence: Han-Jun Wang, hanjunwang@unmc.edu