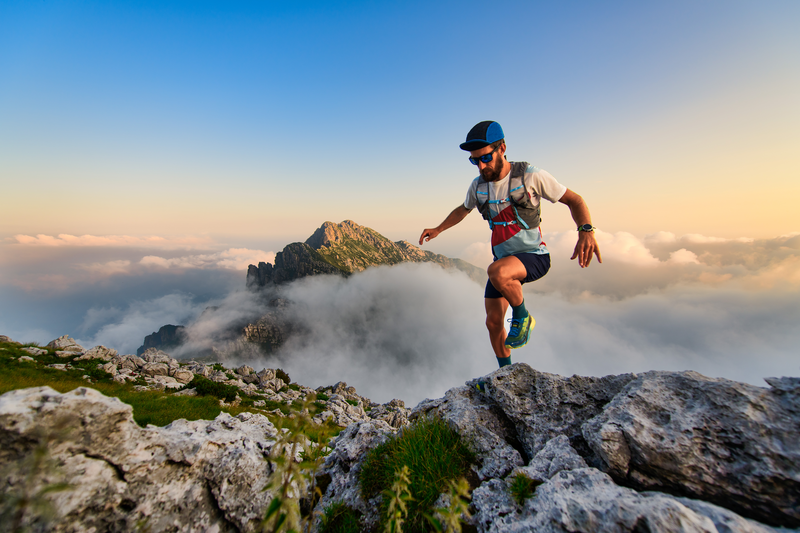
94% of researchers rate our articles as excellent or good
Learn more about the work of our research integrity team to safeguard the quality of each article we publish.
Find out more
ORIGINAL RESEARCH article
Front. Physiol. , 30 June 2023
Sec. Integrative Physiology
Volume 14 - 2023 | https://doi.org/10.3389/fphys.2023.1225552
This article is part of the Research Topic 72nd Annual Meeting of the Italian Society of Physiology: New Perspectives in Physiological Research View all 13 articles
Introduction: Aging is a process characterised by a decline in physiological functions. Reactive species play a crucial role in the aging rate. Due to the close relationship between aging and oxidative stress, functional foods rich in phytochemicals are excellent candidates to neutralise age-related changes.
Aim: This investigation aims to verify the potential protective role of bergamot (Citrus bergamia, Femminello cultivar) peel and juice extract in a model of aging represented by human red blood cells (RBCs) exposed to D-Galactose (DGal).
Methods: Bergamot peel and juice extracts were subjected to RP-HPLC/PDA/MS for determination of their composition in bioactive compounds. Markers of oxidative stress, including ROS production, thiobarbituric acid reactive substances (TBARS) levels -a marker of lipid peroxidation, oxidation of total protein sulfhydryl groups, as well as the expression and anion exchange capability of band 3 and glycated haemoglobin (A1c) production have been investigated in RBCs treated with D-Gal for 24 h, with or without pre-incubation for 15 min with 5 μg/mL peel or juice extract. In addition, the activity of the endogenous antioxidant system, including catalase (CAT) and superoxide dismutase (SOD), as well as the diversion of the RBC metabolism from glycolysis towards the pentose phosphate pathway shunt, as denoted by activation of glucose-6-phosphate dehydrogenase (G6PDH), have been explored.
Results: Data shown here suggest that bergamot peel and juice extract i) prevented the D-Gal-induced ROS production, and consequently, oxidative stress injury to biological macromolecules including membrane lipids and proteins; ii) significantly restored D-Gal-induced alterations in the distribution and ion transport kinetics of band 3; iii) blunted A1c production; iv) effectively impeded the over-activation of the endogenous antioxidant enzymes CAT and SOD; and v) significantly prevented the activation of G6PDH.
Discussion: These results further contribute to shed light on aging mechanisms in human RBCs and identify bergamot as a functional food rich in natural antioxidants useful for prevention and treatment of oxidative stress-related changes, which may lead to pathological states during aging.
Aging is a biological process that results in a progressive and non-reversible decline in the physiological functions of all body organs and is caused by damage accumulation with concomitant elevation of oxidative stress (Fukagawa, 1999; Akki et al, 2018; Akki et al, 2019; Ferrera et al, 2021). In 1972, Denham Harman (Harman, 1972) postulated the free radical theory of aging, which points to accumulation of reactive species as the underlying reason for the oxidation of the biological macromolecules and consequent cell injury, thereby explaining the alterations in cell functions during natural aging (Junqueira et al, 2004; Remigante and Morabito, 2022).
Aerobic cell metabolism requires oxygen as the final electron acceptor of oxidation reactions and reactive oxygen species (ROS) represent a byproduct of this process. The physiological role of red blood cells (RBCs) is the transport of respiratory gases from the lungs to the tissues and vice versa, in order to supply the cells with oxygen. In the blood stream, RBCs are continuously exposed to both endogenous and exogenous reactive species able to injury their structure, thus impairing their physiology and functionality (Rizvi and Maurya, 2007; Remigante et al, 2021a; Fujii et al, 2021; Arrigo et al, 2023). Un-neutralized reactive species react with both RBC plasma membrane lipids and proteins and promote oxidative alterations at level of lipids (lipid peroxidation) and proteins (protein oxidation) and/or fragmentation, respectively (Avitabile et al, 2020). Alternatively, the accumulation of reactive species results in the induction of glycation reactions, which leads to the increased endogenous production of advanced glycation end products (AGEs) (Moldogazieva et al, 2019). Non-enzymatic glycation of membrane glycoproteins and/or haemoglobin as well as their gradual accumulation within RBCs can account for altered rheologic properties of RBCs (Turk et al, 1998). In RBCs, oxidative stress-related aging is accompanied by a decrease in cell volume and hemoglobin content and an increase in cell density (Pandey and Rizvi, 2010). These alterations are correlated with a loss of cholesterol and phospholipids, resulting in a decrease in the surface area that reflects a loss of membrane lipids and protein constituents. The reduction of surface area could be explained by a gradual membrane blebbing and vesiculation (Willekens et al., 2008), both accelerated in aged RBCs (Pandey and Rizvi, 2011). In addition, these changes might limit the ability of the RBCs to maintain the highly deformable biconcave shape necessary to pass through the narrow capillaries, thus contributing to their removal from circulation (Shiga et al, 1990; Pretorius, 2018).
One of the major goals of RBC redox regulation results in protection of their main plasma membrane protein, band 3 (SLC4A1/AE1) (Abbas et al, 2018). Band 3 is an integral trans-membrane protein that plays different functions: 1) the maintenance of anion homeostasis (Reithmeier et al, 2016); 2) the binding between plasma membrane lipids and cytoskeletal proteins, reflecting on cell shape (De Franceschi et al, 2012; Vallese et al, 2022; Spinelli et al, 2023); 3) the docking, at level of the N-terminal cytosolic domain that protrudes into the cytosol, of a series of structural proteins and glycolytic enzymes (e.g., glyceraldehyde 3-phosphate dehydrogenases: GAPDH) (Campanella et al, 2008; Puchulu-Campanella et al, 2013), as well as cytosolic proteins such as haemoglobin (Zhang et al, 2003). Moreover, haemoglobin, in addition to its central role of carrying oxygen from the lungs to peripheral tissues, may serve as oxygen sensor, in order to appropriately link band 3 to regulation of the RBC metabolism (Ellsworth, 2000; Puchulu-Campanella et al, 2013). In fact, several studies have provided compelling, albeit indirect, evidence that, when not encumbered by deoxy-hemoglobin, the N-terminal of band 3 can bind to and, in turn, inhibit the glycolytic enzyme GAPDH (Issaian et al, 2021). Oxygen-dependent metabolic modulation is mediated by the competitive binding of deoxy-hemoglobin and glycolytic enzymes to the band 3 cytosolic domain. When oxidant stress is high, GAPDH enzyme is bound to band 3 and thereby inhibited (Reisz et al, 2016). In this context, RBCs favor glucose oxidation through the pentose phosphate pathway (PPP), in order to generate the reducing cofactor NADPH and fuel endogenous antioxidant systems. On the other hand, when oxidant stress is low, haemoglobin is deoxygenated and binds to the band 3 N-terminus, which in turn favors the release of glycolytic enzymes from the membrane to promote the generation of energy in the form of ATP and NADH through glycolysis (Castagnola et al, 2010). This balance may be dysregulated by early RBCs aging and/or increased reactive oxygen species (ROS) levels, thus depriving RBCs of their crucial metabolic plasticity and leading to their removal from the blood circulation (Kuhn et al, 2017). Therefore, the regulation of the balance between glycolysis and PPP is essential and enables RBCs to counteract oxidative insults impacting on their cell structures/functions. In addition, unusual levels of reactive species could be the common denominator in the development and progression of different aging-related acute and/or chronic pathologies, although the precise mechanisms contributing to oxidative stress-induced injury are still poorly clarified (Radi et al, 2014; Bo-Htay et al, 2018; Martinelli et al, 2020; Emanuelli et al, 2022; Guo et al, 2022; Remigante and Morabito, 2022).
