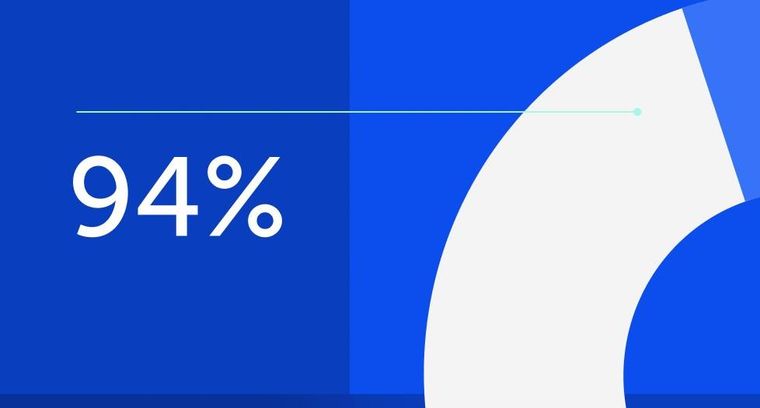
94% of researchers rate our articles as excellent or good
Learn more about the work of our research integrity team to safeguard the quality of each article we publish.
Find out more
ORIGINAL RESEARCH article
Front. Physiol., 15 May 2024
Sec. Avian Physiology
Volume 15 - 2024 | https://doi.org/10.3389/fphys.2024.1381548
This article is part of the Research TopicNutritional Impacts the Health and Physiology of the Avian Gastro-intestinal TractView all 12 articles
Background: Coccidiosis outbreaks in susceptible laying hens can significantly decrease egg production and cause substantial economic loss to the egg industry. The supplementation of poultry diets with chemotherapeutic agents is limited due to antimicrobial resistance and residue in poultry meat or processed products. Therefore, alternative strategies to control coccidiosis are needed, and Artemisia annua (AA) might have the potential to be a phytogenic feed additive, an alternative to anticoccidial agents. This study aimed to investigate the effect of the dietary supplementation of powdered AA on the performance and gut health of laying hens infected with coccidiosis by Eimeria spp.
Methods: A total of 225 Hy-Line W-36 laying hens at 23 weeks of age were allocated into 5 treatment groups: 1) control (NC), 2) pair-fed (PF) control, 3) challenged control (CC), 4) CC with dietary inclusion of 0.5% AA (0.5AA), and v) CC with dietary inclusion of 1% AA (1AA). The hens in the CC, 0.5AA, and 1AA groups were orally inoculated with sporulated oocysts of Eimeria maxima (12,500), Eimeria tenella (12,500), and Eimeria acervulina (62,500) at week 25. The PF hens received the same amount of feed consumed by the CC hens from 0–14 days post-inoculation (dpi) of Eimeria spp. The performance of the laying hens, including body weight (BW), hen–day egg production (HDEP), feed intake (FI), and feed conversion ratio (FCR), was measured weekly. Additionally, markers of intestinal health, including gut permeability, lesion score, intestinal morphometry, and immune responses, were evaluated at 6, 14, and 21 dpi.
Results: At 6 and 14 dpi, laying hens challenged with Eimeria spp. had a lower BW than PF and NC hens (p < 0.0001). Supplementation of 1% AA improved the HDEP by 8.1% compared to CC hens; however, it was still 15.4% lower than that of PF hens (p < 0.0001). The inclusion of 1% AA did not have any beneficial effect on FI; however, the FCR was improved by 0.61 (2.46) than that of CC hens (3.07; p < 0.0001). The inclusion of 1% AA reduced the severity of the intestinal lesions and increased the recovery of intestinal villi (p < 0.05). Additionally, gut permeability was significantly different between the challenged and non-challenged hens; however, among the challenged hens, the inclusion of AA reduced the gut permeability by 29% compared to CC hens (p < 0.0001). Furthermore, the inclusion of 0.5% AA reduced the inflammatory responses in the infected hens.
Conclusion: Dietary inclusion of AA partially restored the performance and gut health of the laying hens and modulated their inflammatory immune response following Eimeria infection; however, further studies are needed to better understand the mode of action and effective dosages to improve the gut health without negative impacts on the performance.
The poultry industry worldwide is under tremendous pressure to control and minimize the risk associated with avian coccidiosis (Abbas et al., 2011). Despite several strategies (biosecurity, vaccination, or the use of coccidiostat or coccidiocidal drugs) being employed to control coccidiosis in commercial poultry production, coccidiosis is still the leading cause of economic loss in the poultry industry (Peek and Landman, 2011; Liu et al., 2023). Unfortunately, the development of resistance against currently available coccidiostat or coccidiocidal drugs, potential drug residues in the final products, and consumer interests have challenged the industry for alternatives to control coccidiosis. Recently, the use of phytogenic feed additives has been increasing as it has been shown to improve the performance, immune response, and intestinal barrier functions against coccidiosis (Choi and Kim, 2020; Yadav et al., 2020; de Andrade et al., 2022; El-Shall et al., 2022; Paneru et al., 2022; 2023).
Artemisia annua (AA), also known as sweet wormwood, is an herb used in traditional Chinese medicine to treat malaria caused by multidrug-resistant Plasmodium spp (Klayman, 1985). Artemisinin, a bioactive flavonoid found in AA leaves, inhibits the growth of several stages of Plasmodium spp. (Meshnick, 2002; Golenser et al., 2006). Like Plasmodium spp., Eimeria spp. is an intracellular parasite belonging to the same family, Apicomplexa. The use of AA and its extract, artemisinin, exhibit anticoccidial effects against Eimeria tenella (Allen et al., 1997; Del Cacho et al., 2010; Hady and Zaki, 2012; Drǎgan et al., 2014; Jiao et al., 2018) when infected by it alone. However, in the field, infection from only one species is rare. The use of either dried AA leaves or its extract, artemisinin, has shown potential in improving lesion scores, reducing oocyst shedding and sporulation, and modulating the humoral and immune response in chickens (Allen et al., 1997; Arab et al., 2012; Gholamrezaie et al., 2013; Kaboutari et al., 2014; Wan et al., 2016; Song et al., 2017; Yang et al., 2021). It has been observed that apicomplexan parasites have developed an anti-apoptotic mechanism to survive intracellularly in the host (Jiao et al., 2018). During coccidia infection, Eimeria alters the host cellular pathways, inhibiting the apoptosis of infected cells by upregulating the transcriptional factor (NF-κB) and the anti-apoptotic factor in chickens as a defense mechanism. However, artemisinin suppresses the expression of NF-κB and promotes the apoptotic pathways, limiting the Eimeria infection and inflammation (Jiao et al., 2018). Furthermore, artemisinin from AA alters the cell wall formation of the oocysts by disrupting the Ca2+ homeostasis of the oocysts, leading to the death of the developing oocysts and a reduction in the sporulation rate (Del Cacho et al., 2010). Artemisinin disrupts Ca2+ homeostasis in developing oocysts, affecting Ca-dependent pathways involved in protein secretion and differentiation. This disruption inhibits cell wall formation and leads to the degeneration of micro/macrogametes, ultimately reducing sporulation rates and oocyst shedding (Del Cacho et al., 2010). Therefore, this study aimed to evaluate the effect of dried AA leaves on the performance, gut health, and immune response of laying hens infected with mixed Eimeria species.
