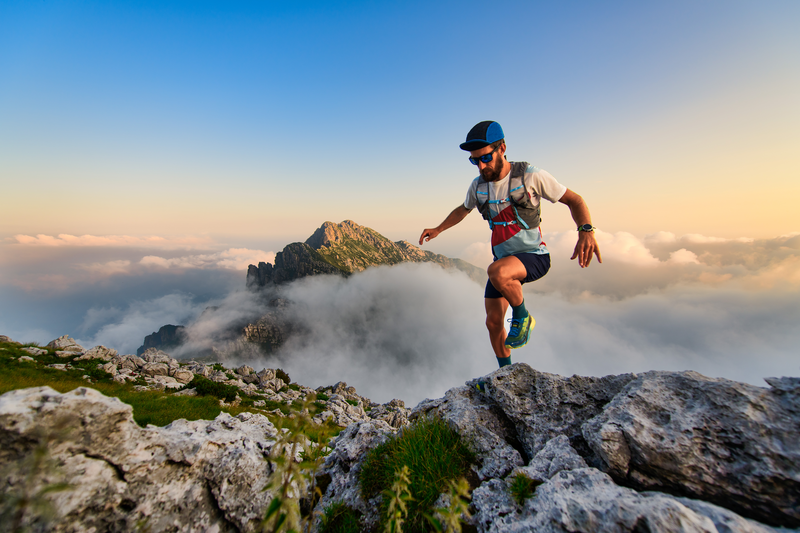
94% of researchers rate our articles as excellent or good
Learn more about the work of our research integrity team to safeguard the quality of each article we publish.
Find out more
ORIGINAL RESEARCH article
Front. Physiol. , 19 April 2024
Sec. Clinical and Translational Physiology
Volume 15 - 2024 | https://doi.org/10.3389/fphys.2024.1368542
This article is part of the Research Topic Regulation of Lipid Metabolism in Adipose Tissue and Skeletal Muscle - Volume II View all 5 articles
Background: Many people infected with COVID-19 develop myocardial injury. Epicardial adipose tissue (EAT) is among the various risk factors contributing to coronary artery disease. However, its correlation with myocardial injury in patients diagnosed with COVID-19 remains uncertain.
Methods: We examined myocardial biomarkers in population affected by COVID-19 during the period from December 2022 to January 2023. The patients without myocardial injury were referred to as group A (n = 152) and those with myocardial injury were referred to as group B (n = 212).
Results: 1) The A group and the B group exhibitedstatistically significant differences in terms of age, TC, CRP, Cr, BUN, LDL-C, IL-6, BNP, LVEF and EAT (p < 0.05). 2) EAT volumehad a close relationship with IL-6, LDL-C, cTnI, and CRP (p < 0.05); the corresponding correlation coefficient values were 0.24, 0.21, 0.24, and 0.16. In contrast to those with lower EAT volume, more subjects with a higher volume of EAT had myocardial injury (p < 0.05). Regression analysis showed that EAT, LDL-C, Age and Cr were established as independent risk variables for myocardial injury in subjects affected by COVID-19. 3) In COVID-19 patients, the likelihood of myocardial injury rised notably as EAT levels increase (p < 0.001). Addition of EAT to the basic risk model for myocardial injury resulted in improved reclassification. (Net reclassification index: 58.17%, 95% CI: 38.35%, 77.99%, p < 0.001).
Conclusion: Patients suffering from COVID-19 with higher volume EAT was prone to follow myocardial injury and EAT was an independent predictor of heart damage in these individuals.
Since March 2020, the COVID-19 pandemic has unfolded as a lethal viral crisis, unleashing widespread devastation on human lives and the global economy (An et al., 2022). The national healthcare systems are overwhelmed because COVID-19 cases often come with respiratory symptoms. Individuals with the virus infection present with a range of clinical symptoms, such as breathlessness, fever, fatigue, coughing, diarrhea, nausea, reduced lymphocytes, and some individuals may also experience neurological issues like headaches, dizziness, and changes in awareness levels. , and in severe cases, mortality can occur as a result of ARDS and low levels of oxygen saturation (Parasher, 2021).
Nevertheless, in clinical practice, the heart damage caused by COVID-19 is frequently disregarded or identified too late. A considerable proportion of patients experience myocardial injury are detected following their infection with COVID-19 (Abdi et al., 2022; Artico et al., 2023). It was shown that 32% of COVID-19 pneumonia patients experience acute injury to the heart muscle, which was observed through elevated levels of cTnI (Chen et al., 2021). Older patients with pre-existing medical conditions and those who have had COVID-19 are more susceptible to heart damage. This can lead to abnormal heart rhythms, heart attacks, and heart failure (Pellicori et al., 2021). COVID-19 patients with pneumonia and heart damage face a higher risk of experiencing severe medical condition, long recovery times, and increased chances of death for which many treatments are not very effective. Moreover, the exact understanding of the pathophysiological process by which COVID-19 causes damage to the heart muscle remains incomplete. As COVID-19 patients who also experience heart injury tend to have a negative prognosis (Al-Wahaibi et al., 2020), it is highly valuable to detect individuals who are prone to heart injury during their COVID-19 infection.