To contain oxidative stress effects, RBCs possess an excellent cytosolic antioxidant machinery composed of non-enzymatic as well as enzymatic antioxidants, which represent an effective antioxidant equipment to protect RBCs themselves along with other body cells and tissues (Zabinski et al, 2000; Inal et al, 2001). The use of natural secondary metabolites such as polyphenol-rich extracts with antioxidant properties could be an excellent and workable alternative for supporting the integrated antioxidant system (Jacob, 1995; Lunceford and Gugliucci, 2005; Dorta et al, 2008; Kouka et al, 2017; Xu et al, 2019; Remigante et al, 2022a; Remigante et al, 2022b). In this regards, special attention has been paid to the potential health benefits of the flavonoid fraction of the bergamot (Citrus bergamia) (Russo et al, 2016).
Bergamot is a small tree belonging to Rutaceae family that is mainly cultivated in a specific area of the region of Calabria (Italy) known to ensure a microclimate suitable for its growth. The essential oil of bergamot, which is obtained from the fruit peel, has been extensively characterized and used in cosmetic and food industries, whereas the bergamot by-products, such as the pulp and juice, have been only recently evaluated for their beneficial properties, which include cholesterol reduction, antioxidant and anti-inflammatory effects (Ferlazzo et al, 2015; Russo et al, 2015; Carresi et al, 2016; Lauro et al, 2016; Musolino et al, 2019). This reassessment may lead to reduction in the disposal costs of industrial processes and gain of a good source of nutraceuticals, thus representing an economic advantage (Russo et al, 2021). To date, no scientific study evaluating the anti-aging properties of peel and/or juice extracts from bergamot in human RBCs has been reported.
The long-term exposure to high doses of D-Galactose (D-Gal) represents a good experimental model of natural aging (Azman and Zakaria, 2019). Thus, the present investigation aims to identify the potential beneficial effects of bergamot peel and juice extracts from Femminello cultivar on the molecular mechanisms underlying natural aging in RBCs, including oxidative damage, glycation events and activation of the endogenous enzymatic defense system. To this aim, juice and peel extracts from bergamot fruits belonging to Femminello cultivar were subjected to RP-HPLC/PDA/MS for determination of their precise composition in bioactive compounds and their effects were evaluated in a D-Gal-induced model of aging in human RBCs.
Water, formic acid, ethanol, acetonitrile and methanol were obtained from Merck Life Science (Merck KGaA, Darmstadt, Germany). The standard compounds ferulic acid, synapic acid, eriocitrin, narirutin, and neohesperidin were obtained from Extrasynthese (Genay Cedex, France). Apigenin 6,8-di-C-glucoside, diosmetin 6,8-di-C-glucoside, naringin, brutieridin, limonin glucoside, nomilin glucoside, nomilinic acid glucoside, limonin, melitidin, nomilin and neoeriocitrin were previously isolated in our laboratory. Bergamot (Citrus bergamia) peels used in this research belong to Femminellocultivar and the experimental protocol has been performed with peels obtained from two fruits. Fruits were collected at the same stage of ripeness from trees grown in Calabria (Melito di Porto Salvo, Reggio Calabria, Italy) on January 2023. Fruits were washed, dried and stored at +4°C, then peeled and squeezed. Peels were dried at 25°C for 48 h.
The juice was subjected to RP-HPLC analysis without any pre-treatment. On the other hand, a solvent extraction procedure was performed on peel samples before RP-HPLC analysis. The bioactive compounds extraction procedure was previously validated by our group (Russo et al, 2015). Briefly, 10 g of samples were extracted with 100 mL of methanol for three times. The extract was subjected to chromatographic analysis. The juice and the peel extract were analysed in triplicate.
A Shimadzu Prominence LC-20A system (Shimadzu, Milan, Italy) was employed to carry out HPLC analyses. The HPLC instrument was equipped with SPD-M20A UV and HPLCMS-2020 detectors. The analytical procedure was previously validated by our group (Russo et al, 2014). This analytical method allowed to quantify the bioactive molecule content in juice and bergamot peel samples. Figure of merits were calculated in accordance with the EURACHEM guidelines (Group, 1998).
Chemicals used to perform experiments were purchased from Sigma (Milan, Italy). With regard to stock solutions, 4, 4′-diisothiocyanatostilbene-2, 2′-disulfonate (DIDS, 10 mM) was prepared in dimethyl sulfoxide (DMSO); D-Galactose (D-Gal, 1 M) was prepared in distilled water and N-ethylmaleimide (NEM, 310 mM) was prepared in ethanol. H2O2 experimental solution was obtained by dilution in distilled water from a 30% w/w stock solution. Ethanol never exceeded 0.001% v/v in the experimental solutions and was previously tested on RBCs to exclude haemolysis. Peel extract obtained as described above and juice devoid of the fibrous part were poured into freeze-drying flasks and placed into the vacuum chamber, frozen at −50°C, and then freeze-dried for 72 h (BenchTop K Series Freeze Dryers, VirTis Gardiner, United States). The concentrated peel extract required a dilution 1:100 w/v with distilled water prior to use in experiments.
Blood needed for the planned experiments was withdrawn from healthy volunteers (age 25–45 years) upon their informed consent. Whole blood samples, put in test tubes containing ethylenediaminetetraacetic acid (EDTA) as anticoagulant, were repeatedly washed in isotonic solution (NaCl 150 mM, 4-(2-hydroxyethyl)-1-piperazineethanesulfonic acid (HEPES) 5 mM, glucose 5 mM, pH 7.4, osmotic pressure 300 mOsm/kgH2O; centrifugation with Neya 16R, 1,200 × g, 5 min) to eliminate plasma along with buffy coat. As a further step, thus obtained RBCs were put in isotonic solution at the haematocrit index requested by each protocol.
To determine the % haemolysis, RBCs (35% haematocrit) were treated with or without peel or juice extract in isotonic solution for 15 min at 37°C and then processed according to Remigante and collaborators (Remigante et al, 2022b). Haemoglobin absorbance was determined at 405 nm wavelength after subtracting the absorbance of blank (0.9% v/v NaCl solution).
The ROS levels were evaluated by the cell-permeable indicator 2′, 7′-dichlorofluorescein diacetate (H2DCFDA, D6883, Sigma-Aldrich), according to the manufacturer’s instructions, with slight modifications. Red blood cells were exposed to 100 mM D-Gal for 24 h at 25°C with or without pre-incubation with different concentrations of peel or juice extract. As the positive control, RBCs were incubated with H2O2. ROS formation was determined by a fluorescence microplate reader (Onda Spectrophotometer, UV-21) at excitation and emission wavelengths of 485 nm and 535 nm, respectively, after subtracting the background fluorescence. Results are expressed in %.
TBARS levels were detected as described by Mendanha and co-authors (Mendanha et al, 2012), with minor modifications. Red blood cells were suspended at 20% haematocrit and incubated with or without different concentrations of peel or juice extract. Next, samples were incubated in 100 mM D-Gal-containing solution and then addressed to quantification of TBARS levels obtained by subtracting 20% of the absorbance at 453 nm from that one at 532 nm (Onda Spectrophotometer, UV-21). Finally, the results were reported as µM TBARS levels.
Measurement of total -SH groups was performed according to Aksenov and Markesbery technique (Aksenov and Markesbery, 2001) with minor modifications. Red blood cells, suspended at 35% haematocrit, were incubated with or without peel or juice extract at different concentrations and successively exposed to D-Gal. To obtain a complete oxidation of total -SH groups, the treatment with NEM was used as the positive control. After that, samples were spectrophotometrically read at 412 nm (Onda spectrophotometer, UV-21). Data were normalised to protein content and results reported as μM TNB/mg protein.
Band 3 expression levels were detected according to Straface and collaborators (Straface et al, 2011). The analysis was performed by an Olympus BX51 Microphot fluorescence microscope or by a FACScan flow cytometer (Becton Dickinson, Mountain View, CA, United States) equipped with a 488 nm argon laser on left untreated RBCs or after their exposure to D-Gal, with or without pre-incubation with peel or juice extract. The median values of fluorescence intensity histograms were used to provide a semi-quantitative analysis.
