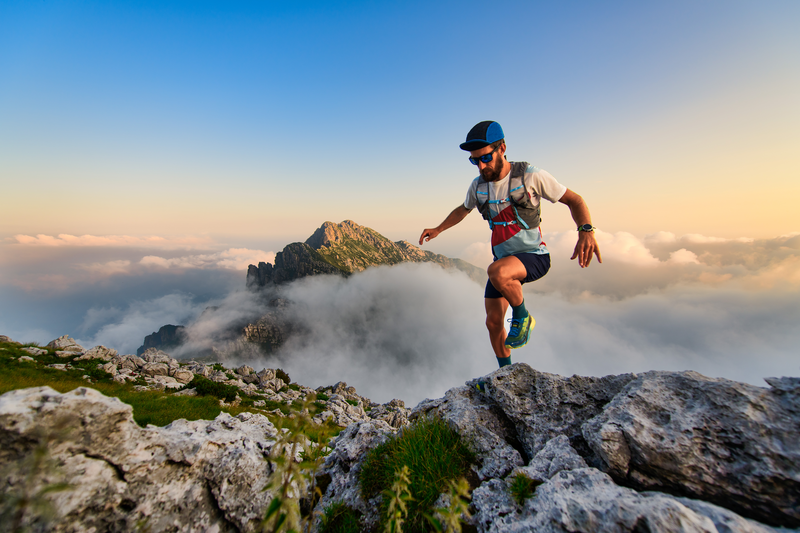
94% of researchers rate our articles as excellent or good
Learn more about the work of our research integrity team to safeguard the quality of each article we publish.
Find out more
ORIGINAL RESEARCH article
Front. Physiol. , 04 October 2023
Sec. Aquatic Physiology
Volume 14 - 2023 | https://doi.org/10.3389/fphys.2023.1272267
This article is part of the Research Topic Endocrine Regulation and Physiological Adaptation of Stress Response in Aquatic Organisms View all 18 articles
The study combined the use of biometric, behavioral, physiological and external tissue damage scoring systems to better understand how high stocking densities drive schooling behavior and other adaptive features during the finishing growing phase of farmed gilthead sea bream in the Western Mediterranean. Fish were grown at three different final stocking densities (LD, 8.5 kg/m3; MD, 17 kg/m3; HD, 25 kg/m3). Water oxygen concentration varied between 5 and 6 ppm in LD fish to 3–4 ppm in HD fish with the summer rise of water temperature from 19°C to 26°C (May–July). HD fish showed a reduction of feed intake and growth rates, but they also showed a reinforced social cohesion with a well-defined endogenous swimming activity rhythm with feeding time as a main synchronization factor. The monitored decrease of the breathing/swimming activity ratio by means of the AEFishBIT data-logger also indicated a decreased energy partitioning for growth in the HD environment with a limited oxygen availability. Plasma glucose and cortisol levels increased with the rise of stocking density, and the close association of glycaemia with the expression level of antioxidant enzymes (mn-sod, gpx4, prdx5) in liver and molecular chaperones (grp170, grp75) in skeletal muscle highlighted the involvement of glucose in redox processes via rerouting in the pentose-phosphate-pathway. Other adaptive features included the depletion of oxidative metabolism that favored lipid storage rather than fatty acid oxidation to decrease the oxygen demand as last electron acceptor in the mitochondrial respiratory chain. This was coincident with the metabolic readjustment of the Gh/Igf endocrine-growth cascade that promoted the regulation of muscle growth at the local level rather than a systemic action via the liver Gh/Igf axis. Moreover, correlation analyses within HD fish displayed negative correlations of hepatic transcripts of igf1 and igf2 with the data-logger measurements of activity and respiration, whereas the opposite was found for muscle igf2, ghr1 and ghr2. This was indicative of a growth-regulatory transition that supported a proactive instead of a reactive behavior in HD fish, which was considered adaptive to preserve an active and synchronized feeding behavior with a minimized risk of oxidative stress and epidermal skin damage.
Global aquaculture production of aquatic animals increased at an average rate of 2.2% from 1990 to 2020 until reaching a milestone of 88 million tonnes per year (FAO, 2022). This increase of animal aquaculture production mostly supported the augmented human per capita consumption of fish from 14 kg (live weigh equivalent) in 1990 to 20.2 kg in 2020. However, the intensification of aquaculture production must deal with inadequate stocking densities that may increase the risk of health issues and welfare impairments due to feed competition and aggressive interactions among other stressful events (Ellis et al., 2002; North et al., 2006; Baldwin, 2011; Jia et al., 2016; Liu et al., 2017; Wu et al., 2018). The legislation limiting stocking density can contribute to support the expansion of a more sustainable and ethical aquaculture production, but a more rational approach might be to define acceptable levels of different welfare indicators for each particular species, life-stage and production system (van de Vis et al., 2020; Saraiva et al., 2022). Certainly, important research efforts have been made over the last decade for finding the golden stocking density of farmed fish, combining criteria of economic profitability with the increasing pressure of consumers in developed countries for an enhanced control and regulation in welfare assurance schemes (Noble et al., 2018). In land-based systems, negative effects on feed utilization and physiological stress markers were found in Atlantic salmon (Salmo salar) at stocking densities above 75 kg/m3, but densities above 25 kg/m3 reduced feed intake and growth of adult fish kept in sea cages (Calabrese et al., 2017). Signs of external tissue lesions, and impaired growth and feed utilization also occurred above 20–30 kg/m3 in gilthead sea bream (Sparus aurata) and European sea bass (Dicentrarchus labrax) (Person-Le Ruyet and Le Bayon, 2009; Carbonara et al., 2019). However, the occurrence of different stress coping styles plays a key role on how rearing density influences welfare-related responses, being generally accepted that fish that respond in a shy and subordinate manner (reactive fish) are more able to cope with higher densities, while individuals that are bold and aggressive (proactive fish) are more able to cope with low densities (Carbonara et al., 2019). In addition, reduced oxygen (O2) availability exacerbates the negative impact of high stocking density on feed intake and growth in gilthead sea bream, triggering different adaptive responses mediated by changes in the gene expression profile of tissue-specific markers of antioxidant defense, oxidative phosphorylation, protein accretion (muscle growth), and lipid metabolism (Martos-Sitcha et al., 2019a).
In gilthead sea bream, there is also now evidence that groups of proactive and reactive individuals did not exhibit consistent escape behavior responses when fish were subjected to restraining tests, which might be indicative that the social context in which fish are kept has an impact on the manifestation of certain personality traits of individuals (Castanheira et al., 2016). Indeed, animal welfare applies to its positive physical and mental condition (Ashley, 2007), and there is now evidence that both swimming activity and schooling behavior are reinforced by high stocking densities in gilthead sea bream (Carbonara et al., 2019; Arechavala-Lopez et al., 2020). Of note, both behavior and swimming performance are also regulated genetically in this species (Perera et al., 2021; Calduch-Giner et al., 2023). At the same time, swimming performance can be improved by a mild-hypoxia pre-conditioning, which leads to a persistent higher critical speed at exercise exhaustion that shifts towards a higher anaerobic fitness following normoxia restoration (Naya-Català et al., 2021). It must be also taken into account that aquaculture finishing production cycle is often accounted during the summer period with relatively high stocking densities and reduced O2 availability, and there is still a lack of practical procedures for assessing changes in behavior, physiological traits and welfare indicators in order to fulfill growth performance and welfare status with intensive aquaculture production. Thus, the present study aims to contribute to solve this gap of knowledge, combining the use of AEFishBIT data-loggers for accurate monitoring of swimming activity and breathing rates (Calduch-Giner et al., 2022) with customized PCR-arrays for the simultaneous gene expression profiling of stress-responsive genes (Calduch-Giner et al., 2010; Magnoni et al., 2017; Martos-Sitcha et al., 2019a; Naya-Català et al., 2021), and welfare scores of muscle fat content, blood biochemistry, and external tissue damage (Sánchez-Muros et al., 2013; Seibel et al., 2021; Weirup et al., 2022). The hypothesis of work is that such integrative approach can contribute to better determine the golden stocking density in a given farming condition, encompassing animal welfare and industry interests, with the double aim of mitigating drawback effects but also recognizing the importance of indicators of positive welfare of both physical health and appropriate social interactions (Ashley, 2007; Toni et al., 2019).
All procedures were approved by the Ethics and Animal Welfare Committee of the Institute of Aquaculture Torre de la Sal (IATS), CSIC Ethics Comittee (permission 1295/2022) and Generalitat Valenciana (permission 2022-VSC-PEA-0230). They were carried out in the IATS’s registered aquaculture infrastructure facility (code ES120330001055) in accordance with the principles published in the European Animal Directive (2010/63/EU) and Spanish laws (Royal Decree RD53/2013) for the protection of animals used in scientific experiments.
Two year-old gilthead sea bream of Mediterranean origin (Avramar, Burriana, Spain) were grown in 3,000L tanks from January to May (12–16 kg/m3) in a flow-through system under the natural photoperiod and temperature conditions at the IATS latitude (40° 5′N; 0° 10′E). At the end of May (Figure 1), 462 fish (initial body weight 479.55 ± 2.42 g) were anesthetized with 0.1 g/L MS-222 (Sigma, Saint Louis, MO, United States) and pit-tagged in the dorsal musculature with passive integrated transponders (ID-100A 1.25 Nano Transponder; Trovan, Madrid, Spain). Fish were then re-allocated in experimental tanks (two replicates per condition) at three different stocking densities (low density-LD: 36 fish per tank, 6 kg/m3; medium density-MD: 72 fish per tank, 12 kg/m3; high density-HD: 123 fish per tank, 22 kg/m3), until reaching final stocking densities of 8.5 kg/m3 (LD), 17 kg/m3 (MD) and 25 kg/m3 (HD) after 53 days (mid-July). Body weight and furcal length were recorded at the beginning and at the end of trial, using a FR-200 FishReader W (Trovan, Madrid, Spain). Over the experimental period (May–July, 53 days), fish were fed once daily at a fixed time (12:00 a.m.) with automated feeders near to visual satiety with a standard commercial diet (EFICO 3053, BioMar, Palencia, Spain). Water aeration and flux of inlet water was daily regulated to maintain differentially controlled the water O2 concentration (LD, 5–6 ppm, 70%–95% saturation; MD, 4–5 ppm, 55%–75% saturation; HD, 3–4 ppm, 45%–60% saturation). Temperature and water O2 concentration was continuously measured through an online environmental monitoring system. Weekly determinations of unionized ammonia were always below the toxic threshold level (<0.05 mg/L).
