- 1College of Plant Protection, Shenyang Agricultural University, Shenyang, China
- 2State Key Laboratory for Biology of Plant Diseases and Insect Pests, Institute of Plant Protection, Chinese Academy of Agricultural Sciences, Beijing, China
Introduction: Trichogramma wasps are egg parasitoids of agricultural lepidopteran pests. The sex of Trichogramma is determined by its ploidy as well as certain sex ratio distorters, such as the endosymbiotic bacteria Wolbachia spp. and the paternal sex ratio (PSR) chromosome. The sex determination systems of hymenopterans, such as Trichogramma spp., involve cascades of the genes transformer (tra), transformer-2 (tra2), and doublesex (dsx) and are associated with sex-specific tra and dsx splicing. First, these genes and their sex-specific variants must be identified to elucidate the interactions between the sex ratio disorders and the sex determination mechanism of Trichogramma.
Methods: Here, we characterized the sex determination genes tra, tra2, and dsx in Trichogramma dendrolimi. Sex-specific tra and dsx variants were detected in cDNA samples obtained from both male and female Trichogramma wasps. They were observed in the early embryos (1–10 h), late embryos (12–20 h), larvae (32 h and 48 h), pre-pupae (96 h), and pupae (144 h, 168 h, 192 h, and 216 h) of both male and female T. dendrolimi offspring.
Results: We detected female-specific tra variants throughout the entire early female offspring stage. The male-specific variant began to express at 9–10 h as the egg was not fertilized. However, we did not find any maternally derived, female-specific tra variant in the early male embryo. This observation suggests that the female-specific tra variant expressed in the female embryo at 1–9 h may not have originated from the maternal female wasp.
Discussion: The present study might be the first to identify the sex determination genes and sex-specific gene splicing in Trichogramma wasps. The findings of this study lay the foundation for investigating the sex determination mechanisms of Trichogramma and other wasps. They also facilitate sex identification in immature T. dendrolimi and the application of this important egg parasitoid in biological insect pest control programs.
Introduction
Trichogramma spp., the egg parasitoids, are efficacious biological control agents against lepidopteran pests in agriculture and forestry (Zhou et al., 2019a; Zang et al., 2020). Female Trichogramma wasps oviposit into the eggs of their insect pest hosts (Zhou et al., 2019b). The sex of the Trichogramma wasp is determined by its ploidy. Female and male offspring develop from fertilized diploid and unfertilized haploid eggs, respectively (Zhou et al., 2003; Heimpel and de Boer, 2008; Zhang et al., 2020). The sex determination systems of Trichogramma are also affected by certain sex ratio distorters, such as the endoparasitic bacteria Wolbachia spp. and the paternal sex ratio (PSR) chromosome (Stouthamer et al., 1999; van Vugt et al., 2003; Zhou et al., 2022a). The endosymbiotic bacteria Wolbachia spp. induce parthenogenesis in Trichogramma wasps by transforming haploid male-destined eggs into diploid embryos during the first mitotic division (Huigens et al., 2000; Zhou et al., 2022b; Zhang et al., 2022). The PSR chromosome causes the generation of male offspring and the loss of the paternal genome during the first mitotic division of the zygote (van Vugt et al., 2003; Dalla Benetta et al., 2020). However, the interactions between the preceding sex ratio disorders and the sex determination systems of Trichogramma have not been studied in depth. Therefore, identification and analysis of the sex determination genes in Trichogramma wasps will help clarify the interactions between these insects and their sex ratio distorters.
Sex determination systems are usually directed by genetic pathways comprising several sex-determining genes (Wilkins, 1995; Verhulst et al., 2010a; Simoni et al., 2020). Sex determination systems consist of a primary genetic signal, sex-specific signal cascades, and the expression of genes regulating sexual development (Peng et al., 2020). The primary sex-specific signals of hymenopterans are governed by paralog genes designated as transformer (tra) or feminizer (fem) (Verhulst et al., 2010a). The rapidly evolving tra encodes arginine/serine (SR)-rich proteins. Order-specific domains have been detected in the tra/fem orthologs such as the hymenopteran (HYM) domain in hymenopterans as well as the domains characteristic of other insect orders. The tra pre-mRNA can be spliced into female-specific and male-specific variants (Verhulst et al., 2010a; Geuverink et al., 2017a). Only a female-specific tra variant produces a functional TRA protein with the Ceritatis-Apis-Musca (CAM) domain and complexes with the protein encoded by tra2 to regulate female-specific doublesex (dsx) splicing (Geuverink et al., 2017b). TRA2 protein is more conserved than TRA. Its RNA-binding domain (RBD) has two regions rich in Ser–Arg (Geuverink et al., 2017b). Dsx interprets the sexual traits of various invertebrates (Chikami et al., 2022). The conserved dsx contains the DNA-binding motif (DM) domain and is localized to the bottom of the tra–tra2–dsx cascade that determines sex in hymenopterans (Verhulst et al., 2010b; Baral et al., 2019).