To establish the anion exchange via band 3, SO42− uptake was measured as formerly described (Romano and Passow, 1984; Galtieri et al, 2002; Romano et al, 2002; Morabito et al, 2013; Morabito et al, 2016; Morabito et al, 2018; Morabito et al, 2019a; Morabito et al, 2020a; Remigante et al, 2020; Perrone et al, 2023). Shortly, this procedure allows for the determination of the kinetic of transport and amount of SO42− internalized by RBCs by turbidimetric analysis after precipitation of the cell content with BaCl2. In particular, each sample was addressed to the spectrophotometer (UV-21, Onda Spectrophotometer, Carpi, Modena, Italy, 425 nm wavelength) and the obtained absorbance was successively converted to (SO42−) L cells × 10–2 based on a calibrated standard curve previously established by precipitating known SO42− concentrations. Sulphate concentration was needed to quantify the rate constant of SO42− uptake (min−1). To this end the following equation was used: Ct = C∞ (1 − e−rt) + C0 (Ct, C∞, and C0 indicate the intracellular SO42− concentrations measured at time t, ∞, and 0, respectively, e indicate the Neper number (2.7182818), r indicates the rate constant accounting for the process velocity, t is the time at which the SO42− concentration was measured). The rate constant is useful to monitor the anion exchange process, as it specifically represents the inverse of the time needed to reach ∼63% of total SO42− intracellular concentration. The parameter (SO42−) L cells × 10–2 reported in figures corresponds to the micromolar concentration of SO42− internalized by 5 mL RBCs at 3% haematocrit.
After 15 min pre-incubation with or without peel or juice extract, RBCs (3% haematocrit) were incubated with D-Gal and then centrifuged (Neya 16R, 4°C, 1,200 × g, 5 min) to discard the supernatant and to suspend RBCs in SO42− medium. Similarly to what described for control conditions, the rate constant for SO42− uptake was quantified.
The glycated haemoglobin content (%A1c) was measured as described by Sompong and collaborators (Sompong et al, 2015) with minor modifications. To this end, RBCs pre-exposed or not to peel or juice extract, were incubated with D-Gal and successively addressed to spectrophotometrically analysis (BioPhotometer Plus, Eppendorf, Manchester, United Kingdom, 610 nm wavelength). Finally, A1c levels (%) were determined from a standard curve obtained from known A1c doses.
Superoxide dismutase (SOD) activity was evaluated by a specific assay kit (CS0009, Sigma-Aldrich, Milan, Italy), according to the manufacturer’s instructions, with slight modifications. Red blood cells were treated with D-Gal, with or without pre-incubation with peel or juice extract. As the positive control, cells were incubated with H2O2. Superoxide dismutase activity was determined by reading the absorbance at 450 nm wavelength (Fluostar Omega, BMG Labtech, Ortenberg, Germany) after subtracting the background.
Catalase activity was evaluated by the catalase assay kit (MAK381, Sigma-Aldrich, Milan, Italy), according to the manufacturer’s instructions, with slight modifications. Red blood cells were treated with D-Gal with or without pre-incubation with peel or juice extract. As the positive control, cells were treated with H2O2. Catalase activity was determined by reading the absorbance at 570 nm wavelength (Fluostar Omega, BMG Labtech, Ortenberg, Germany) after subtracting the background.
RBCs were left untreated or treated with D-Gal, with or without pre-incubation with peel or juice extract. Glucose-6-phosphate dehydrogenase (G6PDH) activity was assessed using a commercial G6PDH activity assay kit (Sigma-Aldrich, Milan, Italy), according to the manufacturer’s instructions. The fluorescence intensity is proportional to the G6PDH activity in the samples. Determination of the reaction rate was performed by a plate spectrophotometer (Onda Spectrophotometer, UV-21) to monitor NAPDH rate of production, such molecule absorbs light at 340 nm, over a 30 min time course. Then the obtained reaction rate, presented as %, was normalized to total protein content by spectrophotometrically analysis at 540 nm wavelength needed to detect haemoglobin absorbance.
GSH levels were quantified according to Teti and collaborators (Teti et al, 2005). Blood samples (20% hematocrit), which were left untreated or exposed to D-Gal with or without pre-incubation, were centrifuged (Neya 16R, 4°C, 1,200× g, 5 min) and resuspended in isotonic solution. After treatments, the content of GSH was measured by Cayman’s GSH assay kit using an enzymatic recycling method with glutathione reductase. Sample absorbance was measured at 412 nm (Onda spectrophotometer, UV-21). The amount of GSSG was calculated by the following formula: 1/2 GSSG = GSH total-GSH reduced. Results are expressed as a GSH/GSSG ratio.
All data are expressed as arithmetic means ± standard error of the mean. GraphPad Prism (version 9.0, GraphPad Software, San Diego, CA, United States) and Excel (Version 2021, Microsoft, Redmond, WA, United States) software were used to perform statistical analysis and graphics. One-way analysis of variance (ANOVA) followed by Bonferroni’s multiple comparison or Dunnet’s post-test as appropriate, unless otherwise specified, was used to determine significant differences between mean values. Statistically significant differences between data sets were assumed at p < 0.05; (n) corresponds to the number of independent measurements.
Table 1 reports qualitative and quantitative features of bioactive molecules deriving from bergamot juice and peel samples. In particular, thanks to the RP-HPLC/PDA/MS analyses, 26 bioactive molecules, of which three are phenolic acids, six limonoids and seventeen flavonoids, were identified and quantified. As seen in Table 1, the two sample sources showed the same qualitative profile.
TABLE 1. Concentration (mg Kg−1 ± standard deviation) of bioactive molecules in Femminello bergamot juice and peel. Each sample was analyzed in triplicate.
On the other hand, juice and peels differed from a quantitative point of view. Peel sample was richer in bioactive molecules (16,132.3 mg kg−1) than juice sample (498.1 mg kg−1). For both sources, the most abundant class of bioactive molecules (88%–94% of the total content) was represented by flavonoids. Neohesperidin was the most abundant bioactive compound in these samples.
A series of experiments were conducted with increasing concentrations (1–250 μg/mL) of peel or juice extracts and incubation times (15 min-3 h) to exclude a possible hemolytic and pro-oxidant effect and estimate the antioxidant capacity of the peel or juice extract. Incubation with 1–5 μg/mL of peel or juice extract for 15 min at 37°C failed to induce hemolysis, increase TBARS levels, and reduce SH group content in RBCs (Supplementary Figures S1, S2). As expected, incubation of RBCs with 100 mM D-Gal for 24 h at 25°C led to a substantial increase in ROS and TBARS levels as well as a reduction in -SH group content compared to untreated RBCs (Supplementary Figure S3), which denotes induction of oxidative stress and is consistent our former findings (Remigante et al, 2022c). However, pre-incubation with 1 μg/mL of peel or juice extract for 15 min at 37°C did not significantly reduced ROS levels in D-Gal treated RBCs, while leading to minor effects on TBARS levels and -SH group content (Supplementary Figures S3A–C). Increasing concentration and pre-incubation times revealed a clear antioxidant effect of the peel and juice extracts, as denoted by a significant reduction in ROS and TBARS levels, as well as increase in total SH group content compared to D-Gal treated RBCs (Supplementary Figures S3D–I). Based on these data, we selected the most effective antioxidant concentration as well as the shortest effective time of incubation and pre-treatment with 5 μg/mL of peel or juice extract for 15 min has been chosen to carry out the following experiments.
Reactive oxygen species were detected in RBCs left untreated or, alternatively, exposed to 100 mM D-Gal for 24 h at 25°C with or without pre-exposure to 5 μg/mL peel or juice extract for 15 min at 37°C. Figure 1A displays the intracellular ROS levels at 0 and 24 h after exposure to D-Gal. As seen, 100 mM D-Gal treated samples showed a significant increase of ROS levels compared to left untreated samples. In samples pre-exposed to 5 μg/mL peel or juice extract, the incubation with 100 mM D-Gal failed to significantly increase ROS levels, which remained unchanged when compared to control values (Figure 1A). As expected, ROS levels of RBCs treated with 20 mM H2O2 for 30 min were significantly higher with respect to those of RBCs left untreated (control). In addition, peel or juice extract alone did not significantly alter the intracellular ROS levels (data not shown).
FIGURE 1. Antioxidant capacity estimation of peel and juice extract. human RBCs have been left untreated or exposed to 20 mM H2O2 or 2 mM NEM for 1 h or 100 mM D-Gal for 24 h at 25°C with or without pre-exposure to peel or juice extract for 15 min at 37°C. (A) estimation of ROS levels; (B) estimation of TBARS levels; (C) estimation of total sulfhydryl group content (µM TNB/µg protein). ns, not statistically significant versus control (untreated); **p < 0.01 and ***p < 0.001 versus control, ◦◦◦ p < 0.001 versus 100 mM D-Gal, ANOVA followed by Bonferroni’s multiple comparison post-test (n = 12).