Epicardial adipose tissue (EAT) is a distinct fat deposit situated between the heart muscle and the inner layer of the heart’s outer covering. It has various impacts on cardiovascular disease. Localized inflammation is a direct result of EAT on coronary atherosclerosis (Karampetsou et al., 2022). EAT leads to myocarditis and harm to the heart by releasing substances that cause inflammation through the paravalvular wall (Lasbleiz et al., 2021). Additionally, the volume of epicardial fat can predict the severity of COVID-19 symptoms (Erdöl et al., 2022). It is possible that EAT could play a part in the cardiac syndrome associated with COVID-19 (Iacobellis, 2022). The reported diagnostic value of EAT for the severity and occurrence of heart damage in people who had a COVID-19 infection remains uncertain. Additional research is still required to examine the factors that contribute to the development of cardiac damage following infection with the novel coronavirus. In our study, we divided individuals with COVID-19 infection into groups with myocardial injury and groups without myocardial injury to study the role and significance of EAT in myocardial injury. This research will offer a fresh approach in diagnosing patients who have both myocardial injury and COVID-19, pointing them towards a new direction.
The individuals were adult patients diagnosed with COVID-19 by completing reverse transcription-polymerase chain reaction (RT-PCR) test and admitted to the Second Affiliated Hospital of Soochow University and the Tongren Hospital affiliated to Shanghai Jiao Tong University from Dec. 2022 to Jan. 2023, all of whom were tested for chest CT scan. The patients admitted to the hospital mainly have COVID-19 pneumonia according CT scan. We enrolled 364 COVID-19 patients without oxygen saturation decrease in our study. And it adhered to the STROBE checklist and obtained approval from the ethics board of the Second Affiliated Hospital of Soochow University (JD-HG-2023-76).
A total of 5 mL venous blood samples were collected from the study participants in the morning after a 12-h fasting period. After immediate centrifugation at 4°C, aliquots were stored at −80°C until analysis. FBG was measured by an automated glucose oxidase method (Automatic Analyzer 2700, Olympus, Tokyo, Japan). Serum total cholesterol (TC), triglyceride (TG), high-density lipoprotein cholesterol (HDL), low-density lipoprotein cholesterol (LDL-C), Cr, BUN, CRP, IL-6, BNP were measured by enzymatic methods. Troponin markers were evaluated within 24 h of hospital admission for all patients included in both cohorts to ensure consistency in the timing of exposure assessment. Tropoin I was measured using Troponin I (cTnI) detection kit, with each value reported as the mean of duplicate measurements made on the same serum sample. Test range was 0.01–50 ng/mL, and intraassay and interassay coefficient of variability (CVs) were less than 10% and 15%, respectively. The normal standard for cTnI with the kit is 0–30 ng/L.
Myocardial injury is defined as patients with cTnI elevated (Cao et al., 2020; Sandoval et al., 2020), and two cardiologists ruled out acute myocardial infarction based on clinical manifestations and electrocardiogram. According to whether cTnI levels exceed 30 ng/L, all participants are divided into two groups:group A comprised 152 cases of subjects who were not with myocardial injury, while 212 cases with myocardial injury were in group B. Apical two-chamber and four-chamber echocardiographic images were used to calculate volumes and left ventricular ejection fraction (LVEF) using Simpson’s biplane rule by investigators blinded to all patient data. In addition, Gender, BMI were recorded.
Patients with following conditions were excluded. 1) Combination of severe cardiac emergencies, such as myocardial infarction, aortic dissection, etc., 2) Combination of severe heart valve diseases; 3) Combined with severe liver and kidney disfunction; 4) Combination of severe autoimmune diseases; 5) Combination of tumor and hematologic related diseases (Figure 1).
Figure 1. The process diagram depicting the participant pool illustrates the overall figures and rationales for exclusion at every stage.
Epicardial adipose tissue volume was assessed by two clinicians who underwent specialized training in measuring EAT. In terms of chest CT, we chose electron beam CT scanning that would not need contrast agents, and we used GE (Healthcare, Milwaukee, United States) or Siemens (Healthiness, Erlangen, Germany) scanners to do the examinations. Tissue was classified as EAT if it fell within the parietal pericardial boundary and exhibited fat density attenuation on CT, with measurements ranging from −30 to −190 Hounsfield units (HU) (Xu et al., 2018). The superior and inferior boundary of the heart were determined as the pulmonic artery bifurcation and tip of the left ventricle, respectively. Right and left atrioventricular sulcus, anterior interventricular sulcus, and left atrioventricular sulcus were measured in the standard long-axis view of the heart, upper and lower interventricular sulcus were measured in short-axis views of the base of the heart, and finally in the right ventricular free wall (Xu et al., 2018). Epicardial fat pockets were manually outlined to determine their respective areas, and the cumulative epicardial fat area per slice was documented. Volumetric assessment was derived by the epicardial fat area in conjunction with a thickness of 3 mm (Figure 2).