The haplodiploid sex determination system in hymenopterans is described by the Maternal Effect Genomic Imprinting Sex Determination (MEGISD) model (Beukeboom et al., 2007). In MEGISD, the sex-specific signal involves maternal tra mRNA imprinting and the alternative tra splicing induced by the zygote (Beukeboom and Kamping, 2006; Verhulst et al., 2010a). In Nasonia vitripennis, female-specific tra splicing is initiated by timely expression of the paternal wasp overruler of masculinization (wom) allele upon fertilization (Zou et al., 2020). Sex-specific tra cascade tra2 splicing initiates sexual development by directing dsx. In other hymenopterans, offspring sex is determined according to the MEGISD model and is regulated by the complementary sex determination (CSD) mechanism (Matthey-Doret et al., 2019). In CSD, the heterozygotic csd locus initiates female development, while the homozygotic csd locus promotes diploid male formation (Hagan and Gloag, 2021). CSD proteins direct the sex-specific tra/fem variants, which, in turn, leads to female development (Matthey-Doret et al., 2019; Hagan and Gloag, 2021). Our previous study showed that the CSD mechanism is absent in Trichogramma dendrolimi (Liu et al., 2019). The parasitoid wasps Trichogramma spp. could be the most important egg parasitoid hosts in biological insect pest control programs (Zang et al., 2020; Zhou et al., 2020). To the best of our knowledge, the sex determination genes and their sex-specific splicing have never been previously identified in Trichogramma wasps.
The present study aimed to identify the sex determination genes tra, tra2, and dsx, their sex-specific variants, and their expression patterns at different developmental stages of male and female Trichogramma dendrolimi Matsumura. The results of this work could help elucidate the sex determination mechanisms in Trichogramma wasps. The output of this study also facilitates sex identification in immature T. dendrolimi as it shows how sex-specific tra and dsx variants may be detected.
Materials and methods
Insects
The isofemale T. dendrolimi line was established using a single mating pair of wasps. The insects were reared over several generations on the eggs of the rice moth Corcyra cephalonica (Lepidoptera: Pyralidae) at 25°C and 70% relative humidity (RH) under a photoperiod of L16:D8. The host eggs were grouped in lots of ∼500, glued onto a white card, and allowed to parasitize the host eggs. The latter were irradiated with ultraviolet (UV) light (TUV 30W UV lamps; Philips, Amsterdam, The Netherlands) for 45 min. These host egg cards were used for parasitization by T. dendrolimi.
Sample collection
A group of 100 adult male or female T. dendrolimi wasps was collected. Male T. dendrolimi offspring were obtained from host eggs parasitized by virginal female wasps. Mixtures of male and female offspring were obtained from host eggs parasitized by mated female wasps. Immature T. dendrolimi cannot be distinguished by sex. They include early embryos (at 1 h, 2 h, 3 h, 4 h, 5 h, 6 h, 7 h, 8 h, 9 h, and 10 h), late embryos (at 12 h, 14 h, 16 h, 18 h, and 20 h), larvae (at 32 h and 48 h), pre-pupae (at 96 h), and pupae (at 144 h, 168 h, 192 h, and 216 h). All the foregoing stages were collected in lots of ∼1,000.
RNA extraction and cDNA synthesis
The total RNA of each sample was extracted. Samples included embryos, larvae, pupae, and adults. Total RNA of the T. dendrolimi wasp was extracted with TRIzol reagent (Invitrogen, Carlsbad, CA, United States). Total RNA (1 µg) was reverse-transcribed using a PrimeScript RT Kit (TaKaRa, Dalian, China) according to the manufacturer’s protocol. The cDNA product was then immediately stored at −80°C until subsequent use.
Identification and cloning of tra, tra2, and dsx orthologs
tra, tra2, and dsx orthologs were identified using the TBLASTN algorithm (https://blast.ncbi.nlm.nih.gov/Blast.cgi?PROGRAM=tblastn&PAGE_TYPE=BlastSearch&LINK_LOC=blasthome) by aligning the T. dendrolimi genomic assembly dataset (unpublished data). The study applied the available sequence of TRA/FEM proteins from N. vitripennis (NP_001128299), Apis mellifera (AAS86667.1), and Drosophila melanogaster (NP_524114.1) as the query sequences for the aligning of TRA orthologs. The sequences of TRA2 proteins from N. vitripennis (ATD84850.1), Aphidius gifuensis (XP_044008082.1), and Leptopilina clavipes (AXJ14345.1) were used as the query sequences for identifying TRA2 orthologs. The sequences of DSX proteins from N. vitripennis (XP_008205423.1), A. mellifera (NP_001104725.1), and D. melanogaster (NP_001262353.1) were used as the query sequences for identifying DSX2 orthologs (Figure 1). The start–stop codons and intron–exon boundary of tra, tra2, and dsx were determined using the genome assembly database and the transcriptome constructed by the present group. Similarity analyses were conducted on these genes using Clustal X v. 1.83 (http://www.clustal.org/download/current), Jalview v. 2.10.3 (https://www.jalview.org/download/), and DNAMAN v. 6.0 (https://www.lynnon.com/downloads.html). Primer Premier v. 5.0 (http://www.premierbiosoft.com) was used to design the primers used to amplify the tra, tra2, and dsx transcripts and their sex-specific variants (Table 1). The polymerase chain reaction (PCR) was conducted using 1.0 μL cDNA template and PCR Master Mixture (Promega, Madison, WI, United States) or high-fidelity DNA polymerase (Yeasen, Shanghai, China). The PCR products were confirmed by 1.5% agarose gel electrophoresis, cloned, and sequenced.