FIGURE 1. Experimental design of gilthead sea bream reared at three different stocking densities (LD—low density, MD—medium density, HD—high density) and dissolved oxygen concentrations during the summer period. Initial stocking density, ρ, and final density (in parenthesis) is shown. Temporal variation (over 53 days experimental period) in dissolved oxygen concentration for each group and temperature are represented. IBW—Initial body weight; FBW—Final body weight.
At the end of trial, 12 randomly selected fish per experimental condition (n = 6 per replicate tank) were anesthetized with 0.1 g/L MS-222, AEFishBIT devices were externally attached to the operculum for the simultaneous monitoring of physical activity and respiratory frequency, and returned to their original tanks. AEFishBIT is a stand-alone, small, and lightweight motion embedded-microsystem with a tri-axial accelerometer that, by means of attachment to the operculum, monitors physical activity by mapping of the accelerations in X- and Y-axes, while the operculum beats (Z-axis) serve as a measure of respiratory frequency (Calduch-Giner et al., 2022). The devices were programmed for data acquisition of physical activity and respiratory frequency during 2 min every 15 min along two consecutive days, in which fish remained unfed. The sampling frequency of the AEFishBIT device was 100 Hz, and the software pre-processing of raw data was made as described elsewhere (Martos-Sitcha et al., 2019b; Ferrer et al., 2020). At the end of the recording period (48 h post-tagging), all AEFishBIT devices were successfully recovered and pre-processed data were downloaded for tracking the recorded behavioral traits.
After AEFishBIT retrieval, muscle fat content was determined in situ with Distell Fish Fat-meter, FM 692 (Distell Ltd., United Kingdom). Fish were then photographed for the evaluation of indicators of external damage (cataracts, exophthalmia, gill status, fin damage and skin lesions) by using a scoring system from 1 to 5 adapted from Hoyle et al. (2007), where 5 indicates maximum damage (Supplementary Figure S1). Such image welfare scoring was completed with 23 additional fish from each experimental condition (35 fish per experimental condition, 105 in total). Additionally, from AEFishBIT recorded fish, blood was taken from caudal vessels with heparinized syringes, and centrifuged at 3,000 g for 20 min at 4°C. The retrieved plasma was aliquoted and stored at −20°C until glucose and cortisol assays. These sampled fish were then killed by cervical section, and portions of liver and dorsal white skeletal muscle (150–200 mg) were excised and collected in RNA later for its storage at −80°C until RNA extraction for gene expression analyses.
Plasma glucose was determined using the Invitrogen™ Glucose Colorimetric Detection Kit (Invitrogen, EIAGLUC). Plasma cortisol levels were determined with an enzyme Immunoassay Kit (Arbor Assays, K003-H1W) following the manufacturer’s indications. Tissue RNA was extracted using the MagMAX-96 total RNA isolation kit (Life Technologies) after tissue homogenization in TRI reagent following manufacturers’ instructions. RNA quantity and purity was determined by Nanodrop (Thermo Scientific) with absorbance ratios at 260 nm/280 nm of 1.9–2.1. Reverse transcription (RT) of 500 ng of total RNA was performed with random decamers using the High-Capacity cDNA Archive Kit (Applied Biosystems). RT reactions were incubated for 10 min at 25°C and 2 h at 37°C. Negative control reactions were run without reverse transcriptase. Real-time quantitative PCR was carried out with an Eppendorf Mastercycler Ep Realplex, using 96-well PCR array layouts designed for the simultaneous profiling of 44 selected genes of liver and white skeletal muscle (Table 1). The genes comprised in the liver array included markers of the Gh/Igf system (9), lipid metabolism (14), oxidative metabolism and energy sensing (11), and antioxidant defense (10). The analyzed transcripts of muscle included markers of the Gh/Igf system (10), muscle cell growth (8), immune response (5), oxidative metabolism and energy sensing (12), and antioxidant defense (9). Specific primer pair sequences for liver and muscle are listed in Supplementary Tables S1, S2, respectively. Controls of general PCR performance were included on each array, and all the pipetting operations were performed by means of an EpMotion 5070 Liquid Handling Robot (Eppendorf). Briefly, reverse transcription reactions were diluted to convenient concentrations and the equivalent of 660 pg of total input RNA was used in a 25 μL volume for each PCR reaction. PCR-wells contained a 2× SYBR Green Master Mix (Bio-Rad) and specific primers at a final concentration of 0.9 μM were used to obtain amplicons of 50–150 bp in length. The PCR amplification program consisted of an initial denaturation step at 95°C for 3 min, followed by 40 cycles of denaturation for 15 s at 95°C and annealing/extension for 60 s at 60 °C. The efficiency of the PCR reactions was consistently higher than 90% and similar among all genes. The specificity of the reactions was verified by melting curve analysis (ramping rates of 0.5°C/10 s over a temperature range of 55–95°C), and linearity of serial dilutions of RT reactions. Gene expression was calculated using the delta-delta Ct method (Livak and Schmittgen, 2001). β-actin was tested for gene expression stability (GeNorm software, M score = 0.21), and it was used as housekeeping gene in the samples normalization procedure. For multigene expression analysis, all values in the liver were referenced to the expression levels of gpx1 in LD fish with an arbitrary assigned value of 1. In muscle, gene expression values were referenced to those of gpx4 in LD fish with an arbitrary assigned value of 1.
AEFishBIT data were post-processed using a simple cosinor model, which fitted the achieved measurements to a one-harmonic sinusoidal function (Refinetti et al., 2007). Recorded data from incomplete light and dark phases were excluded to avoid any temporal bias. Statistically significant differences (p < 0.05) on cosinor-derived data, external damage, blood biochemistry and tissue gene expression were assessed by one-way ANOVA followed by a Holm-Sidak post hoc test, using the SigmaPlot software 14.5 (Systat Software, San Jose, CA, United States). Correlation analysis was also assessed using the SigmaPlot software. Graphical representations of gathered biomarkers networks was made with the Cytoscape v3.9.1 software. Gene expression patterns were further analyzed by partial least-squares discriminant analysis (PLS-DA) using EZinfo v3.0 (Umetrics, Umeå, Sweden). The quality of the PLS-DA model was evaluated by the parameters R2Y (cum) and Q2 (cum), which indicate the fit and prediction ability, respectively. A validation test of the PLS-DA model consisting of 500 random permutations (Ojala and Garriga, 2010) was performed using the Bioconductor R package ropls (Thévenot et al., 2015). The list of genes contributing to group separation was determined by the minimum Variable Importance in the Projection (VIP) values. Discriminant genes were considered with a VIP threshold ≥1.0 (Li et al., 2012; Kieffer et al., 2016).
As shown in Table 2, the initial body weight (474–484 g) did not differ significantly among the three experimental groups. At the end of trial, the mean body weight of HD fish (598 g) was significantly lower than in MD and LD fish (662 and 669 g, respectively). In parallel, feed intake was significantly higher in LD and MD fish than in HD fish, and specific growth rates (SGR) in HD fish highlighted a 40% reduction (SGR HD, 0.39%; SGR LD-MD, 0.62%–0.66%). Fulton´s body condition factor K (CFK) ranged from 2.95 in LD fish to 2.72 in HD fish. These observations paralleled with a significant increase of feed conversion ratio (FCR) from 1.61–1.66 in LD-MD fish to 1.92 in HD fish. A concurrent decrease of liver weight and hepatosomatic index (HSI) was also found with the increase of stocking density, varying the achieved HSI from 1.17 to 1.14 in LD-MD fish to 0.91 in HD fish. Additionally, a slight (not statistically significant) decrease in muscle fat content was observed in HD fish, pointing out a leaner body shape with a decreased CFK. Regarding blood biochemical parameters, plasma glucose levels were significantly higher in HD fish (108 mg/dL) than in LD fish (89.5 mg/dL), with intermediate values in the MD group that was closer to HD rather than LD fish. Plasma cortisol levels showed the same trend, and the measured values in HD and MD fish (114–106 ng/mL) were significantly higher than in LD fish (64 ng/mL).
TABLE 2. Data on growth performance and basic blood biochemistry of gilthead sea bream reared at three different stocking densities (final density, 8.5–25 kg/m3) from end of May to mid-July 2021 (53 days). Data on whole body biometrics, feed intake and feed conversion are the mean ± SEM of duplicated tanks. Liver weight, hepatosomatic index, muscle fat content, and plasma cortisol and metabolite levels are the mean ± SEM of 12 fish per experimental condition. Different letters indicate statistically significant differences (Holm-Sidak post hoc test, p < 0.05).
Scores of indicators of external damage are represented in the Radar Plot of Figure 2. Epidermal status was progressively impaired with the increase of stocking density and the worst status was clearly achieved in fish held at HD. Dorsal, caudal, pelvic and pectoral fin status were also affected by the stocking density, but in this case HD fish clearly evolved as a differential group in comparison to both LD and MD fish. Signs of cataracts or exophthalmia were absent in all fish groups, and gill status was visually similar and in good condition in all fish.
FIGURE 2. Radar plot representing external welfare indicators of gilthead sea bream reared at three different densities (scoring system from 1 to 5 adapted from Hoyle et al. (2007). Numbered fish body parts are indicated for visualization. Colored points are the mean (n = 35) of each welfare indicator. Symbols, † and #, indicate significant differences between the three density groups and between HD and the other two experimental densities, respectively, (p < 0.05, ANOVA, Holm-Sidak test).
Visual observations highlighted a more heterogeneous swimming behavior in fish held at low densities than in HD fish. Certainly, the recorded physical activity and respiratory frequency fitted better to the cosinor model in HD fish than in the other two experimental groups, which became especially evident in the case of LD fish (Supplementary Figure S2). This would reflect an improved social cohesion in HD fish, acting the feeding time as a major zeitgeber synchronization factor with the acrophase of physical activity (φ, the time period in which the cycle peaks) clearly surrounding the programmed feeding time in the absence of feed provision (Figure 3). The rhythm of activity in HD fish also disclosed a higher adjusted mean (M, mesor) and a wide range of variation (A, amplitude), which became statistically significant when the comparison was made with the other extreme group (LD fish) (intermediate values were achieved in MD fish). Over the entire diurnal recording period (09:00 to 21:00 h), correlation analyses highlighted a close positive lineal correlation (p < 0.001) between physical activity and respiratory frequency for all tracked fish considered as a whole (Figure 4A), which in turn rendered a decreased respiration/activity ratio with the increase of stocking densities (Figure 4B). With independence of this, the respiration tracking rendered a strong negative correlation (p < 0.001) with the continuously recorded water O2 concentration (Figure 4C).