Figure 2. Computed tomography is utilized to evaluate epicardial adipose tissue. From original CT (A), then selected the threshold value from −190HU to −30HU, and Marked the EAT tissue (B), updated a flat EAT tissue (C), finally reconstructed the images of EAT. Adipose tissue was emphasized in red, while the process was indicated by blue arrows. (D) Is reconstruction of EAT.
Utilizing the statistical program SPSS 26.0, the data were statistically evaluated. Continuous variables were presented as median [interquartile range (IQR)], categorical variables were presented as frequencies (percentages), and intergroup comparisons conducted the two independent sample t-test. The relationships between EAT levels and other variables were assessed using Pearson’s chi-squared test. Logistic regression was employed to analyze the association between EAT and myocardial injury in individuals. We utilized restricted cubic spline (RCS) analysis with 4 knots to examine the nonlinear correlation between increasing EAT and myocardial injury. Model comparisons were performed utilizing continuous and categorical net reclassification index (NRI) as well as integrated discrimination improvement (IDI). p < 0.05 (two-tailed) was considered meaningful. NRI indicated the improved accuracy in categorizing higher-risk patients into myocardial injurygroup and those who did not develop a heart damage into the lower-risk categories after incorporating EAT into prediction models. IDI measured the increased difference in mean probability of predicting myocardial injury risk in the cases compared to controls, indicating whether the addition of EAT improved the model’s ability to differentiate between cases and controls.
In this research, 364 patients in total were analyzed, of whom 152 were in group without myocardial injury and 212 in group with myocardial injury according cTnI levels more than 30 ng/L. The two patient groups’ general information and biochemical indicators were compared, as indicated in Table 1. Compared to the without myocardial injury group, individuals within the myocardial injury group displayed a tendency towards older age, raised levels of Cr, BUN, CRP, LDL-C, IL-6, TC, BNP, and lower LVEF(p < 0.05). In addition, Gender, BMI, and TG levels found no notable discrepancies between the two cohorts statistically. (p > 0.05).
Within myocardial injury group, the correlation between the volume of EAT and other parameters in COVID-19 patients was analyzed. The findings revealed that the volume of EAT was positively associated with LDL-C, cTnI, CRP and IL-6 (correlation index: r = 0.21, 0.24, 0.16, and 0.24, respectively; p < 0.05), negatively relation with LVEF (r = −0.25, p = 0.024). The findings were displayed in Table 2.
Univariate and multivariate logistic regression analyses for risk factors related to myocardial injury in the overall cohort are displayed in Table 3. The findings excluded the following variables as factors affecting myocardial injury- EAT (p trend < 0.001), age (p < 0.001), LDL-C (p < 0.001), CRP (p = 0.001), IL-6 (p = 0.009), TC (p = 0.007) and Cr (p < 0.001). The aforementioned variables were included in the multiple linear regression model, where it was observed that the following variables significantly contributed to myocardial injury—EAT (p trend < 0.001), Cr (p < 0.001), LDL-C (p = 0.003), and age (p < 0.001), as depicted in Table 3. OR (95% CI) associated with the third quartile of EAT was 4.10 (2.02–8.30) (p < 0.001).
The ROC curves of the EAT, LDL-C, Cr, and age in the overall cohort are shown in Figure 3, and their AUCs were 0.685, 0.652, 0.66, and 0.714, respectively (Table 4). Restricted cubic splines were employed to assess the link between EAT and the risk of cardiac muscle damage. As depicted in Figure 4, a positive correlation between EAT and myocardial injury risk was identified (likelihood ratio test, p for non-linearity = 0.020). Knots were tested between 3 and 7, with the model featuring the lowest Akaike information criterion value chosen for RCS analysis. Finally, we used RCS with 4 knots. The reference value was set depending on the RCS shape).
Figure 3. Receiver operating characteristic curves for myocardial injury estimated by the LDL-C, age, Cr, and EAT.
Table 4. Area under the curve for various significant independent risk factors of myocardial injury.
