- 1Department of Physical Education, Ningbo University of Technology, Ningbo, China
- 2Ningde Vocational and Technical College, Ningde, China
Objective: This study aims to systematically evaluate the effects of blood flow restriction (BFR) training on lower limb muscle activation and post-activation potentiation (PAP) in athletes through a meta-analysis and discuss methods to improve instant muscle strength so as to provide a reference for training in this field.
Methods: Randomized controlled trials (RCTs) that examined the impact of BFR training on muscle activation and PAP were gathered through database searches, such as CNKI, Wanfang, Web of Science, PubMed, and others. The Cochrane risk of bias tool was used to include and exclude literature. Quality evaluation and statistical analysis were conducted using ReviewManager 5.3 software, STATA 16.0, and other software programs. The sensitivity analysis and funnel plots were employed to assess result stability and publication bias.
Results: In total, 18 literature studies were included with a total of 267 subjects. The meta-analysis showed that BFR could significantly improve the RMS value of lower limb muscles [SMD = 0.98, 95% CI (0.71, 1.24), and p < 0.00001]. BFR had a significant effect on the immediate explosive power of the lower limbs [SMD = 0.28, 95% CI (0.02, 0.53), and p = 0.03], but the heterogeneity was obvious (I2 = 51%). The subgroup analysis showed that different training methods may be influencing factors that lead to the heterogeneity between studies. The measurement indexes were the counter movement jump (CMJ) [SMD = 0.45, 95% CI (0.20, 0.69), and p = 0.0004], training mode to overcome body weight [SMD = 0.57, 95% CI (0.33, 0.82), and p < 0.00001], and compressive strength of 40%–60% arterial occlusion pressure (AOP) [SMD = 0.57, 95% CI (0.31, 0.83), and p < 0.0001], which reached the maximum effect and was statistically significant.
Conclusion: BFR training can induce lower extremity muscle activation and PAP. Combining self-weight training with BFR exercises set at 40%–60% AOP appears to be particularly effective in inducing PAP, especially for enhancing CMJ. Furthermore, combining body-weight training with BFR is considered an effective warm-up method to improve CMJ.
Systematic Review Registration: http://inplasy.com, identifier INPLASY2023100087
1 Introduction
With increasing competitiveness in sports, traditional training methods often fall short of meeting athletes’ demands for enhancing their competitive abilities. Developing explosive muscle power is a common objective for athletes participating in disciplines such as jumping, throwing, or sprinting. During their training, athletes often incorporate specialized exercises tailored to the unique characteristics of their sport, which may include augmentation or resistance exercises. Researchers have discovered that blood flow restriction (BFR) training can serve as a supplementary approach to high-intensity resistance training, effectively improving muscle contraction function and promoting muscle strength growth (Xu and Wang, 2013). It achieves this by applying pressure to the outer regions of the limbs, which causes occlusion of venous blood flow at the distal ends of the limbs. Subsequently, training is conducted at lower exercise intensities, thereby facilitating optimal muscle activation during the warm-up (Che et al., 2021). Studies have indicated that the combination of BFR training with low-intensity resistance training can improve the recruitment capacity of the lower limb muscles (Wang, 2021).
As a method for rapidly enhancing strength, post-activation potentiation (PAP) is achieved through controlled training, such as squats and deadlifts, which induce intense neuromuscular excitement and, consequently, rapidly improving muscle explosiveness within a short timeframe (Batista Mauro et al., 2011). High-intensity exercise is achieved through the rapid recruitment of muscle fibers by the nervous system. Consequently, enhancing muscle nerve activation before a competition holds significant importance for improving sports performance (Lin et al., 2017; Xu et al., 2020). Nevertheless, there is controversy surrounding the induction mode and timing of PAP. Most coaches tend to use maximum resistance exercise to induce PAP (Hanson et al., 2007; Mccann and Flanagan, 2010). However, this high-intensity and high-load training approach can lead to sports injuries and muscle fatigue. By contrast, BFR training is a low-energy consumption, high-efficiency exercise mode. By restricting blood flow to the limbs, metabolites accumulate, prompting the body to falsely simulate stress in muscle fibers by intensive exercising, thereby optimizing training (Hu, 2020).
Warm-up exercises have been extensively researched, and scientific warm-up routines can effectively enhance athletic performance. Studies have shown that the PAP effect can optimize warm-up programs for endurance sports, resulting in improved athletic achievements (Wu et al., 2020). However, the potential of utilizing BFR to enhance warm-up effects remains largely unexplored. In light of this, this study conducts a meta-analysis that summarizes the impact of BFR training combined with different exercise modalities on lower limb muscle activation and PAP, finding the optimal pressure intensity to induce an enhancement effect of lower limb activation and determining the appropriate exercise mode that can be combined with BFR training during warm-up. Our focus lies on the lower limb muscles below the iliac crest (Zhao et al., 2006). The selected experiments are all randomized controlled trials that aim to provide a reference for enhancing athletes’ muscle recruitment capacity and explosiveness.