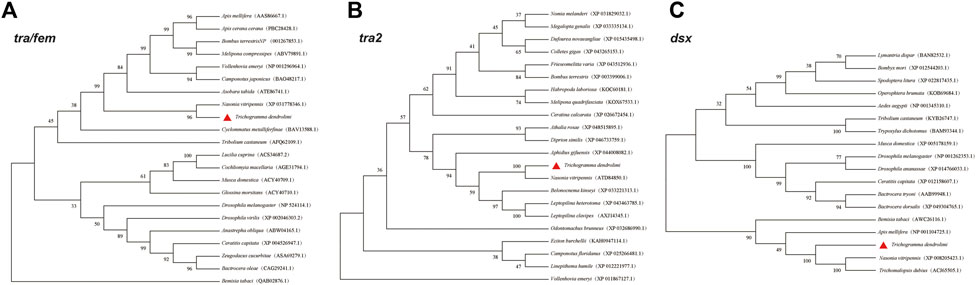
FIGURE 1. Phylogenetic analysis of amino acid sequences of tra (A), tra2 (B), and dsx (C) in different insect species.
Splicing of sex-specific tra and dsx
Alternative tra and dsx variants were amplified using the specific primers listed in Table 1. The PCR products of the alternative splices were confirmed, cloned, and sequenced as previously described. Sex-specific tra and dsx exons were characterized using the fragments amplified from the cDNA samples of male and female T. dendrolimi. The full-length sex-specific transcripts were then merged with the predicted tra or dsx models for T. dendrolimi using the sequenced sex-specific fragments.
Phylogenetic and molecular evolutionary analyses
Sequences of the homologs in ten insect species from the National Center for Biotechnology Information (NCBI) database (https://www.ncbi.nlm.nih.gov/) were collected to delineate the evolution of tra, tra2, and dsx. A phylogenetic tree was generated using PhyloSuite v. 1.2.2 (Zhang et al., 2020) with the IQ-TREE fitted by the JTT model (Nguyen et al., 2015). Orthologous relationships were identified using 1,000 bootstrap replicates. Conserved domains were identified using the Conserved Domain Database of NCBI.
Sex-specific splicing expression patterns in T. dendrolimi
The primers used for sex-specific tra and dsx splicing were selected based on the different sequences of female- and male-specific variants and designed using Primer Premier v. 5.0. Thus, the specific regions of female- and male-specific variants can be amplified to different sizes by the single pair of primers. Sex-specific tra and dsx splicing was validated based on the real-time (RT)-PCR products amplified using the male and female cDNA samples. The dynamic expression of sex-specific tra and dsx splicing was detected in the male and mixed male–female offspring samples. The RT-PCR products of the sex-specific variants were then detected by 1.5% agarose gel electrophoresis.
Results
Identification of tra, tra2, and dsx
The full-length tra sequence was 1,592 bp long and included an open reading frame (ORF) of 1,128 bp encoding 375 amino acids (aa) (Table 2). The tra aa sequence had the same structure as those of other known insect tra. The HYM domain was detected in all tra/fem orthologs in hymenopterans. All orthologs had a CAM, a proline (Pro)-rich, and an Arg/Ser domain. The phylogenetic tree showed that the aa sequence of T. dendrolimi tra was closely related to that of N. vitripennis tra (Figure 1; Figure 2; Supplementary Figure S1). Alignment of the aa sequences in T. dendrolimi and N. vitripennis tra revealed 41.12% identity.

TABLE 2. Length of the full sequence, ORF, and protein of tra, tra2, and dsx genes in T. dendrolimi and N. vitripennis.
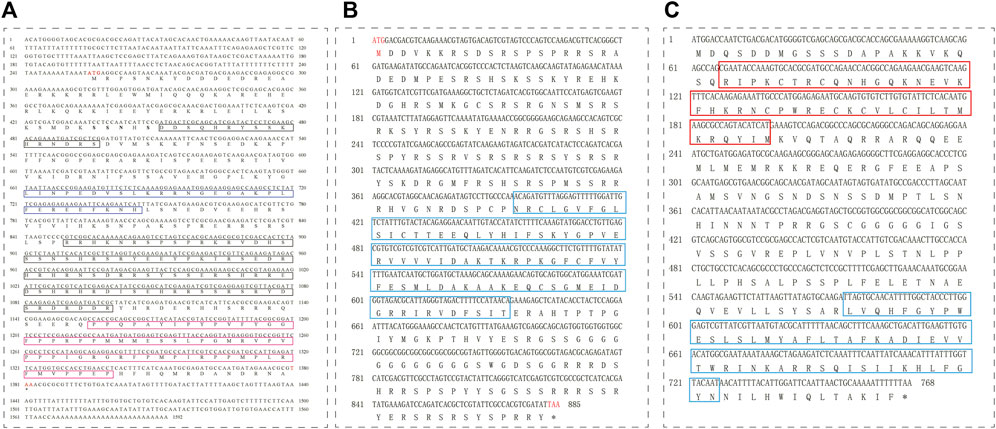
FIGURE 2. cDNA and amino acid sequences and domains of tra (A), tra2 (B), and dsx (C) in T. dendrolimi. (A) The blue box indicates the putative autoregulation domain; the black box indicates the Arg/Ser domain; and the fuchsia box indicates the proline (Pro)-rich region. (B) The cyan box indicates the RNA recognition motif (RRM) domain. (C) The red box indicates the DNA-binding motif (DM) domain; the cyan box indicates the dimer domain.