The Institutional Animal Care and Use Committee of the University of Georgia approved the animal study protocol (A2021 12–012). A total of 225 23-week-old Hy-Line W-36 laying hens were randomly divided into 5 treatment groups (n = 45), each comprising 5 replicates. The laying hens were adapted to the diets for 2 weeks. At 25 weeks of age, the hens were challenged with mixed Eimeria species (12,500 Eimeria maxima, 12,500 E. tenella, and 62,500 Eimeria acervulina oocysts) according to the treatment groups. The five different treatments were 1) non-challenged and fed a basal diet (NC), 2) non-challenged and pair-fed (PF), 3) basal diet with mixed Eimeria spp. challenge (CC), 4) dietary inclusion of 0.5% AA leaf powder and challenged with mixed Eimeria spp. (0.5AA), and 5) dietary inclusion of 1% AA leaf powder and challenged with mixed Eimeria spp. (1AA). The powdered AA leaves were purchased from Artennua® (Barcelona, Spain). The experimental mash diets were isocaloric and isonitrogenous and formulated to meet the nutrient requirements of laying hens (Hy-Line International, Mansfield, GA). The nutritional composition of AA powder and diets are shown in Tables 1, 2, respectively. Feed and water were provided ad libitum throughout the experimental period except for the PF group. The PF group was fed the exact amount of the basal diet consumed by the CC group from 0–14 days post-inoculation (dpi) of Eimeria spp. (dpi). The feed consumption of the CC group was measured daily at the same time following the Eimeria challenge, and the average daily feed consumption was calculated. The exact amount of feed was then provided to the PF group on the next day. To ensure that the PF group had the same amount of feed as the CC group, feed addition and consumption were carefully monitored to prevent any wastage. The laying hens were housed in environmentally controlled battery cages (475 cm2/hen) equipped with trough feeders and nipple drinkers. The lighting regime was adjusted based on the breeder management guidelines (Hy-Line International, Mansfield, GA).
The laying hens were weighed at 23 and 25 weeks of age and 6, 14, and 21 dpi for growth performance. The number of eggs laid per replicate was recorded daily, and hen–day egg production (HDEP) was calculated. The daily feed consumption (ADFI) and feed conversion ratio (FCR) were measured. The FCR was expressed as the kg of feed consumed per dozen eggs produced (Sharma et al., 2022b).
At 5 dpi, 2.2 mg/mL of FITC-d (MW 4000; Sigma-Aldrich, Canada) was orally inoculated to one hen per replicate, and blood was collected 2 hours post-inoculation from the wing vein. The concentration of FITC-d that leaked into the bloodstream was measured using a plate reader at an excitation/emission wavelength of 485/525 nm (SpectraMax ABS Plus, Molecular Devices, San Jose, CA). At 6 dpi, 2 hens per replicate were euthanized, and intestinal lesion scoring was carried out for E. acervulina (in the duodenum), E. maxima (in the jejunum and ileum), and E. tenella (in the ceca) on a scale of 0–4 following the methods described by Johnson and Reid, (1970). In brief, the lesion scores were ranked based on the severity of lesions from 0 to 4, with 0 indicating no lesions and 4 indicating extremely severe lesions. The severity of intestinal lesions was determined based on the density of the characteristic lesion of each Eimeria species, inflammation and thickening of the intestinal wall, and intestinal content at their predilection sites (Johnson and Reid, 1970). On 5, 6, 7, and 8 dpi, feces were allowed to accumulate on a manure belt. On day 8, the feces on the belt were thoroughly mixed within each replicate, and feces samples were collected for oocyst shedding. The oocyst counts for E. acervulina, E. maxima, and E. tenella were counted following the procedure proposed by Goo et al. (2023). In brief, 5 g of the feces was mixed in 35 mL of tap water and left to soften for 48 h at room temperature. Afterward, the sample was vortexed and diluted in a saturated saltwater solution (1:11 ratio). Then, 650 µL of the diluted solution was added to a McMaster counting chamber (Vetlab Supply, Palmetto Bay, FL) for oocyst enumeration. The different species were identified under a microscope based on the size and morphology of the oocysts.
At 6, 14, and 21 dpi, 1 hen per replicate was randomly selected for sampling and was euthanized by cervical dislocation. For intestinal morphometric analysis, 2-cm-long sections of the duodenum, jejunum, and ileum were collected and fixed in 10% neutral-buffered formalin. The villus height (VH), crypt depth (CD), and VH-to-CD (VHCD) ratio were measured following the procedure outlined by Sharma et al. (2024b). Furthermore, samples of the jejunum and cecal tonsils were collected and snap-frozen in liquid nitrogen at the abovementioned time points for gene expression. The RNA extraction from the above samples was carried out using the TRIzol reagent (QIAGEN; Valencia, CA) following the procedures proposed by Sharma et al. (2022a). In brief, the total RNA concentration was determined using the NanoDrop-8 spectrophotometer (Thermo Fisher Scientific, Waltham, MA). It was later reverse-transcribed to cDNA after normalizing it to 2 μg/μL using a commercial kit (Applied Biosystems, Foster City, CA), and qRT-PCR was performed in duplicate. The ΔCt values of each marker gene from the NC group were used to calculate the ΔΔCt value. The expression levels of the NC group were then normalized to 1 using the 2−ΔΔCt method, and the relative fold change of the mRNA expression levels was calculated using the 2−ΔΔCT relative quantification methods compared to the NC group. The list of primers for the target and housekeeping genes is shown in Table 3.
The oxidative status of the laying hens following the Eimeria challenge was measured from 1 hen per replicate at 6, 14, and 21 dpi. The concentration of the total antioxidant capacity (TAC) in the serum was measured following the manufacturer’s instructions (BioAssay Systems, Hayward, CA). In brief, blood was collected from the wing vein, serum was separated by centrifugation at 1,000 × g for 15 min, and the TAC concentration was measured using a plate reader (λ = 485 nm; SpectraMax ABS Plus, SoftMax Pro 7 software, Molecular Devices, San Jose, CA). The superoxide dismutase (SOD) and malondialdehyde (MDA) concentrations in the liver were analyzed as a marker of oxidative status using commercial kits (MDA: BioAssay Systems, Hayward, CA; SOD: Cayman Chemical assay kits, Ann Arbor, MI). Approximately 50–70 mg of the liver sample was homogenized in the respective solutions following the manufacturer’s instructions, and the concentrations of MDA (λex/em = 530/550 nm) and SOD (λ = 450 nm) were measured using a plate reader (SpectraMax ABS Plus, SoftMax Pro 7 software, Molecular Devices, San Jose, CA). The concentrations of SOD and MDA were standardized for the protein concentration in the sample.