FIGURE 3. Gilthead sea bream physical activity synchronization by feeding at low density (A), medium density (B) and high density (C). AEFishBIT data (measures taken every 15 min along 2 consecutive days) of representative individuals (n = 8) is shown as a continuous dotted line in each panel. Best-fit curve (red sinusoidal line) derived from the cosinor analysis of physical activity is only represented in the HD group. Values of mesor (M), amplitude (A), acrophase (φ) and p-value (P) of best-fit curves are shown for each density. Gray shaded areas represent dark phases. Arrow in vertical dotted line indicates the feeding time. Different letters represent significant (p < 0.05, ANOVA, Holm-Sidak test) differences between density groups.
FIGURE 4. (A) Correlation plot of respiratory frequency and physical activity. (B) Respiratory frequency/physical activity ratio in each stocking density group. Different letters represent significant (p < 0.05, ANOVA, Holm-Sidak test) differences between groups. (C) Correlation plot of respiratory frequency and water dissolved oxygen concentration. Values are the mean of physical activity and/or respiratory frequency (n = 8) of each density treatment and dissolved oxygen concentration each 15 min during the diurnal period.
All the genes in the liver and muscle PCR-arrays were expressed at detectable levels. In liver, up to 10 genes were differentially expressed by One-way ANOVA (Table 3), being 6 downregulated (igf1, igf2, cyp7a1, pparα, cox1, cox2) and 4 upregulated (igfbp1a, scd1a, mn-sod/sod2, grp94) in HD fish in comparison to LD fish. The hepatic expression pattern of MD fish was related to HD fish rather than LD fish, and PLS-DA was able to differentiate LD fish and MD-HD fish with 20 genes out of 44 of discriminant value (VIP >1.0) (Figure 5A). Among them, up to 16 were downregulated in HD-MD fish. The fitness and predictability of the PLS-DA model was validated by a 500-random permutation test (Supplementary Figure S3A) (p < 0.05), explaining the two first components the 83% and 66% of the observed and predicted variance, respectively.
TABLE 3. Relative gene expression of liver mRNA transcripts of fish reared at three different stocking densities (final density; 8.5-17-25 kg/m3). Values are the mean ± SEM of 10–12 fish per experimental condition. All data are in reference to the expression level of gpx1 in fish from LD group with an arbitrary value of 1. Different letters indicate statistically significant differences (Holm-Sidak post hoc test, p < 0.05). Differentially expressed genes are in bold.
FIGURE 5. Two-dimensional PLS-DA score plot of (A) liver and (B) white skeletal muscle molecular signatures of fish under different stocking densities, representing the distribution of the samples between the first two components in the model. Top left inserted figures represent the cumulative explained [R2Y(cum), green bars] and predicted [Q2(cum), blue bars] variance. Blue tables represent the ordered list of markers by variable importance in the projection (VIP) of the PLS-DA model. Upregulated genes are indicated by VIP values in bold and downregulated genes are shown as VIP values in regular font.
Gene expression profiling of white skeletal muscle displayed significant differences among experimental groups with 9 upregulated genes (ghr2, igf2, igfbp3a, il1β, sirt1, sirt2, grp170, grp94, grp75) and one downregulated gene (ghr1) in HD fish by One-way ANOVA (Table 4). However, in this case, MD fish was related to LD fish rather than HD fish, and PLS-DA was able to differentiate HD fish from MD-LD fish with 13 genes out of 44 of discriminant value (VIP >1.0). Among them, up to 12 were upregulated in HD fish (Figure 5B). The fitness and predictability of the PLS-DA model was validated by a 500-random permutation test (Supplementary Figure S3B) (p < 0.01), explaining the two first components the 88% and 67% of the observed and predicted variance, respectively.
TABLE 4. Relative gene expression of white skeletal muscle mRNA transcripts of fish reared at three different stocking densities (final density; 8.5-17-25 kg/m3). Values are the mean ± SEM of 10–12 fish per experimental condition. All data are in reference to the expression level of gpx4 in fish from LD group with an arbitrary value of 1. Different letters indicate statistically significant differences (Holm-Sidak post hoc test, p < 0.05). Differentially expressed genes are in bold.
Based on the differential HD outcomes on growth performance, external damage, behavior, plasma stress markers and tissue gene expression, a biomarker network was built to disclose at a glance the tissue-specific adaptive responses of liver and white skeletal muscle in this group of fish. In the case of liver, a total of 19 significant correlations (p ≤ 0.05) were established linking hepatic discriminant genes with other welfare indicators (Figure 6A). A direct link between CFK and SGR was presented at the center of the hub, being the rest of markers indirectly connected by different discriminant genes. Hence, SGR and CFK were positively correlated with igf1 and igf2, which were at the same time negatively correlated with physical activity and plasma cortisol, respectively. Moreover, plasma cortisol was negatively correlated with cyp7a1 and the igf1/igf2 ratio. The same trend was found between physical activity and the antioxidant defense gene prdx5. The CFK was also positively correlated with other defense antioxidant enzymes (mn-sod, gpx4) and lipid metabolism-related genes (cyp7a1, pparγ). Lastly, the pparγ was negatively correlated with the respiratory frequency, while plasma glucose was positively correlated with signs of skin damage and with gpx4 and mn-sod genes. There was also a positive correlation between skin damage and gpx4. The significance of all the gathered interrelationships with hepatic transcripts can be accessed in Supplementary Table S3.
FIGURE 6. (A) Diagram network showing the positive (green) and negative (red) significant (p < 0.05) Spearman correlation coefficients (7–12 fish from the HD group) between hepatic genes (VIP > 1), biometric indicators (SGR, CFK, Fat and HSI), external damage (Skin) and behavioral parameters (Activity and Respiration), and blood stress markers (Glucose and Cortisol). (B) Correlation matrix showing graphically the entire set of correlation coefficients. The values of Spearman correlation coefficients can be accessed at Supplementary Table S3.
Regarding the white skeletal muscle, a total of 21 significant correlations (p < 0.05) were established among muscle discriminant genes, biometric, behavioral and biochemical blood markers (Figure 7A). As in liver, the SGR-CFK link was indirectly connected to the rest of welfare indicators through discriminant genes. SGR was positively correlated with hif1α, igf1/igf2 ratio and ghr2. At the same time, physical activity was indirectly connected to SGR through ghr2 and igf1/igf2, either in a positive or negative manner, respectively. Physical activity was also positively correlated with a muscle cell growth marker (myod1), and the same trend was found between myod1 and respiratory frequency. Genes related to Gh/Igf system (igf2 and ghr1) were also positively correlated with respiration. On the other side of the SGR-CFK link, sirt2 was positively correlated with CFK, plasma glucose and skin damage. Plasma glucose was negatively correlated with muscle fat content, and positively correlated with sirt1, gr and skin damage. In a similar way, skin damage was negatively correlated with muscle fat content, and positively correlated with antioxidant defense genes (gr, grp75). By last, muscle fat content was negatively correlated with gr and grp170. The significance of all the gathered interrelationships with muscle transcripts can be accessed in Supplementary Table S4.
FIGURE 7. (A) Diagram network showing the positive (green) and negative (red) significant (p < 0.05) Spearman correlation coefficients (7–12 fish from the HD group) between white skeletal muscle genes (VIP > 1), biometric indicators (SGR, CFK, Fat and HSI), external damage (Skin) and behavioral parameters (Activity and Respiration), and blood stress markers (Glucose and Cortisol). (B) Correlation matrix showing graphically the entire set of correlation coefficients. The values of Spearman correlation coefficients can be accessed at Supplementary Table S4.
The negative impact of climate change in fisheries and aquaculture has been extensively studied and reviewed at regional and global scales (FAO, 2020; Maulu et al., 2021). Most studies, however, tend to explore the negative effects of climate change, while giving far less attention to the positive ones that are especially critical for the adaptation strategies. Certainly, warmer periods can promote shorter growing periods, helping to the expansion of aquaculture production in both temperate and cold regions, though such positive achievements become outweighed below the Limiting Oxygen Saturation (LOS) level, defined as the threshold level where regulatory mechanism are no longer sufficient to maintain O2 consumption without compromising any physiological function (Remen et al., 2015; Remen et al., 2016). Thus, in the present study, fish stocked at the highest density (25 kg/m3, 45%–60% O2 saturation level) experienced an impaired growth in comparison to fish reared at low (LD, 8.5 kg/m3) or intermediate (MD, 17 kg/m3) densities. Similar results were reported by Carbonara et al. (2019) at 15–30 kg/m3, but negative effects on growth performance were avoided in the range of 5–20 kg/m3 when the water O2 concentration remained above 55%–70% saturation level (Araújo-Luna et al., 2018). Moreover, the rearing density can be increased up to 36–44 kg/m3 without drawback effects in growth performance when the water O2 level is maintained above the 100% saturation (Parma et al., 2020). Thus, as pointed out by Saraiva et al. (2022), the effects of stocking density on fish welfare are complex and would involve many interacting factors. This notion is supported at the transcriptional level by changes in the tissue-specific expression patterns with a different tissue orchestration of the stress response, according to the nature and intensity of the hypoxic and crowding stress challenge (Martos-Sitcha et al., 2019a). Thus, the liver and heart of gilthead sea bream mostly contributed to cope with a global hypoxic response, involving changes in energy sensing, antioxidant defense and tissue repair. By contrast, the skeletal muscle disclosed changes in the expression pattern of the components of the Gh/Igf system that appears more related to changes in fish density and behavioral traits rather than water O2 levels. In any case, wide-transcriptomic analysis in zebrafish (Danio rerio), Atlantic salmon and European sea bass did not highlight a conserved transcriptomic signature for a proactive behavior across fish species (Rey et al., 2021), which is perhaps indicative of the complexity and polygenic nature of the behavioral phenotype. Indeed, as discussed below, our gathered biomarker approach was able to connect proactive behavior in HD fish with locally regulated growth, while systemic growth regulation via the liver Gh/Igf system was apparently more related with a reactive coping stress style.
