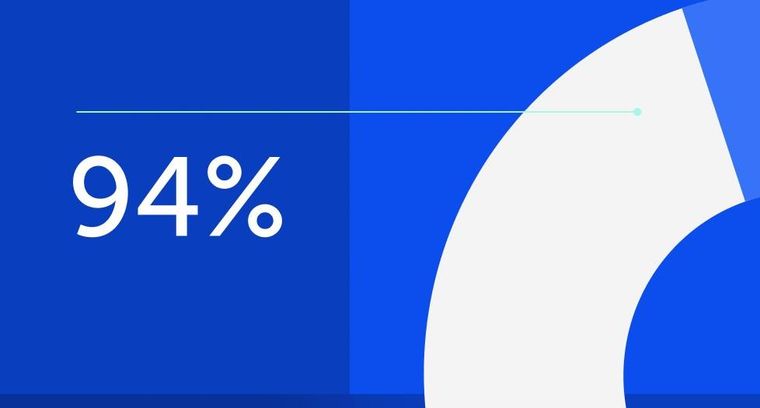
94% of researchers rate our articles as excellent or good
Learn more about the work of our research integrity team to safeguard the quality of each article we publish.
Find out more
EDITORIAL article
Front. Physiol., 06 September 2023
Sec. Cardiac Electrophysiology
Volume 14 - 2023 | https://doi.org/10.3389/fphys.2023.1254596
This article is part of the Research TopicExperimental Models and Model Organisms in Cardiac Electrophysiology: Opportunities and ChallengesView all 10 articles
Editorial on the Research Topic
Experimental models and model organisms in cardiac electrophysiology: opportunities and challenges
Cardiac diseases are the main cause of death worldwide and their incidence and prevalence are likely to increase because of ageing of the population and unhealthy changes in lifestyle. Prevailing theories about the mechanisms of cardiac disease onset, encompass molecular and electrophysiological changes in the cardiac tissue and cells. Insights into the underlying molecular mechanisms are essential to create a deeper understanding of key concepts of cardiac disease development. Moreover, mechanistic insights may drive the development of novel technologies to explore further mechanisms as well as diagnostic and therapeutic tools for cardiac disease management in the clinic.
In this Research Topic “Experimental Models and Model Organisms in Cardiac Electrophysiology: Opportunities and Challenges” various experimental model systems are discussed that enable mechanistic studies on molecular mechanisms and electrophysiological changes that drive cardiac diseases. Experimental model systems include induced pluripotent stem cell (iPSC)-derived cardiomyocytes, as well as cultured neonatal monolayers of cardiomyocytes and fibroblasts. The contribution of Carvalho et al. describes the potential of iPSC-derived cardiomyocytes from healthy donors as a disease model to study electrical properties. By testing the action potential variability of 780 cardiomyocytes from six healthy heart donors, they observed that the action potential data per cell line, per differentiation protocol and per batch fluctuates. This has important consequences for mechanistic studies on arrhythmias. Further standardization of iPSC cardiomyocyte culture is needed for arrhythmia research. In line with Carvalho, the paper of Isamili et al. points out the biological and methodological issues researchers have to consider when working with iPSC-derived cardiomyocytes. Challenges include the variation in ion-channel currents, action potential durations, and atrial phenotype of atrial cardiomyocytes compared to isolated adult human cardiomyocytes. Despite these drawbacks, the authors are hopeful as they expect that the concerted expertise of experimental electrophysiologists and stem cell experts will eventually solve the challenges.
An innovative emerging model system in arrhythmia research is the use of (human) living myocardial tissue slices. Amesz et al. provide an overview of the potential of utilizing living myocardial slices in electrophysiological studies. The authors state that human atrial and ventricular tissue slices of 150–400 µm thickness (6–17 cardiomyocyte layers) can be used for action potential recordings, optical mapping, and extracellular field potential mapping. So far, living myocardial tissue slices hold the promise to facilitate detailed research on cardiac arrhythmias. Optogenetic light-based pacing is an emerging technique to manipulate cardiac activity in a spatial- and temporal-specific manner and improve our understanding of arrhythmogenic mechanisms. Marchal et al. show their results on the application of low-intensity, sub-threshold illumination to selectively manipulate cardiac electrical activity in defined areas of the heart. This approach enables the study of conduction slowing and repolarization heterogeneities during cardiac disease.
