- 1State Key Laboratory for Biology of Plant Diseases and Insect Pests, Institute of Plant Protection, Chinese Academy of Agricultural Sciences, Beijing, China
- 2National Nanfan Research Institute, Chinese Academy of Agricultural Sciences, Sanya, China
- 3Henan Agricultural University, Henan, China
- 4Guangxi Key Laboratory for Biology of Crop Diseases and Insect Pests, Institute of Plant Protection, Guangxi Academy of Agricultural Sciences, Nanning, China
- 5Hubei Insect Resources Utilization and Sustainable Pest Management Key Laboratory, College of Plant Science and Technology, Huazhong Agricultural University, Wuhan, China
The melon fly, Bactrocera cucurbitae (Coquillett) (Tephritidae: Diptera), is an invasive pest that poses a significant threat to agriculture in Africa and other regions. Flies are known to use their olfactory systems to recognise environmental chemical cues. However, the molecular components of the chemosensory system of B. cucurbitae are poorly characterised. To address this knowledge gap, we have used next-generation sequencing to analyse the antenna transcriptomes of sexually immature B. cucurbitae adults. The results have identified 160 potential chemosensory genes, including 35 odourant-binding proteins (OBPs), one chemosensory protein (CSP), three sensory neuron membrane proteins (SNMPs), 70 odourant receptors (ORs), 30 ionotropic receptors (IRs), and 21 gustatory receptors (GRs). Quantitative real-time polymerase chain reaction quantitative polymerase chain reaction was used to validate the results by assessing the expression profiles of 25 ORs and 15 OBPs. Notably, high expression levels for BcucOBP5/9/10/18/21/23/26 were observed in both the female and male antennae. Furthermore, BcucOROrco/6/7/9/13/15/25/27/28/42/62 exhibited biased expression in the male antennae, whereas BcucOR55 showed biased expression in the female antennae. This comprehensive investigation provides valuable insights into insect olfaction at the molecular level and will, thus, help to facilitate the development of enhanced pest management strategies in the future.
Introduction
The olfactory system is essential for insect reproduction and survival (Song et al., 2020) and is involved in a wide variety of insect behaviours, including host location, identification of oviposition sites and mates, and avoidance of predators (Hansson and Stensmyr, 2011; Leal, 2013; Suh et al., 2014; Lin et al., 2015; Wang et al., 2016). Furthermore, the antennae, which house different types of sensilla (Steinbrecht, 1996; Shanbhag et al., 1999), serve as the primary organs for olfactory sensing and, thus, play a crucial role in a variety of different behaviours (Liu J. et al., 2021; Liu X. et al., 2021). Insect olfaction relies on the olfactory receptor neurones (ORNs) in the sensilla (Zhu et al., 2021). Odourant molecules reach the dendrites of ORNs through the sensillary lymph (Leitch et al., 2015; Hu et al., 2022), with the assistance of odourant-binding proteins (OBPs) and chemosensory proteins (Pelosi et al., 2014; Mei et al., 2018; Pelosi et al., 2018; Zhou et al., 2021). The OBP-odour complex is then transported to specific odourant receptor proteins (ORs) located on the dendritic membrane of ORNs (Christine et al., 2014; Andersson et al., 2013; Sun et al., 2020), triggering the conversion of chemical signals into electrical signals. These signals are eventually processed by the central nervous system (CNS), leading to appropriate insect behavioural responses (Wicher and Miazzi, 2021; Wu et al., 2022). Orco, a highly conserved olfactory co-receptor present in various insect species, plays a crucial role in OR localisation and odour signal reception (Fan et al., 2015; Li et al., 2016). During the odour-recognition process, ORs form tetramers with Orco, acting as channels for odour-gated ions in response to odourants and pheromones (Koutroumpa et al., 2016; Sun et al., 2019; Task et al., 2020). Other olfactory genes are involved in signal recognition and play a role in insect olfaction. Sensory neuron membrane proteins (SNMPs) belong to the CD36 protein family (Li et al., 2021) and are essential for sensory pheromone detection (Vogt et al., 2009; Cassau and Krieger, 2021). Ionotropic receptors (IRs), members of the ionotropic glutamate receptor (iGluR) gene family (Zhang et al., 2019), play a key role in odour identification, as well as in sensing acids, salts, aldehydes, ammonia, temperature, humidity, and taste (Chen et al., 2015; Enjin et al., 2016; Knecht et al., 2016; Frank et al., 2017). While gustatory receptors (GRs) are responsible for gustatory substance detection (Xing et al., 2021) and can be categorised as CO2, sugar, and bitter receptors (Kwon et al., 2007; Sato et al., 2011; Ning et al., 2016; Xu, 2020).
The melon fly, Bactrocera cucurbitae (Coquillett) (Diptera: Tephritidae) is native to India and is widely distributed in tropical and subtropical regions worldwide (Dhillon et al., 2005; Mir et al., 2014). Currently, B. cucurbitae control primarily relies on the use of insecticides. However, this approach is becoming increasingly ineffective due to the development of insecticide resistance and the negative impacts of pesticide residues on human health and the environment (Aktar et al., 2009; Curl et al., 2020; Shivaramu et al., 2022). To explore alternative control methods, it is crucial to understand the molecular mechanisms underlying fly behaviour. Female B. cucurbitae use host-plant volatiles as cues to locate suitable hosts (Vargas et al., 2018; Piñero et al., 2020; Jaime et al., 2021). Although the role of these volatiles in host-seeking behaviour has been documented, the molecular basis of chemoreception in B. cucurbitae remains poorly understood. The identification of olfaction-related genes, is thus required to enable the elucidation of the olfactory perception mechanism in this species.
In this investigation, transcriptomic analysis of the antennae from immature male and female adult B. cucurbitae was conducted. The analysis identified 160 candidate chemosensory genes, including 70 odourant receptors (ORs), 35 odourant-binding proteins (OBPs), one chemosensory protein (CSP), 30 ionotropic receptors (IRs), 21 gustatory receptors (GRs), and three sensory neuron membrane proteins (SNMPs). To further investigate the expression patterns of these genes, quantitative real-time polymerase chain reaction (qPCR) was performed for 15 OBPs and 25 ORs. The results provide a foundation for future functional studies on chemoreception in B. cucurbitae. Ultimately, understanding the molecular mechanisms involved in the fly olfactory system could contribute to the development of novel and sustainable approaches for pest control.
Materials and methods
Insect rearing
B. cucurbitae specimens were collected from rotting fruits in the fields surrounding cities in Guangxi province, China. They were then placed in rearing cages (30 × 30 × 30 cm) until they emerged as adults. These insects were maintained for over 20 generations under controlled conditions, including a temperature of 26°C ± 1°C, relative humidity of 70% ± 10%, and a photoperiod of 14 h light and 10 h dark (14L:10D). They were provided with water and a forage mixture consisting of yeast and sugar at a ratio of 1:2.
Newly emerged male and female adults that were sexually immature and between 4 and 6 days of age were selected for the experiments. The antennae of these individuals were carefully grasped by their roots using tweezers and transferred to Eppendorf tubes. Additionally, to study the gene expression profiles in the different tissues, samples of the male antennae (M-T), female antennae (F-T), head, thorax, abdomen, legs, and wings were also collected. All the collected samples were immediately frozen in liquid nitrogen and stored at −80°C until RNA extraction for further analysis.
RNA extraction, cDNA library construction, and sequencing
RNA was extracted from the collected samples using a TRIzol reagent kit (Invitrogen), according to the manufacturer’s instructions. The RNA quality was assessed using an Agilent 2,100 Bioanalyzer and agarose gel electrophoresis. Eukaryotic mRNA was enriched using oligo (dT) beads, whereas prokaryotic mRNA was enriched by removing the rRNA using the Ribo-Zero™ Magnetic Kit (Epicentre). The enriched mRNA was fragmented and reverse transcribed into cDNA using random primers. Second-strand cDNA synthesis was performed, and the resulting fragments were purified and sequenced by ligating Illumina adapters. The cDNA libraries were sequenced using the Illumina Novaseq6000 platform (Gene Denovo Biotechnology Co., Guangzhou, China).
Transcriptomic profiling
Clean reads were obtained using Perl scripts, as follows: (1) adapters were removed, (2) low-quality reads were removed (base numbers with a mass value Q ≤ 20 accounted for more than 50% of the whole read), (3) reads containing more than 10% N were removed, and (4) all A-base reads were removed. The Q20, Q30, GC content, and sequence duplication levels for the clean data were then determined. Genomic localisation analysis of the clean reads was mapped to B. cucurbitae using HISAT2 (Kim et al., 2015), and Stringtie (Pertea et al., 2015) was used to reconstruct the transcripts. RSEM (Li and Dewey, 2011) was used to calculate the expression levels of all genes in each sample.
Sequence analysis and phylogenetic analysis
The open reading frames (ORFs) of the candidate chemosensory genes were identified using the NCBI ORF finder (https://www.ncbi.nlm.nih.gov/orffinder/), and similarity searchers performed using the National Center for NCBI-BLAST server. The TMHMM Server Version 2.0 (http://www.cbs.dtu.dk/services/TMHMM) was used to predict TMDs, and Signal IP4.1 (http://www.cbs.dtu.dk/services/SignalP/) was used to predict signal peptide sequences. Amino acid sequence alignment was performed using the ClustalW method in MEGA v6.0. The OBP dataset contained 52 sequences from Drosophila melanogaster, 34 from Bactrocera correcta, 43 from Bactrocera dorsalis, 33 from Bactrocera latifrons, 25 from Zeugodacus tau, 26 from Anastrepha ludens, 33 from Rhagoletis pomonella, 28 from Calliphora stygia, and 35 from Ceratitis capitata. The OR dataset contained 37 sequences from D. melanogaster, 38 from B. correcta, 36 from B. dorsalis, 40 from B. latifrons, 46 from Bactrocera minax, 39 from Z. tau, 26 from A. ludens, 53 from R. pomonella, 50 from C. stygia, and 42 from C. capitata. The GR dataset contained 39 sequences from D. melanogaster, 6 from B. correcta, 18 from B. dorsalis, 18 from B. latifrons, 4 from Z. tau, 10 from A. ludens, 14 from R. pomonella, 4 from C. stygia, and 12 from C. capitata. The IR dataset contained 26 sequences from D. melanogaster, 9 from B. correcta, 11 from B. dorsalis, 10 from B. latifrons, 11 from Z. tau, 7 from A. ludens, 9 from R. pomonella, 7 from C. stygia, and 10 from C. capitata. The CSP dataset contained two sequences from D. melanogaster, 1 from B. correcta, 2 from B. dorsalis, 1 from B. latifrons, 1 from Z. tau, 1 from A. ludens, 1 from R. zephyria, 1 from R. pomonella, 1 from C. stygia, 1 from C. capitata, 1 from Procecidochares utilis, 1 from Lucilia sericata, 1 from Lucilia cuprina, and 1 from Bactrocera oleae. The SNMP dataset contained 2 sequences from D. melanogaster, 3 from B. correcta, 3 from B. dorsalis, 2 from B. latifrons, 3 from Z. tau, 1 from A. ludens, 2 from R. zephyria, 2 from R. pomonella, 3 from C. stygia, 2 from C. capitata, 2 from L. sericata, 2 from L. cuprina, 2 from B. oleae, and 2 from Bactrocera neohumeralis.
Phylogenetic trees were constructed using the neighbour-joining method and p-distance modelling, and the trees were adjusted using FigTree v1.4.4. The candidate chemosensory genes were named “BcucOBP”, “BcucCSP”, “BcucSNMP”, “BcucOR”, “BcucGR”, and “BcucIR”, followed by a numeral indicating the coding region length. All amino acid sequences from the B. cucurbitae and other insects used in the phylogenetic analyses are listed in Supplementary Material S3.