2 Materials and methods
2.1 Search strategy
A total of 159 documents were retrieved from the CNKI, Wanfang, VIP, PubMed, and Web of Science databases up to 4 February 2023. The English search terms used were as follows: (“blood flow restriction training” or “BFR” or “KAATSU training” or “pressure training”) and (“Potentiation after activation” or “PAP” or “muscle recruitment” or “explosive power” or “lower extremity”) and (“RCT”).
2.2 Inclusion and exclusion criteria
2.2.1 Inclusion criteria
Type of study: All included literature were publicly published and involved randomized controlled trials (RCTs) that studied the effects of BFR training on muscle activation and post-activation enhancement effects. Study subjects: The study subjects included healthy adults, both with and without prior training experience. Interventions: The experimental group received BFR training, while the control group received either other training modalities or no training at all. Outcome measures: The primary outcome measure was related to the quantitative lower limb (below the point of the iliac crest) (Zhao et al., 2006), which included measures of muscle activation and PAP such as MVC moment, EMG value, and longitudinal jump height. Additional criteria: The studies should provide details about the experimental design and intensity of BFR training, among other relevant information. Source inclusion: To minimize the risk of bias in the included literature, this study considers only articles indexed in the SCI (Science Citation Index).
2.2.2 Exclusion criteria
Unclear research type: Studies lacking clear documentation of their research type were excluded. Non–BFR training: Studies that involved interventions other than BFR training were excluded. Duplicate publications: Repeatedly published articles for which full text could not be obtained and review articles were excluded. Lack of quantitative outcome data: Studies without quantitative outcome indicators or valid data were excluded. Animal experiments: Research that involved animal experiments were excluded.
2.3 Data Extraction
The collected literature were imported into EndNote software and underwent independent screening by two researchers. The steps of literature screening and inclusion are illustrated in Figure 1. Ultimately, 18 articles were included in this review.
Data extraction: Two researchers independently extracted information according to a custom-made form, which primarily encompassed the following categories:
1. General information: first author and year of publication.
2. Sample information: details about the research subjects such as age and sample sizes for both the experimental and control groups.
3. Characteristics of exercise intervention: information on intervention measures for both the experimental and control groups, and specifics of the intervention programs for the experimental group (such as training methods, training volume, and training intensity).
4. Outcome indicators: quantitative measures of lower limb muscle activation and test indicators related to PAP.
2.4 Statistical analysis
Statistical analysis was performed using ReviewManager 5.3 software. The outcome indicators included in the literature in this study were continuous variables, and standardized mean differences (SMDs) and 95% confidence intervals were chosen for effect sizes because of the different testing methods used for each indicator. The Cochrane risk bias assessment tool was referenced for literature quality assessment. The homogeneity test (Q test, test level a = 0.1) was used to test for heterogeneity, and I2 values from 0% to 100%, I2 > 50%, and P < a indicated the existence of heterogeneity, and the random effects model was selected. The random effects model was chosen for meta-analysis, while the fixed effects model was chosen for the opposite. The subgroup analysis was used for the treatment of heterogeneity and STATA 16.0 was used for sensitivity analysis to test the stability of the results. The Egger test and funnel map were used to check for the presence of publication bias.
3 Results
3.1 Study characteristics
A total of 18 publications were included in this study, all of which were RCTs, which included 267 subjects with mixed genders and an age range of 18–33 years, with the basic characteristics shown in Table 1.
3.2 Study quality assessment
The quality of the collected literature was evaluated with reference to the Cochrane risk of bias assessment tool (Higgins and Altman, 2007). Review Manager 5.3 software was used to assess seven aspects, namely, the random allocation method, allocation scheme concealment, participant blinding, outcome blinding, completeness of outcome data, selective reporting of study results, and other sources of bias (Figures 2A, B). Twelve articles did not clearly describe whether the implementer of the assignment had strictly performed the random assignment, and 18 articles had a high risk of bias due to informed consent being signed before the experiment. Thus, there was a high risk of bias in the blinding.
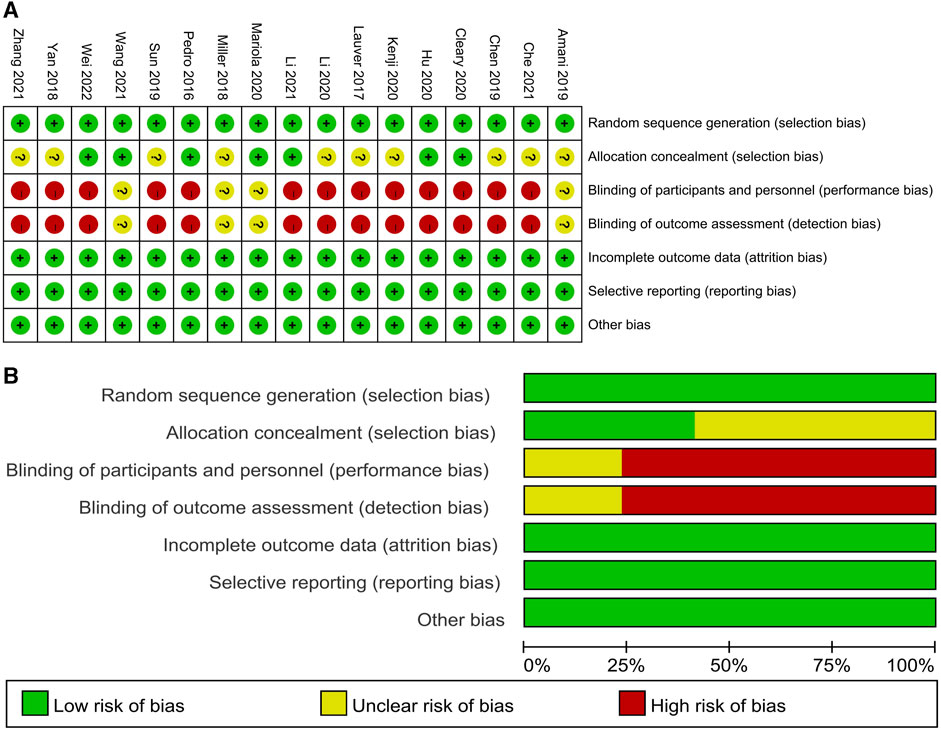
FIGURE 2. Methodological quality graph and summary of the included studies: (A) risk of bias summary and (B) risk of bias graph.