The full-length sequence of tra2 was 2,932 bp long and included an ORF of 885 bp encoding 294 aa (Table 2). The RBD was detected in the tra2 orthologs in hymenopterans. The aa sequence of T. dendrolimi tra2 was similar to that of N. vitripennis tra2 (Figure 2). Both the T. dendrolimi and N. vitripennis orthologs had a glycine (Gly)-rich domain (Figure 1; Supplementary Figure S2). Alignment of the aa sequences in T. dendrolimi and N. vitripennis tra2 disclosed 76.92% identity.
The dsx ortholog was 3,719 bp long and included an ORF of 768 bp encoding 255 aa (Table 2). The aa sequences of the T. dendrolimi and other insect dsx orthologs all had the DM and dimer domains (Figure 1; Supplementary Figure S3). The aa sequence of T. dendrolimi dsx was similar to that of N. vitripennis dsx (Figure 2). Alignment of the aa sequences in T. dendrolimi and N. vitripennis dsx revealed 76.63% identity.
Sex-specific variants of tra and dsx
Expression of the sex-specific tra and dsx variants was determined using the cDNA samples obtained from male and female wasps (Figure 3). Both the female-specific variant (ID: OQ847082) and male-specific variant (ID: OQ847083) of tra were registered on the NCBI database. The female-specific tra variant was composed of nine exons. The male-specific tra variant encoded a truncated protein consisting of 172 aa and an Arg/Ser domain. The Pro-rich and CAM domains were absent in the male-specific tra variant. Hence, these domains are only implicated in female-specific signals.
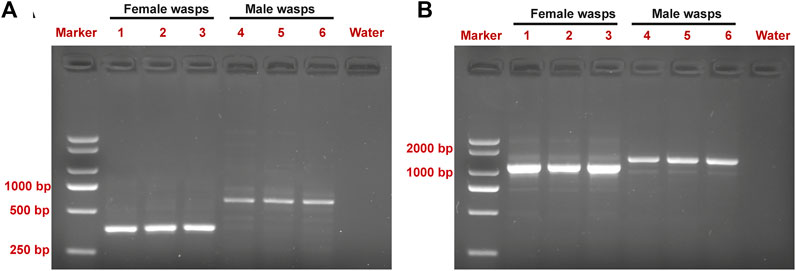
FIGURE 3. Detection of sex-specific tra (A) and dsx (B) splices from pooled cDNA samples of male and female wasps. Lane M is the DL2000 DNA marker. Lanes 1–3 in (A) are female-specific tra splices. Lanes 4–6 in (A) are male-specific tra splices. Lanes 1–3 in (B) are female-specific dsx splices. Lanes 4–6 in (B) are male-specific dsx splices.
Both the female-specific variant (ID: OQ847080) and male-specific variant (ID: OQ847081) of dsx were registered on the NCBI database. Dsx included five exons. Female-specific exclusion was observed at the fifth exon, while male-specific exclusions occurred at the fifth exon and the fourth intron (Figure 4).
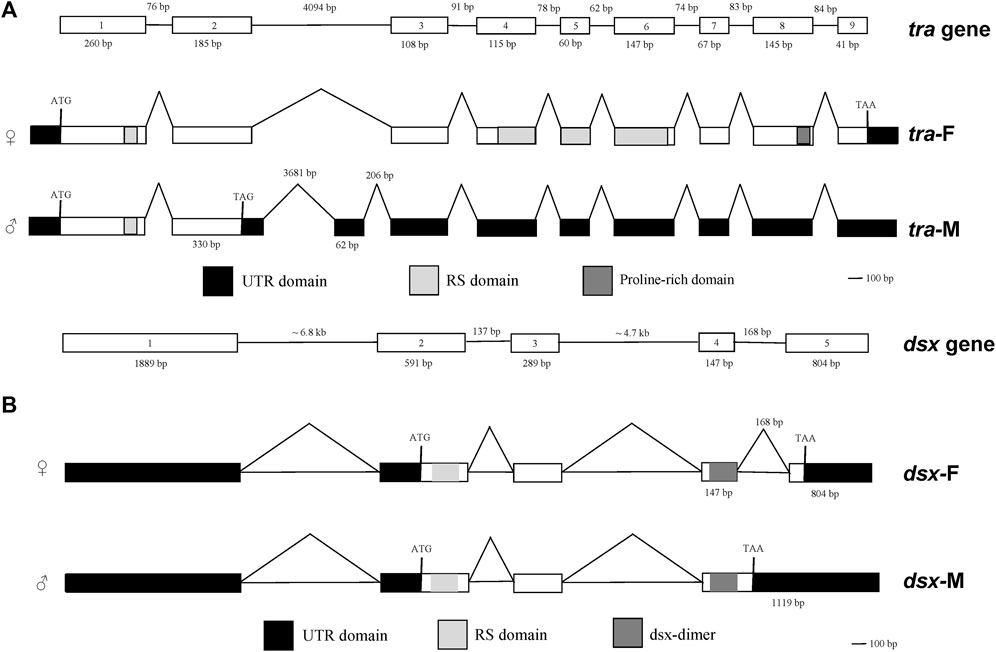
FIGURE 4. Genomic structures of sex-specific tra (A) and dsx splices (B). Boxes represent exons. White boxes represent protein-coding exons. Black boxes represent non-translated exons. Light gray boxes indicate the arginine/serine (RS)-rich domain. Dark gray boxes in (A) indicate the proline (Pro)-rich domain. Dark gray boxes in (B) indicate the dsx dimer.