It is worth noting that gilthead sea bream is a schooling fish that displays social hierarchies for the use of space and competition for feed (Goldan et al., 2003; Montero et al., 2009; Arechavala-Lopez et al., 2019; Oikonomidou et al., 2019), which reinforced an enhanced physical activity in HD environments (Carbonara et al., 2019). Similarly, we found herein that measurements of body tail jerk accelerations at a high recording frequency clearly highlighted an endogenous swimming activity rhythm with a higher amplitude and adjusted-mean in the group of HD fish (Figure 3). This occurred in parallel with the rise of respiratory frequency (Figure 4A), though this in turn resulted in a slight but progressive decrease of the respiration/activity ratio from LD to HD fish (Figure 4B). Such metabolic feature would be indicative of a decreased energy partitioning for growth in a HD environment with a limited O2 availability, which makes sense with the observed FCR impairment in this group of fish (Table 2). Certainly, the association of better growth with a lower activity is a well-known selected pattern in livestock production (Rosenfeld et al., 2015; Sibly et al., 2015). According to this, the behavioral monitoring with the AEFishBIT accelerometer have rendered divergent patterns of energy use for growth and activity across representative European farmed fish (gilthead sea bream, European sea bass and Atlantic salmon) (Ferrer et al., 2020; Kolarevic et al., 2021; Rosell-Moll et al., 2021), but also in genetically improved gilthead sea bream for either growth or FCR (Perera et al., 2021; Calduch-Giner et al., 2023). Such genetic progress has an impact in fillet yield (Besson et al., 2022), and perhaps other productive traits affected by the establishment of different social hierarchies and interactions. In that sense, Arechavala-Lopez et al. (2020) pointed out that the time of the first gilthead sea bream response during hypoxia or risk-taking tests is shorter in HD than in LD environments, which might lead to a higher competitiveness for feed if it is not provided in a sufficient quantity and quality. At the same time, however, HD environments can serve to better synchronize the phase of locomotor and metabolic rhythms with the daily feeding time, generating an internal rhythmicity close to 24 h for a better synchronization of the individuals with the environment and their congeners (Mistlberger, 2011; Challet, 2019). This notion was fitted herein by the better cosinor adjustment of the recorded jerk accelerations in the HD fish than in the other two experimental groups (Figure 3). Feeding frequency also operates in a similar manner, and fish fed with a single meal per day showed a higher ability to concentrate the self-feeding activity around the programmed meal in comparison to those fed hourly during a 3h-window (Calduch-Giner et al., 2022). Moreover, gilthead sea bream becomes mostly arrhythmic in winter, but the use of feeding schedules with alternate days (maintaining constant the total feed intake, but with an increased supply by the feeding day) restored the typical feeding behavior of the warm season (active feeding period). The pervasiveness of biological rhythms is, thereby, adaptive in nature on a daily and/or seasonal basis, functioning as a timing reference that allows organisms to anticipate and take advantage of the diel fluctuations in their environments (Van der Zee et al., 2008). The bad thing is that the increased contact among individuals in a HD environment might lead to physical injuries (Oppedal et al., 2011; Sveen et al., 2018; Weirup et al., 2021), promoting an aggressive behavior rather than an improved individual social cohesion by the feeding time zeitgeber. In the present study, aggressive interactions among individuals are not specifically monitored, but visual observations did not reveal biting-chasing activities before-after feeding in any fish group, and more importantly, correlation analysis within the HD group highlighted a positive association between growth and skin erosion and damage (Figure 7). Therefore, it appears likely that the external body signs of welfare impairment in this group of fish (Figure 2) would be primarily due to an active feeding behavior with involuntary collisions during feed dispensation. How this non-desirable effect can be solved by more appropriate feeding schedules and housing environments is becoming an important challenge for the future development of aquaculture in a scenario of a limited space allowance for livestock production.
Plasma/serum cortisol is currently the most widely used stress biomarker in fish (Sadoul and Geffroy, 2019; Noble et al., 2020), though its reliability is limited by a number of drawbacks, including among others: i) exhaustion or adaptive HPI-axis habituation to chronic stress, ii) dramatic increase in circulating cortisol levels by sampling itself, and iii) high biological variability within and among species that hampers a clear conclusion of stress condition. This makes sense because the underlying hypothesis for the fitted low plasma cortisol levels with the increase of stocking density is that acute stress sampling can become minimized in fish coming from a HD environment (van de Nieuwegiessen et al., 2009; Santos et al., 2010; Othman et al., 2022). Furthermore, it was previously established that the regulation of gilthead sea bream plasma cortisol levels is largely dependent of the type and intensity of stressor (Bermejo-Nogales et al., 2014). It is expected, therefore, that the use of alternative water and fish scales matrix-samples gain more interest in the forthcoming years as a less invasive and reproducible cortisol assessment approach (Fanouraki et al., 2011; Weirup et al., 2021; Vercauteren et al., 2022). In the meantime, cortisol measurements should be supported by other stress indicators for an accurate assessment of fish stress/welfare status. Thus, we considered herein plasma cortisol levels as part of an integrative biomarker procedure in which the measured levels in LD fish were in the lower range of values reported for unstressed gilthead sea bream, while those from MD and HD resulted almost doubled (Table 2). The closeness found between cortisol levels of MD and HD fish can also be indicative of the different discriminant value of the assessed stress/welfare indicators. Indeed, liver and muscle gene expression patterns disclosed a different gene clustering, according to which the expression signature of MD was closer to HD in liver and to LD in muscle (Figure 5). With independence of this, the high plasma cortisol levels in HD fish were associated with a reduced growth through changes in hepatic igf2 and cyp7α1 expression (Figure 6), which can be considered adaptive in nature in fish facing a limited O2 availability that was not able to support the maximum growth of the species.
Cortisol works raising circulating glucose levels across species (Mommsen et al., 1999; Sapolsky et al., 2000), and their concurrent increase would reflect the nature and intensity of stress stimuli as well as the different stress species-specific susceptibility (Fanouraki et al., 2011; Bordin and Freire, 2021). Thus, both glucose and cortisol levels increased at a population level in a HD environment (Table 2), but the gathered biomarker analysis revealed a negative correlation between them in HD fish via the interconnections driven by different hepatic molecular transcripts (Figure 6), which might dictate a negative feedback loop that limits cortisol release promoting the use of blood glucose not only as a fast metabolic fuel, but also as a major antioxidant agent. Certainly, the rerouting of glucose into a pentose-phosphate-pathway (PPP) is a major protective mechanism to counteract acute and severe oxidative stress (Ralser et al., 2007; Stincone et al., 2015) through the production of NADPH that is required for the regeneration of reduced glutathione, a well-known antioxidant that is present in most living cells from bacteria to mammals (Hamilton et al., 2014). At the same time, however, there are several mechanisms contributing to oxidative stress during diabetes and hyperglycemia, which is indicative that exists a delicate balance between the protective and damaging effects of glucose to maintain redox homeostasis through the evolution (Cherkas et al., 2020). In the present study, the protective glucose effects in HD fish were shaped by a positive association of glucose with a number of antioxidant enzymes (mn-sod, gpx4, prdx5) in the liver (Figure 6) and glucose responsive proteins (grp170, grp75) in skeletal muscle (Figure 7), all of them already identified as highly stress-responsive genes in gilthead sea bream (Bermejo-Nogales et al., 2008; Saera-Vila et al., 2009; Calduch-Giner et al., 2010; Pérez-Sánchez et al., 2013; Malandrakis et al., 2014; Magnoni et al., 2017; Martos-Sitcha et al., 2019a; Naya-Català et al., 2021). Indeed, regardless of glucose-gene correlations, the overall trend in both liver and skeletal muscle was the upregulated expression of grp genes (grp170, grp94 and grp75) in HD fish. Such genes are normally overexpressed when cells are starved of glucose (Tanaka et al., 1988; Franklin et al., 2022), but the opposite is also true and the grp75 was consistently upregulated with the increase of glycemia by crowding stress in gilthead sea bream pair-fed fish (Bermejo-Nogales et al., 2008). Likewise, when comparisons are made between gilthead sea bream and common dentex (Dentex dentex, a highly sensitive sparid fish to handling stress), this another sparid fish exhibited higher levels of secondary stress markers (glucose, lactate) in combination with an increased expression of pro-inflammatory cytokines and non-enzymatic antioxidant genes, such as grp75 and metallothionein (mt) (Bermejo-Nogales et al., 2007). Most of these stress-responsive genes are primarily located in the mitochondria and endoplasmic reticulum, scavenging the production of reactive oxygen species (ROS) (Rahman et al., 2017; Dhamad et al., 2020). Accordingly, we found herein that the fine adjustments of metabolism in HD fish was also encompassed by the hepatic downregulation of catalytic enzyme subunits of the mitochondrial respiration chain (Complex IV; cox1, cox2) and the well-known lipolytic transcription factor pparα, which in turn resulted in the upregulated expression of a strong lipogenic marker, the scd1a (Table 3). This transcriptional signature is prone to promote a general depletion of oxidative metabolism, redirecting the surplus of metabolic fuels towards lipid storage as reported elsewhere during episodes of heat stress in broilers (Guo et al., 2021; Lan et al., 2022). However, this changing transcriptional signature is part of a more complex and extensive metabolic reprograming of lipid metabolism that also included a strong-down regulation of the hepatic cyp7α1, the first rate limiting enzyme of bile acid synthesis from cholesterol (Chiang and Ferrell, 2020). This would adjust the effective dietary fat absorption to O2 availability, according to the oxystatic theory that assumes that voluntary feed intake is limited by the maximal physiological capacity of O2 usage (Saravanan et al., 2012). Conversely, the consumption of cholesterol-rich diets can promote the shift of carbohydrate to lipid metabolism, preventing strong hypoglycemic effects following exposure to acute hypoxic stress (Miron and Tirosh, 2019). In any case, most adaptive hypoxia responses appear to be tissue-specific (Martos-Sitcha et al., 2019a), and in the absence of transcriptional changes in markers of oxidative metabolism, the skeletal muscle of HD fish disclosed a pronounced upregulation of sirt1 and sirt2 (Table 4). Such metabolic feature is viewed as a higher energy demanding condition that is susceptible to be epigenetically regulated in the case of sirt1 by changes in the DNA methylation pattern of several CpG positions of a CG island close to the transcription start site (Simó-Mirabet et al., 2020). Indeed, it is well known that fasting upregulated the expression of sirt1 in the skeletal muscle of gilthead sea bream (Simó-Mirabet et al., 2017). However, the muscle expression of sirt2 appeared poorly responsive to nutrient deprivation, but it was upregulated in a fast growing fish strain (Simó-Mirabet et al., 2018). If this is also part of an adaptive feature to preserve muscle growth in HD fish warrants further research.