3.3 Lower limb muscle activation
Out of the 18 included articles, 10 compared 127 subject differences in RMS standard values before and after BFR training (Figure 3). After testing for heterogeneity, I2 = 0.0% < 50% and p = 0.70 > 0.1 for the Q test, suggesting no heterogeneity among the included literature. The fixed effects were selected for the meta-analysis. The SMD value for the pooled 10 studies was 0.98, with a 95% confidence interval from 0.71 to 1.224 and statistically significant, with Z = 7.21 and p ≤ 0.05, indicating that BFR exercises can significantly improve RMS values in lower limb muscles.
3.4 PAP
For BFR training–induced PAP, 13 articles were included (16 studies with a total of 261 subjects) and were tested for heterogeneity, and I2 = 51% > 50% and Q-test of p = 0.01 < 0.1, indicating a strong heterogeneity among the included literature, and random effects models were selected. A meta-analysis was performed (Figure 4) and the results showed that the combined effect size was SMD = 0.28 and statistically significant (Z = 2.13 and p = 0.03), which indicates that BFR training could significantly induce the production of PAP when compared with the control group.
3.5 Subgroup analysis
Based on the data of this study, the authors suspect that the source of heterogeneity may be the inconsistency of PAP effect test indicators. Therefore, according to the test indicators of the PAP effect (MVC: torque, CMJ: longitudinal jump, and P(W): output power), 13 articles (16 studies) were divided into three groups for the subgroup analysis (Table 2). According to the exercise modality, the included studies were divided into three subgroups: ≤30% 1RM resistance exercises, ≥70% 1RM resistance exercises, and self-weight exercises. For compressive strength, the subgroups were categorized based on the arterial occlusion and thigh circumference, according to Loenneke Jeremy et al. (2015), and three subgroups were identified: 40% AOP and below, 40%–60% AOP, and 60% AOP and above.
The subgroup analysis of the outcome indicators showed that the heterogeneity of the three groups was 66%, 28%, and 0%. When compared with the overall combined effect (I2 = 51%),the within-group heterogeneity of the MVC moments had increased to 66%, which suggests strong heterogeneity among the studies with respect to the outcome indicator of the MVC moment. The heterogeneity within the literature on the outcome indicator of the MVC moment was strong. The CMJ longitudinal jump group had the highest effect size and was statistically significant (SMD = 0.45 and p = 0.0004 < 0.01). The available results suggest that BFR training can significantly improve CMJ performance. The available results also suggest that BFR training can significantly increase the vertical jump height of CMJ.
The subgroup analysis of exercise patterns showed that the heterogeneity of the three groups was 40%, 0%, and 33%, which is lower than the overall combined effect (I2 = 51%). It is speculated that different exercise methods may be the influencing factors that lead to the heterogeneity between studies. When compared to resistance exercises at 30% 1RM and below, as well as resistance exercises at 70% and above, self-weight exercise had the largest effect, with an SMD of 0.57, and it was statistically significant (p < 0.01). This indicates that BFR training combined with self-weight exercise can significantly induce the generation of PAP.
The subgroup analysis of pressure intensity revealed varying degrees of heterogeneity among the three groups: 73%, 19%, and 0%, respectively, in comparison to the overall combined effect (I2 = 51%). Notably, within the subgroup of compressive strength of 40% AOP and below, the intra-group heterogeneity increased to 73% (I2 = 73%), which indicates significant heterogeneity among the studies in this category. Among these, the effect size of the 40%–60% AOP group was the highest and statistically significant (SMD = 0.57 and p < 0.01), which suggests that the pressurization intensity of BFR training at 40%–60% AOP significantly induces PAP production.
3.6 Sensitivity analysis
Sensitivity analysis was conducted on the included literature, both by including and excluding individual groups of studies, to assess heterogeneity.
In Table 3, the combined effect of BFR training on lower limb muscle activation, as included in all studies, resulted in an SMD of 0.98 (p < 0.01 and I2 = 0%). The SMD range from individual studies was from 0.94 to 1.06, with I2 = 0%, and all p-values were <0.01. There was no single literature that posed a threat to the meta-analysis results, which indicates the study’s stability.