Quantitative real-time PCR validation
For expression verification, 25 ORs and 15 OBPs were selected based on their high abundance levels from fragments per kilobase of exon per million reads mapped (FPKM), as measured using (Supplementary Material S4). Total RNA was isolated from each tissue and normalised to a concentration of 1 μg/μL. First-strand cDNA synthesis was performed using a commercial kit (Transgen Biotech, Beijing, China). A qPCR assay was conducted using a 20 μL master mix containing TransStart® TipGreen qPCR SuperMix, cDNA templates, primers, and water. Real-time quantitative PCR was performed using an ABI 7500 instrument (Applied Biosystems). The PCR program consisted of cycles of denaturation, annealing, extension, and amplification (Transgen Biotech, Beijing, China). RPL32 was used as an internal reference for normalisation. Primers were designed using Primer Premier 5.0, and their efficiencies were verified. Each experiment included three biological and three technical replicates. The expression levels were quantified using the 2−ΔΔCT method, with RPL32 as the reference gene (Livak et al., 2001).
Statistical analysis
Data analysis was performed using the 2−ΔΔCT method, and data were analysed using SAS 9.0 (SAS Institute Inc., Cary, NC, USA). Statistical significance was analysed using ANOVA followed by Tukey’s multiple comparison test. Statistical significance was set at p < 0.05. All figures were generated using OriginPro 9.1 (Northampton, Massachusetts, USA).
Results
Transcriptome overview
The sequencing data generated for all samples in this study ranged from 37 to 42 million clean reads. Overall, the transcriptomic data were highly valid, with a minimum sample accuracy of 97%. The Q30 score, which represents a 0.1% base error rate, ranged from 92.95% to 100% (Supplementary Material S1). The Q20 score, which indicates a 1% base error rate, ranged from 97.23% to 100%. The GC content of the reads was determined to be 41.23%. The quality control measures ensured that all samples met the necessary criteria for the subsequent analyses.
After mapping the reads to the reference genome, an average total mapped value of approximately 87% was obtained for all samples (Supplementary Material S2). This indicated that a substantial proportion of the reads were successfully aligned to known genes. In total, 13,473 genes were identified from the sequencing data. These included 12,984 reference and 489 novel genes. The presence of novel genes suggests that previously uncharacterised genetic elements could be discovered in the melon fly transcriptome.
Furthermore, the high Pearson correlation coefficient values (R2 ≥ 0.97) observed for the three biological replicates for the male and female antennae indicated strong reproducibility between samples. This confirms the reliability of the experimental setup and strengthens confidence in the subsequent analysis and data interpretation.
Characterisation of odourant binding proteins
In this study, 35 candidate odourant binding protein (OBP) transcripts were identified in B. cucurbitae. These OBPs ranged in length from 130 to 313 amino acids. Sequence analysis revealed that 33 of these unigenes had putative full-length ORFs, 32 of which were predicted to contain a signal peptide. OBPs are highly conserved when compared to odourant receptors (ORs). The sequence alignment results demonstrated the presence of 16 classical OBPs with six conserved cysteines. These included BcucOBP3, BcucOBP4, BcucOBP7, BcucOBP8, BcucOBP12, BcucOBP13, BcucOBP25, BcucOBP26, BcucOBP27, BcucOBP29, BcucOBP30, BcucOBP31, BcucOBP32, BcucOBP33, BcucOBP34, and BcucOBP35 (Figure 1).
Phylogenetic analysis revealed that the B. cucurbitae OBPs formed distinct branches and shared homology with OBPs from other Diptera species. These branch groupings were supported by high bootstrap values, indicating robustness. Notably, BcucOBP23 was clustered with other Dipteran Lush OBPs, whereas BcucOBP27 formed a clade with OBP28a. Furthermore, BcucOBP9 and BcucOBP10 were clustered within the OBP84a branch, and BcucOBP13/32/34 were grouped in the OBP56 h branch. These specific groupings suggest the potential functional significance of OBPs in B. cucurbitae (Figure 2). Information including the unigene reference, length, and best Blastx hit for all 35 OBPs is listed in Table 1.
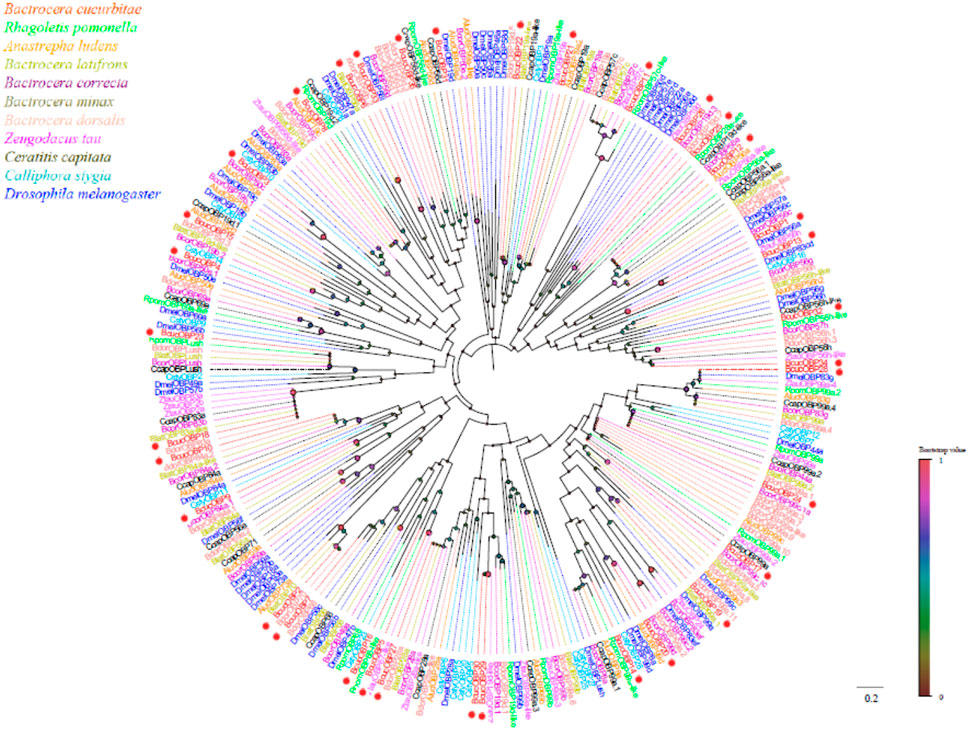
FIGURE 2. Neighbor joining phylogenetic tree of the candidate BcucOBPs with known Diptera OBP sequences.
Conserved residues are highlighted using white letters with a red background, alignment positions are framed in a blue box if the corresponding residues are identical or similar, and six conserved cysteine residues are labelled with red stars.
Rpom, Rhagoletis pomonella (N = 29); Alud, Anastrepha ludens (N = 22); Blat, Bactrocera latifrons (N = 32); Bcor, Bactrocera correcta (N = 34); Bdor, Bactrocera dorsalis (N = 42); Ztau, Zeugodacus tau (N = 24); Ccap, Ceratitis capitata (N = 35); Csty, Calliphora stygia (N = 19); Dmel, Drosophila melanogaster (N = 52). The candidate BcucOBPs are indicated by red circles.
In this study, a candidate chemosensory protein (CSP) transcript was identified for B. cucurbitae, which was 2,263 bp in length and encoded 573 amino acids. Furthermore, it was found to exhibit a characteristic protein domain (A10/OSD) typically found in the CSP family. Homology analysis revealed that all B. cucurbitae CSPs were clustered with CSPs from other Dipteran species (Figure 3). This clustering indicates their evolutionary relationships and suggests functional similarities among these CSPs. For detailed information on the identified CSPs, including the unigene reference, length, and best Blastx hit, please refer to Supplementary Material S5.
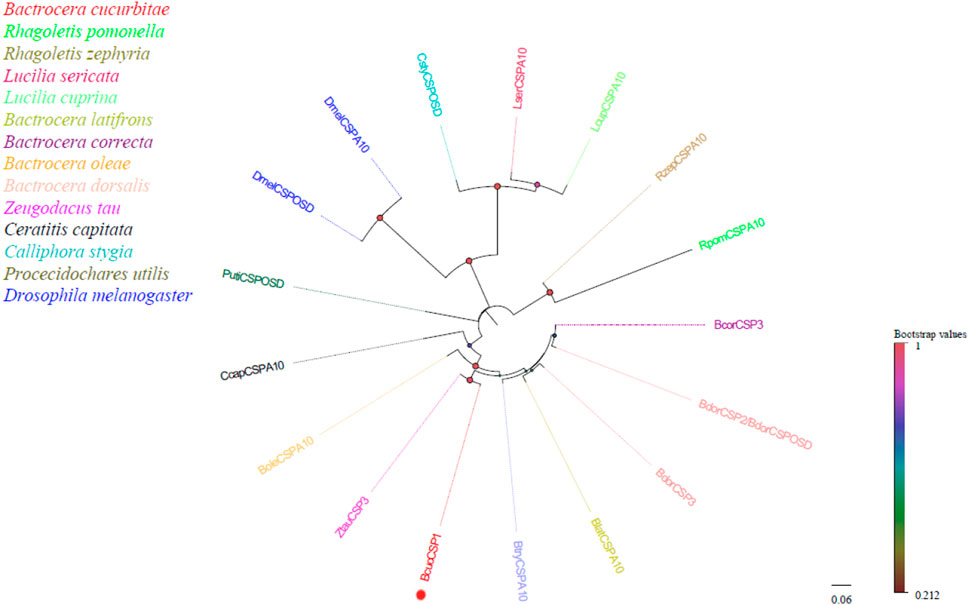
FIGURE 3. Neighbor joining phylogenetic tree of candidate BcucCSP with known Diptera CSP sequences. Rpom, Rhagoletis pomonella (N = 1); Rzep, Rhagoletis zephyria (N = 1); Lser, Lucilia sericata (N = 1); Lcup, Lucilia cuprina (N = 1); Blat, Bactrocera latifrons (N = 1); Bcor, Bactrocera correcta (N = 1); Bole, Bactrocera oleae (N = 1); Bdor, Bactrocera dorsalis (N = 1); Ztau, Zeugodacus tau (N = 1); Ccap, Ceratitis capitata (N = 1); Csty, Calliphora stygia (N = 1); Puti, Procecidochares utilis (N = 1); Dmel, Drosophila melanogaster (N = 2). Candidate BcucCSP is indicated by red circles.
Four candidate sensory neuron membrane protein (SNMP) transcripts were identified in the antennal transcriptome of B. cucurbitae. These SNMP transcripts varied in length, ranging from 1777 to 2,263 bp, and encoded proteins consisting of 496–573 amino acids. Conserved domain annotation indicated that these SNMPs belonged to the CD36 family, which is consistent with their known functional role, and phylogenetic analysis revealed that all B. cucurbitae SNMPs were clustered together with the SNMPs from other Diptera species (Figure 4). This clustering indicates evolutionary relatedness and suggests similar functions across these species. The SNMPs can be further classified into two major groups: SNMP1 and SNMP2. Within SNMP1, two subgroups have been identified: SNMP1a and SNMP1b. This classification provides a high level of insight into the potential functional diversity of the SNMP family. Information, including the unigene reference, length, and best Blastx hits for all SNMPs, is listed in Supplementary Material S5.