We utilized clinical variables and univariate analysis to identify significant covariates, and subsequently evaluated whether EAT enhances the discrimination of COVID-19 myocardial injury compared to other risk factors. Initially, upon incorporating EAT into the baseline risk model consisting of age, Cr, and LDL-C, the c-index increased from 0.794 to 0.818 (p = 0.035) compared to the original model (Table 5). Furthermore, we examined the enhanced reclassification. Risk categories were delineated as low, medium, and high-risk, with thresholds set at 0.2 and 0.8, signifying probabilities of <20%, 20%–80%, and >80% for cardiac damage occurrence in subjects with the novel coronavirus. Finally, we obtained a continuous NRI (95% CI) of 58.17% (38.35%–77.99%) (p < 0.001), and a categorical NRI (95% CI) of 8.28% (1.19%–15.37%) (p = 0.022), indicating that incorporating EAT into the model increased the accurate reclassification percentage by 58.17% and improved the ordered categorical risk probability by 8.28%. It suggested that adding the predictive variable (EAT) improved the model’s accuracy in predicting myocardial injury. Additionally, we obtained an integrated discrimination improvement (IDI) of 3.94% (95% CI: 1.84%–6.03%), indicating that after incorporating additional factors—EAT, the model’s predictive ability increased by 3.94% (p < 0.001) (Table 5).
Table 5. Metrics for reclassification and discrimination of myocardial injury by EAT in subjects. Patients were divided into 3 risk categories: <20%, 20%–80%, >80%.
Our research findings show that higher volumes of epicardial adipose tissue are linked to increased myocardial injury, as assessed by cTnI (Cao et al., 2020; Sandoval et al., 2020). EAT volume has a positive correlation observed with the parameters of CRP and LDL-C in our COVID-19 subjects, which agrees with observations from previous studies of coronary heart disease populations (Sever et al., 2013; Packer, 2018). However, it is noteworthy that EAT independently contributes to the risk of myocardial harm in individuals with COVID-19. EAT also serves as a predictor of cardiac tissue damage among subjects.
Since COVID-19 became popular worldwide in March 2020, many studies have focused on the multiple organ complications caused by it, such as acute myocardial injury, renal failure or thromboembolic events (Salyer et al., 2021; Ahamed and Laurence, 2022). COVID-19 poses potential implications for the cardiovascular health around the globe. The virus has the capability to affect not only the lungs but also the heart, vascular tissues, and circulating cells. The occurrence of acute cardiac muscle damage is increasingly recognized as a common extrapulmonary complication of the novel coronavirus, carrying potential long-lasting implications (Chung et al., 2021). Considering the significant inflammatory nature of COVID-19, COVID-19 survivors may be at risk of developing persistent residual myocardial injury (Shchendrygina et al., 2021). Our study also finds that the population of patients with myocardial injury has higher inflammatory indicators.
Numerous studies have delved into the potential mechanisms underlying myocardial injury induced by COVID-19 (Babapoor-Farrokhran et al., 2020). Vascular endothelial cells are pivotal in maintaining vascular homeostasis and regulating the coagulation system. In a healthy state, endothelial cells express factors that promote vascular relaxation, enhance blood flow, inhibit platelet aggregation and coagulation, and facilitate fibrinolysis. However, dysfunctional endothelial cells disrupt this balance, leading to vascular constriction and thrombus formation (Broekman et al., 1991). Infection with COVID-19 may directly lead to endothelial injury and dysfunction. Examinations of autopsies and biomarkers indicate that endotheliitis plays a role in causing acute respiratory distress syndrome (ARDS) in patients with the novel coronavirus. SARS-CoV-2 infects and disrupts the functions of lung endothelial cells (Joffre et al., 2022). Endothelial dysfunction linked to COVID-19 can initiate organ impairment and thrombosis occurrences (Gu et al., 2021). Abnormal endothelial function and thrombus formation are important mechanisms of myocardial injury, therefore, the mechanism of myocardial injury caused by COVID-19 may be caused by endothelial dysfunction and thrombosis.
While the heart and blood vessels are susceptible to novel coronavirus, there is limited substantial evidence supporting direct myocardial infection by SARS-CoV-2. Further pathological investigations and autopsy analyses are essential for elucidating the virus’s potential to directly induce myocarditis (Halushka and Vander Heide, 2021). Endomyocardial biopsy conducted on five patients demonstrate heightened macrophage counts in all cases, with one patient showing signs of lymphocytic myocarditis. Notably, qRT-PCR do not identify any SARS-CoV-2 RNA in any of the biopsies (Weckbach et al., 2021).
Inflammation increase is involved in cardiac injury was reports by many studies (Patel et al., 2022). Histologically, no COVID-19 samples have been detected as viral myocarditis. However, as time progresses, quantitative analysis using MPX reveals a notable rise in the quantity of perivascular CD11b/TIE2+ macrophages in the cases. This indicates that the development of heart damage in the individuals with the virus is primarily driven by a macrophage-driven perivascular inflammatory process (Werlein et al., 2023). Patients experiencing severe complications have higher C-reactive protein and troponin T level, the severity of cardiovascular complications like heart rate variability and heart failure following virus infection associated with elevated CRP (Lewek et al., 2021; Patel et al., 2022). Our research also finds that IL-6 and CRP is significantly increased, suggesting that inflammation may be one of the mechanisms contributing to myocardial harm in cases of COVID-19.