Table 4 presents the combined effect of BFR training on the PAP effect across all included studies, with an SMD of 0.28 (p = 0.03 and I2 = 51%). After excluding the studies by Hongwen and Xiang (2022) and Lauver et al. (2017), the combined effects were 0.09 and 0.002, respectively, with I2 values of 37% and 31%. This resulted in a significant reduction in heterogeneity. The combined effect, after excluding other single studies, had an SMD of 0.02–0.07, with p-values < 0.1.
3.7 Publication bias
We conducted bias tests separately by drawing funnel plots within the subgroups to assess the publication bias of the included studies. The funnel plots exhibited symmetry, which indicates no publication bias, as illustrated in Figures 5A–D.
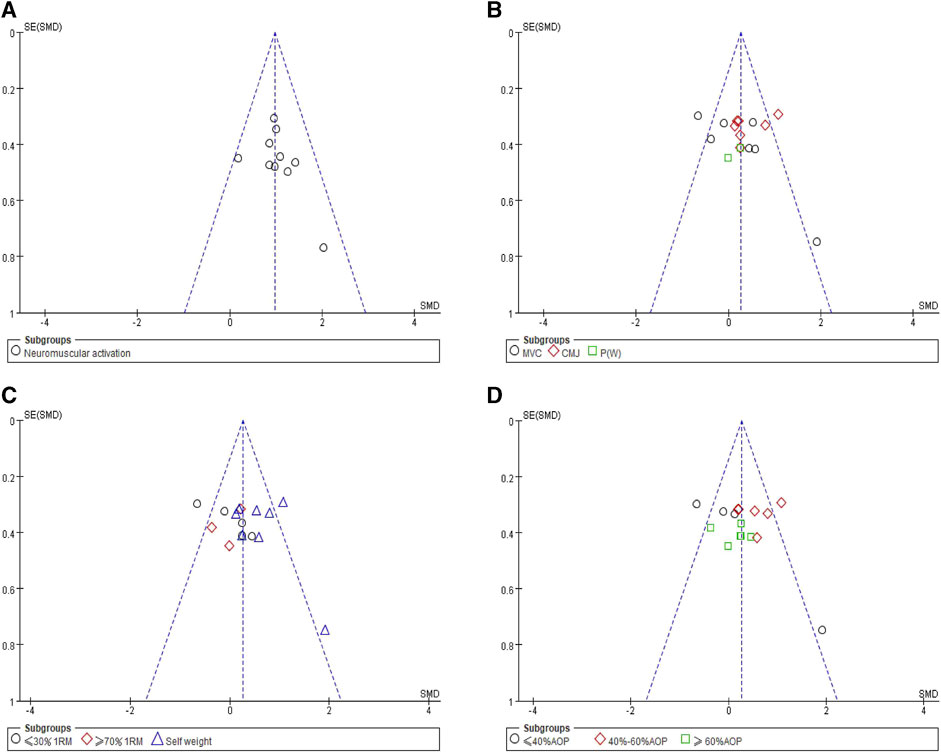
FIGURE 5. Funnel plots of meta-analysis: (A) neuromuscular activation, (B) outcome extracted, (C) resistance strength, and (D) compressive strength.
Furthermore, we continued to examine potential bias using the Egger test, and all p-values were found to be >0.05, as presented in Table 5. Therefore, it can be concluded that there is no publication bias present in the literature analyzed in this study.
4 Discussion
4.1 BFR training–induced muscle activation in the lower limbs
The induction method employed in this study is BFR training. By combining low-intensity resistance exercises (Lauver et al., 2017; Cleary Christopher and Cook Summer, 2020; Hu, 2020; Li, 2020; Che et al., 2021; Wang, 2021), high-intensity resistance exercises (Pedro et al., 2016; Pan et al., 2019), and aerobic activities such as running (Amani et al., 2019; Yun-Tsung et al., 2019), we assessed their impact on electromyography (EMG) readings of lower limb muscles. As for the criteria for evaluating lower limb muscles, specific sites below the iliac crest point (Wu et al., 2020) were selected, which primarily included the vastus medialis (VM), rectus femoris (RF), gluteus maximus (GM), biceps femoris (BF), and other muscle groups.
Muscle mass and strength development are governed by neural modulation and stimuli (Marcotte et al., 2015). The results of the meta-analysis indicate that the effect sizes from the overall effect tests in all 10 studies were consistently positive (p < 0.01). Therefore, BFR training significantly enhances the root mean square (RMS) values of lower limb muscles. The heterogeneity test results indicate complete homogeneity among the study outcomes (I2 = 0%), and the sensitivity analysis showed no significant changes in heterogeneity or combined effects when any single study was removed. This suggests that there are no differences related to participant characteristics, exercise methods, pressure intensity, etc., with all included studies pointing to the same outcome.