At 14 dpi, 10 mL of blood was withdrawn from 1 hen per replicate through the wing vein into the Na-heparin glass tubes. The peripheral mononuclear blood cells were isolated following the procedures proposed by Choi et al. (2022) to a final concentration of 4.0 × 106/mL. The CD4+ and CD8+ cell percentages were analyzed using flow cytometry following the procedures proposed by Krunkosky et al. (2020). In brief, 100 µL of the isolated cells were incubated with CD4 (mouse anti-chicken CD4-PE; SouthernBiotech, Birmingham, AL) and CD8 (mouse anti-chicken CD8a-FITC; SouthernBiotech, Birmingham, AL) antibodies. After incubation, the cells were washed with FACS-PBS and centrifuged at 250 g for 10 min, and the supernatant was discarded and resuspended in 100 μL FACS-PBS and 100 μL flow fixing solution (Thermo Fisher Scientific). Flow cytometry was conducted on a BD Accuri C6 Flow Cytometer (San Jose, CA). A total of 10,000 events were conducted per sample within the lymphocyte gate, and the expression percentages for each of the utilized markers were analyzed, as shown in Figure 1.
Figure 1. Representative histogram of the gating protocol used on the enriched chicken peripheral blood lymphocytes stained with PE-CD4 and FITC CD-8. The cells were first gated based on the size (FSC-H) and granularity (SSC-H) (A). The single cell population was then gated from the histogram (A) based on the cell area (SSC-A) and the cell height (SSC-H) (Fig. 1B). Singlet cells identified in (B) were then analyzed for PE-CD4 and FITC-CD8 staining (C).
The data related to laying hen performance, gene expression, oxidative status, and intestinal morphology were analyzed using one-way analysis of variance in SAS (SAS Institute, Cary, NC). The lesion score data were analyzed as nonparametric data using the Kruskal–Wallis test. In contrast, the feed intake and egg production data were analyzed as split plots over time using the PROC GLM procedure of SAS (SAS Institute, Cary, NC). Statistical significance was set at p < 0.05, and the means were compared using Fisher’s LSD.
The effects of feeding AA leaves on the growth performance of the laying hens following the Eimeria challenge are shown in Table 4. During the adaptation period, the BW of the hens fed 0.5% AA was reduced compared to the other treatment groups (p = 0.0642). At 6 dpi, the BW of hens infected with Eimeria spp. was reduced by at least 7% compared to 3% in the PF hens (p < 0.0001). However, at 14 dpi, the BW loss increased up to 8% in PF hens and at least 9% in the infected hens (p < 0.0001). The lower BW observed in the 0.5AA group during the adaptation period was exacerbated by the Eimeria infection, with the lowest BW observed at 14 dpi (p < 0.0001). By 21 dpi, the BW of the PF hens and challenged hens had recovered to levels comparable to those of NC hens (p = 0.1926).
Table 4. Effects of the dietary inclusion of phytogenic feed additive Artemisia annua in laying hen’s diets on the growth performance of the laying hens pre- and post-Eimeria challenge.
At 23 weeks of age, the HDEP of the laying hens fed AA was reduced, but it recovered back to the level of the NC group by 24 weeks of age (p < 0.0001; Figure 2). Following the Eimeria challenge at 25 weeks of age, the HDEP was significantly reduced in Eimeria-challenged hens compared to PF and NC hens. The daily egg production of laying hens started to decrease in Eimeria-challenged groups from 5 dpi, peaked at 9 dpi, and was not able to recover by 21 dpi, except for those hens fed 1% AA (Figure 3). The laying hens fed 0.5% and 1% AA increased their overall egg production (0–21 dpi) from 46.7% in CC hens to 52.9% and 54.8%, respectively. However, the HDEP was still lower than that of the PF (70.2%) and NC (98.0%) groups. The difference in the HDEP (0–21 dpi) between NC and PF hens was 27.8%, while it was observed to be 23.5% between PF and CC hens. The results for the ADFI following the Eimeria challenge at 25 weeks of age have a similar trend for the daily HDEP, as shown in Figure 4. An interaction was observed between different treatment groups and dpi for the ADFI (p < 0.0001). The ADFI started to decrease in challenged hens, beginning at 4 dpi, peaking at 7 dpi, and recovering to the same level as that of NC hens at 19 dpi. However, feeding AA did not have any beneficial effect on improving the ADFI. The FCR increased significantly in CC hens (7.93 kg feed/dozen of eggs), followed by 0.5AA (4.75 kg feed/dozen eggs) and 1AA (4.63 kg feed/dozen eggs) hens compared to NC hens (1.19 kg feed/dozen of eggs; p < 0.0001) at 26 weeks of age, as shown in Figure 5. However, 0.5% AA and 1%AA inclusion reduced the overall FCR by 0.50 and 0.61 during the challenged period (25–27 weeks of age), respectively.
Figure 2. Effect of the inclusion of phytogenic feed additive Artemisia annua in the diets of laying hens on their weekly hen–day egg production from 23 weeks to 27 weeks of age. At 25 weeks of age, 12,500 Eimeria maxima, 12,500 Eimeria tenella, and 62,500 Eimeria acervulina oocysts were inoculated in laying hens grouped into challenged control, 0.5% A. annua, and 1% A. annua. NC: non-challenged control; PF: pair-fed control; CC: challenged control (12,500 E. maxima; 12,500 E. tenella; and 62,500 E. acervulina oocysts per mL); 0.5AA: challenged control with the inclusion of 0.5% A. annua in the diet; 1AA: challenged control with the inclusion of 1% A. annua in the diet. Before the Eimeria challenge at 25 weeks of age, treatments of NC, PF, and CC hens at 23 and 24 weeks of age were not different among themselves. Pair-feeding was carried out from 0–14 dpi after the Eimeria challenge. HDEP: hen–day egg production (%); Trt: treatment groups; AOH: age of the hens. Graphs not sharing the same superscripts (a–j) are significantly different at p < 0.05.
Figure 3. Effect of the inclusion of phytogenic feed additive A. annua in the diets of laying hens on their daily hen–day egg production from the day of a challenge until 21 days post-Eimeria challenge. At 25 weeks of age, 12,500 E. maxima, 12,500 E. tenella, and 62,500 E. acervulina oocysts were inoculated in laying hens grouped into challenged control, 0.5% A. annua, and 1% A. annua. NC: non-challenged control; PF: pair-fed control; CC: challenged control (12,500 E. maxima; 12,500 E. tenella; and 62,500 E. acervulina oocysts per mL); 0.5AA: challenged control with the inclusion of 0.5% A. annua in the diet; 1AA: challenged control with the inclusion of 1% A. annua in the diet. HDEP: hen–day egg production (%); Trt: treatment groups; dpi: days post-inoculation of Eimeria spp.