Thiobarbituric-acid-reactive substances (TBARS) levels measured in RBCs are reported in Figure 1B. As expected, TBARS levels after treatment with 20 mM H2O2 for 1 h were significantly higher than those detected in control (left untreated RBCs). Similarly, after 24 h treatment with 100 mM D-Gal, TBARS levels were significantly increased with respect to control. Importantly, in RBCs pre-treated with 5 μg/mL of peel or juice extract and then exposed to 100 mM D-Gal, TBARS levels were significantly lower than those measured in 100 mM D-Gal-treated RBCs. Of note, peel or juice extract alone did not significantly alter TBARS levels (Supplementary Figures S1D, S2D).
Figure 1C shows the total content of sulfhydryl groups in left untreated RBCs or treated with either the oxidizing molecule NEM as positive control (2 mM for 1 h) or d-Gal (100 mM for 24 h) with or without pre-treatment with peel or juice extract (5 μg/mL). As expected, treatment with NEM led to a significant reduction in the content of the sulfhydryl groups compared to untreated RBCs (control). Sulfhydryl groups in - RBCs treated with 100 mM D-Gal were also significantly lower than control. The total content of the sulfhydryl groups in 100 mM D-Gal-treated RBCs was significantly restored in case of pre-treatment with peel or juice extract (5 μg/mL) (Figure 1C). Additionally, peel or juice extract alone did not significantly alter the total content of the sulfhydryl groups (Supplementary Figures S1G, S2G).
The levels of band 3 expression were significantly decreased in human RBCs incubated with 100 mM D-Gal for 24 h with respect to control (Figure 2A). Pre-treatment with 5 μg/mL peel extract partially restored band 3 expression in RBCs treated with 100 Mm D-Gal. Also, band 3 expression was totally restored in RBCs pre-exposed to 5 μg/mL juice extract. Moreover, Band 3 distribution was assessed by immunofluorescence technique. In particular, band 3 was mainly clustered (arrows) in leptocytes after 100 mM D-Gal exposure, with respect to untreated RBCs (Figure 2B). These changes were attenuated by pre-treatment with 5 μg/mL or juice extract. Of note, band 3 expression was altered neither by peel nor by juice extracts given alone (data not shown).
FIGURE 2. Flow cytometry immunofluorescence of band 3 expression. Red blood cells were left untreated or treated with 100 mM D-Gal for 24 h at 25°C, with or without pre-exposure to 5 μg/mL peel or juice extract for 15 min at 37°C. (A) Histograms report median values of fluorescence intensity. (B) Flow cytometry immunofluorescence representative micrographs showing band 3 distribution in left untreated RBCs, treated with 100 D-Gal, or alternatively, pre-incubated with 5 μg/mL peel or juice extract, and then exposed to 100 mM D-Gal. Samples were observed with a ×100 objective. Note the significant morphological changes in 100 mM D-Gal (arrows). ns, not statistically significant versus control (untreated); **p < 0.01 and ***p < 0.001 versus control, ◦◦ p < 0.01 and ◦◦◦ p < 0.001 versus 100 mM D-Gal, ANOVA followed by Bonferroni’s multiple comparison post-test (n = 3).
To evaluate the band 3 activity, the SO42− uptake during the time was determined in RBCs left untreated (control) or treated with 100 mM d-Gal for 24 h at 25°C, with or without pre-exposure to 5 μg/mL peel or juice extract for 15 min at 37°C (Figure 3). In left untreated RBCs, SO42− uptake is seen to progressively increase reaching equilibrium in 16.68 min (rate constant of SO42− uptake = 0.059 ± 0.001 min−1, Table 2). The transport rate constant in RBCs treated with 100 mM d-Gal (0.092 ± 0.001 min−1) was significantly increased with respect to control, thus denoting an accelerated transport kinetics, which was in agreement with former findings (Remigante et al, 2022c). Pre-exposure to 5 μg/mL peel or juice extract significantly reduced the rate constant of SO42− uptake, which did not differ from control in the case of pre-treatment with peel extract. The SO42− amount internalized by RBCs after 45 min of incubation in SO42− medium was not significantly altered by d-Gal with or without peel or juice extract. In DIDS-treated cells, rate constant of SO42− uptake and the SO42− amount internalized were substantially reduced compared to control, consistent with a SO42− transport via band 3 (Table 2). Red blood cells treated with 5 μg/mL peel or juice extract alone showed a time course of SO42−uptake that was not significantly altered compared to control (Supplementary Figure S4).
FIGURE 3. Time course of SO42− uptake. Red blood cells were left untreated (control) or treated with 100 mM D-Gal (24 h, at 25°C) with or without pre-incubation with 5 μg/mL peel or juice extract (15 min at 37°C). Red blood cells were also exposed to a specific inhibitor of band 3 (10 µM DIDS). ns, not statistically significant versus untreated (control); **p < 0.01 and ***p < 0.001 versus control; ◦ p < 0.05 and ◦◦ p < 0.01 versus 100 mM D-Gal, one-way ANOVA followed by Bonferroni’s multiple comparison post hoc test (n = 15).
TABLE 2. Rate constant of SO42− uptake, time needed to reach equilibrium, and SO42− quantity internalized by either RBCs untreated or treated as indicated. Data are presented as means ± S.E.M. from (n) separate experiments. ns not statistically significant versus untreated (control); ***p < 0.001 versus untreated; ° p < 0.01 versus 100 mM D-Gal, as attested by one-way ANOVA followed by Bonferroni’s multiple comparison post hoc test.
Figure 4 displays the % A1c determined in left untreated RBCs or treated with 100 mM d-Gal for 24 h, with or without pre-exposure to 5 μg/mL peel or juice extract for 15 min at 37°C. The data obtained showed that %A1c levels measured after exposure to 100 mM d-Gal were significantly higher than those of RBCs left untreated (control). Instead, in RBCs pre-treated with 5 μg/mL peel or juice extract, a trend towards a decrease in %A1c levels was seen, which was statistically significant although %A1c levels remained elevated compared to control. In Figure 4, A1c levels measured in diabetes patients -used as the positive control-are also reported (Chandalia and Krishnaswamy, 2002). As expected, a significant increase in the A1c levels were measured in RBCs from diabetic patients. Lastly, %A1c content was not significantly altered by peel or juice extract when applied alone (data not shown).
FIGURE 4. Glycated haemoglobin levels (%A1c). Red blood cells were left untreated or treated with 100 mM D-Gal (24 h, at 25°C) with or without pre-incubation with 5 μg/mL peel or juice extract (15 min at 37°C). ns, not statistically significant versus untreated; ***p < 0.001 versus untreated; ◦◦◦ p < 0.01 versus 100 mM D-Gal, one-way ANOVA followed by Bonferroni’s multiple comparison post hoc test (n = 10).
In Figure 5A, the SOD activity was evaluated in RBCs left untreated or treated with D-Gal (100 mM for 24 h at 25°C) with or without pre-exposure to 5 μg/mL peel or juice extract for 15 min at 37°C. In RBCs exposed to D-Gal, the SOD activity was found significantly increased compared to the untreated cells. Conversely, pre-incubation with peel or juice extract resulted in a significant recovery of SOD activity with respect to D-Gal-treated cells. As expected, SOD activity in RBCs treated with 20 mM H2O2 for 30 min at 25°C as the positive control was significantly higher than that of control RBCs. Of note, peel and juice extracts did not significantly alter SOD activity when given alone (data not shown).
FIGURE 5. Effects of D-Gal (100 mM) exposure with or without pre-treatment with peel or juice extract for 15 min in RBCs. (A) SOD activity, (B) CAT activity, and (C) G6PDH activity. ns, not significant versus untreated (control); ***p < 0.001 versus untreated; ◦◦◦p < 0.001 versus 100 mM D-Gal, as determined by one-way ANOVA followed by Bonferroni’s multiple comparison post hoc test (n = 10).
Catalase was assayed in RBCs left untreated or treated with D-Gal (100 mM for 24 h at 25°C), with or without pre-treatment with 5 μg/mL peel or juice extract (15 min at 37°C). D-Gal treatment caused an increased CAT activity compared to control cells, which was consistent with an elevated oxidative stress (Figure 5B). Unlike, the pre-incubation with peel and/or juice extract (5 μg/mL for 15 min at 37°C) showed a CAT activity almost comparable to that of control (Figure 5B). Exposure to 20 mM H2O2 for 30 min (25°C) has been considered as the positive control. As expected, CAT activity in RBCs treated with H2O2 was significantly higher than those of control RBCs. Moreover, the extracts of peel and juice alone did not significantly alter CAT activity (data not shown).