It is well known that high-intensity exercise is achieved through the recruitment of both fast and slow muscle fibers by the nervous system. Studies have demonstrated a positive correlation between the degree of muscle activation and number of nerve fibers (Westing et al., 1991). When compared to centrifugal exercise alone, combining centrifugal exercise with BFR leads to greater neuromuscular activation (Schoenfeld Brad, 2012). This increased neuromuscular activation observed during BFR exercises is attributed to reduced muscle oxygen availability due to compression, which inhibits α motor neurons. To meet the altered energy demands, more type II (fast-twitch) muscle fibers are recruited for participation in the exercise (Yasuda et al., 2015). In the literature within this study, the control group’s intervention measures involved non-pressurized resistance exercises. Therefore, in comparison to traditional resistance exercises, BFR exercises may facilitate the recruitment of more fast-twitch muscle fibers. This recruitment potentially has a positive impact on improving explosive strength and muscle strength in athletes.
4.2 Possible mechanism of inducing PAP by BFR training
As a physiological phenomenon characterized by an acute increase in explosive force, methods aimed at inducing PAP are primarily based on heavy-load activities such as squats, bench presses, and high-intensity exercises (Zhuang, 2021). In this study, we conducted a meta-analysis of various explosive force measurement indices, such as MVC moment, CMJ vertical jump height, and P maximum output power, before and after BFR training. The results revealed an overall effect size of 0.28 (p < 0.05) across 16 studies, which indicates that BFR training can induce PAP.
Traditional PAP induction methods typically require heavy training equipment for short-term maximum or sub-maximum resistance training, which can pose a risk of sports injuries and subsequent muscle fatigue due to their high-intensity and heavy-load nature. By contrast, BFR training is characterized by low intensity and rapid recovery. Furthermore, creatine kinase (an index representing the degree of muscle damage) did not significantly increase during BFR training, suggesting that muscle fiber damage is lower than it is in high-intensity training (Xu et al., 2021).
In recent years, new warm-up programs based on the PAP effect have gained attention in the academic community. These programs combine resistance training with isometric training to enhance sports performance and have been adopted as pre-match warm-up routines to induce PAP (Yang and Huan, 2022). In summary, BFR training offers a relatively simple and convenient method for effectively inducing the PAP phenomenon when compared to traditional high-intensity resistance exercises.
The production of PAP is primarily attributed to factors such as muscle acidification, strengthening of the H-reflex, and changes in muscle fiber recruitment angles under load stimulation, which result in an increased number of recruited motor units due to motor nerve excitation (Chong et al., 2020). This phenomenon aligns with the neuromuscular activation observed in BFR exercises as mentioned earlier. Cleary Christopher and Cook Summer (2020) also found that EMG amplitudes in activated hamstrings were the highest under BFR conditions during blood restriction training, suggesting a potential link between lower limb muscle activation and BFR training–induced PAP.
4.2.1 Outcome indicators
Following the heterogeneity test (I2 = 51%), we conducted a subgroup analysis to explore the reasons for variation among studies, focusing on the outcome indicators related to the PAP effect (Table 2). The results showed that 1) BFR training resulted in a significant increase in height of the CMJ vertical jump; 2) the MVC moment did not exhibit a significant effect on the combined results, which might be attributed to the high heterogeneity in the literature (I2 = 66% and p = 0.07), with studies using the MVC moment as the outcome indicator, which is the primary source of heterogeneity; and 3) there are only two references on P (output power), and the results of the subgroup analysis also did not reach a significant level.
Three out of the seven studies, which used the MVC moment as the outcome indicator, reported negative effect sizes. The author found that these three studies when compared to the rest of the literature were all conducted on people with no athletic experience. Some researchers suggested that high-level athletes (track and field and rugby) may cause greater PAP than non-athletes (Hamada et al., 2000). Wilson et al. (2013) also demonstrated that athletes exhibited a larger effect size of PAP than non-athletes. The analysis suggests that athletes might display an enhanced response to PAP protocols due to their training adaptations. Therefore, the MVC moment test index in non-athletes may be insensitive to BFR exercises.
As an effective indicator of the PAP effect, CMJ vertical jump height is usually quantified by the following formula (Cleary Christopher and Cook Summer, 2020):
A value >100 indicates the generation of PAP. In the literature comprising seven studies on CMJ longitudinal jump, all reported positive effects, and there was no observed heterogeneity within this group (I2 = 28%). This suggests that combining BFR exercises may further enhance the PAP effect and optimize the CMJ vertical jump index, thus aligning with Cleary’s perspective.
4.2.2 Exercise mode
An analysis of subgroups based on different exercise methods found that when compared to combining BFR training with low-intensity resistance exercise and high-intensity resistance training, combining BFR with body-weight exercises is more likely to induce PAP.
In traditional resistance training, effective improvements in muscle absolute strength typically requires an intensity of ≥70% 1RM (Ratame et al., 2009). However, the occurrence of the PAP phenomenon depends on post-training fatigue levels (Boullosa et al., 2013). Consequently, determining the optimal method to induce PAP is challenging, and for various intensity resistance exercises combined with BFR, participants may experience levels of fatigue greater than that induced by PAP. Additionally, Che et al. (2021) found that the subjective fatigue level during pressurized low-intensity resistance exercises, measured using RPE, was more pronounced in the pressurized training state.