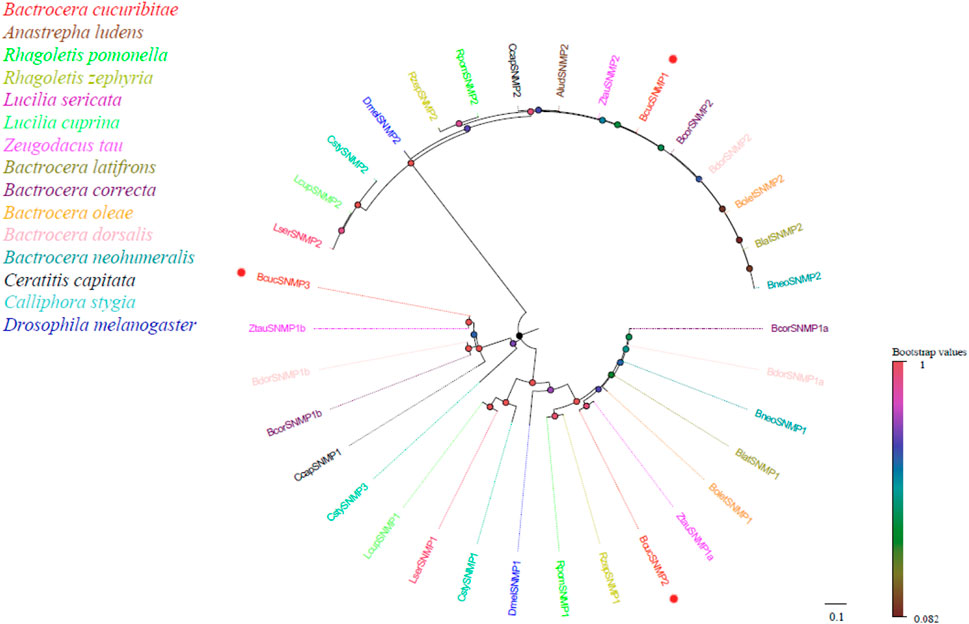
FIGURE 4. Neighbor joining phylogenetic tree of candidate BcucSNMPs with known Diptera SNMP sequences.
Alud, Anastrepha ludens (N = 1); Rpom, Rhagoletis pomonella (N = 2); Rzep, Rhagoletis zephyria (N = 2); Lser, Lucilia sericata (N = 2); Lcup, Lucilia cuprina (N = 2); Ztau, Zeugodacus tau (N = 3); Blat, Bactrocera latifrons (N = 2); Bcor, Bactrocera correcta (N = 3); Bole, Bactrocera oleae (N = 2); Bdor, Bactrocera dorsalis (N = 3); Bneo, Bactrocera neohumeralis (N = 2); Ccap, Ceratitis capitata (N = 2); Csty, Calliphora stygia (N = 3); Dmel, Drosophila melanogaster (N = 2). The candidate BcucSNMPs are indicated by red circles.
Seventy OR transcripts were identified in the antennal transcriptome of B. cucurbitae. Among these, 61 putative ORs were predicted to have a full-length ORFs ranging from 233 to 757 amino acids. These ORs have two-eight transmembrane domains which are characteristic of odourant receptors. Phylogenetic analysis revealed that BcucORs display a high degree of differentiation, with most clustering into different clades (Figure 5). These clades were well supported by high bootstrap values, indicating a robust grouping. Notably, the Orco branch exhibited relatively high conservation as it possessed a complete ORF and seven transmembrane domains, consistent with the typical characteristics of ORs. BcucOrco, the orthologue of Orco in other insects, clustered with Orco from various species, such as ZtauOrco, BlatOrco, BcorOrco, BminOrco, BdorOrco, CcapOrco, CstyOrco, RpomOrco, AludOrco, and DmelOrco. Furthermore, a specific increase in the number of ORs has been observed in B. cucurbitae. These expansions were evident in branches, such as BcucOR2/8/29/35/38/67, BcucOR45, and BcucOR48, suggesting potential functional diversification within these specific receptor groups. Additional information, including the unigene reference, length, and best Blastx hits for all identified ORs, is shown in Table 2.
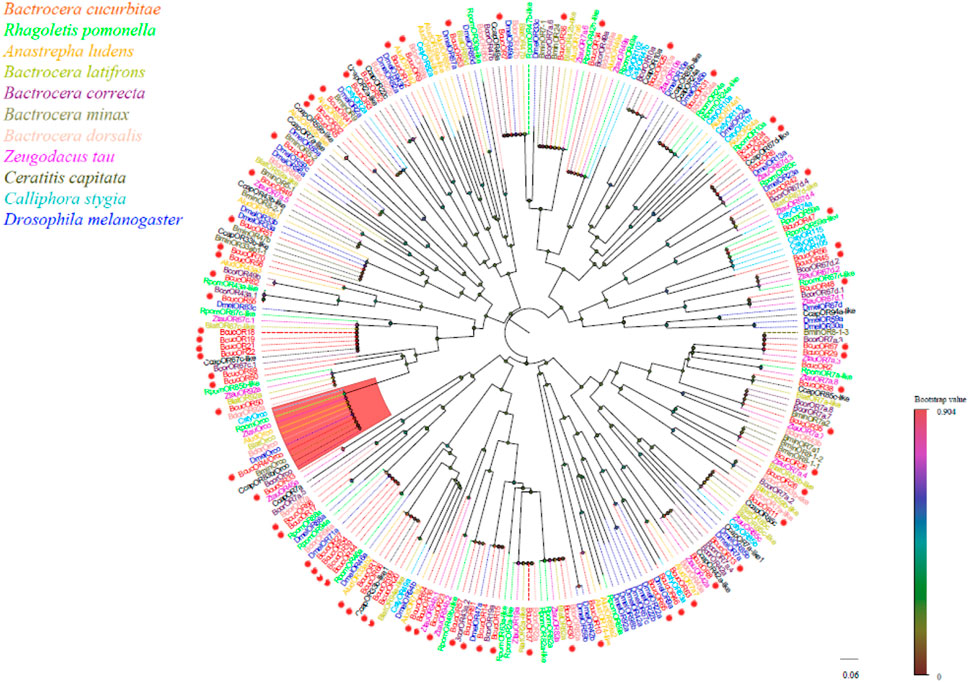
FIGURE 5. Neighbour joining phylogenetic tree for the candidate BcucORs with known Diptera OR sequences. Rpom, Rhagoletis pomonella (N = 26); Alud, Anastrepha ludens (N = 18); Blat, Bactrocera latifrons (N = 16); Bcor, Bactrocera correcta (N = 34); Bmin, Bactrocera minax (N = 14); Bdor, Bactrocera dorsalis (N = 18); Ztau, Zeugodacus tau (N = 22); Ccap, Ceratitis capitata (N = 20); Csty, Calliphora stygia (N = 18); Dmel, Drosophila melanogaster (N = 37). The candidate BcucORs are indicated by red circles.
Twenty-one GR transcripts in the antennal transcriptome of B. cucurbitae were identified. Of these, 10 BcucGRs were found to have putative full-length open reading frames (ORFs) with six to seven transmembrane domains. These ORFs encode proteins ranging from 330 to 434 amino acids in length.
Based on phylogenetic analysis, BcucGRs were classified into three subfamilies: sugar, bitter, and saponin receptors (Figure 6). Within these subfamilies, specific clustering patterns were observed among the BcucGRs. For instance, BcucGR7/16/21 showed homology and clustered with the GR43a sugar receptors, while the BcucGR3 and BcucGR18 were grouped with the GR64a, GR64c, GR64e, and GR64f sugar receptors. Furthermore, BcucGR8 was as a GR10a sugar receptor and BcucGR5/14/15/17/19 were identified as co-receptors of BcucGRs and clustered with the GR22a-f sugar receptors. BcucGR12 and BcucGR20 were also identified as homologues of GR21a, which mediates CO2 recognition, while BcucGR1/2/9/10 correspond to the GR66a, GR33a, and GR93a bitter receptors, respectively. Notably, BcucGR4 formed a GR28b branch, indicating its classification as a saponin receptor. For detailed information on the identified GRs, including the unigene reference, length, and best Blastx hit, please refer to Supplementary Material S5.
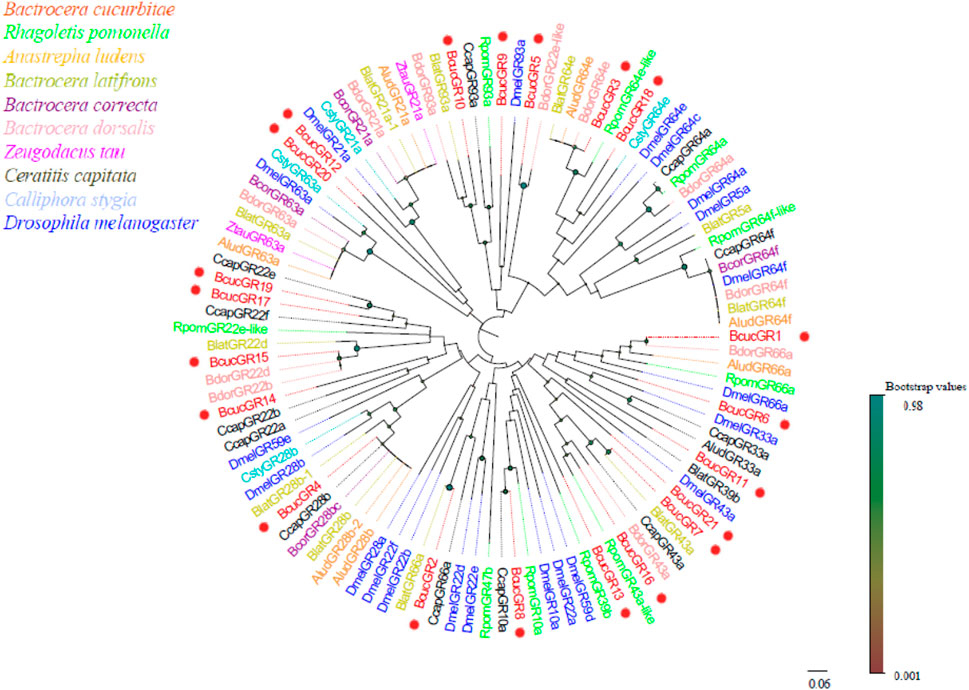
FIGURE 6. Neighbour joining phylogenetic tree showing the candidate BcucGRs with known Diptera GR sequences. Rpom, Rhagoletis pomonella (N = 26); Alud, Anastrepha ludens (N = 20); Blat, Bactrocera latifrons (N = 25); Bcor, Bactrocera correcta (N = 8); Bdor, Bactrocera dorsalis (N = 34); Ztau, Zeugodacus tau (N = 6); Ccap, Ceratitis capitata (N = 8); Csty, Calliphora stygia (N = 4); Dmel, Drosophila melanogaster (N = 59). The candidate BcucGRs are indicated by red circles.
Thirty IR transcripts were identified in the antennal transcriptome of B. cucurbitae, and they ranged from 1,249 to 5,216 bp in length, and encode proteins with 365–1,425 amino acids. Phylogenetic analysis revealed that the BcucIRs clustered together with other IRs, indicating their evolutionary relatedness (Figure 7). The phylogenetic tree showed that IR genes exhibited relatively close evolutionary distances, suggesting a certain degree of conservation. Specifically, BcucIR2/3/6/9/10/11/12/13/16/17/18/23/28/30 were found to cluster with IRkainate clades in the phylogenetic tree, while BcucIR4 and BcucIR14 formed the IRNMDA branch. BcucIR5 and BcucIR7 were associated with the coreceptors IR25a and IR8a, respectively, and clustered with conserved branches. BcucIR1 clustered with DmelIR64a, BcucIR21 clustered with DmelIR84a, and BcucIR25/26/29 were grouped with IR75a. Information, including the unigene reference, length, and best BlastX hit for all identified IRs can be found in Supplementary Material S5.