We detected sex-specific tra and dsx variants in pooled male and mixed male–female progeny samples. Male-specific tra variant was observed in the male offspring after 9 h. Female-specific tra variant was found in the mixed male–female progeny samples at all developmental stages (Figure 5). Both female-specific and male-specific dsx variants were detected in the offspring after 9 h (Figure 6).
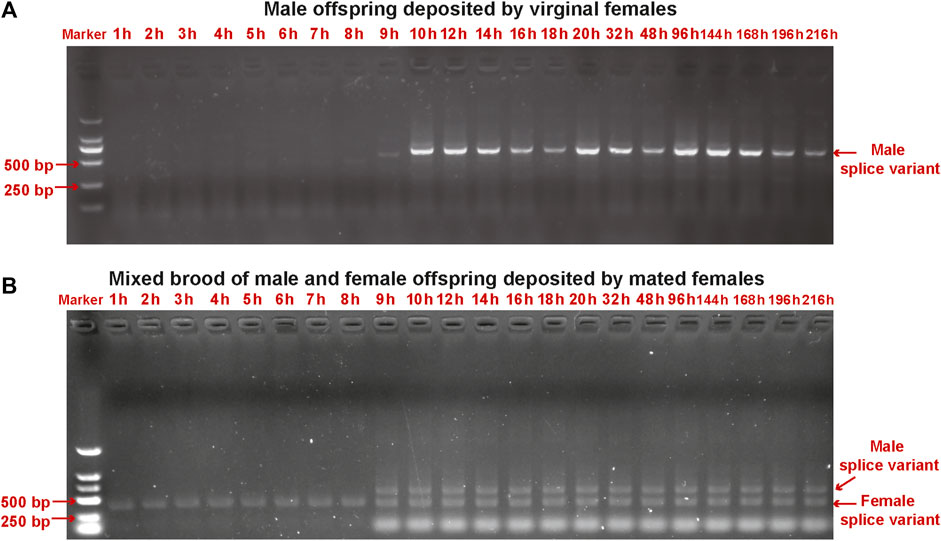
FIGURE 5. Detection of sex-specific tra variants in pooled samples of male offspring deposited by unmated female wasps (A) and mixed male and female offspring deposited by mated female wasps (B) collected from early embryos (at 1 h, 2 h, 3 h, 4 h, 5 h, 6 h, 7 h, 8 h, 9 h, and 10 h), late embryos (at 12 h, 14 h, 16 h, 18 h, and 20 h), larvae (at 32 h and 48 h), pre-pupae (at 96 h), and pupae (at 144 h, 168 h, 192 h, and 216 h) of T. dendrolimi. Lane M is the DL2000 DNA marker.
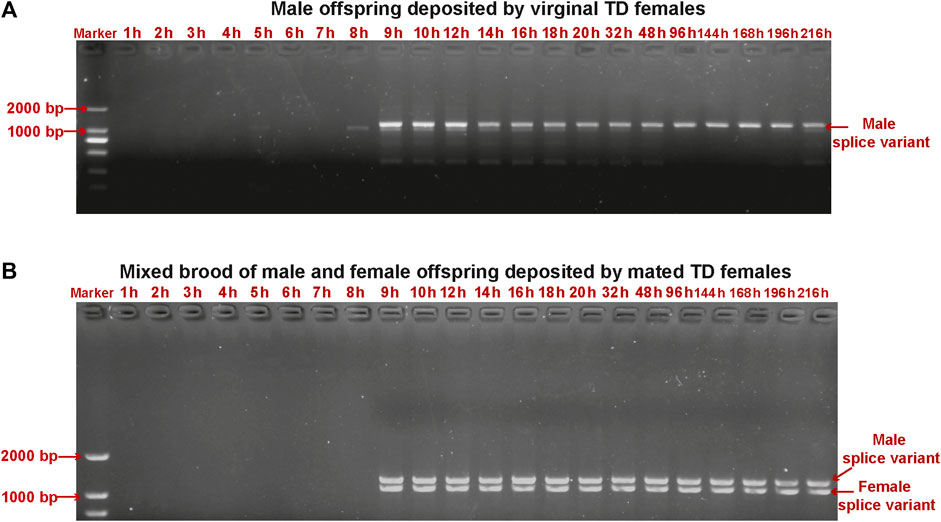
FIGURE 6. Detection of sex-specific dsx variants in pooled samples of male offspring deposited by unmated female wasps (A) and mixed male and female offspring deposited by mated female wasps (B) collected from early embryos (at 1 h, 2 h, 3 h, 4 h, 5 h, 6 h, 7 h, 8 h, 9 h, and 10 h), late embryos (at 12 h, 14 h, 16 h, 18 h, and 20 h), larvae (at 32 h and 48 h), pre-pupae (at 96 h), and pupae (at 144 h, 168 h, 192 h, and 216 h) of T. dendrolimi. Lane M is the DL2000 DNA marker.