The recruitment of high-threshold motor units is one of the mechanisms proposed by PAP, as observed by Chen et al. (2022); we found that during a 120-s warm-up at varying intensities, the higher the exercise intensity, the more pronounced the warm-up effect. Although more muscle fibers are recruited, contributing to enhanced muscle strength (Sweeney et al., 1993), BFR exercises combined with light loads (such as self-weight) may also trigger similar responses as heavy-load exercises. For instance, Miller et al. (2018) discovered that in a study combining BFR with whole-body vibration, both whole-body vibration and maximum isometric contraction exercises significantly improved vertical jump height under BFR conditions. This indicates that BFR training can yield comparable effects to high-load training, even with lower training intensity, thereby reducing the risk of sports injuries and excessive exercise loads.
4.2.3 Compressive strength
With the change in pressure intensity, the induction degree of PAP is also different. When compared to no BFR training conducted conditions, lower limb occlusion above the brachial artery systolic pressure induced a significant amount of PAP stimulation. However, intramuscular hypoxia caused by high-intensity compression easily accelerated the fatigue response of muscle fibers (García-Pinillos et al., 2015).
A study also showed that low-intensity exercise with BFR pressure hardly induces the body’s stress response (Wang, 2021). High-intensity BFR pressure can lead to anaerobic metabolism, which results in lactic acid accumulation and a significant decrease in muscle torque. On the other hand, excessive AOP can easily cause severe limb ischemia and cardiovascular adverse events due to muscle edema (Spranger et al., 2015). Therefore, it is recommended to use BFR exercise with 40%∼60% AOP for immediate muscle strength growth.
4.3 Study limitations
The 18 articles included were not blinded and the methodology is limited in this respect. It is difficult to use blinding in this type of study because of the corresponding ethical requirement for human subjects to sign an informed consent form. In the few articles retrieved for this study, the authors could not be contacted to obtain the required data, which is theoretically biased, but the authors do not believe that this would have a subversive effect on the results of this study. Limitations in the research field and theme, such as the small number of studies evaluating lower extremity muscle function, the generally low sample sizes, and variations in intervals after BFR exercises among studies, may have introduced potential bias. Furthermore, despite extensive research, there remains a shortage of large-scale, high-quality studies on BFR training. Future studies should improve the credibility of the study by expanding the sample size as much as possible by improving the experimental design. For PAP induction, the effects of the training status, exercise interval time, and other factors on the PAP effect should be further clarified.
5 Conclusion
BFR exercises induce lower limb muscle activation and PAP effects to overcome self-weight. BFR exercises with 40%–60% AOP are more likely to induce PAP. BFR exercises in combination with a warm-up to overcome dead weight help improve the height of the CMJ vertical jump.
Data availability statement
The original contributions presented in the study are included in the article/Supplementary material; further inquiries can be directed to the corresponding author.
Author contributions
JW, HL, and LJ: Conceptualization. JW: Methodology, resources, and supervision. HL and LJ: Software, formal analysis, and visualization. JW: Validation. LJ: Investigation. HL: Data curation. JW: Manuscript writing–original draft preparation. HL: Manuscript writing–review and editing. All authors contributed to the article and approved the submitted version.
Conflict of interest
The authors declare that the research was conducted in the absence of any commercial or financial relationships that could be construed as a potential conflict of interest.
Publisher’s note
All claims expressed in this article are solely those of the authors and do not necessarily represent those of their affiliated organizations, or those of the publisher, the editors and the reviewers. Any product that may be evaluated in this article, or claim that may be made by its manufacturer, is not guaranteed or endorsed by the publisher.
References
Amani S., Farhani F., Rajabi H., Abbasi A., Sarikhani A. (2019). Blood flow restriction during futsal training increases muscle activation and strength. Front. Physiology 10 (1), 06–14. doi:10.3389/fphys.2019.00614
Batista Mauro A. B., Hamilton R., Renato B., Ugrinowitsch C., Tricoli V. (2011). Influence of strength training background on postactivation potentiation response. J. strength Cond. Res. 25 (9), 2496–2502. doi:10.1519/JSC.0b013e318200181b
Boullosa D. A., Abreu L., Beltrame L. G. N., Behm D. G. (2013). The acute effect of different half squat set configurations on jump potentiation. J. Strength & Cond. Res. 27 (8), 2059–2066. doi:10.1519/JSC.0b013e31827ddf15
Che T., Yang T., Liang Y. (2021). The effect of lower limb low intensity compression squat training on the activation level and subjective fatigue of core muscle groups. Sports Sci. 41 (7), 59–66+78. doi:10.16469/j.css.202107007
Chen H., Xu J., Shen X. (2022). The effect of high-intensity and moderate intensity warm-up on 120s exercise output power. J. Hangzhou Normal Univ. Nat. Sci. Ed. 21 (02), 213–218+224. doi:10.19926/j.cnki.issn.1674-232X.2022.02.015
Chong C., Wang A., Duan Y. (2020). A review of research on postactivation enhancement effect. Sports Res. Educ. 35 (6), 87–91. doi:10.16207/j.cnki.2095-235x.2020.06.014