Like the confounding regulation of glucose and redox homeostasis, Gh-transgenic fish have a limited capacity to manage hypoxic environments efficiently (McKenzie et al., 2003; Almeida et al., 2013), though paradoxically studies in mammals indicate that circulating GH can be increased by either the increase in O2 requirements or the reduction in O2 availability (VanHelder et al., 1987). In that way, diet and exercise modulate the activity of the Gh/Igf axis in gilthead sea bream (Perelló-Amorós et al., 2021), and circulating Gh during steady states is becoming a subrogate marker of critical swimming speed (swimming activity that can be maintained theoretically indefinitely without exhaustion) (Martos-Sitcha et al., 2018). However, circulating Gh was lowered after acute or chronic confinement in a wide range of species, including tilapia (Aupérin et al., 1997), salmonids (Wilkinson et al., 2006) and gilthead sea bream (Saera-Vila et al., 2009). In the present study, circulating Gh was not measured, but the downregulated expression of hepatic igf1 and igf2 in HD fish highlighted a lower sensitivity of liver to the anabolic action of Gh, probably via post-transcriptional mechanisms because the expression of both ghr1 and ghr2 remained almost unaltered among all fish groups (Table 3). However, as reviewed in Pérez-Sánchez et al., 2018, and most recently stated during early development (Naya-Català et al., 2021), a large body of evidence highlighted the tissue-specific regulation of the two Ghr and Igf subtypes by nutrition and season across development in gilthead sea bream. Certainly, in contrast to that found in the liver, the muscle expression of ghr2 was upregulated in HD fish in comparison to the other two experimental groups (Table 4), which would trigger a compensatory growth response through the enhanced expression of igf2 as reported elsewhere in fish fed alternative feeds or semi-synthetic diets formulated to be deficient in specific nutrients (Ballester-Lozano et al., 2015; Pérez-Sánchez et al., 2018). Thus, as also pointed out before, the varying contribution of systemic (via liver Gh/Igf axis) and local growth-promoting actions on global growth are indicative of a different welfare condition and metabolic readjustment of the endocrine-growth cascade. This notion was further supported by the expression pattern of the two phylogenetically and functionally divergent clades of Igfbp1/2/4 and Igfbp3/4/6 (see Pérez-Sánchez et al., 2018), which resulted herein in an enhanced expression of hepatic igfp1a and muscle igfbp3a in HD fish (Tables 3 and 4). A common role of Igfbps among the fish lineage has not been established, but transgenic studies support a main role of Igfbp1 as a negative regulator of fish growth. Thus, igfbp1 knockdown alleviates the hypoxic growth and development delay in zebrafish, whereas its overexpression caused growth and development retardation under normoxia (Kajimura et al., 2005). By contrast, the enhanced igfbp3 expression has been related to growth acceleration by Gh-transgenesis in coho salmon (Oncorhynchus kisutch) (Alzaid et al., 2018). Therefore, it appears likely that all the observed transcriptional changes in the Gh/Igf axis are adaptive attempts to drive a different contribution of systemic and local growth regulatory mechanisms. According to this, our integrative biomarker survey disclosed negative correlations of hepatic Gh/Igf markers (igf1, igf2) with behavioral measurements of activity/respiration, whereas the opposite was found for muscle components of the Gh/Igf system (igf2, ghr1, ghr2), according to which this growth-regulatory transition was prone to a proactive instead of a reactive behavior. The way in which this differential tissue regulation was driven by a different threshold level of O2 sensors requires further warrant, though it is noteworthy that the hif1α, a master regulator of hypoxia-mediated responses, was apparently more sensitive to the changing crowding and hypoxic condition in muscle (p < 0.1) than in liver, being also positively correlated with the individual changes in growth within the HD fish population (Table 4; Figure 7).
In summary, an integrative approach depicting the way in which high stocking densities, accompanied by a decrease in O2 levels, drive different adaptive features in behavior, growth, antioxidant defense and lipid metabolism has been shown. Of particular relevance are the concomitant changes in behavior, antioxidant defense and growth regulatory mechanisms, which emphasizes on the importance of adaptive stress responses from the cell to the global organism level. The challenge is to translate this new knowledge into effective measures that serve to mitigate the drawback effects of a challenging and poor predictable milieu in a context of global warming, where the fish life history can largely affect the capacity of the farmed livestock to cope with most of the envisaged production threats (see Alfonso et al., 2021). According to the revisited paradigm of epigenetic nutritional/environmental regulation, this assumes the convenience of a precise and adjusted metabolic regulation over time that avoids as much as possible excessive counter-regulatory responses (Belenguer et al., 2023). Meanwhile, the present study has contributed to prescribe new and more appropriate values of welfare indicators for a better definition of a golden stocking density in a challenging environment that mimics the crowding and hypoxic stress condition of most Mediterranean farms during the summer on-growing finishing phase.
The raw data supporting the conclusion of this article will be made available by the authors, without undue reservation.
The animal study was approved by the Animal Welfare Committee of the Institute of Aquaculture Torre de la Sal (IATS), CSIC Ethics Comittee (permission 1295/2022) and Generalitat Valenciana (permission 2022-VSC-PEA-0230). The study was conducted in accordance with the local legislation and institutional requirements.
PH: Formal Analysis, Methodology, Writing–original draft, Writing–review and editing. FN-C: Formal Analysis, Methodology, Writing–review and editing. ÁB: Methodology, Writing–review and editing. JC-G: Funding acquisition, Methodology, Writing–review and editing. JP-S: Conceptualization, Formal Analysis, Funding acquisition, Methodology, Writing–original draft, Writing–review and editing.
The author(s) declare financial support was received for the research, authorship, and/or publication of this article. This work was supported by the EU H2020 Research Innovation Program under grant agreement no. 871108 (AQUAEXCEL3.0). This output reflects only the author’s view and the European Union cannot be held responsible for any use that may be made of the information contained therein. Additionally, this study forms part of the ThinkInAzul program and was supported by MCIN with funding from European Union NextGenerationEU (PRTR-C17.I1) and by Generalitat Valenciana (THINKINAZUL/2021/024).
We acknowledge the support of Inmaculada Vicent from the Animalarium Service of IATS for her help during fish rearing and sampling. The authors are grateful to María Angeles González for her assistance on PCR analyses. They also acknowledge the effort of personnel from CSIC (Manuel Lozano, Enric Cabruja) and University of Las Palmas de Gran Canaria (Juan Manuel Afonso, Miguel Àngel Ferrer, Juan Antonio Montiel-Nelson, Javier Sosa) in the previous work of design and validation leading to the invention of the AEFishBIT device.
The authors declare that the research was conducted in the absence of any commercial or financial relationships that could be construed as a potential conflict of interest.
All claims expressed in this article are solely those of the authors and do not necessarily represent those of their affiliated organizations, or those of the publisher, the editors and the reviewers. Any product that may be evaluated in this article, or claim that may be made by its manufacturer, is not guaranteed or endorsed by the publisher.
The Supplementary Material for this article can be found online at: https://www.frontiersin.org/articles/10.3389/fphys.2023.1272267/full#supplementary-material
SUPPLEMENTARY FIGURE S1 | Welfare scoring images of (A) skin erosion, (B) caudal fin status, (C) pelvic fin status and (D) pectoral fin status. Numbers in parenthesis indicate the assigned welfare score.
SUPPLEMENTARY FIGURE S2 | Gilthead sea bream physical activity synchronization by feeding at low density (A), medium density (B) and high density (C), and respiratory frequency synchronization by feeding at low density (D), medium density (E) and high density (F). AEFishBIT data (measures taken every 15 min along 2 consecutive days) of representative individuals (n = 8) is shown as a continuous dotted line in each panel. Best-fit curves (red sinusoidal line) derived from the cosinor analysis of physical activity and respiratory frequency are represented in the HD group. Values of mesor (M), amplitude (A), acrophase (φ) and p-value (P) of best-fit curves are shown for each density. Gray shaded areas represent dark phases. Arrow in vertical dotted line indicates the feeding time. Different letters represent significant (P < 0.05, ANOVA, Holm-Sidak test) differences between density groups.
SUPPLEMENTARY FIGURE S3 | Validation plots of the PLS-DA models in Figures 5A, B (A,B) consisting in 500 random permutations each.
SUPPLEMENTARY TABLE S3 | Pearson correlation matrix among outcomes on growth performance, skin damage, behavior, plasma stress markers and hepatic gene expression in HD fish. Upper values are the correlation coefficients and lower values are the p-values.
SUPPLEMENTARY TABLE S4 | Pearson correlation matrix among outcomes on growth performance, skin damage, behavior, plasma stress markers and muscle gene expression in HD fish. Upper values are the correlation coefficients and lower values are the p-values.