Discussion
The present study identified the genes tra, tra2, and dsx which determine the sex of the natural enemy wasp T. dendrolimi. Sex-specific tra and dsx variants were detected in the male and female wasps. To the best of our knowledge, the present study may be the first to conduct a systematic analysis of the genes that determine sex in Trichogramma wasps.
In hymenopterans, the tra/fem orthologs have widely diverged, whereas those of tra2 and dsx are relatively more conserved. These factors might partially explain the observed variation among different insect species in terms of their sex determination systems (Beye et al., 2003). For example, the sex determination of CSD mechanisms has been found in at least 60 species of Hymenoptera (van Wilgenburg et al., 2006). Sex-specific tra/fem splicing is governed by CSD proteins in hymenopterans (Hasselmann et al., 2008). In contrast, the CSD mechanism is virtually absent in parasitoid wasps such as Nasonia spp. In the members of this genus, the instructor wom directs tra splicing and is activated only in the early embryo after fertilization (Zou et al., 2020). Divergence of the tra/fem orthologs may account for the observed differences in the instructor signals among various MEGISD and CSD mechanisms.
Similar to the previously reported Nasonia wasps (Zou et al., 2020), the Trichogramma wasps presented with a female-specific tra variant in the early female embryo (1–20 h). The male-specific variant began to express its tra variant at 9–10 h as fertilization was somewhat delayed. Unlike the Nasonia wasps (Zou et al., 2020), the Trichogramma wasps presented no maternally provided female-specific tra variant at the early male embryo stage. Thus, the female-specific tra variant expressed in the female embryo at 1–9 h may not have originated from the maternal female wasp. In the Asobara tabida wasp, no female-specific variant was detected in the early fertilized or unfertilized eggs (Geuverink et al., 2017a). A non-sex-specific tra variant was detected in early A. tabida embryos, and it contained a putative duplicate CAM domain. However, the role of this non-sex-specific tra variant in A. tabida remains to be investigated. According to the MEGISD model, sex determination is initiated by a paternal instructor wom. In Nasonia, wom may only be expressed in the early embryo within several hours (Zou et al., 2020). In Trichogramma, the male-specific tra and dsx variants were detected and began to express, respectively, in the male embryo after 9 h. Therefore, the sex-determination mechanisms may be similar for both Nasonia and Trichogramma wasps and lack the effects of the maternally provided female-specific tra variant observed in A. tabida wasps. Nevertheless, it remains to be determined whether early sex-specific tra splicing is initially governed by a paternal factor such as wom.
To the best of our knowledge, this study may be the first case for analyzing alternative splicing of the sex determination genes tra, tra2, and dsx in Trichogramma wasps. The dynamics of sex-specific tra and dsx variant expression indicated that these sex-specific splicing events may be initially governed by an early paternal instructor such as wom found in Nasonia wasps. However, primers for the sex-specific tra and dsx variants could efficiently determine the sex of immature Trichogramma. The results of this work provide an important reference for investigating the sex determination mechanisms of Trichogramma wasps and help control the sex ratio of these natural enemy insects as they are being reared on a large scale for use in biological insect pest control programs.
Data availability statement
The original contributions presented in the study are included in the article/Supplementary Material; further inquiries can be directed to the corresponding authors.
Author contributions
All authors listed have made a substantial, direct, and intellectual contribution to the work and approved it for publication.
Conflict of interest
The authors declare that the research was conducted in the absence of any commercial or financial relationships that could be construed as a potential conflict of interest.
Publisher’s note
All claims expressed in this article are solely those of the authors and do not necessarily represent those of their affiliated organizations, or those of the publisher, the editors, and the reviewers. Any product that may be evaluated in this article, or claim that may be made by its manufacturer, is not guaranteed or endorsed by the publisher.