In Figure 5C, G6PDH activity was measured in RBC left untreated or treated with D-Gal (100 mM for 24 at 25°C), with or without pretreatment with 5 μg/mL peel or juice extract (15 min at 37°C). G6PDH activity was severely stimulated by an increased oxidative stress in the D-Gal-treated cells compared to control RBCs. On the contrary, in cells pre-exposed to peel or juice extract, G6PDH activity was brought back to the control levels. As expected, G6PDH activity of RBCs treated with G6PDH positive control supplied by the manufacturer for 30 min at 25°C was significantly higher than that of RBCs left untreated. In addition, both peel and juice extracts did not significantly alter G6PDH activity (data not shown).
Figure 6 displays the GSH/GSSG ratio measured in RBCs treated with 100 mM D-Gal for 24 h at 25°C with or without pre-treatment with 5 μg/mL peel and juice extract for 15 min at 37°C. The GSH/GSSG ratio measured after treatment with D-Gal was significantly lower than that detected in left untreated RBCs. This effect could be associated with an increased GSSG levels and/or decreased GSH levels, both of which are indicative of an increased intracellular oxidative stress. However, pre-incubation with peel and juice extract restored GSH levels. As expected, incubation with 2 mM NEM for 1 h (used as the positive control) led to a significant reduction in the GSH/GSSG content compared to left untreated RBCs. On the contrary, both peel and juice extract alone did not significantly alter the GSH/GSSG content (data not shown).
FIGURE 6. Assessment of the GSH/GSSG ratio measured in RBCs incubated for 24 h at 25°C with D-Gal with or without pre-treatment with peel or juice extract (15 min at 37°C). GSH, reduced glutathione; GSSG, oxidized glutathione. ***p < 0.001 versus left untreated RBCs; ◦◦p < 0.01 versus D-Gal, one-way ANOVA followed by Bonferroni’s multiple comparison post hoc test (n = 7).
Recently, an increasing body of evidence has supported the hypothesis that natural molecules -referred to as antioxidants-may have a protective role in retarding or reversing the course of age-related diseases (Tan et al, 2018; Singh et al, 2020; Lu et al, 2021; Remigante et al, 2022b; Cui et al, 2022). These compounds are able to compete with substrates which are sensitive to oxidation, thus inhibiting or delaying the reactions between reactive species and biological macromolecules (Pisoschi and Pop, 2015). Even though reactive species are involved in several biological processes, their over-production can lead to cell damage and consequently, development of chronic diseases (Costa et al, 2020; Luo et al, 2020; Bertelli et al, 2021; Remigante et al, 2021b; Forman and Zhang, 2021; Zuccolini et al, 2022). Thus, dietary intake of natural antioxidant compounds could work as a boost to the endogenous antioxidant machinery against reactive species and/or free radicals, thus playing an important role in the prevention of pathological states (Pingitore et al, 2015; Gantenbein and Kanaka-Gantenbein, 2021). Herein, the composition in bioactive molecules of bergamot peel and juice (Table 1) and their effects on oxidative-stress induced aging were described in a cell-based model represented by human RBCs subjected to prolonged (24 h) exposure to 100 mM D-Gal. This cell-based model, which was validated in our former studies (Remigante et al, 2022c; Remigante et al, 2022d; Spinelli et al, 2023), recapitulates the cellular and molecular mechanisms of natural aging, i.e., oxidative stress and haemoglobin glycation.
Though various investigations described the numerous activities of bioactive compounds of bergamot extracts, their effects on aging RBCs have not yet been fully analysed. Then, the first step of the present investigation was to test a broad range of concentrations (from 1 μg/mL to 250 μg/mL) of bergamot peel and juice extract, as well as increasing incubation time intervals (15 min, 1 and 3 h), to exclude any damage in terms of haemolysis, lipid peroxidation and protein oxidation that could potentially be caused by direct exposure of RBCs to peel or juice extracts (Supplementary Figures S1, S2). Indeed, concentrations of peel or juice extract equal to or greater than 10 μg/mL could induce haemolysis and exhibited a pro-oxidant effect, especially following prolonged incubation times. This is not surprising, given that various antioxidants may act as pro-oxidants depending on their concentration (Osseni et al, 2000; Sahebkar, 2015; Giordano et al, 2020). These and our findings point to the importance of carefully assessing concentration and incubation time when novel potential antioxidant compounds are tested in cell-based assays. Based on these considerations, we selected a 15 min pre-treatment with 5 μg/mL peel or juice extract in order to estimate the antioxidant capacity by measuring ROS and TBARS levels, and total content of sulfhydryl groups in RBCs incubated for 24 h with 100 mM D-Gal (Figure 1).
Red blood cells are susceptible to ROS-induced damage because of their high polyunsaturated fatty acid content and their abundance in iron (Fe2+)-rich haemoglobin. This latter acts as a catalyst in redox reactions and lipid peroxidation, resulting in TBARS production as the final product (Pandey and Rizvi, 2010). Also, RBCs often undergo membrane protein oxidation and/or carbonylation. Therefore, the oxidation of protein sulfhydryl groups (-SH) and/or the formation of carbonyl groups are indicators of oxidative injury to proteins in human RBCs. Since ROS generated during cellular metabolism cause the oxidation of biological macromolecules, the effect of peel and juice extract on the intracellular ROS levels has been evaluated as a first step of the investigation. A 15 min pre-exposure of RBCs to 5 μg/mL peel or juice extract could effectively prevent the ROS production caused by exposure to D-Gal (Figure 1A).
To better elucidate the process of oxidation of plasma membrane macromolecules, the estimation of both TBARS level and sulfhydryl group content of total proteins, which in RBCs mainly belong to band 3 protein (Rao and Reithmeier, 1979), have been investigated. Our findings show that pre-exposure (15 min) to 5 μg/mL peel or juice extract completely prevented TBARS levels increase in RBCs treated with 100 D-Gal (Figure 1B) and could at least partially (peel) or completely (juice) restore sulfhydryl group content (Figure 1C). These findings denote that peel or juice extract could effectively protect both the lipid and protein component of the RBC plasma membrane from oxidation. Data related to oxidative stress assessment are in line with what previously showed by other researchers and suggest that polyphenols and phytochemicals could play scavenger activity by directly neutralizing reactive species and free radicals and avoid their detrimental impact on biological macromolecules (Zhao et al, 2006; Kelen and Tepe, 2007; Luo et al, 2021; Kruk et al, 2022).
One of the most interesting and still unknown implications of oxidative stress-related aging is its impact on plasma membrane transport systems. In this regard, several investigations demonstrated that the aging process may impact the anion (Cl−/HCO3−) exchange, mediated by band 3, on the RBC plasma membrane. The structure of this protein consists of two rather different domains of a similar size (Arakawa et al, 2015). The C-terminal domain carries out Cl−/HCO3− exchange across the plasma membrane (Reithmeier et al, 2016; Remigante et al, 2022e). This function can be monitored by the rate constant for sulphate (SO42−) uptake (Morabito et al, 2016; Morabito et al, 2017a; Morabito et al, 2017b), which is slower and more easily detectable than Cl− or HCO3− uptake (Jennings, 1976; Romano and Passow, 1984; Morabito et al, 2019b; Crupi et al, 2019). SO42− uptake measurement has been firmly confirmed as an efficient tool to investigate the impact of redox conditions on RBCs homeostasis (Morabito et al, 2016; Morabito et al, 2019b; Remigante et al, 2019). Hence, the SO42− uptake through band 3 was measured in RBCs after treatment with D-Gal (100 mM) with or without pre-exposure (15 min) to 5 μg/mL of bergamot peel or juice extract. In RBCs treated with D-Gal (100 mM), the rate constant for SO42− uptake was accelerated compared to the control (Figure 3; Table 2). The evidence that oxidative stress provokes functional modifications of band 3 in human RBCs has been provided also in other cell-based models of oxidative stress. In particular, acute H2O2-induced oxidative stress provoked a reduction in the rate constant for SO42− uptake (Morabito et al, 2016; Morabito et al, 2017b), whereas in an oxidative stress model induced by high glucose concentrations an accelerate rate of ì anion exchanging has been seen (Morabito et al, 2020b). Thus, a possible a two-faced effect on the velocity of anion exchange could depend on specific cell component (lipids, proteins, as well as enzymes) affected by both the stressors and the related pathways. In this context, although no functional alteration was reported in RBCs treated exclusively with 5 μg/mL peel or juice extract (Supplementary Figure S4), a 15 min pre-treatment of RBCs formerly exposed to D-Gal partially or totally recovered the rate constant for SO42− uptake (Figure 3; Table 2). Based on data hitherto obtained, we can point out that both extracts show a similar protective effect on anionic exchange and could, therefore, act a key role in counteracting oxidative stress–induced functional changes in human RBCs.