Alfonso S., Gesto M., Sadoul B. (2021). Temperature increase and its effects on fish stress physiology in the context of global warming. J. Fish Biol. 98, 1496–1508. doi:10.1111/jfb.14599
Almeida D. V., Bianchini A., Marins L. F. (2013). Growth hormone overexpression generates an unfavorable phenotype in juvenile transgenic zebrafish under hypoxic conditions. General Comp. Endocrinol. 194, 102–109. doi:10.1016/j.ygcen.2013.08.017
Alzaid A., Kim J. H., Devlin R. H., Martin S. A., MacQueen D. J. (2018). Growth hormone transgenesis in coho salmon disrupts muscle immune function impacting cross-talk with growth systems. J. Exp. Biol. 221, jeb173146. doi:10.1242/jeb.173146
Araújo-Luna R., Ribeiro L., Bergheim A., Pousão-Ferreira P. (2018). The impact of different rearing condition on gilthead seabream welfare: dissolved oxygen levels and stocking densities. Aquacult. Res. 49, 3845–3855. doi:10.1111/are.13851
Arechavala-Lopez P., Diaz-Gil C., Saraiva J. L., Moranta D., Castanheira M. F., Nuñez-Velázquez S., et al. (2019). Effects of structural environmental enrichment on welfare of juvenile seabream (Sparus aurata). Aquac. Rep. 15, 100224. doi:10.1016/j.aqrep.2019.100224
Arechavala-Lopez P., Nazzaro-Alvarez J., Jardí-Pons A., Reig L., Carella F., Carrassón M., et al. (2020). Linking stocking densities and feeding strategies with social and individual stress responses on gilthead seabream (Sparus aurata). Physiol. Behav. 213, 112723. doi:10.1016/j.physbeh.2019.112723
Ashley P. J. (2007). Fish welfare: current issues in aquaculture. Appl. Anim. Behav. Sci. 104, 199–235. doi:10.1016/j.applanim.2006.09.001
Aupérin B., Baroiller J. F., Ricordel M. J., Fostier A., Prunet P. (1997). Effect of confinement stress on circulating levels of growth hormone and two prolactins in freshwater-adapted tilapia (Oreochromis niloticus). General Comp. Endocrinol. 108, 35–44. doi:10.1006/gcen.1997.6938
Baldwin L. (2011). The effects of stocking density on fish welfare. Plymouth Student Sci. 4, 372–383.
Ballester-Lozano G. F., Benedito-Palos L., Estensoro I., Sitjà-Bobadilla A., Kaushik S., Pérez-Sánchez J. (2015). Comprehensive biometric, biochemical and histopathological assessment of nutrient deficiencies in gilthead sea bream fed semi-purified diets. Br. J. Nutr. 114, 713–726. doi:10.1017/S0007114515002354
Belenguer A., Naya-Català F., Montero D., Torrecillas S., Soriano B., Calduch-Giner J., et al. (2023). Genetics drives hepatic transcriptome and DNA methylome of farmed gilthead sea bream after broodstock nutritional programming. Viena, Austria: Aquaculture Europe 2023 Congress.
Bermejo-Nogales A., Benedito-Palos L., Saera-Vila A., Calduch-Giner J. A., Sitjà-Bobadilla A., Pérez-Sánchez J. (2008). Confinement exposure induces glucose regulated protein 75 (GRP75/mortalin/mtHsp70/PBP74/HSPA9B) in the hepatic tissue of gilthead sea bream (Sparus aurata L.). Comp. Biochem. Physiology B 149, 428–438. doi:10.1016/j.cbpb.2007.11.003
Bermejo-Nogales A., Nederlof M., Benedito-Palos L., Ballester-Lozano G. F., Folkedal O., Olsen R. E., et al. (2014). Metabolic and transcriptional responses of gilthead sea bream (Sparus aurata L.) to environmental stress: new insights in fish mitochondrial phenotyping. General Comp. Endocrinol. 205, 305–315. doi:10.1016/j.ygcen.2014.04.016
Bermejo-Nogales A., Saera-Vila A., Calduch-Giner J. A., Navarro J. C., Sitjà-Bobadilla A., Pérez-Sánchez J. (2007). Differential metabolic and gene expression profile of juvenile common dentex (Dentex dentex L.) and gilthead sea bream (Sparus aurata L.) in relation to redox homeostasis. Aquaculture 267, 213–224. doi:10.1016/j.aquaculture.2007.01.024
Besson M., Rombout N., Salou G., Vergnet A., Cariou S., Bruant J. S., et al. (2022). Potential for genomic selection on feed efficiency in Gilthead sea bream (Sparus aurata), based on individual feed conversion ratio, carcass and lipid traits. Aquac. Rep. 24, 101132. doi:10.1016/j.aqrep.2022.101132
Bordin D., Freire C. A. (2021). Remarkable variability in stress responses among subtropical coastal marine teleosts. Mar. Biol. 168, 122–217. doi:10.1007/s00227-021-03929-5
Calabrese S., Nilsen T. O., Kolarevic J., EbbessonPedrosa L. O. E. C., Fivelstad S., et al. (2017). Stocking density limits for post-smolt Atlantic salmon (Salmo salar L.) with emphasis on production performance and welfare. Aquaculture 468, 363–370. doi:10.1016/j.aquaculture.2016.10.041
Calduch Giner J., Rosell-Moll E., Besson M., Vergnet A., Bruan J.-S., Clota F., et al. (2023). Changes in transcriptomic and behavioural traits in activity and ventilation rates associated with divergent individual feed efficiency in gilthead sea bream (Sparus aurata). Aquac. Rep. 29, 101476. doi:10.1016/j.aqrep.2023.101476
Calduch-Giner J. A., Davey G., Saera-Vila A., Houeix B., Talbot A., Prunet P., et al. (2010). Use of microarray technology to assess the time course of liver stress response after confinement exposure in gilthead sea bream (Sparus aurata L.). BMC Genomics 11, 193. doi:10.1186/1471-2164-11-193
Calduch-Giner J., Holhorea P. G., Ferrer M. Á., Naya-Català F., Rosell-Moll E., Vega García C., et al. (2022). Revising the impact and prospects of activity and ventilation rate bio-loggers for tracking welfare and fish-environment interactions in salmonids and Mediterranean farmed fish. Front. Mar. Sci. 9, 854888. doi:10.3389/fmars.2022.854888
Carbonara P., Alfonso S., Zupa W., Manfrin A., Fiocchi E., Pretto T., et al. (2019). Behavioral and physiological responses to stocking density in sea bream (Sparus aurata): do coping styles matter? Physiol. Behav. 212, 112698. doi:10.1016/j.physbeh.2019.112698
Castanheira M. F., Cerqueira M., Millot S., Gonçalves R. A., Oliveira C. C. V., Conceição L. E. C., et al. (2016). Are personality traits consistent in fish? The influence of social context. Appl. Anim. Behav. Sci. 178, 96–101. doi:10.1016/j.applanim.2016.02.004
Challet E. (2019). The circadian regulation of food intake. Nat. Rev. Endocrinol. 15, 393–405. doi:10.1038/s41574-019-0210-x
Cherkas A., Holota S., Mdzinarashvili T., Gabbianelli R., Zarkovic N. (2020). Glucose as a major antioxidant: when, what for, and why it fails? Antioxidants 9, 140. doi:10.3390/antiox9020140
Chiang J. Y., Ferrell J. M. (2020). Up to date on cholesterol 7 alpha-hydroxylase (CYP7A1) in bile acid synthesis. Liver Res. 4, 47–63. doi:10.1016/j.livres.2020.05.001
Dhamad A. E., Greene E., Sales M., Nguyen P., Beer L., Liyanage R., et al. (2020). 75-kDa glucose-regulated protein (GRP75) is a novel molecular signature for heat stress response in avian species. Am. J. Physiology-Cell Physiology 318, C289–C303. doi:10.1152/ajpcell.00334.2019
Ellis T., North B., Scott A. P., Bromage N. R., Porter M., Gadd D. (2002). The relationships between stocking density and welfare in farmed rainbow trout. J. Fish. Biol. 61, 493–531. doi:10.1111/j.1095-8649.2002.tb00893.x
Fanouraki E., Mylonas C. C., Papandroulakis N., Pavlidis M. (2011). Species specificity in the magnitude and duration of the acute stress response in Mediterranean marine fish in culture. General Comp. Endocrinol. 173, 313–322. doi:10.1016/j.ygcen.2011.06.004
FAO (2020). The state of world fisheries and aquaculture 2020. Sustainability in action. Rome: Food and Agriculture Organization of the United Nations.