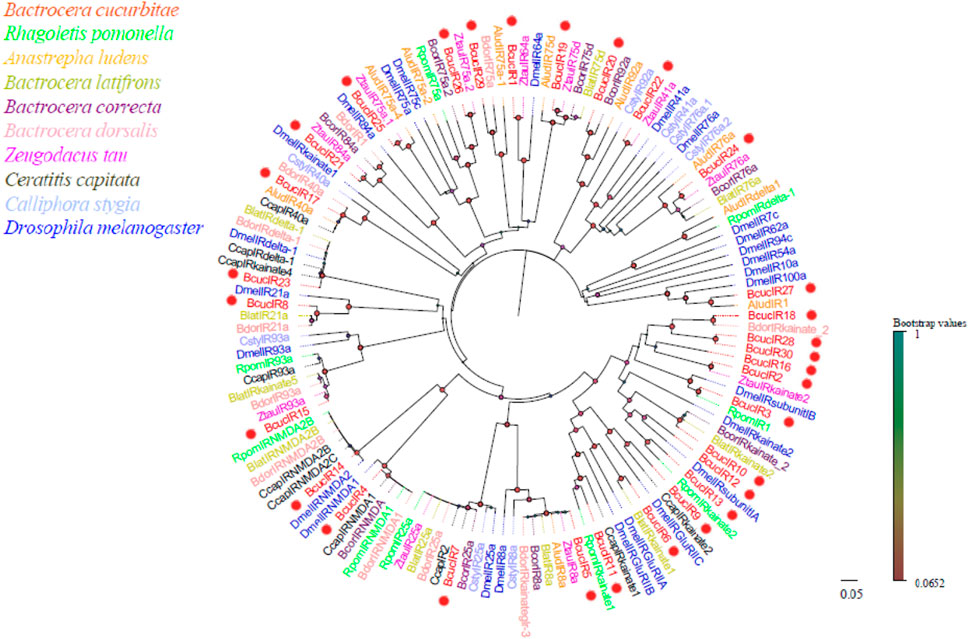
FIGURE 7. Neighbour joining phylogenetic tree for the candidate BcucIRs with known Diptera IR sequences. Rpom, Rhagoletis pomonella (N = 9); Alud, Anastrepha ludens (N = 10); Blat, Bactrocera latifrons (N = 10); Bcor, Bactrocera correcta (N = 9); Bdor, Bactrocera dorsalis (N = 11); Ztau, Zeugodacus tau (N = 11); Ccap, Ceratitis capitata (N = 10); Csty, Calliphora stygia (N = 8); Dmel, Drosophila melanogaster (N = 26). The candidate BcucIRs are indicated by red circles.
RT-qPCR analysis of the 25 ORs and 15 OBPs in different B. cucurbitae tissues, including the male and female antennae, heads, thorax, abdomen, legs, and wings, revealed distinct tissue expression profiles.
Among the OBPs, BcucOBP5/9/10/18/21/23/26 showed significantly higher expression in the male and female antennae than in other tissues. BcucOBP13 exhibited the highest expression in the head and legs, whereas BcucOBP24 and BcucOBP31 were significantly more highly expressed in the head and BcucOBP27 and BcucOBP30 were significantly expressed in the legs (Figure 8).
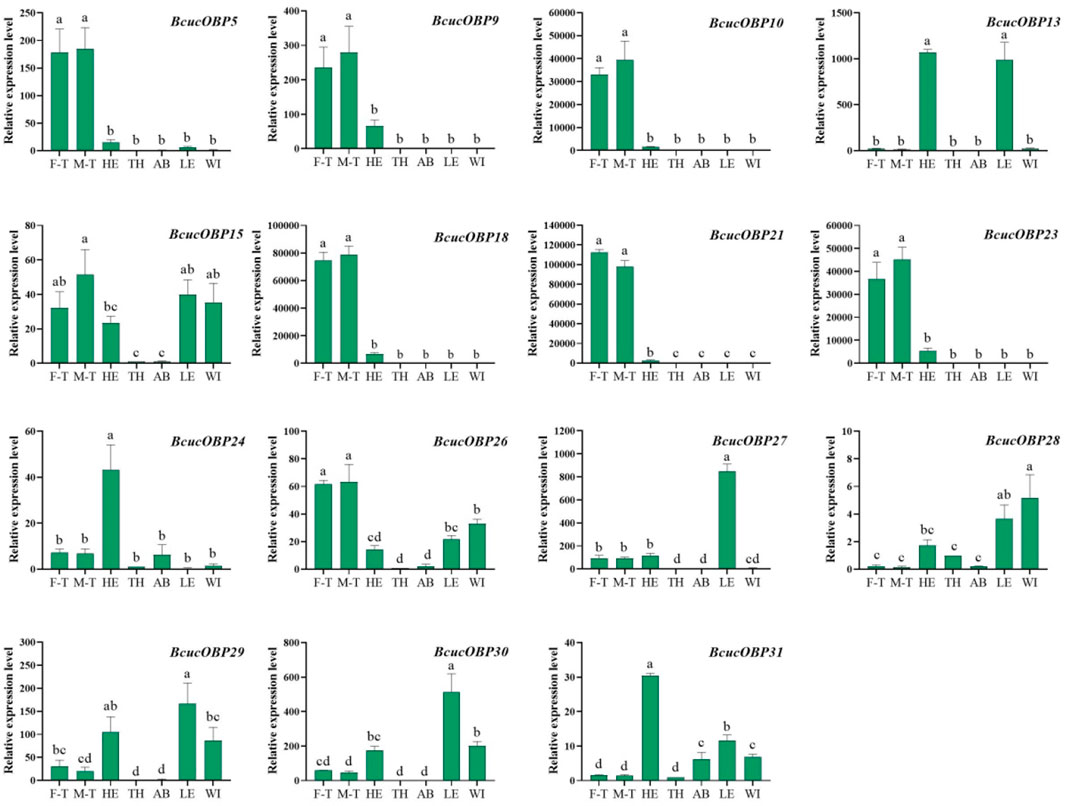
FIGURE 8. Expression profiles for 15 OBPs in adult male antennae (M–T), female antennae (F–T), head (HE), thorax (TH), abdomen (AB), legs (LE), and wings (WI) using qPCR. The relative expression level is indicated as the mean ± SE (N = 3). Different capital letters indicate significant difference between tissues (p < 0.05, ANOVA, LSD).
In terms of OR expression, BcucOR23 was significantly expressed in the wings, BcucOR35 showed significantly higher expression in the antennae and thoraxes, and BcucOR48 was significantly expressed in the antennae and wings. BcucOR18 and BcucOR30 were expressed in all the examined tissues. The remaining ORs are predominantly expressed in the antennae. Among them, the expression levels of BcucOrco/6/7/9/13/15/25/27/28/42/62 were significantly higher in the male than in the female antennae. In contrast, BcucOR55 exhibited a significantly higher level of expression in the female antennae when compared with the male antennae (Figure 9).
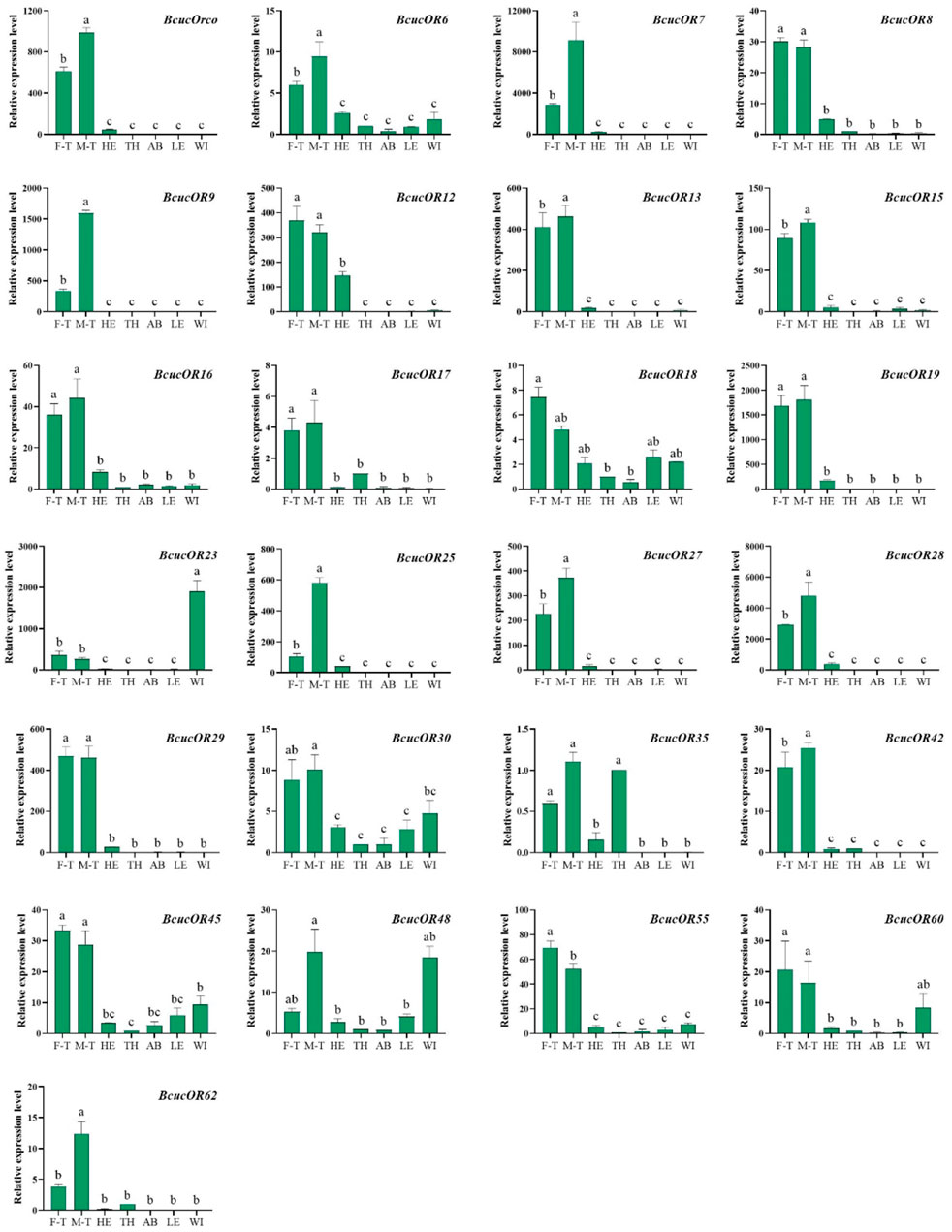
FIGURE 9. Expression profiles for 25 ORs in adult male antennae (M–T), female antennae (F–T), head (HE), thorax (TH), abdomen (AB), legs (LE), and wings (WI) using qPCR. The relative expression level is indicated as mean ± SE (N = 3). Different capital letters indicate significant differences between tissues (p < 0.05, ANOVA, LSD).
The results provide insights into the tissue-specific expression patterns of ORs and OBPs in B. cucurbitae and highlight their potential roles in olfactory perception and signalling.
Discussion
B. cucurbitae is a significant pest that feeds on plants and many countries now use quarantines to aid in its control (Shivaramu et al., 2022). Although some plant-based compounds have been identified that can help with the control and quarantine of this pest (Miller et al., 2004; Vargas et al., 2018; Piñero et al., 2020), research to elucidate the chemical communications in B. cucurbitae has been limited. B. cucurbitae olfaction research, in particular, has been hindered by the absence of transcriptome data for its primary olfactory organs, the antennae (Elfekih et al., 2016). While this study has tried to address this, only OBP and GR were reported on, and data on OR, which plays a key role in olfactory signal transduction, was lacking. There is thus a need for more complete transcriptome data to aid with future B. cucurbitae olfactory research.
The antenna is the major organ for insect olfactory sensing, and genes highly expressed in the antennae may be involved in olfactory recognition. The OBP involved in B. cucurbitae host selection can be screened by in vitor, purify and functionally verify the odourant binding protein in the antenna of B. cucurbitae, and this can provide a theoretical foundation for the development of B. cucurbitae attractants. Although Wu et al. (2020) carefully analysed and compared the transcriptomes of five Bactrocera species, the tissue-specific expression of B. cucurbitae was not fully elucidated. To address this, transcriptomic sequencing analysis of the antennae from adult male and female B. cucurbitae was conducted. In total, 160 potential olfactory receptor proteins were identified, including 70 ORs, 35 OBPs, 1 CSP, 30 IRs, 21 GRs, and 3 SNMPs. Compared with the previous results, this study found more OBP, OR, GR, less CSP and IR. Different genes found from those reported may be caused by transcriptome analysis methods and different strains of insects. These findings significantly enhance our understanding of the olfactory-related profile of B. cucurbitae and provide crucial insights into its chemosensory mechanisms. Additionally, we have employed quantitative polymerase chain reaction (qPCR) to validate the expression patterns of 25 ORs and 15 OBPs in various B. cucurbitae tissues, enabling the functional roles of these olfactory genes to be assessed.