The binding sites for cytoskeletal and cytoplasmic proteins, including haemoglobin, are located on the N-terminal cytoplasmic domain of band 3 (Anong et al, 2009; Wu et al, 2011). Haemoglobin glycation represents a case of non-enzymatic protein glycation associated with production of AGEs (Luevano-Contreras and Chapman-Novakofski, 2010). To better explore the molecular interaction between band 3 and haemoglobin, levels of both proteins were evaluated. The data obtained show that 100 mM D-Gal treatment caused both a loss and a redistribution of band 3, most probably due to the shedding of protein-containing vesicles (Kuo et al, 2017) (Figures 2A, B). Yet, despite this, band 3 expression levels were partially or totally restored in RBCs pre-treated with 5 μg/mL peel or juice extract, respectively (Figures 2A, B). The shedding of membrane area is a critical point for cell fate, since a reduced surface-to-volume ratio is thought to correlate with the early phagocytosis of aged RBCs (Kuo et al, 2017). Consequently to membrane shedding, the cytoskeleton reduces to a 3- to 5-fold smaller area (Li and Lykotrafitis, 2015). The proteins affected are mainly band 3, ankyrin, spectrin, and occasionally protein 4.2 and the Rh protein. These proteins are all part of one of the complexes by which the cytoskeleton is anchored to the lipid bilayer (Gardel et al, 2008; Anong et al, 2009; Vallese et al, 2022). Presumably, damage of these proteins is responsible for the impaired cellular deformability associated with oxidative stress (Mohanty et al, 2014). A decrease in RBC deformability leads to impaired microcirculation and tissue oxygenation (Schwartz et al, 1991; Silva-Herdade et al, 2016). For example, Spinelli and co-authors have shown that both band 3 phosphorylation and rearrangements in cytoskeleton proteins can lead to cell deformation and structural alteration of human RBCs during the aging processes (Spinelli et al, 2023).
As mentioned above, haemoglobin is anchored to band 3 cytoplasmic domain. In this regard, our findings indicated that exposure of RBCs to 100 mM D-Gal for 24 h increased the content of A1c levels (Figure 4), contributing to both biochemical and structural changes, including clustering of band 3 regions. At a more advanced stage of aging, such clusters could represent a recognition site for antibodies directed against aged RBCs, thus triggering the early removal of RBCs from the blood circulation (Briglia et al, 2017). Importantly, in RBCs pre-treated with 5 μg/mL peel or juice extract, a clear trend towards a decrease in %A1c production was seen (Figure 4). Collectively, these findings (Figures 2, 4) suggest that the active biomolecules of the bergamot juice or peel extract might efficiently prevent RBCs membrane shedding, formation of glycated haemoglobin, and RBCs structural instability, all of which are hallmarks of aging.
At last, we investigated the activity of endogenous antioxidant enzymes CAT and SOD (Figures 5A, B). These antioxidant enzymes own outstanding free radical scavenging capacities and play vital roles in human RBCs (Ulanczyk et al, 2020). The SOD and CAT activity in RBCs incubated with 100 mM D-Gal was much higher than in control cells, which could reflect the activation of the endogenous antioxidant defense system to suppress the formation of free radicals (Figure 1A). Nevertheless, the increase in SOD and CAT activity failed to compensate for the increase in free radicals (Figure 1A), as also demonstrated by the increase in lipid peroxidation levels as well as total protein oxidation (Figures 1B, C). Upregulation in CAT and SOD activity with concomitant substantial elevation of oxidative stress markers might reflect exhaustion of the endogenous antioxidant system. These biochemical changes may provoke injury to the membrane proteins and lipid structure. As a result, the membrane mechanical properties could be modified, resulting in deformability and fluidity reduction or altered permeability of the phospholipid bilayer, which, in turn, reduce the ability of the membrane to withstand osmotic changes (Spinelli et al, 2023). In this context, pre-exposure of RBCs to peel and juice extracts could significantly prevent the upregulation in both SOD and CAT activity observed in D-Gal-treated cells (Figures 5A, B). These findings denote that the active biomolecules of the bergamot juice or peel extract might act synergistically with endogenous antioxidant system to counteract oxidative stress in RBCs and preserve cell integrity.
RBCs are unable to generate ATP using molecular oxygen because of lack of mitochondria. Glycolysis is the only source of ATP generation in mature RBCs. It has been found that under normal conditions about 90% of total imported glucose is used to generate ATP through glycolysis and the remaining 10% of glucose is addressed to PPP (Reisz et al, 2016). Glucose-6-phosphate dehydrogenase (G6PDH) is a crucial enzyme of this latter pathway, which produces reduced NADPH and represents the most important defense mechanism for RBCs in case of excessive oxidant generation, or of ineffective antioxidant defense (Maha, 2009). In these conditions, glycolysis and its key enzyme GAPDH are inhibited (Reisz et al, 2016). In addition to its importance in the RBC as a major metabolic determinant during oxidative stress, G6PDH activity is also employed as an indication of cell aging (Subasinghe and Spence, 2008). The G6PDH activity was severely stimulated - presumably by an increased oxidative stress - in D-Gal-treated cells compared to control RBC cells (Figure 5C), which was consistent with inhibition of glycolysis and activation of the PPP shunt. Vice versa, in cells pre-exposed to peel and/or juice extract the G6PDH activity was brought back to the control levels (Figure 5C). In addition, as already mentioned, the underlying mechanisms of aging can severely alter enzyme activities, including glutathione (Maher, 2005). Glutathione is an important endogenous non-enzymatic antioxidant that neutralizes ROS production (Ahmad and Mahmood, 2019). Its depletion makes cells more prone to oxidative damage. Then, the GSH/GSSG ratio was assayed. The obtained results confirmed that 100 mM D-Gal treatment reduced the GSH/GSSG ratio. However, the pre-incubation of RBCs with peel and juice extract partially restored the redox balance (Figure 6). In summary, our findings suggest that the active biomolecules of the bergamot juice or peel extract might prevent metabolic alterations in RBCs exposed to oxidative stress.
Here we characterize the precise composition in bioactive compounds of the bergamot (Citrus bergamia, Femminello cultivar) peel and juice extracts and we assess their protective effect in an oxidative stress-related model of cellular aging in RBCs. The data presented here indicate, for the first time, that polyphenol-rich extracts of peel and juice from bergamot fruit may act on oxidative stress-induced alterations of the lipid and protein cellular components, including the endogenous antioxidant system as well as proteins with ion transport activity and enzymatic metabolic activity, thus protecting the structural and functional integrity of human RBCs. This study identifies bergamot as an antioxidant functional food and further suggests that diet supplementation with bergamot or its derivatives might contribute to the prevention or attenuation of pathophysiological events linked to RBCs dysfunction during aging.
The raw data supporting the conclusion of this article will be made available by the authors, without undue reservation.
The studies involving human participants were reviewed and approved by the study was conducted in accordance with the Declaration of Helsinki and approved by the Institutional Review Board (or Ethics Committee) of University of Messina (prot.52-22). The patients/participants provided their written informed consent to participate in this study.
AR and RM conceived and designed the research; SS, MR, GC, ES, LG, DC, GF, and PD performed the experiments and analyzed the data; AR and RM interpreted the results of the experiments; SS and AR prepared the figures; AR drafted the manuscript; AM, AR, and SD edited and revised the manuscript. All authors contributed to the article and approved the submitted version.
The authors thank 1) Azienda Agricola Cilea Rosaria for kindly providing us bergamot fruits, 2) Shimadzu and Merck Life Science corporations for the continuous support, 3) Professor Caterina Faggio (from University of Messina) for helpful discussion, and iv) Dr. Federica De Gaetano (from University of Messina) for technical assistance.
The authors declare that the research was conducted in the absence of any commercial or financial relationships that could be construed as a potential conflict of interest.
All claims expressed in this article are solely those of the authors and do not necessarily represent those of their affiliated organizations, or those of the publisher, the editors and the reviewers. Any product that may be evaluated in this article, or claim that may be made by its manufacturer, is not guaranteed or endorsed by the publisher.