Figure 4. Effect of the inclusion of phytogenic feed additive A. annua in the diets of laying hens on their average daily feed intake from the day of a challenge until 21 days post-Eimeria challenge. At 25 weeks of age, 12,500 E. maxima, 12,500 E. tenella, and 62,500 E. acervulina oocysts were inoculated in laying hens grouped into challenged control, 0.5% A. annua, and 1% A. annua. NC: non-challenged control; PF: pair-fed control; CC: challenged control (12,500 E. maxima; 12,500 E. tenella; and 62,500 E. acervulina oocysts per mL); 0.5AA: challenged control with the inclusion of 0.5% A. annua in the diet; 1AA: challenged control with the inclusion of 1% A. annua in the diet. ADFI: average daily feed intake (g/hen/day); Trt: treatment groups; dpi: days post-inoculation of Eimeria spp.
Figure 5. Effect of the inclusion of phytogenic feed additive A. annua in the diets of laying hens on their feed conversion ratio from 23 weeks to 27 weeks of age. At 25 weeks of age, 12,500 E. maxima, 12,500 E. tenella, and 62,500 E. acervulina oocysts were inoculated in laying hens grouped into challenged control, 0.5% A. annua, and 1% A. annua. NC: non-challenged control; PF: pair-fed control; CC: challenged control (12,500 E. maxima; 12,500 E. tenella; and 62,500 E. acervulina oocysts per mL); 0.5AA: challenged control with the inclusion of 0.5% A. annua in the diet; 1AA: challenged control with the inclusion of 1% A. annua in the diet. Before the Eimeria challenge at 25 weeks of age, treatments of NC, PF, and CC hens at 23 and 24 weeks of age were not different among themselves. Pair-feeding was carried out from 0–14 dpi after the Eimeria challenge. FCR: feed conversion ratio (kg feed per dozen eggs); Trt: treatment groups; AOH: age of the hens. Graphs not sharing the same superscripts (a–f) are significantly different at p < 0.05.
The Eimeria-challenged hens showed higher gut permeability than non-challenged hens (p < 0.0001; Table 5). Among the challenged hens, the inclusion of AA reduced the gut permeability by 0.14 μg/mL compared to CC hens (0.48 μg/mL). The inclusion of AA in the diet resulted in a reduction in the severity of intestinal lesions, transitioning from higher to lower lesion scores across all three sections of the intestine (Figure 6). Oocyst shedding was only observed in Eimeria-challenged hens, confirming that the NC and PF hens were not infected. The inclusion of 0.5% AA in the diet increased the oocyst shedding of E. tenella and reduced the oocyst shedding of E. acervulina (p < 0.05; Table 5).
Table 5. Effects of the dietary inclusion of phytogenic feed additive Artemisia annua in the diets of laying hens on their gut permeability at 5 dpi and oocyst shedding of Eimeria acervulina, Eimeria maxima, and Eimeria tenella from 5–8 dpi.
Figure 6. Effect of the inclusion of phytogenic feed additive A. annua in the diets of laying hens on their intestinal lesion score for E. acervulina (duodenum), E. maxima (part of the jejunum and ileum), and E. tenella (ceca) at 6 days post-Eimeria challenge. The lesion scores were ranked based on the severity of lesions from 0 to 4, with 0 indicating no lesions and 4 indicating extremely severe lesions. At 25 weeks of age, 12,500 E. maxima, 12,500 E. tenella, and 62,500 E. acervulina oocysts were inoculated in laying hens grouped into challenged control, 0.5% A. annua, and 1% A. annua. NC: non-challenged control; PF: pair-fed control; CC: challenged control (12,500 E. maxima; 12,500 E. tenella; and 62,500 E. acervulina oocysts per mL); 0.5AA: challenged control with the inclusion of 0.5% A. annua in the diet; 1AA: challenged control with the inclusion of 1% A. annua in the diet; dpi: days post-inoculation of Eimeria spp.
The effects of feeding AA leaves on the intestinal barrier function and nutrient transporter gene expression of the laying hens post-Eimeria challenge are shown in Table 6. At 6 and 14 dpi, the expression of JAM-2, OCLN, ZO-2, and MUC-2 was downregulated in Eimeria-challenged hens compared to non-challenged hens, whereas the expression of CLDN-1 was upregulated only at 6 dpi (p < 0.05). The inclusion of AA leaves upregulated the mRNA expression of iNOS at 6, 14, and 21 dpi (p < 0.05). The expression of nutrient transporter genes (b0+ AT, EAAT-3, y+LAT, b0AT, pepT-1, SGLT-1, and GLUT-1) was slightly upregulated in the PF group, whereas they were downregulated in the Eimeria-challenged hens at 6 dpi (p < 0.05). At 14 dpi, 1% AA inclusion upregulated the amino acid transporter genes compared to CC hens but remained lower than that of the NC and PF hens (p < 0.05). Furthermore, y+LAT, GLUT-1, and SGLT-1 expression was downregulated in PF hens compared to NC hens (p < 0.05; Table 6). At 6 dpi, the expression of cytokines IL-10 and IFN-γ in Eimeria-challenged hens (CC and 1AA) was upregulated compared to non-challenged hens. However, this upregulation was lower in hens grouped in 0.5AA than that in CC and 1AA hens (p < 0.05; Table 7). On the other hand, the expression of IL-4 was downregulated by the inclusion of AA (p = 0.0808). No significant differences in any genes among the treatment groups were observed at 14 dpi (p > 0.05). However, at 21 dpi, all treatments exhibited lower INF-γ expression than the NC treatment (p < 0.05).
Table 6. Effects of the dietary inclusion of phytogenic feed additive Artemisia annua in the diet of laying hens on their intestinal barrier function and nutrient transporter genes 6, 14, and 21 dpi.
Table 7. Effects of the dietary inclusion of phytogenic feed additive Artemisia annua in the diets of laying hens on their expression of inflammatory cytokines at 6, 14, and 21 dpi.
The effect of dietary inclusion of AA leaves in a laying hen diet infected with Eimeria spp. is presented in Table 8. At 6 dpi, the VH of the duodenum and jejunum decreased, whereas CD increased by the Eimeria challenge, resulting in a lower VH-to-CD ratio (p < 0.05). Although no significant difference was observed in ileal VH, CD was significantly increased, lowering the VH-to-CD ratio in challenged hens (p < 0.05). The inclusion of 0.5% AA resulted in a higher VH in all three sections relative to CC at 6 dpi. At 14 and 21 dpi, the inclusion of AA reduced the CD in challenged hens in all three sections of the intestine compared to the CC hens (p < 0.1). However, VH of the jejunum increased at 6 dpi (p < 0.05), and as feed restriction progressed in the PF group, a slight reduction in jejunum VH was observed at 14 and 21 dpi (p > 0.05). Although a higher jejunum CD was observed in challenged hens at 14 dpi (p < 0.05), it became similar to that of NC hens by 21 dpi. In the ileum, the VH decreased and CD increased through 21 dpi in the PF group compared to the NC group (p < 0.05).
Table 8. Effects of the dietary inclusion of phytogenic feed additive Artemisia annua in the diets of laying hens on their small intestinal histomorphology at 6, 14, and 21 dpi.
No significant differences were observed in the peripheral mononuclear blood cell count at 14 dpi (p > 0.05; Figure 7). However, the monocyte counts in the peripheral blood increased by 71% in hens grouped in CC and by 138% in those fed 1% AA compared to NC hens. Likewise, no significant differences were observed for CD4+, CD8+, and their ratio.