Elevated lipid levels may exacerbate the prognosis of individuals with the novel coronavirus. The research indicates that the ratios of TG/HDL-C, TC/HDL-C, LDL-C/HDL-C, and the TG index exhibit statistical correlations with death rate from the coronavirus disease 2019 (Rohani-Rasaf et al., 2022). Moreover, individuals with heterozygous familial hypercholesterolemia face heightened risks of cardiovascular complications in the acute stage of COVID-19 infection. During the heightened inflammatory response in COVID-19 pneumonia, elevated LDL-C levels can exacerbate endothelial dysfunction and promote the release of prothrombotic mediators (Vuorio et al., 2023). Another study indicates that patients who has minimal LDL-C upon admission are more prone to immune and inflammation dysfunction, as well as multi-organ dysfunction (Zhao et al., 2021). Additionally, Gong et al. identified a U-shaped curve relationship between LDL-C levels and the risk of severe COVID-19, both diminished and heightened LDL-C levels were significantly linked to a greater risk of severe COVID-19 outcomes (Gong et al., 2021). In conclusion, dyslipidemia may be closely related to the prognosis of COVID-19, but there is currently no study exploring the clinical correlation between LDL-C and myocardial injury. Our research has made a valuable discovery that increased blood lipid level is one of the elements contributing to COVID-19-related myocardial injury.
Numerous studies highlight the close correlation between epicardial adipose tissue (EAT) and the severity of COVID-19-related illnesses (Mehta et al., 2022). EAT attenuation independently predicted critical illness respect to time to death or admission to ICU (Conte et al., 2021). EAT volume shows associations with inflammatory parameters, while EAT area serves as a predictive marker for mortality among young adult patients (Malavazos et al., 2020; Emekli et al., 2022), and an EAT volume of 97 cm³ demonstrates strong sensitivity and specificity in predicting a more extensive pulmonary involvement and consequently, a poorer clinical outcome in patients with SARS-CoV-2 pneumonia (Marcucci et al., 2022). Additionally, irrespective of BMI, age, and comorbidities, there is a notable correlation between EAT thickness and both the severity and mortality of COVID-19, Notably, heightened EAT thickness is linked to elevated levels of ultrasensitive cardiac troponins (Börekçi et al., 2014). Our research also found that in patients suffering from COVID-19, EAT volume was related to the degree of pneumonia, and the CUT OFF value of EAT volume had a very valuable predictive significance for myocardial injury among these patients. We did not investigate the mechanism between EAT and myocardial injury. The underlying mechanism may be as follows, firstly, high levels of EAT have a pro-inflammatory effect, and EAT patients may cause myocardial damage through inflammatory mechanisms (Malavazos et al., 2020). Secondly, high EAT is related to LDL-C, and LDL-C also plays a significant role in COVID-19 related myocardial injury (Gong et al., 2021), Therefore, high levels of EAT leads to myocardial injury may be associated with high levels of LDL-C. Finally, high EAT is associated with coronary microcirculation disorders (Nakanishi et al., 2017), while COVID patients usually have a hypercoagulable state (Levi et al., 2020). So high EAT patients are prone to form micro thrombosis and lead to myocardial damage.
The observations of the current investigation are limited by the small number of patients. Therefore, the clinical implementation of the results may be restricted. Further studies with many patients are required to confirm our results. Secondly, as this is a cross-sectional study, the causal relationship between EAT and myocardial injury needs to be confirmed by longitudinal and/or interventional studies. Thirdly, our study does not further analyze the duration of patients infected with COVID-19, so it may have a certain impact on inflammation and the quantification of myocardial enzymes.
The raw data supporting the conclusion of this article will be made available by the authors, without undue reservation.
The studies involving humans were approved by the Ethics Committee of the Second Affiliated Hospital of Soochow University (No. JD-HG-2023-76). The studies were conducted in accordance with the local legislation and institutional requirements. The participants provided their written informed consent to participate in this study.
TS: Data curation, Investigation, Writing–original draft. BZ: Writing–original draft. CT: Writing–original draft. SQ: Formal Analysis, Methodology, Writing–original draft. YF: Project administration, Supervision, Writing–review and editing. HP: Formal Analysis, Software, Writing–review and editing. XG: Funding acquisition, Project administration, Supervision, Writing–review and editing.
The author(s) declare that financial support was received for the research, authorship, and/or publication of this article. This was provided by the Project of State Key Laboratory of Radiation Medicine and Protection, Soochow University (No. GZK1202135), as well as the Academic Lifts Project of the Second Affiliated Hospital of Soochow University (No. XKTJ-HRC2021007). Additionally, support was provided by the National Natural Science Foundation of China (No. 82170831).