In addition to innovative model systems and technologies as described above, novel methodological insight into the interpretation of ECG data may improve the identification of cardiac arrhythmias. Mulla et al. review differences in rate-adaptation of ECG properties in mice and rats compared to humans. Although mice and rats have been used for decades as model systems to study cardiac arrhythmias, conflicting data on QT interval rate-dependence still exists, and therefore the authors state that the empirical ways by which QT intervals are corrected in rodent studies should be revisited. In addition to improved interpretation of ECG data in rodents, optimal programmed electrical stimulation is a prerequisite to assess atrial fibrillation susceptibility. Murphy et al. provide important insight into the optimal pacing protocol to elicit an atrial fibrillation phenotype in mice. Their main message is that for each study, an individualized protocol should be developed. Amorós-Figueras et al. describe a pig model for atrial arrhythmia by scarring of the atrium. Scarring is induced via selective occlusion of the atrial branches, resulting in atrial infarction in the left atrium, and low voltage of bipolar electrograms in affected areas. This model may have potential applicability for studying atrial arrhythmia mechanisms. According to Alameh et al. improved characterization of ion channel activity is required to more accurately study the role of hERG, the pore-forming subunit of the rapid component of the delayed rectifier K+ current, in ventricular repolarization. Mutations in hERG are associated with Long QT syndrome, but most identified variants have unknown or unclear functional consequences and are thus classified as variants of unknown significance. The authors advocate for a unique homogeneous protocol that could ultimately facilitate Long QT syndrome management. Lastly, Ernault et al. study the impact of atrial fibrosis on the development of atrial fibrillation. They describe that the selective disruption of primary cilia in fibroblasts via Ift88 knock-out induces extracellular matrix production, decreases conduction velocity, increases the number of block lines, and increases the risk or reentrant arrhythmias without changing the action potential (AP) characteristics of co-cultured cardiomyocytes with fibroblasts. The data suggest that dysregulation of primary cilia causes fibrosis and hinders myocardial conduction, thereby producing an arrhythmogenic substrate.
In summary, multiple model systems and technical approaches have been developed to study molecular and electrophysiological changes that drive cardiac arrhythmia in recent years. Novel mechanistic findings are important as they will fuel the identification of druggable and diagnostic targets, to ultimately improve clinical arrhythmia management. Hereto, a novel standardized methodology for the design of the models, induction of arrhythmias, and interpretation of the data is of prime importance. This Research Topic provides insights into all items relevant for solid translational research on cardiac arrhythmias.
BB: Writing–original draft, Writing–review and editing. IE: Writing–review and editing. YE: Writing–review and editing. CJ: Writing–review and editing. FC: Writing–review and editing. All authors contributed to the article and approved the submitted version.
BB is funded by the Dutch Heart Foundation (2020-2020B003, DnAFix project), and NWO (NWA.1389.20.157 CIRCULAR). FC is funded by the ANR (ANR-19-CE14-0031-02 & ANR-22-CE17-0051-01).
The authors declare that the research was conducted in the absence of any commercial or financial relationships that could be construed as a potential conflict of interest.
All claims expressed in this article are solely those of the authors and do not necessarily represent those of their affiliated organizations, or those of the publisher, the editors and the reviewers. Any product that may be evaluated in this article, or claim that may be made by its manufacturer, is not guaranteed or endorsed by the publisher.
Keywords: experimental models, cardiac electrophysiology, arrhythmia, model organisms, cardiac electrophysiologic technique
Citation: Brundel BJJM, Jopling C, Efimov IR, Charpentier F and Etzion Y (2023) Editorial: Experimental models and model organisms in cardiac electrophysiology: opportunities and challenges. Front. Physiol. 14:1254596. doi: 10.3389/fphys.2023.1254596
Received: 07 July 2023; Accepted: 24 July 2023;
Published: 06 September 2023.
Edited and reviewed by:
Jordi Heijman, Maastricht University, NetherlandsCopyright © 2023 Brundel, Jopling, Efimov, Charpentier and Etzion. This is an open-access article distributed under the terms of the Creative Commons Attribution License (CC BY). The use, distribution or reproduction in other forums is permitted, provided the original author(s) and the copyright owner(s) are credited and that the original publication in this journal is cited, in accordance with accepted academic practice. No use, distribution or reproduction is permitted which does not comply with these terms.
*Correspondence: Bianca J. J. M. Brundel, Yi5icnVuZGVsQGFtc3RlcmRhbXVtYy5ubA==
Disclaimer: All claims expressed in this article are solely those of the authors and do not necessarily represent those of their affiliated organizations, or those of the publisher, the editors and the reviewers. Any product that may be evaluated in this article or claim that may be made by its manufacturer is not guaranteed or endorsed by the publisher.
Research integrity at Frontiers
Learn more about the work of our research integrity team to safeguard the quality of each article we publish.