Figure 7. Effect of the inclusion of phytogenic feed additive A. annua in the diets of laying hens on their peripheral mononuclear blood cell count and CD4+ and CD8+ T cell population 14 days post-Eimeria challenge. At 25 weeks of age, 12,500 E. maxima, 12,500 E. maxima, and 62,500 E. tenella oocysts were inoculated in laying hens grouped into challenged control, 0.5% A. annua, and 1% A. annua. NC: non-challenged control; PF: pair-fed control; CC: challenged control (12,500 E. maxima; 12,500 E. tenella; and 62,500 E. acervulina oocysts per mL); 0.5AA: challenged control with the inclusion of 0.5% A. annua in the diet; 1AA: challenged control with the inclusion of 1% A. annua in the diet; dpi: days post-inoculation of Eimeria spp.
The effect of the inclusion of AA leaves on the oxidative status of laying hens post-Eimeria challenge is shown in Figure 8. At 6 dpi, Eimeria challenges and pair feeding reduced the total antioxidant capacity; however, at 14 dpi, the TAC increased in challenged hens but not in the PF group (p < 0.05). Likewise, MDA levels increased in Eimeria-challenged hens at 6 dpi (p < 0.05) and became similar to that of NC hens by 14 dpi. The inclusion of AA did not have a beneficial effect on improving the oxidative status of the laying hens post-Eimeria challenge.
Figure 8. Effect of the inclusion of phytogenic feed additive A. annua in the diets of laying hens on their oxidative status at 6 and 14days post-Eimeria challenge. At 25 weeks of age, 12,500 E. maxima, 12,500 E. maxima, and 62,500 E. tenella oocysts were inoculated in laying hens grouped into challenged control, 0.5% A. annua, and 1% A. annua. NC: non-challenged control; PF: pair-fed control; CC: challenged control (12,500 E. maxima; 12,500 E. tenella; and 62,500 E. acervulina oocysts per mL); 0.5AA: challenged control with the inclusion of 0.5% A. annua in the diet; 1AA: challenged control with the inclusion of 1% A. annua in the diet; TAC: total antioxidant capacity (µmol/L); MDA: malondialdehyde (µM per mg of BCA); dpi: days post-inoculation of Eimeria spp. Graphs not sharing the same superscripts (a–b) are significantly different at p < 0.05.
In this study, we investigated the role of phytogenic feed additive (A. annua) supplementation on the performance, intestinal barrier functions, oxidative status, and immune response of laying hens infected with mixed Eimeria species. Supplementing the phytogenic feed additive (A. annua) showed some beneficial effects on improving the laying hen performance, intestinal barrier functions, and, to some extent, immune responses against Eimeria spp. tested in the current study.
As expected, Eimeria infection reduced the egg-laying performance and feed intake of laying hens while increasing the feed conversion ratio over the 21-day period. Although the inclusion of AA did not improve the growth performance, it did improve the egg production and feed efficiency of the laying hens. The results of growth performance were different from those observed previously, where the inclusion of the AA leaves or its extract (artemisinin) at various levels prevented weight loss in broilers compared to Eimeria-challenged hens (Allen et al., 1997; Hady and Zaki, 2012; Pop et al., 2015). However, in this study, AA inclusion was ineffective in preventing BW loss. As the hens were not growing, they might have utilized their fat reserve to lay eggs during the acute phase (0–6 dpi) while simultaneously fighting the infection (Sharma et al., 2024b). In the current study, the distinct smell and bitter taste of AA led to strong feed refusal in the lower AA-inclusion group compared to the higher inclusion group. However, the exact reason for strong feed refusal in the lower inclusion group remains unclear. Consequently, this resulted in a decrease in body weight and egg production in the 0.5% AA-inclusion group during the adaptation period (Engberg et al., 2012). The literature on the effect of AA on laying hen performance is scarce; however, the increase in egg production in hens during the challenge period might be due to the antioxidant, antimicrobial, and antiprotozoal properties of artemisinin (Allen et al., 1997; Del Cacho et al., 2010; Engberg et al., 2012; Baghban-Kanani et al., 2019). The difference between NC and CC hens (51.3%) for the HDEP was attributed to the reduced feed intake and symptoms associated with disease during the infection period. Additionally, the difference in the HDEP between PF and CC hens (23.5%) might be attributed to the nutritional redistribution associated with inflammation and immune responses, whereas 27.8% was associated with reduced feed intake following coccidiosis.
Gastrointestinal health is of great importance in poultry as it affects nutrient digestion, absorption, and utilization, and, most importantly, if compromised, affects the health and welfare of the chickens (Choi et al., 2023a; Tompkins et al., 2023; Sharma et al., 2024a; 2024b). The findings from the current study confirmed that Eimeria species damage the intestinal tract with mild-to-severe intestinal lesions and structural damage to the intestinal architecture of the villi, ultimately affecting the performance of the laying hens. However, the inclusion of the AA in the diet reduced the severity of intestinal lesions in the duodenum, jejunum, ileum, and ceca. Previously, it had been observed that either the AA leaves or its extract (artemisinin) reduced the lesion score, oocyst shedding, and symptoms related to coccidiosis (Allen et al., 1997; Almeida et al., 2012; Hady and Zaki, 2012; Kaboutari et al., 2014; Mo et al., 2014; Jiao et al., 2018). Unlike the findings obtained by Allen et al. (1997), who reported a significant reduction in the oocyst shedding of E. tenella, our study observed only a slight decrease in the oocyst shedding of E. acervulina. The reduced oocyst shedding observed may be attributed to the artemisinin derived from the AA leaves, which alters the formation of the oocyst wall, resulting in both death and reduced sporulation rate of oocysts (Del Cacho et al., 2010; Fatemi et al., 2015; Pop et al., 2015). The variation in oocyst shedding observed between the studies might be due to differences in the source of artemisinin used, such as extracted artemisinin or artemisinin released from the AA leaves. In this study, the differences observed in E. tenella and E. acervulina oocyst shedding between the 0.5% and 1% AA inclusion groups might be associated with the environments where they reside. Unlike E. acervulina, E. tenella develops under relatively anaerobic conditions in the ceca, filled with cecal contents. This anaerobic environment in the ceca may require a higher concentration of artemisinin to exert its effects (Allen et al., 1997). Furthermore, it was reported that artemisinin from AA leaves induces apoptotic events in Eimeria-infected cells, resulting in increased apoptosis of merozoites and protozoal host cells. This ultimately reduces the second-generation merozoites released into the intestinal lumen (Del Cacho et al., 2010; Mo et al., 2014). Consequently, this reduces the intestinal lesion score and gut permeability in the infected hosts, as observed in this study.