The collaboration between the Department of Cardiology at the Second Affiliated Hospital of Soochow University and the Department of Emergency at Tongren Hospital of Shanghai Jiaotong University facilitated the execution of this study. We express our gratitude to all staff members for their contributions to data collection.
The authors declare that the research was conducted in the absence of any commercial or financial relationships that could be construed as a potential conflict of interest.
All claims expressed in this article are solely those of the authors and do not necessarily represent those of their affiliated organizations, or those of the publisher, the editors and the reviewers. Any product that may be evaluated in this article, or claim that may be made by its manufacturer, is not guaranteed or endorsed by the publisher.
Abdi A., AlOtaiby S., Badarin F. A., Khraibi A., Hamdan H., Nader M. (2022). Interaction of SARS-CoV-2 with cardiomyocytes: insight into the underlying molecular mechanisms of cardiac injury and pharmacotherapy. Biomed. Pharmacother. 146, 112518. doi:10.1016/j.biopha.2021.112518
Ahamed J., Laurence J. (2022). Long COVID endotheliopathy: hypothesized mechanisms and potential therapeutic approaches. J. Clin. Invest. 132 (15), e161167. doi:10.1172/JCI161167
Al-Wahaibi K., Al-Wahshi Y., Mohamed Elfadil O. (2020). Myocardial injury is associated with higher morbidity and mortality in patients with 2019 novel coronavirus disease (COVID-19). SN Compr. Clin. Med. 2 (12), 2514–2520. doi:10.1007/s42399-020-00569-6
An X., Xiao L., Yang X., Tang X., Lai F., Liang X. H. (2022). Economic burden of public health care and hospitalisation associated with COVID-19 in China. Public Health 203, 65–74. doi:10.1016/j.puhe.2021.12.001
Artico J., Shiwani H., Moon J. C., Gorecka M., McCann G. P., Roditi G., et al. (2023). Myocardial involvement after hospitalization for COVID-19 complicated by troponin elevation: a prospective, multicenter, observational study. Circulation 147 (5), 364–374. doi:10.1161/CIRCULATIONAHA.122.060632
Babapoor-Farrokhran S., Gill D., Walker J., Rasekhi R. T., Bozorgnia B., Amanullah A. (2020). Myocardial injury and COVID-19: possible mechanisms. Life Sci. 253, 117723. doi:10.1016/j.lfs.2020.117723
Börekçi A., Gür M., Özaltun B., Baykan A. O., Harbalioğlu H., Seker T., et al. (2014). Epicardial fat thickness in stable coronary artery disease: its relationship with high-sensitive cardiac troponin T and N-terminal pro-brain natriuretic peptide. Coron. Artery Dis. 25 (8), 685–690. doi:10.1097/MCA.0000000000000140
Broekman M. J., Eiroa A. M., Marcus A. J. (1991). Inhibition of human platelet reactivity by endothelium-derived relaxing factor from human umbilical vein endothelial cells in suspension: blockade of aggregation and secretion by an aspirin-insensitive mechanism. Blood 78 (4), 1033–1040. doi:10.1182/blood.v78.4.1033.1033
Cao J., Zheng Y., Luo Z., Mei Z., Yao Y., Liu Z., et al. (2020). Myocardial injury and COVID-19: serum hs-cTnI level in risk stratification and the prediction of 30-day fatality in COVID-19 patients with no prior cardiovascular disease. Theranostics 10 (21), 9663–9673. doi:10.7150/thno.47980
Chen B. H., Shi N. N., Wu C. W., An D. A., Shi Y. X., Wesemann L. D., et al. (2021). Early cardiac involvement in patients with acute COVID-19 infection identified by multiparametric cardiovascular magnetic resonance imaging. Eur. Heart J. Cardiovasc Imaging 22 (8), 844–851. doi:10.1093/ehjci/jeab042
Chung M. K., Zidar D. A., Bristow M. R., Cameron S. J., Chan T., Harding C. V., et al. (2021). COVID-19 and cardiovascular disease: from bench to bedside. Circ. Res. 128 (8), 1214–1236. doi:10.1161/CIRCRESAHA.121.317997
Conte C., Esposito A., De Lorenzo R., Di Filippo L., Palmisano A., Vignale D., et al. (2021). Epicardial adipose tissue characteristics, obesity and clinical outcomes in COVID-19: a post-hoc analysis of a prospective cohort study. Nutr. Metab. Cardiovasc Dis. 31 (7), 2156–2164. doi:10.1016/j.numecd.2021.04.020