Cleary Christopher J., Cook Summer B. (2020). Postactivation potentiation in blood flow-restricted complex training. J. strength Cond. Res. 34 (4), 905–910. doi:10.1519/JSC.0000000000003497
Doma K., Leicht A. S., Boullosa D., Woods C. T. (2020). Lunge exercises with blood-flow restriction induces post-activation potentiation and improves vertical jump performance. Eur. J. Appl. Physiology 120 (3), 687–695. doi:10.1007/s00421-020-04308-6
García-Pinillos F., Soto-Hermoso V. M., Latorre-Román P. A. (2015). Acute effects of extended interval training on countermovement jump and handgrip strength performance in endurance athletes: postactivation potentiation. J. Strength & Cond. Res. 29 (1), 11–21. doi:10.1519/JSC.0000000000000591
Gepfert M., Krzysztofik M., Kostrzewa M. (2020). The acute impact of external compression on back squat performance in competitive athletes. Int. J. Environ. Res. Public Health 17 (13), 46–74. doi:10.3390/ijerph17134674
Hamada T., Sale D. G., Macdougall J. D. (2000). Postactivation potentiation in endurance-trained male athletes. Med. Sci. Sports Exerc. 32 (2), 403–411. doi:10.1097/00005768-200002000-00022
Hanson E. D., Leigh S., Mynark R. G. (2007). Acute effects of heavy- and light-load squat exercise on the kinetic measures of vertical jumping. J. Strength & Cond. Res. 21 (4), 1012–1017. doi:10.1519/R-20716.1
Higgins J., Altman D. (2007). Quality evaluation of included studies: new suggestions for data extraction forms. Chin. J. Evidence-based Med. (1), 61. doi:10.3969/j.issn.1672-2531.2007.01.010
Hongwen W., Xiang J. (2022). Study on the post activation enhancement effect of rapid stretching compound exercise accompanied by blood flow restriction. J. Henan Normal Univ. Nat. Sci. Ed. 50 (1), 144–149. doi:10.16366/j.cnki.1000-2367.2022.01.017
Hu Y. (2020). The effect of compression low intensity resistance training on lower limbmuscle activation of swimmer. Suzhou University.
Lauver J. D., Cayot T. E., Rotarius T. (2017). The effect of eccentric exercise with blood flow restrictionon neuromuscular activation, microvascular oxygenation, and the repeated bout effect. Eur. J. Appl. Physiology 117 (5), 1–11. doi:10.1007/s00421-017-3589-x
Li Z. (2020). The effect of pressure resistance training on lower limb muscle activation and strength gain in male handball players. Fujian Normal University.
Li Z., Yu S., Li J. (2021). The effect of applying different arterial occlusive pressures and intermittent methods during weight-bearing squats on the activation characteristics of thigh muscles. Chin. J. Appl. Physiology 37 (3), 300–305. doi:10.12047/j.cjap.6056.2021.017
Lin T. C., Cheng C. C., Cai Z. Y. (2017). Effects of local vibration with blood flow restriction on muscle activation. Isokinet. Exerc. Sci. 26 (1), 171–174. doi:10.3233/IES-171174
Loenneke Jeremy P., Kim D., Fahs Christopher A., Thiebaud R. S., Abe T., Larson R. D., et al. (2015). Effects of exercise with and without different degrees of blood flow restriction on torque and muscle activation. Muscle & nerve 51 (5), 713–721. doi:10.1002/mus.24448
Marcotte G. R., West D. W. D., Baar K. (2015). The molecular basis for load-induced skeletal muscle hypertrophy. Calcif. Tissue Int. 96 (3), 196–210. doi:10.1007/s00223-014-9925-9
Mccann M. R., Flanagan S. P. (2010). The effects of exercise selection and rest interval on postactivation potentiation of vertical jump performance. J. Strength & Cond. Res. 24 (5), 1285–1291. doi:10.1519/JSC.0b013e3181d6867c
Miller R. M., Victoria M. K., Freitas E. D. S., Heishman A. D., Knehans A. W., Bemben D. A., et al. (2018). Effects of blood-flow restriction combined with postactivation potentiation stimuli on jump performance in recreationally active men. J. Strength Cond. Res. 32 (7), 1869–1874. doi:10.1519/JSC.0000000000002110
Pan Y., Zhao Y., Ma X., Tian Y., Sun Q. (2019). The effect of blood flow restriction combined with low intensity resistance exercise on skeletal muscle microcirculation, neuromuscular activation, and subjective fatigue in low weight women. Chin. J. Sports Med. 38 (8), 677–684. doi:10.16038/j.1000-6710.2019.08.007
Pedro F., Reis Joana F., Mendonca Goncalo V., Avela J., Mil-Homens P. (2016). Acute effects of exercise under different levels of blood-flow restriction on muscle activation and fatigue. Eur. J. Appl. physiology 116 (5), 985–995. doi:10.1007/s00421-016-3359-1
Ratame S. S. N. A., Alvar B. A., Evetoch T. K. (2009). Progression models in resistance training for healthy adults. Med. Sci. Sports Exerc. 41 (41), 687–708. doi:10.1249/MSS.0b013e3181915670
Schoenfeld Brad J. (2012). Does exercise-induced muscle damage play a role in skeletal muscle hypertrophy. J. strength Cond. Res. 26 (5), 1441–1453. doi:10.1519/JSC.0b013e31824f207e
Spranger M. D., Krishnan A. C., Levy P. D., O'Leary D. S., Smith S. A. (2015). Blood flow restriction training and the exercise pressor reflex: a call for concern. Am. J. Physiol. Heart Circ. Physiol. 309 (H9), 1440–1452. doi:10.1152/ajpheart.00208.2015
Sun Ke, Wenzhe W., Zhao Z. (2019). Differences in muscle activity between blood flow restricted and unrestricted parts during low intensity compression training of the lower limbs. Chin. Sports Sci. Technol. 55 (5), 14–19. doi:10.16470/j.csst.2019044
Sweeney H. L., Bowman B. F., Stull J. T. (1993). Myosin light chain phosphorylation in vertebrate striated muscle: regulation and function. Am. J. Physiology 264 (1), 1085–1095. doi:10.1152/ajpcell.1993.264.5.C1085
Wang M. (2021). Study on the immediate effects of low load resistance exercise onMuscle volume and strength under different blood flow restriction pressures. Chengdu Sport University.