The ability of an insect to recognise odours relies heavily on their OBPs (Barbosa et al., 2018), as these small, water-soluble proteins facilitate the transport of odour molecules through the sensory lymph and serve as connections between external environmental stimuli and odour receptors (Pelosi et al., 2006; Leal, 2013; Han et al., 2020). In this study, 35 candidate OBPs were identified in the antennal transcriptome of B. cucurbitae. This is lower than the number found in D. melanogaster (52 OBPs; Vieira and Rozas, 2011) but similar to the number found in Zeugodacus. papayae (35 OBPs; Wu et al., 2020), B. correcta (34 OBPs; Wu et al., 2020), A. ludens (31 OBPs; Segura-Leon et al., 2022), Z. tau (33 OBPs; Wu et al., 2020), and B. dorsalis (31 OBPs; Wu et al., 2020), and higher than that in C. stygia (28 OBPs; Leitch et al., 2015) and B. minax (25 OBPs; Cheng et al., 2020). Furthermore, the number of BcucOBPs was different from that previously reported (13 and 38 OBPs), and this wide variation in the number of OBPs may be attributed to the differences in the physiological stages during transcriptome analysis, sequencing methodologies, sequencing depth, sample preparation, and coverage of the sequencing platform (Wu et al., 2015; Liu et al., 2016; Cheng et al., 2020).
Phylogenetic analysis showed that BcucOBP23 forms a clade with other Dipteran LUSH proteins known to have a specific binding affinity for the pheromone 11-cis vaccenyl acetate. This suggests that BcucOBP23 is involved in pheromone recognition (Laughlin et al., 2008; Wu et al., 2020). Additionally, BcucOBP23 was highly expressed in both male and female antennae, indicating a potential role in pheromone recognition by melon flies. Furthermore, BcucOBP27 was also identified, and clustered with other Diptera species in the OBP28a clade and is associated with olfactory sensitivity modulation (Larter et al., 2016).
The expansion of OBP gene families is a common occurrence, and orthologues of the OBPs play similar roles in olfactory functions (Vieira et al., 2007; Rihani et al., 2021). In B. cucurbitae, the expansion of the OBP19d (BcucOBP8/15/25/26/29/30), OBP56a (BcucOBP6 and BcucOBP12), OBP56d (BcucOBP31/33/35), OBP56 h (BcucOBP13/32/34), OBP84a (BcucOBP9 and BcucOBP10), and OBP99a (BcucOBP14/19/24/28) branches was observed. In particular, OBP84a is involved in detecting organic acids and amines (Larter et al., 2016), while OBP56 h modulates mating behaviour (Swarup et al., 2011).
Previously, OBPs were thought to be exclusively expressed in olfactory organs and solely involved in chemoreception (Damberger et al., 2013; Shorter et al., 2016; Rihani et al., 2021). However, recent reports have shown that OBPs are expressed in multiple insect organs, including the legs (Yasukawa et al., 2010; Chen et al., 2019; Gu et al., 2019), proboscis (Mitaka et al., 2011; Rihani et al., 2016), labellum and tarsus (Jeong et al., 2013; Sparks et al., 2014), wings (Galindo and Smith, 2001; Calvello et al., 2005), reproductive organs (Findlay et al., 2008; Takemori and Yamamoto, 2009; Sun et al., 2012; Costa-Da-Silva et al., 2013; Sepil et al., 2019), abdomen (Xu et al., 2010; Andersson et al., 2014), eyes (Zhu et al., 2016; Rihani et al., 2021), and digestive tract (Ishida et al., 2013; Benoit et al., 2017). These OBPs reportedly have unconventional roles, including involvement in taste perception (Yasukawa et al., 2010; Jeong et al., 2013), immune response (Benoit et al., 2017), and humidity detection (Sun et al., 2018).
In this study, high expression levels for BcucOBP5/9/10/18/21/23/26 were observed in the antennae of both male and female B. cucurbitae. As few studies have investigated the functions of BcucOBPs, their functions in the host recognition of B. cucurbitae were investigated by gene cloning, construction of a prokaryotic expression vector, expression and purification of recombinant proteins, fluorescence competitive binding experiment, RNAi, and other technologies.
CSPs, another class of soluble proteins found in the sensory lymph, are also highly expressed in insects (Forêt et al., 2007). A CSP was identified in the study of the B. cucurbitae antennal transcriptome, and a BLASTX comparison revealed its high level of similarity to CSP genes in other insects. However, our understanding of the evolution and function of CSPs is currently limited to data from only a few species (Yi et al., 2013; Wu et al., 2019; Xu et al., 2019; Wang et al., 2021). Further comprehensive research on CSPs will thus be required to elucidate their diverse functions.
SNMPs were initially discovered in the pheromone-sensitive neurones of Lepidoptera (Rogers et al., 2001) and are associated with pheromone recognition (Vogt et al., 2009; Gomez-Diaz et al., 2016; Cassau and Krieger, 2021). They are generally categorised into two families, SNMP1 and SNMP2, with SNMP1 further divided into the SNMP1a and SNMP1b subtypes (Jiang et al., 2016). Three SNMPs were identified in the B. cucurbitae transcriptome, however, the function of BcucSNMPs in the sexual communication of B. cucurbitae needs to be further studied.
In the insect olfactory system, ORs are known to play a central role (Leal, 2013). B. cucurbitae has 70 putative ORs, which is similar to the number in D. melanogaster (63 ORs; Gao and Chess, 1999) and Anopheles gambiae (76 ORs; Hill et al., 2002), but higher than the number in Z. papayae (41 ORs; Wu et al., 2020), B. correcta (39 ORs; Wu et al., 2020), B. minax (53 ORs; Cheng et al., 2020), Z. tau (39 ORs; Wu et al., 2020), B. dorsalis (35 ORs; Wu et al., 2015), C. stygia (50 ORs; Leitch et al., 2015), Glossina morsitans (50 ORs; Obiero et al., 2014), and A. ludens (42 ORs; Segura-Leon et al., 2022). It seems that Bactrocera species requires homologous ORs to detect specific odorants. Highly differentiated and expanded ORs indicate the continuous evolution of insect odor detection. The expansion of OR7a, OR67d, and OR74a suggests their involvement in pheromone acceptance and insect behaviour. Tissue expression profiling has shown that all ORs are expressed in the antennae, with differences between the male and female antennae indicating their potential roles in sex pheromone recognition and other behaviours (Lin et al., 2015; Wu et al., 2020). Future studies should focus on the functions of these OR genes to provide potential molecular targets for the development of new insect behaviour regulators.
Phylogenetic tree analysis revealed that BcucOrco is clustered into a specific co-receptor lineage with other Dipteran species. The function of Orco has been studied in a large variety of insects, may be potentially involved in oviposition, mating, or social behavior (Fan et al., 2022; David et al., 2023; Liu et al., 2023). The olfactory function of Orco can be further confirmed by RNAi technology and EAG reaction analysis. For example, the mating rate and response to sex pheromone of BoleOrco decreased significantly after injecting dsRNA (Tsoumani et al., 2020). Xu et al. (2022) used the CRISPR/Cas9 system to knockout BdorOrco, olfactory preference assays and oviposition behavior are significantly affected. These research can provide a reference for the functional verification of BcucOrco.
There were 30 IRs identified in B. cucurbitae, which is fewer than in D. melanogaster (66 IRs; Rytz et al., 2013), but similar to the number in A. gambiae (30 IRs; Hill et al., 2002), Z. papayae (23 IRs; Wu et al., 2020), B. correcta (23 IRs; Wu et al., 2020), Z. tau (23 IRs; Wu et al., 2020), C. stygia (22 IRs; Leitch et al., 2015), B. dorsalis (23 IRs; Wu et al., 2020), and higher than the number in A. ludens (17 IRs) (Segura-León et al., 2022). BcucIR5 and BcucIR7 belong to the conserved IR25a/IR8a co-expression group. BcucIR1 may detect acids while BcucIR21 may recognise phenylacetic acid and phenylacetaldehyde, while BcucIR25/26/29 may be involved in acetic acid sensing and the enhanced perception of fermented fruits (Ai et al., 2010; Grosjean et al., 2011; Gorter et al., 2016).
There were 21 candidate GRs identified in B. cucurbitae, which is fewer than in D. melanogaster (59 GRs; Robertson et al., 2003), but similar to the number in A. ludens (24 GRs; Segura-León et al., 2022), and C. stygia (21 GRs; Leitch et al., 2015), and higher than the number in A. gambiae (10 GRs; Hill et al., 2002), Z. papayae (9 GRs; Wu et al., 2020), Z. tau (6 GRs; Wu et al., 2020), B. correcta (9 GRs; Wu et al., 2020), and B. dorsalis (6 GRs; Wu et al., 2015). Phylogenetic analysis grouped BcucGRs into different clades with specific putative functions. BcucGRs include sugar receptors (BcucGR3/5/7/8/14/15/16/17/18/19), bitter receptors (BcucGR1/2/7), CO2 receptors (BcucGR12 and BcucGR12), and saponin receptors (BcucGR4) (Lee et al., 2009; Apostolopoulou et al., 2016; Poudel and Lee, 2016; Rass et al., 2019; Sang et al., 2019).
In summary, the expression patterns and observed gene expansion for the ORs, IRs, and GRs indicates that they are involved in odour detection, pheromone reception, and host plant selection in B. cucurbitae (Kurtovic et al., 2007; Liu et al., 2018; MacWilliam et al., 2018; Rebecca and John, 2016; Ono et al., 2021; Baumgartner et al., 2022; De Obaldia et al., 2022).
Conclusion
The extensive set of chemosensory genes identified in this study will provide a solid foundation for future research into the molecular communications of B. cucurbitae and advances our understanding of olfactory mechanisms in Diptera. Moreover, the discovery of high antenna and sex-biased chemosensory expression proteins will also help to guide functional studies of key olfactory genes in B. cucurbitae.
Data availability statement
The datasets presented in this study can be found in online repositories. The names of the repository/repositories and accession number(s) can be found below: https://www.ncbi.nlm.nih.gov/bioproject/PRJNA1019880.
Ethics statement
The manuscript presents research on animals that do not require ethical approval for their study.
Author contributions
JW: Formal Analysis, Investigation, Writing–original draft. CM: Writing–review and editing. YY: Writing–review and editing, Methodology. JY: Writing–review and editing, Data curation. LC: Writing–review and editing, Methodology. YW: Writing–review and editing, Data curation. CZ: Writing–review and editing, Supervision. XG: Writing–review and editing, Funding acquisition. HC: Funding acquisition, Writing–review and editing. WM: Writing–review and editing, Data curation. ZZ: Writing–review and editing, Conceptualization.
Funding
The author(s) declare financial support was received for the research, authorship, and/or publication of this article. This study was supported by grant from the National program Key Basic Research Project (Grant No. 2022YFC2601400) and the project was supported by Nanfan special project, CAAS (Grant No. ZDXM2312).
Acknowledgments
We thank reviewers for many helpful suggestions of earlier versions of the manuscript. We are thankful to Guangxi Key Laboratory for Biology of Crop Diseases and Insect Pests, Institute of Plant Protection, Guangxi Academy of Agricultural Sciences for Providingthe insect. We thank State Key Laboratory for Biology of Plant Diseases and Insect Pests, Institute of Plant Protection, Chinese Academy of Agricultural Sciencesfor providing necessary instrumental facilities.
Conflict of interest
The authors declare that the research was conducted in the absence of any commercial or financial relationships that could be construed as a potential conflict of interest.