Emekli E., Türkkanı M. H., S Ballı S. (2022). Is there a relationship between epicardial adipose tissue, inflammatory markers and prognosis in COVID-19 in patients under 65 years? Biomark. Med. 16 (12), 925–933. doi:10.2217/bmm-2022-0237
Erdöl M. A., Civelek Eser F., Aslan A. N., Erdoğan M., Altunsoy Aypak A., Beşler M. S., et al. (2022). The predictive value of epicardial fat volume for clinical severity of COVID-19. Rev. Port. Cardiol. 41 (9), 729–737. doi:10.1016/j.repc.2021.05.016
Gong J., Chen Y., Jie Y., Tan M., Jiang Z., Yuan L., et al. (2021). U-shaped relationship of low-density lipoprotein cholesterol with risk of severe COVID-19 from a multicenter pooled analysis. Front. Cardiovasc Med. 8, 604736. doi:10.3389/fcvm.2021.604736
Gu S. X., Tyagi T., Jain K., Gu V. W., Lee S. H., Hwa J. M., et al. (2021). Thrombocytopathy and endotheliopathy: crucial contributors to COVID-19 thrombo inflammation. Nat. Rev. Cardiol. 18 (3), 194–209. doi:10.1038/s41569-020-00469-1
Halushka M. K., Vander Heide R. S. (2021). Myocarditis is rare in COVID-19 autopsies: cardiovascular findings across 277 postmortem examinations. Cardiovasc Pathol. 50, 107300. doi:10.1016/j.carpath.2020.107300
Iacobellis G. (2022). Epicardial adipose tissue in contemporary cardiology. Nat. Rev. Cardiol. 19 (9), 593–606. doi:10.1038/s41569-022-00679-9
Joffre J., Rodriguez L., Matthay Z. A., Lloyd E., Fields A. T., Bainton R. J., et al. (2022). COVID-19-associated lung microvascular endotheliopathy: a "from the bench" perspective. Am. J. Respir. Crit. Care Med. 206 (8), 961–972. doi:10.1164/rccm.202107-1774OC
Karampetsou N., Tzani A., Doulamis I. P., Bletsa E., Minia A., Pliaka V., et al. (2022). Epicardial adipocyte-derived TNF-α modulates local inflammation in patients with advanced coronary artery disease. Curr. Vasc. Pharmacol. 20 (1), 87–93. doi:10.2174/1570161119666211029110813
Lasbleiz A., Gaborit B., Soghomonian A., Bartoli A., Ancel P., Jacquier A., et al. (2021). COVID-19 and obesity: role of ectopic visceral and epicardial adipose tissues in myocardial injury. Front. Endocrinol. (Lausanne) 12, 726967. doi:10.3389/fendo.2021.726967
Levi M., Thachil J., Iba T., Levy J. H. (2020). Coagulation abnormalities and thrombosis in patients with COVID-19. Lancet Haematol. 7 (6), 438–e440. doi:10.1016/S2352-3026(20)30145-9
Lewek J., Jatczak-Pawlik I., Maciejewski M., Jankowski P., Banach M. (2021). COVID-19 and cardiovascular complications - preliminary results of the LATE-COVID study. Arch. Med. Sci. 17 (3), 818–822. doi:10.5114/aoms/134211
Malavazos A. E., Goldberger J. J., Iacobellis G. (2020). Does epicardial fat contribute to COVID-19 myocardial inflammation? Eur. Heart J. 41 (24), 2333. doi:10.1093/eurheartj/ehaa471
Marcucci M., Fogante M., Tagliati C., Papiri G. (2022). Cut-off point of CT-assessed epicardial adipose tissue volume for predicting worse clinical burden of SARS-CoV-2 pneumonia. Emerg. Radiol. 29 (4), 645–653. doi:10.1007/s10140-022-02059-9
Mehta R., Bello-Chavolla O. Y., Mancillas-Adame L., Rodriguez-Flores M., Pedraza N. R., Encinas B. R., et al. (2022). Epicardial adipose tissue thickness is associated with increased COVID-19 severity and mortality. Int. J. Obes. (Lond). 46 (4), 866–873. doi:10.1038/s41366-021-01050-7
Nakanishi K., Fukuda S., Tanaka A., Otsuka K., Taguchi H., Shimada K. (2017). Relationships between periventricular epicardial adipose tissue accumulation, coronary microcirculation, and left ventricular diastolic dysfunction. Can. J. Cardiol. 33 (11), 1489–1497. doi:10.1016/j.cjca.2017.08.001
Packer M. (2018). Epicardial adipose tissue may mediate deleterious effects of obesity and inflammation on the myocardium. J. Am. Coll. Cardiol. 71 (20), 2360–2372. doi:10.1016/j.jacc.2018.03.509
Parasher A. (2021). COVID-19: current understanding of its pathophysiology, clinical presentation and treatment. Postgrad. Med. J. 97 (1147), 312–320. doi:10.1136/postgradmedj-2020-138577
Patel T., Kelleman M., West Z., Peter A., Dove M., Butto A., et al. (2022). Comparison of multisystem inflammatory syndrome in children-related myocarditis, classic viral myocarditis, and COVID-19 vaccine-related myocarditis in children. J. Am. Heart Assoc. 11 (9), e024393. doi:10.1161/JAHA.121.024393