Westing S. H., Cresswell A. G., Thorstensson A. (1991). Muscle activation during maximal voluntary eccentric and concentric knee extension. Eur. J. Appl. physiology Occup. physiology 62 (2), 104–108. doi:10.1007/BF00626764
Wilson J. M., Duncan N. M., Marin P. J., Brown L. E., Loenneke J. P., Wilson S. M. C., et al. (2013). Meta-analysis of postactivation potentiation and power: effects of conditioning activity, volume, gender, rest periods, and training status. J. Strength Cond. Res. 27 (3), 854–859. doi:10.1519/JSC.0b013e31825c2bdb
Wu C., Zhang Y., Zhai Y. (2020). Pre activation enhancing effect and endurance exercise: performance, application, and regulation. Hubei Sports Sci. Technol. 39 (1), 53–57. doi:10.3969/j.issn.1003-983X.2020.01.013
Xu D., Wu J., Gui P. (2021). The effect of lower limb blood flow restriction based on limb linkage training on human lung endurance, lower limb muscle endurance, and balance function in healthy young people. Chin. Rehabil. Theoryand Pract. 27 (12), 1470–1475. doi:10.3969/j.issn.1006-9771.2021.12.014
Xu F., Wang J. (2013). Pressurized strength training: definition and application. Sports Sci. 33 (12), 71–80. (in Chinese). doi:10.3969/j.issn.1000-677X.2013.12.014
Xu J., Zhan G., Wang Lu (2020). Content construction of unstable resistance training and its activation effect on muscles. Hubei Sports Technol. 39 (2), 135–138+171. doi:10.3969/j.issn.1003-983X.2020.02.009
Yan Y., Guo L. (2018). Research on the effect of running combined with lower LimbCompression on the performance of tennis players. J. Southwest Normal Univ. Nat. Sci. Ed. 43 (6), 167–175. doi:10.13718/j.cnki.xsxb.2018.06.027
Yang K., Huan C. (2022). Application of a warm-up plan based on the PAP effect in shot put training and competition. Sports Sci. Technol. Literature Bull. 30 (1), 61–64+246. doi:10.19379/j.cnki.issn.1005-0256.2022.01.019
Yasuda T., Loenneke J. P., Thiebaud R. S., Abe T. (2015). Effects of detraining after blood flow-restricted low-intensity concentric or eccentric training on muscle size and strength. J. Physiological Sci. Jps 65 (1), 139–144. doi:10.1007/s12576-014-0345-4
Yun-Tsung C., Hsieh Y. Y., Ho J. Y., Lin J. C. (2019). Effects of running exercise combined with blood flow restriction on strength and sprint performance. J. strength Cond. Res. 35 (11), 3590–3596. doi:10.1519/jsc.0000000000003313
Zhang Yu. (2021). Research on the effect of warming up methods on the training effects of different intensities for teenage cyclists. Shanghai Normal University.
Zhao C., Jin L., Yang Q. (2006). Lower limb length measurement and postoperative unequal length treatment after total hip replacement. Int. J. Osteol. 027 (4), 205–207. doi:10.3969/j.issn.1673-7083.2006.04.005
Keywords: blood flow restriction training, muscle activation, post-activation potentiation, meta-analysis, lower limb muscle
Citation: Wang J, Liu H and Jiang L (2023) The effects of blood flow restriction training on PAP and lower limb muscle activation: a meta-analysis. Front. Physiol. 14:1243302. doi: 10.3389/fphys.2023.1243302
Received: 20 June 2023; Accepted: 20 October 2023;
Published: 09 November 2023.
Edited by:
Erika Zemková, Comenius University, SlovakiaReviewed by:
Jan Mieszkowski, Gdansk University of Physical Education and Sport, PolandEmiliano Cè, University of Milan, Italy
Copyright © 2023 Wang, Liu and Jiang. This is an open-access article distributed under the terms of the Creative Commons Attribution License (CC BY). The use, distribution or reproduction in other forums is permitted, provided the original author(s) and the copyright owner(s) are credited and that the original publication in this journal is cited, in accordance with accepted academic practice. No use, distribution or reproduction is permitted which does not comply with these terms.
*Correspondence: Haiyang Liu, YWNjNjY3Nzg4OUAxMjYuY29t