In this study, the oxidative status of the laying hens following the Eimeria challenge among the infected groups was not different. It has been observed that artemisinin is capable of inducing oxidative stress by increasing free radicals in specific host cells where Eimeria resides. This increased production of free radicals is suggested to kill the intracellular parasite, in this case, Eimeria (Meshnick et al., 1989; Kaboutari et al., 2014; Jiao et al., 2018). Moreover, the inducible nitric oxide synthase (iNOS) expression was upregulated in hens in 0.5% AA and 1% AA groups compared to CC hens. Inducible nitric oxide synthase helps in the production of nitric oxide, which induces oxidative stress, thereby limiting Eimeria multiplication in the host cells (Castro et al., 2020). Although artemisinin has an antioxidant capacity to prevent free radicals and lipid peroxidation in non-infected birds (Drǎgan et al., 2014), the Eimeria infection may have changed its functions to specifically increase oxidative stress in infected host cells to cause apoptosis of the infected host cells and merozoites residing in them (Meshnick et al., 1989; Kaboutari et al., 2014; Jiao et al., 2018).
As previously observed, Eimeria infection significantly influenced duodenal, jejunal, and ileal morphology by decreasing the VH and increasing the CD of laying hens (Sharma et al., 2024a; 2024b). Conversely, the dietary inclusion of AA partially alleviated the negative effect of coccidiosis on the small intestinal morphometric architecture during the recovery phase (8–21 dpi). The selective apoptosis of Eimeria-infected host cells by artemisinin might have facilitated the quick recovery of intestinal villi (Mo et al., 2014; Jiao et al., 2018). As observed in this study, the beneficial effect of AA on intestinal villus recovery may depend on multiple factors, including intestinal location, dpi, and dosage. For instance, 0.5% AA inclusion increased the VH in duodenal and jejunal regions, while 1% AA reduced the CD. However, 1% AA increased the VH and reduced the CD at 21 dpi in the ileum. In PF hens, a dietary restriction similar to that of CC hens from 0–14 dpi might be one of the reasons for the regression in intestinal villus height and increased crypt depth at 21 dpi. The regression in the villi may contribute to the downregulation of glucose transporters (GLUT-1 and SGLT-1) and y+LAT amino acid transporters at 14 dpi in the PF hens. This regression in villi and downregulation of specific nutrient transporters might have also played a role in the prominent production loss observed in PF groups by 14 dpi.
The breach in the intestinal mucosa by Eimeria triggers several signaling cascades, leading to the production of cytokines involved in inflammation (Lillehoj and Lillehoj, 2000; Yun et al., 2000; Hong et al., 2006a). Following the Eimeria infection, the expression of pro-inflammatory (IFN-γ) and anti-inflammatory cytokine (IL-10) increased in challenged birds, consistent with the findings from previous studies (Hong et al., 2006b; Sharma et al., 2024a; 2024b). However, 0.5% AA inclusion reduced the expression of both IFN-γ and IL-10 compared to the CC, suggesting that AA can reduce the inflammatory response of chickens by various means and help the normal functioning and upregulation of humoral responses (Song et al., 2017; Yang et al., 2021). Artemisinin is known to suppress the production of these cytokines by inhibiting the activation of NF-κB; however, the reason why the 1% inclusion of AA did not have the same effect as 0.5% AA inclusion remains unclear (Jiao et al., 2018). Furthermore, the inclusion of 1% AA upregulated cell-mediated immunity by increasing the monocyte counts and CD8+ cells, which are responsible for clearing intracellular pathogens (Liu et al., 2023; Shah et al., 2023). The partial reduction in inflammation and increased cell-mediated immunity to kill the intracellular Eimeria parasite, along with reduced intestinal lesions and permeability in AA-fed hens, might contribute to reducing energy allocation for host defense. This could potentially improve the performance of the hens by increasing the available energy for production, consequently increasing the HDEP by at least 6.25% in the AA groups.
Based on the findings from the current study, it can be concluded that the adverse effects of the Eimeria challenge are associated with reduced feed intake and redistribution of the nutritional reserve of the host for inflammation and oxidative stress rather than the performance. However, dietary supplementation of the phytogenic feed additive (A. annua leaves) partially helped restore the egg production and intestinal integrity of the laying hens infected with Eimeria spp. Furthermore, it also helped modulate the immune response to alleviate the negative effect of coccidiosis on the performance. However, further studies need to be conducted to find the optimal dose of AA leaves to prevent feed refusal and improve the performance of laying hens.
The raw data supporting the conclusion of this article will be made available by the authors, without undue reservation.
The animal study was approved by the Institutional Animal Care and Use Committee of the University of Georgia. The study was conducted in accordance with the local legislation and institutional requirements.
MS: conceptualization, formal analysis, investigation, methodology, and writing–original draft. GL: formal analysis, investigation, methodology, and writing–review and editing. VC: formal analysis, investigation, methodology, and writing–review and editing. HR-N: formal analysis, investigation, methodology, and writing–review and editing. FM: formal analysis, investigation, methodology, and writing–review and editing. BM: investigation, methodology, and writing–review and editing. RG: formal analysis, investigation, methodology, and writing–review and editing. WK: conceptualization, data curation, funding acquisition, investigation, project administration, supervision, and writing–review and editing.
The author(s) declare that no financial support was received for the research, authorship, and/or publication of this article.
The authors declare that the research was conducted in the absence of any commercial or financial relationships that could be construed as a potential conflict of interest.
All claims expressed in this article are solely those of the authors and do not necessarily represent those of their affiliated organizations, or those of the publisher, the editors, and the reviewers. Any product that may be evaluated in this article, or claim that may be made by its manufacturer, is not guaranteed or endorsed by the publisher.
Abbas R. Z., Iqbal Z., Blake D., Khan M. N., Saleemi M. K. (2011). Anticoccidial drug resistance in fowl coccidia: the state of play revisited. World’s Poult. Sci. J. 67, 337–350. doi:10.1017/s004393391100033x
Allen P. C., Lydon J., Danforth H. D. (1997). Effects of components of Artemisia annua on coccidia infections in chickens. Poult. Sci. 76, 1156–1163. doi:10.1093/ps/76.8.1156
Almeida G. F. de, Horsted K., Thamsborg S. M., Kyvsgaard N. C., Ferreira J. F. S., Hermansen J. E. (2012). Use of Artemisia annua as a natural coccidiostat in free-range broilers and its effects on infection dynamics and performance. Veterinary Parasitol. 186, 178–187. doi:10.1016/j.vetpar.2011.11.058
Arab H. A., Katadj J. K., Rahbari S., Nabian S., Mohammadi A. R. S., Pirali-Kheirabadi K. (2012). Comparison between anticoccidial effect of granulated Artemisia siberi extract and pure Artemisinin in affected broilers. J. Veterinary Res. 67, 119–125.