Pellicori P., Doolub G., Wong C. M., Lee K. S., Mangion K., Ahmad M., et al. (2021). COVID-19 and its cardiovascular effects: a systematic review of prevalence studies. Cochrane Database Syst. Rev. 3 (3), CD013879. doi:10.1002/14651858.CD013879
Rohani-Rasaf M., Mirjalili K., Vatannejad A., Teimouri M. (2022). Are lipid ratios and triglyceride-glucose index associated with critical care outcomes in COVID-19 patients? PLoS One 17 (8), e0272000. doi:10.1371/journal.pone.0272000
Salyer S. J., Maeda J., Sembuche S., Kebede Y., Tshangela A., Moussif M., et al. (2021). The first and second waves of the COVID-19 pandemic in Africa: a cross-sectional study. Lancet 397 (10281), 1265–1275. doi:10.1016/S0140-6736(21)00632-2
Sandoval Y., Januzzi J. L., Jaffe A. S. (2020). Cardiac troponin for assessment of myocardial injury in COVID-19: JACC review topic of the week. J. Am. Coll. Cardiol. 76 (10), 1244–1258. doi:10.1016/j.jacc.2020.06.068
Sever P. S., Poulter N. R., Chang C. L., Thom S. A., Hughes A. D., Welsh P., et al. (2013). Evaluation of C-reactive protein before and on-treatment as a predictor of benefit of atorvastatin: a cohort analysis from the Anglo-Scandinavian Cardiac Outcomes Trial lipid-lowering arm. J. Am. Coll. Cardiol. 62 (8), 717–729. doi:10.1016/j.jacc.2013.02.098
Shchendrygina A., Nagel E., Puntmann V. O., Valbuena-Lopez S. (2021). COVID-19 myocarditis and prospective heart failure burden. Expert Rev. Cardiovasc Ther. 19 (1), 5–14. doi:10.1080/14779072.2021.1844005
Vuorio A., Raal F., Kovanen P. T. (2023). Familial hypercholesterolemia: the nexus of endothelial dysfunction and lipoprotein metabolism in COVID-19. Curr. Opin. Lipidol. 34 (3), 119–125. doi:10.1097/MOL.0000000000000876
Weckbach L. T., Curta A., Bieber S., Kraechan A., Brado J., Hellmuth J. C., et al. (2021). Myocardial inflammation and dysfunction in COVID-19-associated myocardial injury. Circ. Cardiovasc Imaging 14 (1), e012220. doi:10.1161/CIRCIMAGING.120.011713
Werlein C., Ackermann M., Stark H., Shah H. R., Tzankov A., Haslbauer J. D., et al. (2023). Inflammation and vascular remodeling in COVID-19 hearts. Angiogenesis 26 (2), 233–248. doi:10.1007/s10456-022-09860-7
Xu L., Xu Y., Coulden R., Sonnex E., Hrybouski S., Paterson I., et al. (2018). Comparison of epicardial adipose tissue radiodensity threshold between contrast and non-contrast enhanced computed tomography scans: a cohort study of derivation and validation. Atherosclerosis 275, 74–79. doi:10.1016/j.atherosclerosis.2018.05.013
Keywords: epicardial adipose tissue, COVID-19, myocardial injury, cTnI, LDL-C
Citation: Su T, Zhong B, Tang C, Qiao S, Feng Y, Peng H and Gu X (2024) Correlation between epicardial adipose tissue and myocardial injury in patients with COVID-19. Front. Physiol. 15:1368542. doi: 10.3389/fphys.2024.1368542
Received: 01 February 2024; Accepted: 08 April 2024;
Published: 19 April 2024.
Edited by:
Tizhong Shan, Zhejiang University, ChinaReviewed by:
Moni Nader, Khalifa University, United Arab EmiratesCopyright © 2024 Su, Zhong, Tang, Qiao, Feng, Peng and Gu. This is an open-access article distributed under the terms of the Creative Commons Attribution License (CC BY). The use, distribution or reproduction in other forums is permitted, provided the original author(s) and the copyright owner(s) are credited and that the original publication in this journal is cited, in accordance with accepted academic practice. No use, distribution or reproduction is permitted which does not comply with these terms.
*Correspondence: Xiaosong Gu, eGlhb3NvbmdndUBzdWRhLmVkdS5jbg==
†These authors have contributed equally to this work
Disclaimer: All claims expressed in this article are solely those of the authors and do not necessarily represent those of their affiliated organizations, or those of the publisher, the editors and the reviewers. Any product that may be evaluated in this article or claim that may be made by its manufacturer is not guaranteed or endorsed by the publisher.
Research integrity at Frontiers
Learn more about the work of our research integrity team to safeguard the quality of each article we publish.