Publisher’s note
All claims expressed in this article are solely those of the authors and do not necessarily represent those of their affiliated organizations, or those of the publisher, the editors and the reviewers. Any product that may be evaluated in this article, or claim that may be made by its manufacturer, is not guaranteed or endorsed by the publisher.
Supplementary material
The Supplementary Material for this article can be found online at: https://www.frontiersin.org/articles/10.3389/fphys.2024.1354530/full#supplementary-material
References
Ai M., Min S., Grosjean Y., Leblanc C., Bell R., Benton R., et al. (2010). Acid sensing by the Drosophila olfactory system. Nature 468, 691–695. doi:10.1038/nature09537
Aktar M. W., Sengupta D., Chowdhury A. (2009). Impact of pesticides use in agriculture: their benefits and hazards. Interdiscip. Toxicol. 2 (1), 1–12. doi:10.2478/v10102-009-0001-7
Andersson M. N., Grosse-Wilde E., Keeling C. I., Bengtsson J. M., Yuen M. M., Li M., et al. (2013). Antennal transcriptome analysis of the chemosensory gene families in the tree killing bark beetles, Ips typographus and Dendroctonus ponderosae (Coleoptera: Curculionidae: scolytinae). BMC genomics 14, 198. doi:10.1186/1471-2164-14-198
Andersson M. N., Videvall E., Walden K. K., Harris M. O., Robertson H. M., Löfstedt C. (2014). Sex- and tissue-specific profiles of chemosensory gene expression in a herbivorous gall-inducing fly (Diptera: cecidomyiidae). BMC Genomics 15, 501. doi:10.1186/1471-2164-15-501
Andrews J. C., Fernández M. P., Yu Q., Leary G. P., Leung A. K., Kavanaugh M. P., et al. (2014). Octopamine neuromodulation regulates Gr32a-linked aggression and courtship pathways in Drosophila males. PLoS Genet. 10 (5), e1004356. doi:10.1371/journal.pgen.1004356
Apostolopoulou A. A., Köhn S., Stehle B., Lutz M., Wüst A., Mazija L., et al. (2016). Caffeine taste signaling in Drosophila larvae. Front. Cell. Neurosci. 10, 193. doi:10.3389/fncel.2016.00193
Barbosa A. J. M., Oliveira A. R., Roque A. C. A. (2018). Protein- and peptide-based biosensors in artificial olfaction. Trends Biotechnol. 36 (12), 1244–1258. doi:10.1016/j.tibtech.2018.07.004
Baumgartner M. E., Mastrogiannopoulos A., Kucinski I., Langton P. F., Piddini E. (2022). The Gr64 cluster of gustatory receptors promotes survival and proteostasis of epithelial cells in Drosophila. PLoS Biol. 20 (7), e3001710. doi:10.1371/journal.pbio.3001710
Benoit J. B., Vigneron A., Broderick N. A., Wu Y., Sun J. S., Carlson J. R., et al. (2017). Symbiont-induced odorant binding proteins mediate insect host hematopoiesis. eLife 6, e19535. doi:10.7554/eLife.19535
Calvello M., Brandazza A., Navarrini A., Dani F., Turillazzi S., Felicioli A., et al. (2005). Expression of odorant-binding proteins and chemosensory proteins in some Hymenoptera. Insect biochem. Mol. Biol. 35, 297–307. doi:10.1016/j.ibmb.2005.01.002
Cassau S., Krieger J. (2021). The role of SNMPs in insect olfaction. Cell Tissue Res. 383, 21–33. doi:10.1007/s00441-020-03336-0
Chen C., Buhl E., Xu M., Croset V., Rees J. S., Lilley K. S., et al. (2015). Drosophila ionotropic receptor 25a mediates circadian clock resetting by temperature. Nature 527, 516–520. doi:10.1038/nature16148
Chen X. F., Xu L., Zhang Y. X., Wei D., Wang J. J., Jiang H. B. (2019). Genome-wide identification and expression profiling of odorant-binding proteins in the oriental fruit fly, Bactrocera dorsalis. Bactrocera Dorsalis. Comp. Biochem. Physiol. Part D. Genomics Proteomics. Sep. 31, 100605. doi:10.1016/j.cbd.2019.100605
Cheng J. F., Yu T., Chen Z. J., Chen S., Chen Y. P., Gao L., et al. (2020). Comparative genomic and transcriptomic analyses of chemosensory genes in the citrus fruit fly Bactrocera (Tetradacus) minax. Sci. Rep. 10 (1), 18068. doi:10.1038/s41598-020-74803-5
Costa-da-Silva A. L., Kojin B. B., Marinotti O., James A. A., Capurro M. L. (2013). Expression and accumulation of the two-domain odorant-binding protein AaegOBP45 in the ovaries of blood-fed Aedes aegypti. Parasit. Vectors 6, 364. doi:10.1186/1756-3305-6-364
Curl C. L., Spivak M., Phinney R., Montrose L. (2020). Synthetic pesticides and health in vulnerable populations: agricultural workers. Curr. Environ. Health Rep. Mar. 7 (1), 13–29. doi:10.1007/s40572-020-00266-5
Damberger F. F., Michel E., Ishida Y., Leal W. S., Wüthrich K. (2013). Pheromone discrimination by a pH-tuned polymorphism of the Bombyx mori pheromone-binding protein. Proc. Natl. Acad. Sci. U. S. A. Nov. 12 110 (46), 18680–18685. doi:10.1073/pnas.1317706110
David O. G., Sanchez K. M., Arce A. V., Costa-da-Silva A. L., Bellantuono A. J., DeGennaro M. (2023). Fertility decline in female mosquitoes is regulated by the orco olfactory co-receptor. iScience 26 (6), 106883. doi:10.1016/j.isci.2023.106883
De Obaldia M. E., Morita T., Dedmon L. C., Boehmler D. J., Jiang C. S., Zeledon E. V., et al. (2022). Differential mosquito attraction to humans is associated with skin-derived carboxylic acid levels. Cell 185 (22), 4099–4116. doi:10.1016/j.cell.2022.09.034
Dhillon M. K., Singh R., Naresh J. S., Sharma H. C. (2005). The melon fruit fly, Bactrocera cucurbitae: a review of its biology and management. J. Insect Sci. 5 (5), 40. doi:10.1093/jis/5.1.40
Elfekih S., Chen C. Y., Hsu J. C., Belcaid M., Haymer D. (2016). Identification and preliminary characterization of chemosensory perception-associated proteins in the melon fly Bactrocera cucurbitae using RNA-seq. Sci. Rep. Jan. 11 6, 19112, 19112. doi:10.1038/srep19112
Enjin A., Zaharieva E. E., Frank D. D., Mansourian S., Suh G. S., Gallio M., et al. (2016). Humidity sensing in Drosophila. Curr. Biol. 26, 1352–1358. doi:10.1016/j.cub.2016.03.049
Fan J., Zhang Y., Francis F., Cheng D., Sun J., Chen J. (2015). Orco mediates olfactory behaviors and winged morph differentiation induced by alarm pheromone in the grain aphid, Sitobion avenae. Insect Biochem. Mol. Biol. 64, 16–24. doi:10.1016/j.ibmb.2015.07.006
Fan X. B., Mo B. T., Li G. C., Huang L. Q., Guo H., Gong X. L., et al. (2022). Mutagenesis of the odorant receptor co-receptor (Orco) reveals severe olfactory defects in the crop pest moth Helicoverpa armigera. BMC Biol. 20 (1), 214. doi:10.1186/s12915-022-01411-2
Findlay G. D., Yi X., Maccoss M. J., Swanson W. J. (2008). Proteomics reveals novel Drosophila seminal fluid proteins transferred at mating. PLoS Biol. Jul 29 (7), 178. doi:10.1371/journal.pbio.0060178
Forêt S., Wanner K. W., Maleszka R. (2007). Chemosensory proteins in the honey bee: insights from the annotated genome, comparative analyses and expressional profiling. Insect Biochem. Mol. Biol. Jan. 37 (1), 19–28. doi:10.1016/j.ibmb.2006.09.009
Frank D. D., Enjin A., Jouandet G. C., Zaharieva E. E., Para A., Stensmyr M. C., et al. (2017). Early integration of temperature and humidity stimuli in the Drosophila brain. Curr. Biol. 27, 2381–2388. doi:10.1016/j.cub.2017.06.077
Galindo K., Smith D. P. (2001). A large family of divergent Drosophila odorant-binding proteins expressed in gustatory and olfactory sensilla. Genet. Nov. 159 (3), 1059–1072. doi:10.1093/genetics/159.3.1059
Gao Q., Chess A. (1999). Identification of candidate Drosophila olfactory receptors from genomic DNA sequence. Genomics 60 (1), 31–39. doi:10.1006/geno.1999.5894
Gomez-Diaz C., Bargeton B., Abuin L., Bukar N., Reina J. H., Bartoi T., et al. (2016). A CD36 ectodomain mediates insect pheromone detection via a putative tunnelling mechanism. Nat. Commun. 7, 11866. doi:10.1038/ncomms11866
Gorter J. A., Jagadeesh S., Gahr C., Boonekamp J. J., Levine J. D., Billeter J. C. (2016). The nutritional and hedonic value of food modulate sexual receptivity in Drosophila melanogaster females. Sci. Rep. 6, 19441. doi:10.1038/srep19441
Grosjean Y., Rytz R., Farine J. P., Abuin L., Cortot J., Jefferis G. S., et al. (2011). An olfactory receptor for food-derived odours promotes male courtship in Drosophila 28 (7368), 236–240. doi:10.1038/nature10428
Gu T., Huang K., Tian S., Sun Y., Li H., Chen C., et al. (2019). Antennal transcriptome analysis and expression profiles of odorant binding proteins in Clostera restitura. Comp. Biochem. Physiol. Part D. Genomics Proteomics. Mar. 29, 211–220. doi:10.1016/j.cbd.2018.12.002
Han H., Liu Z., Meng F., Jiang Y., Cai J. (2020). Identification of olfactory genes of a forensically important blow fly, Aldrichina grahami (Diptera: calliphoridae). PeerJ 8, e9581. doi:10.7717/peerj.9581
Hansson B. S., Stensmyr M. C. (2011). Evolution of insect olfaction. Neuron 72, 698–711. doi:10.1016/j.neuron.2011.11.003
Hill C. A., Fox A. N., Pitts R. J., Kent L. B., Tan P. L., Chrystal M. A., et al. (2002). G Protein-Coupled receptors in Anopheles gambiae. Science 298 (5591), 176–178. doi:10.1126/science.1076196
Hu J., Wang X. Y., Tan L. S., Lu W., Zheng X. L. (2022). Identification of chemosensory genes, including candidate pheromone receptors, in phauda flammans (walker) (Lepidoptera: phaudidae) through transcriptomic analyses. Front. Physiol. 13, 907694. doi:10.3389/fphys.2022.907694
Ishida Y., Ishibashi J., Leal W. S. (2013). Fatty acid solubilizer from the oral disk of the blowfly. PLoS One 8 (1), e51779. doi:10.1371/journal.pone.0051779
Jaime C. P., Souder. S. K., Dong H., Max Collignon. R., Vargas R. I. (2021). Age-dependent response of female melon fly, Zeugodacus cucurbitae (Diptera: Tephritidae), to volatiles emitted from damaged host fruits. J. Asia-Pacific Entomology 24 (3), 759–763. doi:10.1016/j.aspen.2021.06.011
Jeong Y. T., Shim J., Oh S. R., Yoon H. I., Kim C. H., Moon S. J., et al. (2013). An odorant-binding protein required for suppression of sweet taste by bitter chemicals. Neuron 79 (4), 725–737. doi:10.1016/j.neuron.2013.06.025