Supplementary material
The Supplementary Material for this article can be found online at: https://www.frontiersin.org/articles/10.3389/fphys.2023.1243753/full#supplementary-material
References
Baral, S., Arumugam, G., Deshmukh, R., and Kunte, K. (2019). Genetic architecture and sex-specific selection govern modular, male-biased evolution of doublesex. Sci. Adv. 5, eaau3753. doi:10.1126/sciadv.aau3753
Beukeboom, L. W., Kamping, A., and van de Zande, L. (2007). Sex determination in the haplodiploid wasp Nasonia vitripennis (Hymenoptera: chalcidoidea): A critical consideration of models and evidence. Semin. Cell. Dev. Biol. 18, 371–378. doi:10.1016/j.semcdb.2006.12.015
Beye, M., Hasselmann, M., Fondrk, M. K., Page, R. E., and Omholt, S. W. (2003). The gene csd is the primary signal for sexual development in the honeybee and encodes an SR-type protein. Cell. 114, 419–429. doi:10.1016/s0092-8674(03)00606-8
Chikami, Y., Okuno, M., Toyoda, A., Itoh, T., and Niimi, T. (2022). Evolutionary history of sexual differentiation mechanism in insects. Mol. Biol. Evol. 39, msac145. doi:10.1093/molbev/msac145
Dalla Benetta, E., Antoshechkin, I., Yang, T., Nguyen, H. Q. M., Ferree, P. M., and Akbari, O. S. (2020). Genome elimination mediated by gene expression from a selfish chromosome. Sci. Adv. 6, eaaz9808. doi:10.1126/sciadv.aaz9808
Geuverink, E., Rensink, A. H., Rondeel, I., Beukeboom, L. W., van de Zande, L., and Verhulst, E. C. (2017b). Maternal provision of transformer-2 is required for female development and embryo viability in the wasp Nasonia vitripennis. Insect biochem. Mol. Biol. 90, 23–33. doi:10.1016/j.ibmb.2017.09.007
Geuverink, E., Verhulst, E. C., van Leussen, M., van de Zande, L., and Beukeboom, L. W. (2017a). Maternal provision of non-sex-specific transformer messenger RNA in sex determination of the wasp Asobara tabida. Insect Mol. Biol. 27, 99–109. doi:10.1111/imb.12352
Hagan, T., and Gloag, R. (2021). Founder effects on sex determination systems in invasive social insects. Curr. Opin. Insect. Sci. 46, 31–38. doi:10.1016/j.cois.2021.02.009
Hasselmann, M., Gempe, T., Schiøtt, M., Nunes-Silva, C. G., Otte, M., and Beye, M. (2008). Evidence for the evolutionary nascence of a novel sex determination pathway in honeybees. Nature 454, 519–522. doi:10.1038/nature07052
Heimpel, G. E., and de Boer, J. G. (2008). Sex determination in the Hymenoptera. Annu. Rev. Entomol. 53, 209–230. doi:10.1146/annurev.ento.53.103106.093441
Huigens, M. E., Luck, R. F., Klaassen, R. H., Maas, M. F., Timmermans, M. J., and Stouthamer, R. (2000). Infectious parthenogenesis. Nature 405, 178–179. doi:10.1038/35012066
Liu, Q. Q., Zhou, J. C., Zhang, C., Dong, Q. j., Ning, S. f., and Hui, D. (2019). Absence of complementary sex determination in Trichogramma dendrolimi matsumura (Hymenoptera: trichogrammatidae). PeerJ. doi:10.7287/peerj.preprints.27871v1
Matthey-Doret, C., van der Kooi, C. J., Jeffries, D. L., Bast, J., Dennis, A. B., Vorburger, C., et al. (2019). Mapping of multiple complementary sex determination loci in a parasitoid wasp. Genome Biol. Evol. 11, 2954–2962. doi:10.1093/gbe/evz219
Nguyen, L. T., Schmidt, H. A., von Haeseler, A., and Minh, B. Q. (2015). IQ-TREE: A fast and effective stochastic algorithm for estimating maximum-likelihood phylogenies. Mol. Biol. Evol. 32, 268–274. doi:10.1093/molbev/msu300
Peng, W., Yu, S., Handler, A. M., Tu, Z., Saccone, G., Xi, Z., et al. (2020). miRNA-1-3p is an early embryonic male sex-determining factor in the Oriental fruit fly Bactrocera dorsalis. Nat. Commun. 11, 932. doi:10.1038/s41467-020-14622-4
Simoni, A., Hammond, A. M., Beaghton, A. K., Galizi, R., Taxiarchi, C., Kyrou, K., et al. (2020). A male-biased sex-distorter gene drive for the human malaria vector Anopheles gambiae. Nat. Biotechnol. 38, 1054–1060. doi:10.1038/s41587-020-0508-1
Stouthamer, R., Breeuwer, J. A., and Hurst, G. D. (1999). Wolbachia pipientis: microbial manipulator of arthropod reproduction. Annu. Rev. Microbiol. 53, 71–102. doi:10.1146/annurev.micro.53.1.71
van Vugt, J. F., Salverda, M., de Jong, J. H., and Stouthamer, R. (2003). The paternal sex ratio chromosome in the parasitic wasp Trichogramma kaykai condenses the paternal chromosomes into a dense chromatin mass. Genome 46, 580–587. doi:10.1139/g03-044
van Wilgenburg, E., Driessen, G., and Beukeboom, L. W. (2006). Single locus complementary sex determination in Hymenoptera: an ‘unintelligent’ design? Front. Zool. 3, 1. doi:10.1186/1742-9994-3-1
Verhulst, E. C., Beukeboom, L. W., and van de Zande, L. (2010b). Maternal control of haplodiploid sex determination in the wasp Nasonia. Science 328, 620–623. doi:10.1126/science.1185805