The Supplementary Material for this article can be found online at: https://www.frontiersin.org/articles/10.3389/fphys.2023.1225552/full#supplementary-material
Abbas, Y. M., Toye, A. M., Rubinstein, J. L., and Reithmeier, R. A. F. (2018). Band 3 function and dysfunction in a structural context. Curr. Opin. Hematol. 25, 163–170. doi:10.1097/MOH.0000000000000418
Ahmad, S., and Mahmood, R. (2019). Mercury chloride toxicity in human erythrocytes: Enhanced generation of ROS and RNS, hemoglobin oxidation, impaired antioxidant power, and inhibition of plasma membrane redox system. Environ. Sci. Pollut. Res. Int. 26, 5645–5657. doi:10.1007/s11356-018-04062-5
Akki, R., Siracusa, R., Cordaro, M., Remigante, A., Morabito, R., Errami, M., et al. (2019). Adaptation to oxidative stress at cellular and tissue level. Arch. Physiol. Biochem. 128, 521–531. doi:10.1080/13813455.2019.1702059
Akki, R., Siracusa, R., Morabito, R., Remigante, A., Campolo, M., Errami, M., et al. (2018). Neuronal-like differentiated SH-SY5Y cells adaptation to a mild and transient H2 O2 -induced oxidative stress. Cell Biochem. Funct. 36, 56–64. doi:10.1002/cbf.3317
Aksenov, M. Y., and Markesbery, W. R. (2001). Changes in thiol content and expression of glutathione redox system genes in the hippocampus and cerebellum in Alzheimer's disease. Neurosci. Lett. 302, 141–145. doi:10.1016/s0304-3940(01)01636-6
Anong, W. A., Franco, T., Chu, H., Weis, T. L., Devlin, E. E., Bodine, D. M., et al. (2009). Adducin forms a bridge between the erythrocyte membrane and its cytoskeleton and regulates membrane cohesion. Blood 114, 1904–1912. doi:10.1182/blood-2009-02-203216
Arakawa, T., Kobayashi-Yurugi, T., Alguel, Y., Iwanari, H., Hatae, H., Iwata, M., et al. (2015). Crystal structure of the anion exchanger domain of human erythrocyte band 3. Science 350, 680–684. doi:10.1126/science.aaa4335
Arrigo, F., Impellitteri, F., Piccione, G., and Faggio, C. (2023). Phthalates and their effects on human health: Focus on erythrocytes and the reproductive system. Comp. Biochem. Physiol. C Toxicol. Pharmacol. 270, 109645. doi:10.1016/j.cbpc.2023.109645
Avitabile, E., Senes, N., D'Avino, C., Tsamesidis, I., Pinna, A., Medici, S., et al. (2020). The potential antimalarial efficacy of hemocompatible silver nanoparticles from Artemisia species against P. falciparum parasite. PLoS One 15, e0238532. doi:10.1371/journal.pone.0238532
Azman, K. F., and Zakaria, R. (2019). D-Galactose-induced accelerated aging model: An overview. Biogerontology 20, 763–782. doi:10.1007/s10522-019-09837-y
Bertelli, S., Remigante, A., Zuccolini, P., Barbieri, R., Ferrera, L., Picco, C., et al. (2021). Mechanisms of activation of LRRC8 volume regulated anion channels. Cell Physiol. Biochem. 55, 41–56. doi:10.33594/000000329
Bo-Htay, C., Palee, S., Apaijai, N., Chattipakorn, S. C., and Chattipakorn, N. (2018). Effects of d-galactose-induced ageing on the heart and its potential interventions. J. Cell Mol. Med. 22, 1392–1410. doi:10.1111/jcmm.13472
Briglia, M., Rossi, M. A., Faggio, C., and Eryptosis, C. (2017). Eryptosis: Ally or enemy. Curr. Med. Chem. 24, 937–942. doi:10.2174/0929867324666161118142425
Campanella, M. E., Chu, H., Wandersee, N. J., Peters, L. L., Mohandas, N., Gilligan, D. M., et al. (2008). Characterization of glycolytic enzyme interactions with murine erythrocyte membranes in wild-type and membrane protein knockout mice. Blood 112, 3900–3906. doi:10.1182/blood-2008-03-146159
Carresi, C., Gliozzi, M., Giancotta, C., Scarcella, A., Scarano, F., Bosco, F., et al. (2016). Studies on the protective role of Bergamot polyphenols in doxorubicin-induced cardiotoxicity. PharmaNutrition 4, S19–S26. doi:10.1016/j.phanu.2015.11.005
Castagnola, M., Messana, I., Sanna, M. T., and Giardina, B. (2010). Oxygen-linked modulation of erythrocyte metabolism: State of the art. Blood Transfus. 8, s53–s58. Suppl 3. doi:10.2450/2010.009S
Costa, R., Remigante, A., Civello, D. A., Bernardinelli, E., Szabo, Z., Morabito, R., et al. (2020). O-GlcNAcylation suppresses the ion current IClswell by preventing the binding of the protein ICln to alpha-integrin. Front. Cell Dev. Biol. 8, 607080. doi:10.3389/fcell.2020.607080
Crupi, R., Morabito, R., Remigante, A., Gugliandolo, E., Britti, D., Cuzzocrea, S., et al. (2019). Susceptibility of erythrocytes from different sources to xenobiotics-induced lysis. Comp. Biochem. Physiol. C Toxicol. Pharmacol. 221, 68–72. doi:10.1016/j.cbpc.2019.03.008
Cui, Z., Zhao, X., Amevor, F. K., Du, X., Wang, Y., Li, D., et al. (2022). Therapeutic application of quercetin in aging-related diseases: SIRT1 as a potential mechanism. Front. Immunol. 13, 943321. doi:10.3389/fimmu.2022.943321
De Franceschi, L., Scardoni, G., Tomelleri, C., Danek, A., Walker, R. H., Jung, H. H., et al. (2012). Computational identification of phospho-tyrosine sub-networks related to acanthocyte generation in neuroacanthocytosis. PLoS One 7, e31015. doi:10.1371/journal.pone.0031015
Dorta, D. J., Pigoso, A. A., Mingatto, F. E., Rodrigues, T., Pestana, C. R., Uyemura, S. A., et al. (2008). Antioxidant activity of flavonoids in isolated mitochondria. Phytother. Res. 22, 1213–1218. doi:10.1002/ptr.2441
Ellsworth, M. L. (2000). The red blood cell as an oxygen sensor: What is the evidence? Acta Physiol. Scand. 168, 551–559. doi:10.1046/j.1365-201x.2000.00708.x
Emanuelli, M., Sartini, D., Molinelli, E., Campagna, R., Pozzi, V., Salvolini, E., et al. (2022). The double-edged sword of oxidative stress in skin damage and melanoma: From physiopathology to therapeutical approaches. Antioxidants (Basel) 11, 612. doi:10.3390/antiox11040612
Ferrera, L., Barbieri, R., Picco, C., Zuccolini, P., Remigante, A., Bertelli, S., et al. (2021). TRPM2 oxidation activates two distinct potassium channels in melanoma cells through intracellular calcium increase. Int. J. Mol. Sci. 22, 8359. doi:10.3390/ijms22168359
Ferlazzo, N., Visalli, G., Smeriglio, A., Cirmi, S., Lombardo, G. E., Campiglia, P., et al. (2015). Flavonoid fraction of orange and bergamot juices protect human lung epithelial cells from hydrogen peroxide-induced oxidative stress. Evidence-Based Complementary Altern. Med. 2015, 1–14. doi:10.1155/2015/957031
Forman, H. J., and Zhang, H. (2021). Targeting oxidative stress in disease: Promise and limitations of antioxidant therapy. Nat. Rev. Drug Discov. 20, 689–709. doi:10.1038/s41573-021-00233-1
Fujii, J., Homma, T., Kobayashi, S., Warang, P., Madkaikar, M., and Mukherjee, M. B. (2021). Erythrocytes as a preferential target of oxidative stress in blood. Free Radic. Res. 55, 781–799. doi:10.1080/10715762.2021.1873318
Fukagawa, N. K. (1999). Aging: Is oxidative stress a marker or is it causal? Proc. Soc. Exp. Biol. Med. 222, 293–298. doi:10.1046/j.1525-1373.1999.d01-146.x
Galtieri, A., Tellone, E., Romano, L., Misiti, F., Bellocco, E., Ficarra, S., et al. (2002). Band-3 protein function in human erythrocytes: Effect of oxygenation-deoxygenation. Biochim. Biophys. Acta 1564, 214–218. doi:10.1016/s0005-2736(02)00454-6
Gantenbein, K. V., and Kanaka-Gantenbein, C. (2021). Mediterranean diet as an antioxidant: The impact on metabolic health and overall wellbeing. Nutrients 13, 1951. doi:10.3390/nu13061951
Gardel, M. L., Kasza, K. E., Brangwynne, C. P., Liu, J., and Weitz, D. A. (2008). Chapter 19: Mechanical response of cytoskeletal networks. Methods Cell Biol. 89, 487–519. doi:10.1016/S0091-679X(08)00619-5
Giordano, M. E., Caricato, R., and Lionetto, M. G. (2020). Concentration dependence of the antioxidant and prooxidant activity of trolox in HeLa cells: Involvement in the induction of apoptotic volume decrease. Antioxidants (Basel) 9, 1058. doi:10.3390/antiox9111058
Group, E. W. (1998). The fitness for purpose of analytical methods: A laboratory guide to method validation and related topics. Available at: http://www.eurachem.org/guides/pdf/valid.pdf 1998.