FAO (2022). The state of world fisheries and aquaculture 2022. Towards Blue Transformation. Rome: Food and Agriculture Organization of the United Nations. doi:10.4060/cc0461en
Ferrer M. A., Calduch-Giner J. A., Díaz M., Sosa J., Rosell-Moll E., Santana Abril J., et al. (2020). From operculum and body tail movements to different coupling of physical activity and respiratory frequency in farmed gilthead sea bream and European sea bass. Insights on aquaculture biosensing. Comput. Electron. Agric. 175, 105531. doi:10.1016/j.compag.2020.105531
Franklin J. L., Amsler M. O., Messina J. L. (2022). Regulation of glucose responsive protein (GRP) gene expression by insulin. Cell Stress Chaperones 27, 27–35. doi:10.1007/s12192-021-01243-z
Goldan O., Popper D., Karplus I. (2003). Food competition in small groups of juvenile gilthead seabream (Sparus aurata). Isr. J. Aquac. Bamidgeh 55, 94–106. doi:10.46989/001c.20340
Guo Y., Balasubramanian B., Zhao Z. H., Liu W. C. (2021). Heat stress alters serum lipid metabolism of Chinese indigenous broiler chickens-a lipidomics study. Environ. Sci. Pollut. Res. 28, 10707–10717. doi:10.1007/s11356-020-11348-0
Hamilton C. J., Arbach M., Groom M. (2014). “Beyond glutathione: different low molecular weight thiols as mediators of redox regulation and other metabolic functions in lower organisms,” in Recent advances in redox active plant and microbial products. Editors C. Jacob, G. Kirsch, A. Slusarenko, P. Winyard, and P. Burkholz (Dordrecht, Netherlands: Springer). doi:10.1007/978-94-017-8953-0_11
Hoyle I., Oidtmann B., Ellis T., Turnbull J., North B., Nikolaidis J., et al. (2007). A validated macroscopic key to assess fin damage in farmed rainbow trout (Oncorhynchus mykiss). Aquaculture 270, 142–148. doi:10.1016/j.aquaculture.2007.03.037
Jia R., Liu B. L., Feng W. R., Han C., Huang B., Lei J. L. (2016). Stress and immune responses in skin of turbot (Scophthalmus maximus) under different stocking densities. Fish Shellfish Immunol. 55, 131–139. doi:10.1016/j.fsi.2016.05.032
Kajimura S., Aida K., Duan C. (2005). Insulin-like growth factor-binding protein-1 (IGFBP-1) mediates hypoxia-induced embryonic growth and developmental retardation. Proc. Natl. Acad. Sci. 102, 1240–1245. doi:10.1073/pnas.0407443102
Kieffer D. A., Piccolo B. D., Vaziri N. D., Liu S., Lau W. L., Khazaeli M., et al. (2016). Resistant starch alters gut microbiome and metabolomic profiles concurrent with amelioration of chronic kidney disease in rats. Am. J. Physiol. Ren. Physiol. 310, F857–F871. doi:10.1152/ajprenal.00513.2015
Kolarevic J., Calduch-Giner J., Espmark Å. M., Evensen T., Sosa J., Pérez-Sánchez J. (2021). A novel miniaturized biosensor for monitoring Atlantic salmon swimming activity and respiratory frequency. Animals 11, 2403. doi:10.3390/ani11082403
Lan R., Wang Y., Wei L., Wu F., Yin F. (2022). Heat stress exposure changed liver lipid metabolism and abdominal fat deposition in broilers. Italian J. Animal Sci. 21, 1326–1333. doi:10.1080/1828051X.2022.2103461
Li H., Ma M.-L., Luo S., Zhang R.-M., Han P., Hu W. (2012). Metabolic responses to ethanol in Saccharomyces cerevisiae using a gas chromatography tandem mass spectrometry-based metabolomics approach. Int. J. Biochem. Cell Biol. 44, 1087–1096. doi:10.1016/j.biocel.2012.03.017
Liu B., Liu Y., Sun G. (2017). Effects of stocking density on growth performance and welfare-related physiological parameters of Atlantic salmon Salmo salar L. recirculating aquaculture system. Aquac. Res. 48, 2133–2144. doi:10.1111/are.13050
Livak K. J., Schmittgen T. D. (2001). Analysis of relative gene expression data using real-time quantitative PCR and the 2(-delta delta CT) method. Methods 25, 402–408. doi:10.1006/meth.2001.1262
Magnoni L. J., Martos-Sitcha J. A., Queiroz A., Calduch-Giner J. A., Magalhàes Gonçalves J. F., Rocha C. M. R., et al. (2017). Dietary supplementation of heat-treated Gracillaria and Ulva seaweeds enhanced acute hypoxia tolerance in gilthead seabream (Sparus aurata). Biol. Open 6, 897–908. doi:10.1242/bio.024299
Malandrakis E. E., Exadactylos A., Dadali O., Golomazou E., Klaoudatos S., Panagiotaki P., et al. (2014). Molecular cloning of four glutathione peroxidase (GPx) homologs and expression analysis during stress exposure of the marine teleost Sparus aurata. Comp. Biochem. Physiology Part B 168, 53–61. doi:10.1016/j.cbpb.2013.11.005
Martos-Sitcha J. A., Simó-Mirabet P., de las Heras V., Calduch-Giner J. A., Pérez-Sánchez J. (2019a). Tissue-specific orchestration of gilthead sea bream resilience to hypoxia and high stocking density. Front. Physiology 10, 840. doi:10.3389/fphys.2019.00840
Martos-Sitcha J. A., Simó-Mirabet P., Piazzon M. C., de las Heras V., Calduch-Giner J. A., Puyalto M., et al. (2018). Dietary sodium heptanoate helps to improve feed efficiency, growth hormone status and swimming performance in gilthead sea bream (Sparus aurata). Aquac. Nutr. 24, 1638–1651. doi:10.1111/anu.12799
Martos-Sitcha J. A., Sosa J., Ramos-Valido D., Bravo F. J., Carmona-Duarte C., Gomes H. L., et al. (2019b). Ultra-low power sensor devices for monitoring physical activity and respiratory frequency in farmed fish. Front. Physiology 10, 667. doi:10.3389/fphys.2019.00667
Maulu S., Hasimuna O. J., Haambiya L. H., Monde C., Musuka C. G., Makorwa T. H., et al. (2021). Climate change effects on aquaculture production: sustainability implications, mitigation, and adaptations. Front. Sustain. Food Syst. 5, 609097. doi:10.3389/fsufs.2021.609097
McKenzie D. J., Martinez R., Morales A., Acosta J., Morales R., Taylor E. W., et al. (2003). Effects of growth hormone transgenesis on metabolic rate, exercise performance and hypoxia tolerance in tilapia hybrids. J. Fish Biol. 63, 398–409. doi:10.1046/j.1095-8649.2003.00162.x
Miron N., Tirosh O. (2019). Cholesterol prevents hypoxia-induced hypoglycemia by regulation of a metabolic ketogenic shift. Oxidative Med. Cell. Longev. 2019, 5829357. doi:10.1155/2019/5829357
Mistlberger R. E. (2011). Neurobiology of food anticipatory circadian rhythms. Physiology Behav. 104, 535–545. doi:10.1016/j.physbeh.2011.04.015
Mommsen T. P., Vijayan M. M., Moon T. W. (1999). Cortisol in teleosts: dynamics, mechanisms of action, and metabolic regulation. Rev. Fish Biol. Fish. 9, 211–268. doi:10.1023/A:1008924418720
Montero D., Lalumera G., Izquierdo M. S., Caballero M. J., Saroglia M., Tort L. (2009). Establishment of dominance relationships in gilthead sea bream Sparus aurata juveniles during feeding: effects on feeding behaviour, feed utilization and fish health. J. Fish. Biol. 74, 790–805. doi:10.1111/j.1095-8649.2008.02161.x
Naya-Català F., Simó-Mirabet P., Calduch-Giner J., Pérez-Sánchez J. (2021). Transcriptomic profiling of Gh/Igf system reveals a prompted tissue-specific differentiation and novel hypoxia responsive genes in gilthead sea bream. Sci. Rep. 11, 16466. doi:10.1038/s41598-021-95408-6
Noble C., Gismervik K., Iversen M. H., Kolarevic J., Nilsson J., Stien L. H., et al. (2018). Welfare indicators for farmed atlantic salmon: tools for assessing fish welfare. Tromsø, Norway: Nofima.
Noble C., Gismervik K., Iversen M. H., Kolarevic J., Nilsson J., Stien L. H., et al. (2020). Welfare indicators for farmed rainbow trout: tools for assessing fish welfare. Tromsø, Norway: Nofima.
North B. P., Turnbull J. F., Ellis T., Porter M. J., Migaud H., Bron J., et al. (2006). The impact of stocking density on the welfare of rainbow trout (Oncorhynchus mykiss). Aquaculture 255, 466–479. doi:10.1016/j.aquaculture.2006.01.004
Oikonomidou E., Batzina A., Karakatsouli N. (2019). Effects of food quantity and distribution on aggressive behaviour of gilthead seabream and European seabass. Appl. Anim. Behav. Sci. 213, 124–130. doi:10.1016/j.applanim.2019.02.010
Ojala M., Garriga G. C. (2010). Permutation tests for studying classifier performance. J. Mach. Learn. Res. 11, 1833–1863. doi:10.5555/1756006.1859913
Oppedal F., Vågseth T., Dempster T., Juell J.-E., Johansson D. (2011). Fluctuating sea-cage environments modify the effects of stocking densities on production and welfare parameters of Atlantic salmon (Salmo salar L.). Aquaculture 315, 361–368. doi:10.1016/j.aquaculture.2011.02.037
Othman R., Wang H. P., Elabd H., Xie D. K., Yao H., O’Bryant P., et al. (2022). The effect of density on sex differentiation, sexual dimorphism, stress, and related gene expression in yellow perch. Plos ONE 17, e0267904. doi:10.1371/journal.pone.0267904
Parma L., Pelusio N. F., Gisbert E., Esteban M. A., D’Amico F., Soverini M., et al. (2020). Effects of rearing density on growth, digestive conditions, welfare indicators and gut bacterial community of gilthead sea bream (Sparus aurata, L. 1758) fed different fishmeal and fish oil dietary levels. Aquaculture 518, 734854. doi:10.1016/j.aquaculture.2019.734854
Perelló-Amorós M., García-Pérez I., Sánchez-Moya A., Innamorati A., Vélez E. J., Achaerandio I., et al. (2021). Diet and exercise modulate GH-IGFs axis, proteolytic markers and myogenic regulatory factors in juveniles of gilthead sea bream (Sparus aurata). Animals 11, 2182. doi:10.3390/ani11082182
Perera E., Rosell-Moll E., Martos-Sitcha J. A., Naya-Català F., Simó-Mirabet P., Calduch-Giner J. A., et al. (2021). Physiological trade-offs associated with fasting weight loss, resistance to exercise and behavioral traits in farmed gilthead sea bream (Sparus aurata) selected by growth. Aquac. Rep. 20, 100645. doi:10.1016/j.aqrep.2021.100645
Pérez-Sánchez J., Borrel M., Bermejo-Nogales A., Benedito-Palos L., Saera-Vila A., Calduch-Giner J. A., et al. (2013). Dietary oils mediate cortisol kinetics and the hepatic expression profile of stress responsive genes in juveniles of gilthead sea bream (Sparus aurata) exposed to crowding stress. Comp. Biochem. Physiology 8, 123–130. doi:10.1016/j.cbd.2013.02.001
Pérez-Sánchez J., Simó-Mirabet P., Naya-Català F., Martos-Sitcha J. A., Perera E., Bermejo-Nogales A., et al. (2018). Somatotropic axis regulation unravels the differential effects of nutritional and environmental factors in growth performance of marine farmed fishes. Front. Endocrinol. 9, 687. doi:10.3389/fendo.2018.00687
Person-Le Ruyet J., Le Bayon N. (2009). Effects of temperature, stocking density and farming conditions on fin damage in European sea bass (Dicentrarchus labrax). Aquat. Living Resour. 22, 349–362. doi:10.1051/alr/2009047