Verhulst, E. C., van de Zande, L., and Beukeboom, L. W. (2010a). Insect sex determination: it all evolves around transformer. Curr. Opin. Genet. Dev. 20, 376–383. doi:10.1016/j.gde.2010.05.001
Wilkins, A. S. (1995). Moving up the hierarchy: A hypothesis on the evolution of a genetic sex determination pathway. Bioessays 17, 71–77. doi:10.1002/bies.950170113
Zang, L. S., Wang, S., Zhang, F., and Desneux, N. (2020). Biological control with Trichogramma in China: history, present status and perspectives. Annu. Rev. Entomol. 66, 463–484. doi:10.1146/annurev-ento-060120-091620
Zhang, C., Liu, Q. Q., Huo, Y. J., Zhao, X., Shang, D., Yang, Y., et al. (2022). Decreased Wolbachia titers cause gradual change in masculinization of intersex individuals of thelytokous Trichogramma dendrolimi. Entomol. Gen. 42, 751–759. doi:10.1127/entomologia/2022/1464
Zhang, D., Gao, F., Jakovlić, I., Zou, H., Zhang, J., Li, X. W., et al. (2020a). PhyloSuite: an integrated and scalable desktop platform for streamlined molecular sequence data management and evolutionary phylogenetics studies. Mol. Ecol. Resour. 20, 348–355. doi:10.1111/1755-0998.13096
Zhang, X., Wang, G., Zhang, S., Chen, S., Wang, Y., Wen, P., et al. (2020b). Genomes of the banyan tree and pollinator wasp provide insights into fig-wasp coevolution. Cell. 183, 875–889. doi:10.1016/j.cell.2020.09.043
Zhou, J. C., Dong, Q. J., Zhang, T. S., Duan, L. J., Ning, S. F., Liu, Q. Q., et al. (2019b). Effect of wind time on the dispersal capacity of Trichogramma dendrolimi Matsumura (Hymenoptera Trichogrammatidae). J. Asia Pac. Entomol. 22, 742–749. doi:10.1016/j.aspen.2019.06.001
Zhou, J. C., Li, Y. Y., Liu, Q. Q., Ning, S. F., Che, W. N., Cong, B., et al. (2019a). Effects of temperature and superparasitism on quality and characteristics of thelytokous Wolbachia-infected Trichogramma dendrolimi Matsumura (Hymenoptera: trichogrammatidae) during mass rearing. Sci. Rep. 9, 18114–18212. doi:10.1038/s41598-019-54719-5
Zhou, J. C., Liu, Q. Q., Wang, Q. R., Ning, S. F., Che, W. N., and Dong, H. (2020). Optimal clutch size for quality control of bisexual and Wolbachia-infected thelytokous lines of Trichogramma dendrolimi Matsumura (Hymenoptera: trichogrammatidae) mass reared on eggs of a substitutive host, Antheraea pernyi Guérin-Méneville (Lepidoptera: saturniidae). Pest Manag. Sci. 76, 2635–2644. doi:10.1002/ps.5805
Zhou, J. C., Shang, D., Liu, S. M., Zhang, C., Huo, L. X., Zhang, L. S., et al. (2023). Wolbachia-infected Trichogramma dendrolimi is outcompeted by its uninfected counterpart in superparasitism but does not have developmental delay. Pest Manag. Sci. 79, 1005–1017. doi:10.1002/ps.7269
Zhou, J. C., Shang, D., Qian, Q., Zhang, C., Zhang, L. S., and Dong, H. (2022b). Penetrance during Wolbachia-mediated parthenogenesis of Trichogramma wasps is reduced by continuous oviposition, associated with exhaustion of Wolbachia titers in ovary and offspring eggs. Pest Manag. Sci. 78, 3080–3089. doi:10.1002/ps.6934
Zhou, J. C., Zhao, X., Huo, L. X., Shang, D., Dong, H., and Zhang, L. S. (2022a). Wolbachia-driven memory loss in a parasitic wasp increases superparasitism to enhance horizontal transmission. mBio 13, e0236222. doi:10.1128/mbio.02362-22
Keywords: alternative splicing, dsx, parasitoid, sex-determining gene, tra, tra2, Trichogramma
Citation: Ning S-F, Huo L-X, Lv L, Wang Y, Zhang L-S, Che W-N, Dong H and Zhou J-C (2023) The identification and expression pattern of the sex determination genes and their sex-specific variants in the egg parasitoid Trichogramma dendrolimi Matsumura (Hymenoptera: Trichogrammatidae). Front. Physiol. 14:1243753. doi: 10.3389/fphys.2023.1243753
Received: 21 June 2023; Accepted: 10 August 2023;
Published: 25 August 2023.
Edited by:
Lian-Sheng Zang, Guizhou University, ChinaReviewed by:
Yuan-Xi Li, Nanjing Agricultural University, ChinaXiaofang He, South China Agricultural University, China
Copyright © 2023 Ning, Huo, Lv, Wang, Zhang, Che, Dong and Zhou. This is an open-access article distributed under the terms of the Creative Commons Attribution License (CC BY). The use, distribution or reproduction in other forums is permitted, provided the original author(s) and the copyright owner(s) are credited and that the original publication in this journal is cited, in accordance with accepted academic practice. No use, distribution or reproduction is permitted which does not comply with these terms.
*Correspondence: Wu-Nan Che, chewunan@syau.edu.cn; Hui Dong, biocontrol@163.com; Jin-Cheng Zhou, parasitoidswasp@163.com
†These authors have contributed equally to this work and share first authorship