Jiang X., Pablo P., Ewald G. W., Heinz B., Jürgen K. (2016). Identification and characterization of two “sensory neuron membrane proteins” (SNMPs) of the desert locust, Schistocerca gregaria (Orthoptera: acrididae). J. Insect Sci. 16 (1), 33. doi:10.1093/jisesa/iew015
Kim D., Langmead B., Salzberg S. L. (2015). HISAT: a fast spliced aligner with low memory requirements. Nat. Methods. Apr 12 (4), 357–360. doi:10.1038/nmeth.3317
Knecht Z. A., Silbering A. F., Ni L., Klein M., Budelli G., Bell R., et al. (2016). Distinct combinations of variant ionotropic glutamate receptors mediate thermosensation and hygrosensation in Drosophila. eLife 5, e17879. doi:10.7554/eLife.17879
Koutroumpa F. A., Monsempes C., François M. C., de C. A., Royer C., Concordet J. P., et al. (2016). Heritable genome editing with CRISPR/Cas9 induces anosmia in a crop pest moth. Sci. Rep. 12 (6), 29620. doi:10.1038/srep29620
Kurtovic A., Widmer A., Dickson B. J. (2007). A single class of olfactory neurons mediates behavioural responses to a Drosophila sex pheromone. Nat. Mar. 29 446 (7135), 542–546. doi:10.1038/nature05672
Kwon J. Y., Dahanukar A., Weiss L. A., Carlson J. R. (2007). The molecular basis of CO2 reception in Drosophila. Proc. Natl. Acad. Sci. U. S. A. 27 (9), 3574–3578. doi:10.1073/pnas.0700079104
Larter N. K., Sun J. S., Carlson J. R. (2016). Organization and function of Drosophila odorant binding proteins. Elife 5, e20242. doi:10.7554/eLife.20242
Laughlin J. D., Ha T. S., Jones D. N. M., Smith D. P. (2008). Activation of pheromone-sensitive neurons is mediated by conformational activation of pheromone-binding protein. Cell 133 (7), 1255–1265. doi:10.1016/j.cell.2008.04.046
Leal W. S. (2013). Odorant reception in insects: roles of receptors, binding proteins, and degrading enzymes. Annu. Rev. Entomol. 58, 373–391. doi:10.1146/annurev-ento-120811-153635
Lee Y., Moon S. J., Montell C. (2009). Multiple gustatory receptors required for the caffeine response in Drosophila. Proc. Natl. Acad. Sci. U. S. A. 17 (11), 4495–4500. doi:10.1073/pnas.0811744106
Leitch O., Papanicolaou A., Lennard C., Kirkbride K. P., Anderson A. (2015). Chemosensory genes identified in the antennal transcriptome of the blowfly Calliphora stygia. BMC Genomics 31 (1), 255. doi:10.1186/s12864-015-1466-8
Li B., Dewey C. N. (2011). RSEM: accurate transcript quantification from RNA-Seq data with or without a reference genome. BMC Bioinforma. 12 (1), 323. doi:10.1186/1471-2105-12-323
Li G. C., Nuo S. M., Wang Z. Q., Yang A. J., Liu N. Y. (2021). Identification and expression profiling of chemosensory membrane protein genes in Achelura yunnanensis (Lepidoptera: zygaenidae). Comp. Biochem. Physiol. Part D. Genomics Proteomics 40, 100876. doi:10.1016/j.cbd.2021.100876
Li Y., Zhang J., Chen D., Yang P., Jiang F., Wang X., et al. (2016). CRISPR/Cas9 in locusts: successful establishment of an olfactory deficiency line by targeting the mutagenesis of an odorant receptor co-receptor (Orco). Insect Biochem. Mol. Biol. 79, 27–35. doi:10.1016/j.ibmb.2016.10.003
Lin C. C., Prokop-Prigge K. A., Preti G., Potter C. J. (2015). Food odors trigger Drosophila males to deposit a pheromone that guides aggregation and female oviposition decisions. Elife. Sep. 30, e08688. doi:10.7554/eLife.08688
Liu H., Chen Z. S., Zhang D. J., Lu Y. Y. (2018). BdorOR88a modulates the responsiveness to methyl eugenol in mature males of Bactrocera dorsalis (hendel). Front. Physiol. 9, 987. doi:10.3389/fphys.2018.00987
Liu J., Liu H., Yi J., Mao Y., Li J., Sun D., et al. (2021a). Transcriptome characterization and expression analysis of chemosensory genes in chilo sacchariphagus (Lepidoptera crambidae), a key pest of sugarcane. Front. Physiol. 12, 636353. doi:10.3389/fphys.2021.636353
Liu X., Tong N., Wu Z., Li Y., Ma M., Liu P., et al. (2021b). Identification of chemosensory genes based on the antennal transcriptomic analysis of plagiodera versicolora. Insects 13 (1), 36. doi:10.3390/insects13010036
Liu X. Y., Yan R., Chen S. J., Zhang J. L., Xu H. J. (2023). Orco mutagenesis causes deficiencies in olfactory sensitivity and fertility in the migratory brown planthopper, Nilaparvata lugens. Pest Manag. Sci. Mar. 79 (3), 1030–1039. doi:10.1002/ps.7286
Liu Z., Smagghe G., Lei Z., Wang J. J. (2016). Identification of male- and female-specific olfaction genes in antennae of the oriental fruit fly (Bactrocera dorsalis). PLoS One 11 (2), e0147783. doi:10.1371/journal.pone.0147783
MacWilliam D., Kowalewski J., Kumar A., Pontrello C., Ray A. (2018). Signaling mode of the broad-spectrum conserved CO2 receptor is one of the important determinants of odor valence in Drosophila. Neuron 7 (5), 1153–1167. doi:10.1016/j.neuron.2018.01.028
Mei T., Fu W. B., Li B., He Z. B., Chen B. (2018). Comparative genomics of chemosensory protein genes (CSPs) in twenty-two mosquito species (Diptera: Culicidae): identification, characterization, and evolution. PLoS One 13 (1), e0190412. doi:10.1371/journal.pone.0190412
Miller N. W., Vargas R. I., Prokopy R. J., Mackey B. E. (2004). State-dependent attractiveness of protein bait and host fruit odor to Bactrocera cucurbitae (Diptera: Tephritidae) females. Ann 97, 1063–1068. doi:10.1603/0013-8746(2004)097[1063:saopba]2.0.co;2
Mir S. H., Dar S. A., Mir G. M., Ahmad S. B. (2014). Biology of Bactrocera cucurbitae (Diptera: Tephritidae) on cucumber. Fla. Entomol. 97, 753–758. doi:10.1653/024.097.0257
Missbach C., Dweck H. K., Vogel H., Vilcinskas A., Stensmyr M. C., Hansson B. S., et al. (2014). Evolution of insect olfactory receptors. eLife 3, e02115. doi:10.7554/eLife.02115
Mitaka H., Matsuo T., Miura N., Ishikawa Y. (2011). Identification of odorant-binding protein genes from antennal expressed sequence tags of the onion fly, Delia antiqua. Mol. Biol. Rep. Mar. 38 (3), 1787–1792. doi:10.1007/s11033-010-0293-x
Miyamoto T., Amrein H. (2014). Diverse roles for the Drosophila fructose sensor Gr43a. Fly. (Austin) 8 (1), 19–25. doi:10.4161/fly.27241
Ning C., Yang K., Xu M., Huang L. Q., Wang C. Z. (2016). Functional validation of the carbon dioxide receptor in labial palps of Helicoverpa armigera moths. Insect Biochem. Mol. Biol. Jun 73, 12–19. doi:10.1016/j.ibmb.2016.04.002
Obiero G. F., Mireji P. O., Nyanjom S. R., Christoffels A., Robertson H. M., Masiga D. K. (2014). Odorant and gustatory receptors in the tsetse fly Glossina morsitans morsitans. PLoS Negl. Trop. Dis. 8 (4), e2663. doi:10.1371/journal.pntd.0002663
Ono H., Hee A. K., Jiang H. (2021). Recent advancements in studies on chemosensory mechanisms underlying detection of semiochemicals in dacini fruit flies of economic importance (Diptera: Tephritidae). Insects. 12(2). doi:10.3390/insects12020106
Ono H., Miyazaki H., Mitsuno H., Ozaki K., Kanzaki R., Nishida R. (2020). Functional characterization of olfactory receptors in three Dacini fruit flies (Diptera: Tephritidae) that respond to 1-nonanol analogs as components in the rectal glands. Comp. Biochem. Physiol. B Biochem. Mol. Biol. 239, 110346. doi:10.1016/j.cbpb.2019.110346
Pelosi P., Iovinella I., Felicioli A., Dani F. R. (2014). Soluble proteins of chemical communication: an overview across arthropods. Front. Physiol. 5, 320. doi:10.3389/fphys.2014.00320
Pelosi P., Iovinella I., Zhu J., Wang G., Dani F. R. (2018). Beyond chemoreception: diverse tasks of soluble olfactory proteins in insects. Biol. Rev. Camb. Philosophical Soc. 93 (1), 184–200. doi:10.1111/brv.12339
Pelosi P., Zhou J. J., Ban L. P., Calvello M. (2006). Soluble proteins in insect chemical communication. Cell. Mol. Life Sci. 63 (14), 1658–1676. doi:10.1007/s00018-005-5607-0
Pertea M., Pertea G. M., Antonescu C. M., Chang T. C., Mendell J. T., Salzberg S. L. (2015). StringTie enables improved reconstruction of a transcriptome from RNA-seq reads. Nat. Biotechnol. Mar. 33 (3), 290–295. doi:10.1038/nbt.3122
Piñero J. C., Souder S. K., Vargas R. I. (2020). Synergistic and additive interactions among components of food-based baits underlie female fruit fly (Diptera: Tephritidae) attraction. Entomol. Exp. Appl. 168, 339–348. doi:10.1111/eea.12890
Poudel S., Lee Y. (2016). Gustatory receptors required for avoiding the toxic compound coumarin in Drosophila melanogaster. Mol. Cells 30 (4), 310–315. doi:10.14348/molcells.2016.2250
Rass M., Oestreich S., Guetter S., Fischer S., Schneuwly S. (2019). The Drosophila fussel gene is required for bitter gustatory neuron differentiation acting within an Rpd3 dependent chromatin modifying complex. PLoS Genet. 15 (2), e1007940. doi:10.1371/journal.pgen.1007940
Rebecca D., John R. C. (2016). Bitter taste receptors confer diverse functions to neurons. Neuroscience 5, e11181. doi:10.7554/eLife.11181
Rihani K., Ferveur J. F., Briand L. (2021). The 40-year mystery of insect odorant-binding proteins. Biomol. Mar. 30 (4), 509. doi:10.3390/biom11040509
Rihani N. K., Sun J. S., Carlson J. R. (2016). Organization and function of Drosophila odorant binding proteins. Elife 5, e20242. doi:10.7554/elife.20242
Robertson H. M., Warr C. G., Carlson J. R. (2003). Molecular evolution of the insect chemoreceptor gene superfamily in Drosophila melanogaster. Proc. Natl. Acad. Sci. U. S. A. 25 (100 Suppl. 2), 14537–14542. doi:10.1073/pnas.2335847100
Rogers M. E., Krieger J., Vogt R. G. (2001). Antennal SNMPs (sensory neuron membrane proteins) of Lepidoptera define a unique family of invertebrate CD36-like proteins. J. Neurobiol. 49 (1), 47–61. doi:10.1002/neu.1065
Rytz R., Croset V., Benton R. (2013). Ionotropic receptors (IRs): chemosensory ionotropic glutamate receptors in Drosophila and beyond. Insect Biochem. Mol. Biol. Sep. 43 (9), 888–897. doi:10.1016/j.ibmb.2013.02.007
Sang J., Rimal S., Lee Y. (2019). Gustatory receptor 28b is necessary for avoiding saponin in Drosophila melanogaster. EMBO Rep. 5 (2), e47328. doi:10.15252/embr.201847328