Rahman M. T., Haque N., Abu Kasim N. H., De Ley M. (2017). Origin, function, and fate of metallothionein in human blood. Rev. Physiology, Biochem. Pharmacol. 173, 41–62. doi:10.1007/112_2017_1
Ralser M., Wamelink M. M. C., Kowald A., Gerisch B., Heeren G., Struys E. A., et al. (2007). Dynamic rerouting of the carbohydrate flux is key to counteracting oxidative stress. J. Biol. 6, 10. doi:10.1186/jbiol61
Refinetti R., Cornélissen G., Halberg F. (2007). Procedures for numerical analysis of circadian rhythms. Biol. Rhythm Res. 38, 275–325. doi:10.1080/09291010600903692
Remen M., Nederlof M. A. J., Folkedal O., Thorsheim G., Sitjà-Bobadilla A., Pérez-Sánchez J., et al. (2015). Effect of temperature on the metabolism, behavior and oxygen requirements of Sparus aurata. Aquacult. Env. Interac. 7, 115–123. doi:10.3354/aei00141
Remen M., Sievers M., Torgersen T., Oppedal F. (2016). The oxygen threshold for maximal feed intake of Atlantic salmon post-smolts is highly temperature-dependent. Aquaculture 464, 582–592. doi:10.1016/j.aquaculture.2016.07.037
Rey S., Jin X., Damsgård B., Bégout M. L., Mackenzie S. (2021). Analysis across diverse fish species highlights no conserved transcriptome signature for proactive behaviour. BMC Genomics 22, 33. doi:10.1186/s12864-020-07317-z
Rosell-Moll E., Piazzon M. C., Sosa J., Ferrer M. A., Cabruja E., Vega A., et al. (2021). Use of accelerometer technology for individual tracking of activity patterns, metabolic rates and welfare in farmed gilthead sea bream (Sparus aurata) facing a wide range of stressors. Aquaculture 539, 736609. doi:10.1016/j.aquaculture.2021.736609
Rosenfeld J., Van Leeuwen T., Richards J., Allen D. (2015). Relationship between growth and standard metabolic rate: measurement artefacts and implications for habitat use and life-history adaptation in salmonids. J. Animal Ecol. 84, 4–20. doi:10.1111/1365-2656.12260
Sadoul B., Geffroy B. (2019). Measuring cortisol, the major stress hormone in fishes. J. Fish Biol. 94, 540–555. doi:10.1111/jfb.13904
Saera-Vila A., Calduch-Giner J. A., Prunet P., Pérez-Sánchez J. (2009). Dynamics of liver GH/IGF axis and selected stress markers in juvenile gilthead sea bream (Sparus aurata) exposed to acute confinement. Differential stress response of growth hormone receptors. Comp. Biochem. Physiology, Part A 154, 197–203. doi:10.1016/j.cbpa.2009.06.004
Sánchez-Muros M. J., Villacreces S., Miranda-de la Lama G., de Haro C., García-Barroso F. (2013). Effects of chemical and handling exposure on fatty acids, oxidative stress and morphological welfare indicators in gilthead sea bream (Sparus aurata). Fish. Physiol. Biochem. 39, 581–591. doi:10.1007/s10695-012-9721-2
Santos G. A., Schrama J. W., Mamauag R. E. P., Rombout J. H. W. M., Verreth J. A. J. (2010). Chronic stress impairs performance, energy metabolism and welfare indicators in European seabass (Dicentrarchus labrax): the combine effects of fish crowding and water quality deterioration. Aquaculture 299, 73–80. doi:10.1016/j.aquaculture.2009.11.018
Sapolsky R. M., Romero L. M., Munck A. U. (2000). How do glucocorticoids influence stress response? Integrative, permissive, suppressive, stimulatory, and preparative actions. Endocr. Rev. 21, 55–89. doi:10.1210/edrv.21.1.0389
Saraiva J. L., Arechavala-Lopez P., Sneddon L. (2022). “Farming fish,” in Routledge handbook of animal welfare. Editors A. Knight, C. Phillips, and P. Sparks (Abingdon, UK: Routledge), 115–127. doi:10.4324/9781003182351-12
Saravanan S., Geurden I., Figueiredo-Silva A. C., Kaushik S. J., Haidar M. N., Verreth J. A., et al. (2012). Control of voluntary feed intake in fish: a role for dietary oxygen demand in nile tilapia (Oreochromis niloticus) fed diets with different macronutrient profiles. Br. J. Nutr. 108, 1519–1529. doi:10.1017/S0007114511006842
Seibel H., Baßmann B., Rebl A. (2021). Blood will tell: what hematological analyses can reveal about fish welfare. Front. Vet. Sci. 8, 616955. doi:10.3389/fvets.2021.616955
Sibly R. M., Baker J., Grady J. M., Luna L. M., Kodric-Vrown A., Venditti C., et al. (2015). Fundamental insights into ontogenetic growth from theory and fish. Proc. Natl. Acad. Sci. U. S. A. 112, 13934–13939. doi:10.1073/pnas.1518823112
Simó-Mirabet P., Bermejo-Nogales A., Calduch-Giner J. A., Pérez-Sánchez J. (2017). Sirtuin energy-sensing at the molecular level. Tissue-specific gene expression and fasting regulation of sirtuin family in gilthead sea bream (Sparus aurata). J. Comp. Physiology 187, 153–163. doi:10.1007/s00360-016-1014-0
Simó-Mirabet P., Perera E., Calduch-Giner J. A., Afonso J. M., Pérez-Sánchez J. (2018). Co-expression analysis of sirtuins and related metabolic biomarkers in juveniles of gilthead sea bream (Sparus aurata) with differences in growth performance. Front. Physiology 9, 608. doi:10.3389/fphys.2018.00608
Simó-Mirabet P., Perera E., Calduch-Giner J. A., Pérez-Sánchez J. (2020). Local DNA methylation helps to regulate muscle sirtuin 1 gene expression across season and advancing age in gilthead sea bream (Sparus aurata). Front. Zoology 17, 15. doi:10.1186/s12983-020-00361-1
Stincone A., Prigione A., Cramer T., Wamelink M. M. C., Campbell K., Cheung E., et al. (2015). The return of metabolism: biochemistry and physiology of the pentose phosphate pathway. Biol. Rev. 90, 927–963. doi:10.1111/brv.12140
Sveen L. R., Timmerhaus G., Krasnov A., Takle H., Stefansson S. O., Handeland S. O., et al. (2018). High fish density delays wound healing in Atlantic salmon (Salmo salar). Sci. Rep. 8, 16907. doi:10.1038/s41598-018-35002-5
Tanaka K., Jay G., Isselbacher K. J. (1988). Expression of heat-shock and glucose-regulated genes: differential effects of glucose starvation and hypertonicity. Biochim. Biophys. Acta 950, 138–146. doi:10.1016/0167-4781(88)90006-1
Thévenot E. A., Roux A., Xu Y., Ezan E., Junot C. (2015). Analysis of the human adult urinary metabolome variations with age, body mass index, and gender by implementing a comprehensive workflow for univariate and OPLS statistical analyses. J. Proteome Res. 14, 3322–3335. doi:10.1021/acs.jproteome.5b00354
Toni M., Manciocco A., Angiulli E., Alleva E., Cioni C., Malavasi S. (2019). Review: assessing fish welfare in research and aquaculture, with a focus on European directives. Animal 13, 161–170. doi:10.1017/S1751731118000940
van de Nieuwegiessen P. G., Olwo J., Khong S., Verreth J. A., Schrama J. W. (2009). Effects of age and stocking density on the welfare of African catfish, Clarias gariepinus Burchell. Aquaculture 288, 69–75. doi:10.1016/j.aquaculture.2008.11.009
van de Vis H., Kolarevic J., Stien L. H., Kristiansen T. S., Gerritzen M., van de Braak K., et al. (2020). “Welfare of farmed fish in different production systems and operations,” in The welfare of fish. Editors T. S. Kristiansen, A. Fernö, M. A. Pavlidis, and H. van de Vis (Cham, Switzerland: Springer), 323–332. doi:10.1007/978-3-030-41675-1_14
Van der Zee E. A., Havekes R., Barf R. P., Hut R. A., Nijholt I. M., Jacobs E. H., et al. (2008). Circadian time-place learning in mice depends on Cry genes. Curr. Biol. 18, 844–848. doi:10.1016/j.cub.2008.04.077
VanHelder W., Casey K., Radomski M. W. (1987). Regulation of growth hormone during exercise by oxygen demand and availability. Eur. J. Appl. Physiology Occup. Physiology 56, 628–632. doi:10.1007/BF00424801
Vercauteren M., Ampe B., Devriese L., Moons C. P. H., Decostere A., Aerts J., et al. (2022). Explorative study on scale cortisol accumulation in wild caught common dab (Limanda limanda). BMC Veterinary Res. 18, 324. doi:10.1186/s12917-022-03385-3
Weirup L., Schulz C., Seibel H., Aerts J. (2021). Scale cortisol is positively correlated to fin injuries in rainbow trout (Oncorhynchus mykiss) reared in commercial flow through systems. Aquaculture 543, 736924. doi:10.1016/j.aquaculture.2021.736924
Weirup L., Schulz C., Seibel H. (2022). Fish welfare evaluation index (fWEI) based on external morphological damage for rainbow trout (Oncorhynchus mykiss) in flow through systems. Aquaculture 556, 738270. doi:10.1016/j.aquaculture.2022.738270
Wilkinson R. J., Porter M., Woolcott H., Longland R., Carragher J. F. (2006). Effects of aquaculture related stressors and nutritional restriction on circulating growth factors (GH, IGF-I and IGF-II) in Atlantic salmon and rainbow trout. Comp. Biochem. Physiology Part A 145, 214–224. doi:10.1016/j.cbpa.2006.06.010
Keywords: gilthead sea bream, stocking density, oxygen availability, welfare indicators, antioxidant defense, lipid metabolism, energy sensing, growth regulation
Citation: Holhorea PG, Naya-Català F, Belenguer Á, Calduch-Giner JA and Pérez-Sánchez J (2023) Understanding how high stocking densities and concurrent limited oxygen availability drive social cohesion and adaptive features in regulatory growth, antioxidant defense and lipid metabolism in farmed gilthead sea bream (Sparus aurata). Front. Physiol. 14:1272267. doi: 10.3389/fphys.2023.1272267
Received: 03 August 2023; Accepted: 18 September 2023;
Published: 04 October 2023.
Edited by:
Yiming Li, Fishery Machinery and Instrument Research Institute, ChinaCopyright © 2023 Holhorea, Naya-Català, Belenguer, Calduch-Giner and Pérez-Sánchez. This is an open-access article distributed under the terms of the Creative Commons Attribution License (CC BY). The use, distribution or reproduction in other forums is permitted, provided the original author(s) and the copyright owner(s) are credited and that the original publication in this journal is cited, in accordance with accepted academic practice. No use, distribution or reproduction is permitted which does not comply with these terms.
*Correspondence: Jaume Pérez-Sánchez, amFpbWUucGVyZXouc2FuY2hlekBjc2ljLmVz
Disclaimer: All claims expressed in this article are solely those of the authors and do not necessarily represent those of their affiliated organizations, or those of the publisher, the editors and the reviewers. Any product that may be evaluated in this article or claim that may be made by its manufacturer is not guaranteed or endorsed by the publisher.
Research integrity at Frontiers
Learn more about the work of our research integrity team to safeguard the quality of each article we publish.