Baghban-Kanani P., Hosseintabar-Ghasemabad B., Azimi-Youvalari S., Seidavi A., Ragni M., Laudadio V., et al. (2019). Effects of using Artemisia annua leaves, probiotic blend, and organic acids on performance, egg quality, blood biochemistry, and antioxidant status of laying hens. J. Poult. Sci. 56, 120–127. doi:10.2141/jpsa.0180050
Castro F. L., Teng P. Y., Yadav S., Gould R. L., Craig S., Pazdro R., et al. (2020). The effects of L-Arginine supplementation on growth performance and intestinal health of broiler chickens challenged with Eimeria spp. Poult. Sci. 99, 5844–5857. doi:10.1016/j.psj.2020.08.017
Choi J., Goo D., Sharma M. K., Ko H., Liu G., Paneru D., et al. (2023a). Effects of different Eimeria inoculation doses on growth performance, daily feed intake, gut health, gut microbiota, foot pad dermatitis, and Eimeria gene expression in broilers raised in floor pens for 35 days. Animals 13, 2237. doi:10.3390/ani13132237
Choi J., Kim W. K. (2020). Dietary application of tannins as a potential mitigation strategy for current challenges in poultry production: a review. Animals 10, 2389. doi:10.3390/ani10122389
Choi J., Marshall B., Ko H., Shi H., Singh A. K., Thippareddi H., et al. (2022). Antimicrobial and immunomodulatory effects of tannic acid supplementation in broilers infected with Salmonella Typhimurium. Poult. Sci. 101, 102111. doi:10.1016/j.psj.2022.102111
Choi J., Yadav S., Vaddu S., Thippareddi H., Kim W. K. (2023b). In vitro and in vivo evaluation of tannic acid as an antibacterial agent in broilers infected with Salmonella Typhimurium. Poult. Sci. 102, 102987. doi:10.1016/j.psj.2023.102987
de Andrade R. M., Pagnussatt H., Talian L. E., Santo A. D., Ribeiro A. B., Leite F., et al. (2022). Interaction between live vaccines for coccidiosis and phytogenic compounds in the diet of broilers. Parasitol. Int. 89, 102584. doi:10.1016/j.parint.2022.102584
Del Cacho E., Gallego M., Francesch M., Quílez J., Sánchez-Acedo C. (2010). Effect of artemisinin on oocyst wall formation and sporulation during Eimeria tenella infection. Parasitol. Int. 59, 506–511. doi:10.1016/j.parint.2010.04.001
Drǎgan L., Györke A., Ferreira J. F. S., Pop I. A., Dunca I., Drǎgan M., et al. (2014). Effects of Artemisia annua and Foeniculum vulgare on chickens highly infected with Eimeria tenella (phylum Apicomplexa). Acta veterinaria Scand. 56, 22. doi:10.1186/1751-0147-56-22
El-Shall N. A., Abd El-Hack M. E., Albaqami N. M., Khafaga A. F., Taha A. E., Swelum A. A., et al. (2022). Phytochemical control of poultry coccidiosis: a review. Poult. Sci. 101, 101542. doi:10.1016/j.psj.2021.101542
Engberg R. M., Grevsen K., Ivarsen E., Fretté X., Christensen L. P., Højberg O., et al. (2012). The effect of Artemisia annua on broiler performance, on intestinal microbiota and on the course of a Clostridium perfringens infection applying a necrotic enteritis disease model. Avian Pathol. 41, 369–376. doi:10.1080/03079457.2012.696185
Fatemi A., Razavi S. M., Asasi K., Torabi Goudarzi M. (2015). Effects of Artemisia annua extracts on sporulation of Eimeria oocysts. Parasitol. Res. 114, 1207–1211. doi:10.1007/s00436-014-4304-z
Gholamrezaie S. L., Mohammadi M., Jalali S. J., Abolghasemi S. A., roostaei A. M. (2013). Extract and leaf powder effect of Artemisia annua on performance, cellular and humoral immunity in broilers. Iran. J. Veterinary Res. 14, 15–20.
Golenser J., Waknine J. H., Krugliak M., Hunt N. H., Grau G. E. (2006). Current perspectives on the mechanism of action of artemisinins. Int. J. Parasitol. 36, 1427–1441. doi:10.1016/j.ijpara.2006.07.011
Goo D., Choi J., Ko H., Choppa V. S. R., Liu G., Lillehoj H. S., et al. (2023). Effects of Eimeria maxima infection doses on growth performance and gut health in dual-infection model of necrotic enteritis in broiler chickens. Front. Physiology 14, 1269398. doi:10.3389/fphys.2023.1269398
Hady M. M., Zaki M. M. (2012). Efficacy of some herbal feed additives on performance and control of cecal coccidiosis in broilers. APCBEE Procedia 4, 163–168. doi:10.1016/j.apcbee.2012.11.028
Hong Y. H., Lillehoj H. S., Lee S. H., Dalloul R. A., Lillehoj E. P. (2006a). Analysis of chicken cytokine and chemokine gene expression following Eimeria acervulina and Eimeria tenella infections. Vet. Immunol. Immunopathol. 114, 209–223. doi:10.1016/j.vetimm.2006.07.007
Hong Y. H., Lillehoj H. S., Lillehoj E. P., Lee S. H. (2006b). Changes in immune-related gene expression and intestinal lymphocyte subpopulations following Eimeria maxima infection of chickens. Vet. Immunol. Immunopathol. 114, 259–272. doi:10.1016/j.vetimm.2006.08.006
Jiao J. Y., Yang Y. Q., Liu M. J., Li J. G., Cui Y., Yin S. J., et al. (2018). Artemisinin and Artemisia annua leaves alleviate Eimeria tenella infection by facilitating apoptosis of host cells and suppressing inflammatory response. Veterinary Parasitol. 254, 172–177. doi:10.1016/j.vetpar.2018.03.017
Johnson J., Reid W. M. (1970). Anticoccidial drugs: lesion scoring techniques in battery and floor-pen experiments with chickens. Exp. Parasitol. 28, 30–36. doi:10.1016/0014-4894(70)90063-9
Kaboutari J., Arab H. A., Ebrahimi K., Rahbari S. (2014). Prophylactic and therapeutic effects of a novel granulated formulation of Artemisia extract on broiler coccidiosis. Trop. Animal Health Prod. 46, 43–48. doi:10.1007/s11250-013-0444-x
Klayman D. L. (1985). Qinghaosu (artemisinin): an antimalarial drug from China. Science 228 (4703), 1049–1055. doi:10.1126/science.3887571
Krunkosky M., García M., Beltran G., Williams S. M., Hurley D. J., Gogal R. M. (2020). Ocular exposure to infectious laryngotracheitis virus alters leukocyte subsets in the head-associated lymphoid tissues and trachea of 6-week-old White Leghorn chickens. Avian Pathol. 49, 404–417. doi:10.1080/03079457.2020.1757036
Lillehoj H. S., Lillehoj E. P. (2000). Avian coccidiosis. A review of acquired intestinal immunity and vaccination strategies. Avian Dis. 44, 408–425. doi:10.2307/1592556
Liu G., Ajao A. M., Shanmugasundaram R., Taylor J., Ball E., Applegate T. J., et al. (2023). The effects of arginine and branched-chain amino acid supplementation to reduced-protein diet on intestinal health, cecal short-chain fatty acid profiles, and immune response in broiler chickens challenged with Eimeria spp. Poult. Sci. 102, 102773. doi:10.1016/j.psj.2023.102773
Meshnick S. R. (2002). Artemisinin: mechanisms of action, resistance and toxicity. Int. J. Parasitol. 32, 1655–1660. doi:10.1016/s0020-7519(02)00194-7
Meshnick S. R., Tsang T. W., Lin F. B., Pan H. Z., Chang C. N., Kuypers F., et al. (1989). “Activated oxygen mediates the antimalarial activity of qinghaosu,” in Malaria and the red cell: 2. Proceedings of the second workshop on malaria and the red cell, held in Ann Arbor (USA: Michigan), 95–104.