Sato K., Tanaka K., Touhara K. (2011). Sugar-regulated cation channel formed by an insect gustatory receptor. Proc. Natl. Acad. Sci. U. S. A. 12 (28), 11680–11685. doi:10.1073/pnas.1019622108
Segura-León O. L., Torres-Huerta B., Estrada-Pérez A. R., Cibrián-Tovar J., Hernandez-Hernandez F. C., Cruz-Jaramillo J. L., et al. (2022). Identification of candidate chemosensory gene families by head transcriptomes analysis in the Mexican fruit fly, Anastrepha ludens loew (Diptera: Tephritidae). Int. J. Mol. Sci. 23 (18), 10531. doi:10.3390/ijms231810531
Sepil I., Hopkins B. R., Dean R., Thézénas M. L., Charles P. D., Konietzny R., et al. (2019). Quantitative proteomics identification of seminal fluid proteins in male Drosophila melanogaster. Mol. Cell. proteomics MCP 18 (Suppl. 1), S46-S58–S58. doi:10.1074/mcp.RA118.000831
Shanbhag S. R., Müller B., Steinbrecht R. A. (1999). Atlas of olfactory organs of Drosophila melanogaster. Int. J. Insect Morphol. Embryology 28(4), 377–397. doi:10.1016/S0020-7322(99)00039-2
Shivaramu S., Parepally S. K., Chakravarthy A. K., Pagadala , Damodaram K. J., Kempraj V. (2022). Ridge gourd volatiles are attractive to gravid female melon fly, Zeugodacus cucurbitae (Coquillett) (Diptera: Tephritidae). J. Appl. Entomology 146, 539–546. doi:10.1111/jen.12975
Shorter J. R., Dembeck L. M., Everett L. J., Morozova T. V., Arya G. H., Turlapati L., et al. (2016). Obp56h modulates mating behavior in Drosophila melanogaster. G3 (Bethesda, Md 6 (10), 3335–3342. doi:10.1534/g3.116.034595
Song Y. Q., Sun H. Z., Dong J. F. (2020). Identification and expression analysis of an olfactory receptor gene family in the yemma signatus hsiao (Hemiptera: berytidae). Pjz 52, 1911–1919. doi:10.17582/journal.pjz/20180616040644
Sparks J. T., Bohbot J. D., Dickens J. C. (2014). The genetics of chemoreception in the labella and tarsi of Aedes aegypti. Insect Biochem. Mol. Biol. May 48, 8–16. doi:10.1016/j.ibmb.2014.02.004
Steinbrecht R. A. (1996). Structure and function of insect olfactory sensilla. Ciba Found. Symp. 200, 158–174. doi:10.1002/9780470514948.ch13
Suh E., Bohbot J. D., Zwiebel L. J. (2014). Peripheral olfactory signaling in insects. Curr. Opin. Insect 6, 86–92. doi:10.1016/j.cois.2014.10.006
Sun D., Huang Y., Qin Z., Zhan H., Zhang J., Liu Y., et al. (2020). Identification of candidate olfactory genes in the antennal transcriptome of the stink bug halyomorpha halys. Front. Physiol. 11, 876. doi:10.3389/fphys.2020.00876
Sun J. S., Larter N. K., Chahda J. S., Rioux D., Gumaste A., Carlson J. R. (2018). Humidity response depends on the small soluble protein Obp59a in Drosophila. Elife Sep. 19, e39249. doi:10.7554/eLife.39249
Sun Y. L., Dong J. F., Ning C., Ding P. P., Huang L. Q., Sun J. G., et al. (2019). An odorant receptor mediates the attractiveness of cis -jasmone to campoletis chlorideae, the endoparasitoid of helicoverpa armigera. Insect Mol. Biol. 28, 23–34. doi:10.1111/imb.12523
Sun Y. L., Huang L. Q., Pelosi P., Wang C. Z. (2012). Expression in antennae and reproductive organs suggests a dual role of an odorant-binding protein in two sibling Helicoverpa species. PLoS One 7 (1), e30040. doi:10.1371/journal.pone.0030040
Swarup S., Williams T. I., Anholt R. R. (2011). Functional dissection of Odorant binding protein genes in Drosophila melanogaster. Genes Brain Behav. Aug 10 (6), 648–657. doi:10.1111/j.1601-183X.2011.00704.x
Takemori N., Yamamoto M. T. (2009). Proteome mapping of the Drosophila melanogaster male reproductive system. Proteomics. May 9 (9), 2484–2493. doi:10.1002/pmic.200800795
Task D., Lin C. C., Vulpe A., Afify A., Ballou S., Brbic M., et al. (2020). Chemoreceptor co-expression in Drosophila melanogaster olfactory neurons. Elife 20, e72599. doi:10.7554/eLife.72599
Tsoumani K. T., Belavilas-Trovas A., Gregoriou M. E., Mathiopoulos K. D. (2020). Anosmic flies: what Orco silencing does to olive fruit flies. BMC Genet. 21, 140. doi:10.1186/s12863-020-00937-0
Vargas R. I., Piñero J. C., Miller N. W. (2018). Effect of physiological state on female melon fly (Diptera: Tephritidae) attraction to host and food odor in the field. J. Econ. Entomol. 111 (3), 1318–1322. doi:10.1093/jee/toy092
Vieira F. G., Rozas J. (2011). Comparative genomics of the odorant-binding and chemosensory protein gene families across the arthropoda: origin and evolutionary history of the chemosensory system. Genome Biol. Evol. 3, 476–490. doi:10.1093/gbe/evr033
Vieira F. G., Sánchez-Gracia A., Rozas J. (2007). Comparative genomic analysis of the odorant-binding protein family in 12 Drosophila genomes: purifying selection and birth-and-death evolution. Genome Biol. 8 (11), R235. doi:10.1186/gb-2007-8-11-r235
Vogt R. G., Miller N. E., Litvack R., Fandino R. A., Sparks J., Staples J., et al. (2009). The insect SNMP gene family. Insect Biochem. Mol. Biol. 39, 448–456. doi:10.1016/j.ibmb.2009.03.007
Wang B., Liu Y., He K., Wang G. (2016). Comparison of research methods for functional characterization of insect olfactory receptors. Sci. Rep. 6, 32806. doi:10.1038/srep32806
Wang Z., Qi Z., Chen J., Wang F., Gui L., Zhang G. (2021). Molecular characterization of chemosensory protein genes in Bactrocera minax (Diptera: Tephritidae). Entomol. Res. 51, 349–356. doi:10.1111/1748-5967.12517
Wicher D., Miazzi F. (2021). Functional properties of insect olfactory receptors: ionotropic receptors and odorant receptors. Cell Tissue Res. 383, 7–19. doi:10.1007/s00441-020-03363-x
Wu Z., Cui Y., Ma J., Qu M., Lin J. (2020). Analyses of chemosensory genes provide insight into the evolution of behavioral differences to phytochemicals in Bactrocera species. Mol. Phylogenet Evol. Oct. 151, 106858. doi:10.1016/j.ympev.2020.106858
Wu Z., Kang C., Qu M., Chen J., Chen M., Bin S., et al. (2019). Candidates for chemosensory genes identified in the Chinese citrus fly, Bactrocera minax, through a transcriptomic analysis. BMC Genom 20 (1), 646. doi:10.1186/s12864-019-6022-5
Wu Z., Ye J., Qian J., Purba E., Zhang Q., Zhang L., et al. (2022). Identification and expression profile of chemosensory receptor genes in aromia bungii (faldermann) antennal transcriptome. Insects 13, 96. doi:10.3390/insects13010096
Wu Z., Zhang H., Wang Z., Bin S., He H., Lin J. (2015). Discovery of chemosensory genes in the oriental fruit fly, Bactrocera dorsalis. PLoS One 10 (6), e0129794. doi:10.1371/journal.pone.0129794
Xing Y., Thanasirungkul W., Adeel M. M., Yu J., Aslam A., Chi D. F. (2021). Identification and analysis of olfactory genes in Dioryctria abietella based on the antennal transcriptome. Comp. Biochem. Physiol. Part D. Genomics Proteomics. Jun 38, 100814. doi:10.1016/j.cbd.2021.100814
Xu L., Jiang H. B., Tang K. Y., Yan Y., Schetelig M. F., Wang J. J. (2022). CRISPR-mediated mutagenesis of the odorant receptor co-receptor (Orco) gene disrupts olfaction-mediated behaviors in Bactrocera dorsalis. Insect Sci. 29 (5), 1275–1286. doi:10.1111/1744-7917.12997
Xu P., Wang Y., Akami M., Niu C. Y. (2019). Identification of olfactory genes and functional analysis of BminCSP and BminOBP21 in Bactrocera minax. PLoS ONE 14, e0222193. doi:10.1371/journal.pone.0222193
Xu W. (2020). How do moth and butterfly taste? - molecular basis of gustatory receptors in Lepidoptera. Insect Sci. 27, 1148–1157. doi:10.1111/1744-7917.12718
Xu W., Cornel A. J., Leal W. S. (2010). Odorant-binding proteins of the malaria mosquito Anopheles funestus sensu stricto. PLoS One 5 (10), e15403. doi:10.1371/journal.pone.0015403
Yasukawa J., Tomioka S., Aigaki T., Matsuo T. (2010). Evolution of expression patterns of two odorant-binding protein genes, Obp57d and Obp57e, in Drosophila. Gene. Nov. 1 467 (1-2), 25–34. doi:10.1016/j.gene.2010.07.006
Yi X., Zhao H., Dong X., Wang P., Hu M., Zhong G. (2013). BdorCSP2 is important for antifeed and oviposition-deterring activities induced by rhodojaponin-III against Bactrocera dorsalis. PLoS ONE 8 (10), e77295. doi:10.1371/journal.pone.0077295
Zhang J., Bisch-Knaden S., Fandino R. A., Yan S., Obiero G. F., Grosse-Wilde E., et al. (2019). The olfactory coreceptor IR8a governs larval feces-mediated competition avoidance in a hawkmoth. Proc. Natl. Acad. Sci. U.S.A. 116, 21828–21833. doi:10.1073/pnas.1913485116
Zhou X., Guo J., Zhang M., Bai C., Wang Z., Li Z. (2021). Antennal transcriptome analysis and candidate olfactory genes in Crematogaster rogenhoferi. Bull. Entomol. Res. Aug 111 (4), 464–475. doi:10.1017/S0007485321000134
Zhu J., Iovinella I., Dani F. R., Liu Y. L., Huang L. Q., Liu Y., et al. (2016). Conserved chemosensory proteins in the proboscis and eyes of Lepidoptera. Int. J. Biol. Sci. 12 (11), 1394–1404. doi:10.7150/ijbs.16517
Keywords: Bactrocera cucurbitae, chemosensory genes, transcriptome, insect olfaction, pest management
Citation: Wang JJ, Ma C, Yue Y, Yang J, Chen LX, Wang YT, Zhao CC, Gao X, Chen HS, Ma WH and Zhou Z (2024) Identification of candidate chemosensory genes in Bactrocera cucurbitae based on antennal transcriptome analysis. Front. Physiol. 15:1354530. doi: 10.3389/fphys.2024.1354530
Received: 13 December 2023; Accepted: 24 January 2024;
Published: 19 February 2024.
Edited by:
Ya-Nan Zhang, Huaibei Normal University, ChinaReviewed by:
Hamzeh Izadi, Vali-E-Asr University of Rafsanjan, IranYifan Zhai, Shandong Academy of Agricultural Sciences, China
Copyright © 2024 Wang, Ma, Yue, Yang, Chen, Wang, Zhao, Gao, Chen, Ma and Zhou. This is an open-access article distributed under the terms of the Creative Commons Attribution License (CC BY). The use, distribution or reproduction in other forums is permitted, provided the original author(s) and the copyright owner(s) are credited and that the original publication in this journal is cited, in accordance with accepted academic practice. No use, distribution or reproduction is permitted which does not comply with these terms.
*Correspondence: Zhongshi Zhou, zhouzhongshi@caas.cn