Mo P., Ma Q., Zhao X., Cheng N., Tao J., Li J. (2014). Apoptotic effects of antimalarial artemisinin on the second generation merozoites of Eimeria tenella and parasitized host cells. Veterinary Parasitol. 206, 297–303. doi:10.1016/j.vetpar.2014.09.025
Paneru D., Tellez-Isaias G., Arreguin-Nava M. A., Romano N., Bottje W. G., Asiamah E., et al. (2023). Effect of fenugreek seeds and Bacillus-based direct-fed microbials on the growth performance, blood biochemicals, and intestinal histomorphology of broiler chickens. Front. Veterinary Sci. 10, 1298587. doi:10.3389/fvets.2023.1298587
Paneru D., Tellez-Isaias G., Romano N., Lohakare G., Bottje W. G., Lohakare J. (2022). Effect of graded levels of fenugreek (trigonella foenum-graecum L.) seeds on the growth performance, hematological parameters, and intestinal histomorphology of broiler chickens. Veterinary Sci. 9, 207. doi:10.3390/vetsci9050207
Peek H. W., Landman W. J. M. (2011). Coccidiosis in poultry: anticoccidial products, vaccines and other prevention strategies. Veterinary Q. 31, 143–161. doi:10.1080/01652176.2011.605247
Pop L., Györke A., Tăbăran A. F., Dumitrache M. O., Kalmár Z., Magdaş C., et al. (2015). Effects of artemisinin in broiler chickens challenged with Eimeria acervulina, E. maxima and E. tenella in battery trials. Veterinary Parasitol. 214, 264–271. doi:10.1016/j.vetpar.2015.10.011
Shah B. R., Hakeem W. A., Shanmugasundaram R., Selvaraj R. K. (2023). Effect of synbiotic supplementation on production performance and severity of necrotic enteritis in broilers during an experimental necrotic enteritis challenge. Poult. Sci. 102, 102959. doi:10.1016/j.psj.2023.102959
Sharma M. K., Liu G., White D. L., Kim W. K. (2024a). Graded levels of Eimeria infection linearly reduced the growth performance, altered the intestinal health, and delayed the onset of egg production of Hy-Line W-36 laying hens when infected at the prelay stage. Poult. Sci. 103, 103174. doi:10.1016/j.psj.2023.103174
Sharma M. K., Liu G., White D. L., Tompkins Y. H., Kim W. K. (2022a). Effects of mixed Eimeria challenge on performance, body composition, intestinal health, and expression of nutrient transporter genes of Hy-Line W-36 pullets (0-6 wks of age). Poult. Sci. 101, 102083. doi:10.1016/j.psj.2022.102083
Sharma M. K., Singh A. K., Goo D., Choppa V. S. R., Ko H., Shi H., et al. (2024b). Graded levels of Eimeria infection modulated gut physiology and temporarily ceased the egg production of laying hens at peak production. Poult. Sci. 103, 103229. doi:10.1016/j.psj.2023.103229
Sharma M. K., White D. L., Singh A. K., Liu H., Tan Z., Peng X., et al. (2022b). Effect of dietary supplementation of probiotic Aspergillus Niger on performance and cecal microbiota in hy-line W-36 laying hens. Animals 12, 2406. doi:10.3390/ani12182406
Song Z., Cheng K., Zhang L., Wang T. (2017). Dietary supplementation of enzymatically treated Artemisia annua could alleviate the intestinal inflammatory response in heat-stressed broilers. J. Therm. Biol. 69, 184–190. doi:10.1016/j.jtherbio.2017.07.015
Tompkins Y. H., Choi J., Teng P. Y., Yamada M., Sugiyama T., Kim W. K. (2023). Reduced bone formation and increased bone resorption drive bone loss in Eimeria infected broilers. Sci. Rep. 2023 13 (1), 616. doi:10.1038/s41598-023-27585-5
Wan X. L., Niu Y., Zheng X. C., Huang Q., Su W. P., Zhang J. F., et al. (2016). Antioxidant capacities of Artemisia annua L. leaves and enzymatically treated Artemisia annua L. in vitro and in broilers. Animal Feed Sci. Technol. 221, 27–34. doi:10.1016/j.anifeedsci.2016.08.017
Yadav S., Teng P. Y., Souza dos Santos T., Gould R. L., Craig S. W., Lorraine Fuller A., et al. (2020). The effects of different doses of curcumin compound on growth performance, antioxidant status, and gut health of broiler chickens challenged with Eimeria species. Poult. Sci. 99, 5936–5945. doi:10.1016/j.psj.2020.08.046
Yang S., Zhang J., Jiang Y., Xu Y. Q., Jin X., Yan S. M., et al. (2021). Effects of Artemisia argyi flavonoids on growth performance and immune function in broilers challenged with lipopolysaccharide. Anim. Biosci. 34, 1169–1180. doi:10.5713/ab.20.0656
Keywords: Artemisia annua, phytogenic feed additive, laying hens, coccidiosis, gut health, egg production
Citation: Sharma MK, Liu G, Choppa VSR, Rafieian-Naeini HR, Mahdavi FS, Marshall B, Gogal RM Jr and Kim WK (2024) Effects of Artemisia annua supplementation on the performance and gut health of laying hens challenged with mixed Eimeria species. Front. Physiol. 15:1381548. doi: 10.3389/fphys.2024.1381548
Received: 04 February 2024; Accepted: 24 April 2024;
Published: 15 May 2024.
Edited by:
Katarzyna B. Miska, Agricultural Research Service (USDA), United StatesReviewed by:
Sara Cloft, Purdue University, United StatesCopyright © 2024 Sharma, Liu, Choppa, Rafieian-Naeini, Mahdavi, Marshall, Gogal and Kim. This is an open-access article distributed under the terms of the Creative Commons Attribution License (CC BY). The use, distribution or reproduction in other forums is permitted, provided the original author(s) and the copyright owner(s) are credited and that the original publication in this journal is cited, in accordance with accepted academic practice. No use, distribution or reproduction is permitted which does not comply with these terms.
*Correspondence: Woo Kyun Kim, d2traW1AdWdhLmVkdQ==
Disclaimer: All claims expressed in this article are solely those of the authors and do not necessarily represent those of their affiliated organizations, or those of the publisher, the editors and the reviewers. Any product that may be evaluated in this article or claim that may be made by its manufacturer is not guaranteed or endorsed by the publisher.
Research integrity at Frontiers
Learn more about the work of our research integrity team to safeguard the quality of each article we publish.