Guo, J., Huang, X., Dou, L., Yan, M., Shen, T., Tang, W., et al. (2022). Aging and aging-related diseases: From molecular mechanisms to interventions and treatments. Signal Transduct. Target Ther. 7, 391. doi:10.1038/s41392-022-01251-0
Harman, D. (1972). Free radical theory of aging: Dietary implications. Am. J. Clin. Nutr. 25, 839–843. doi:10.1093/ajcn/25.8.839
Inal, M. E., Kanbak, G., and Sunal, E. (2001). Antioxidant enzyme activities and malondialdehyde levels related to aging. Clin. Chim. Acta 305, 75–80. doi:10.1016/s0009-8981(00)00422-8
Issaian, A., Hay, A., Dzieciatkowska, M., Roberti, D., Perrotta, S., Darula, Z., et al. (2021). The interactome of the N-terminus of band 3 regulates red blood cell metabolism and storage quality. Haematologica 106, 2971–2985. doi:10.3324/haematol.2020.278252
Jacob, R. A. (1995). The integrated antioxidant system. Nutr. Res. 15, 755–766. doi:10.1016/0271-5317(95)00041-g
Jennings, M. L. (1976). Proton fluxes associated with erythrocyte membrane anion exchange. J. Membr. Biol. 28, 187–205. doi:10.1007/bf01869697
Junqueira, V. B., Barros, S. B., Chan, S. S., Rodrigues, L., Giavarotti, L., Abud, R. L., et al. (2004). Aging and oxidative stress. Mol. Asp. Med. 25, 5–16. doi:10.1016/j.mam.2004.02.003
Kelen, M., and Tepe, B. (2007). Screening of antioxidative properties and total phenolic compounds of various extracts of three different seed of grape varieties (Vitis vinifera L) from Turkish flora. Pak J. Biol. Sci. 10, 403–408. doi:10.3923/pjbs.2007.403.408
Kouka, P., Priftis, A., Stagos, D., Angelis, A., Stathopoulos, P., Xinos, N., et al. (2017). Assessment of the antioxidant activity of an olive oil total polyphenolic fraction and hydroxytyrosol from a Greek Olea europea variety in endothelial cells and myoblasts. Int. J. Mol. Med. 40, 703–712. doi:10.3892/ijmm.2017.3078
Kruk, J., Aboul-Enein, B. H., Duchnik, E., and Marchlewicz, M. (2022). Antioxidative properties of phenolic compounds and their effect on oxidative stress induced by severe physical exercise. J. Physiol. Sci. 72, 19. doi:10.1186/s12576-022-00845-1
Kuhn, V., Diederich, L., Keller IV, T. S., Kramer, C. M., Lückstädt, W., Panknin, C., et al. (2017). Red blood cell function and dysfunction: Redox regulation, nitric oxide metabolism, anemia. Antioxidants redox Signal. 26, 718–742. doi:10.1089/ars.2016.6954
Kuo, W. P., Tigges, J. C., Toxavidis, V., and Ghiran, I. (2017). Red blood cells: A source of extracellular vesicles. Methods Mol. Biol. 1660, 15–22. doi:10.1007/978-1-4939-7253-1_2
Lauro, F., Giancotti, L. A., Ilari, S., Dagostino, C., Gliozzi, M., Morabito, C., et al. (2016). Inhibition of spinal oxidative stress by bergamot polyphenolic fraction attenuates the development of morphine induced tolerance and hyperalgesia in mice. PLoS One 11, e0156039. doi:10.1371/journal.pone.0156039
Li, H., and Lykotrafitis, G. (2015). Vesiculation of healthy and defective red blood cells. Phys. Rev. E Stat. Nonlin Soft Matter Phys. 92, 012715. doi:10.1103/PhysRevE.92.012715
Lu, W., Shi, Y., Wang, R., Su, D., Tang, M., Liu, Y., et al. (2021). Antioxidant activity and healthy benefits of natural pigments in fruits: A review. Int. J. Mol. Sci. 22, 4945. doi:10.3390/ijms22094945
Luevano-Contreras, C., and Chapman-Novakofski, K. (2010). Dietary advanced glycation end products and aging. Nutrients 2, 1247–1265. doi:10.3390/nu2121247
Lunceford, N., and Gugliucci, A. (2005). Ilex paraguariensis extracts inhibit AGE formation more efficiently than green tea. Fitoterapia 76, 419–427. doi:10.1016/j.fitote.2005.03.021
Luo, J., Mills, K., le Cessie, S., Noordam, R., and van Heemst, D. (2020). Ageing, age-related diseases and oxidative stress: What to do next? Ageing Res. Rev. 57, 100982. doi:10.1016/j.arr.2019.100982
Luo, J., Si, H., Jia, Z., and Liu, D. (2021). Dietary anti-aging polyphenols and potential mechanisms. Antioxidants (Basel) 10, 283. doi:10.3390/antiox10020283
Maher, P. (2005). The effects of stress and aging on glutathione metabolism. Ageing Res. Rev. 4, 288–314. doi:10.1016/j.arr.2005.02.005
Maha, A. A. (2009). Effect of glucose-6-phosphate dehydrogenase deficiency on some biophysical properties of human erythrocytes. Hematology 14, 38–45. doi:10.1179/102453309X385061
Martinelli, I., Tomassoni, D., Moruzzi, M., Roy, P., Cifani, C., Amenta, F., et al. (2020). Cardiovascular changes related to metabolic syndrome: Evidence in obese zucker rats. Int. J. Mol. Sci. 21, 2035. doi:10.3390/ijms21062035
Mendanha, S. A., Anjos, J. L., Silva, A. H., and Alonso, A. (2012). Electron paramagnetic resonance study of lipid and protein membrane components of erythrocytes oxidized with hydrogen peroxide. Braz J. Med. Biol. Res. 45, 473–481. doi:10.1590/s0100-879x2012007500050
Mohanty, J. G., Nagababu, E., and Rifkind, J. M. (2014). Red blood cell oxidative stress impairs oxygen delivery and induces red blood cell aging. Front. Physiol. 5, 84. doi:10.3389/fphys.2014.00084
Moldogazieva, N. T., Mokhosoev, I. M., Mel'nikova, T. I., Porozov, Y. B., and Terentiev, A. A. (2019). Oxidative stress and advanced lipoxidation and glycation end products (ALEs and AGEs) in aging and age-related diseases. Oxid. Med. Cell Longev. 2019, 3085756. doi:10.1155/2019/3085756
Morabito, R., Marino, A., Romano, P., Rigano, C., and La Spada, G. (2013). Sulphate and chloride-dependent potassium transport in human erythrocytes are affected by crude venom from nematocysts of the jellyfish pelagia noctiluca. Cell Physiol. Biochem. 32, 86–95. doi:10.1159/000356630
Morabito, R. R., Arcuri, B., Marino, A., Giammanco, M., La Spada, G. M., et al. (2018). Effect of cadmium on anion exchange capability through Band 3 protein in human erythrocytes. J. Biol. Res. 91, 1–7. doi:10.4081/jbr.2018.7203
Morabito, R., Remigante, A., and Marino, A. (2019b). Melatonin protects band 3 protein in human erythrocytes against H2O2-induced oxidative stress. Molecules 24, 2741. doi:10.3390/molecules24152741
Morabito, R., Remigante, A., Cavallaro, M., Taormina, A., La Spada, G., and Marino, A. (2017a). Anion exchange through band 3 protein in canine leishmaniasis at different stages of disease. Pflugers Arch. 469, 713–724. doi:10.1007/s00424-017-1974-2
Morabito, R., Remigante, A., Cordaro, M., Trichilo, V., Loddo, S., Dossena, S., et al. (2020a). Impact of acute inflammation on Band 3 protein anion exchange capability in human erythrocytes. Arch. Physiol. Biochem. 128, 1242–1248. doi:10.1080/13813455.2020.1764048
Morabito, R., Remigante, A., Di Pietro, M. L., Giannetto, A., La Spada, G., and Marino, A. (2017b). SO4(=) uptake and catalase role in preconditioning after H2O2-induced oxidative stress in human erythrocytes. Pflugers Arch. 469, 235–250. doi:10.1007/s00424-016-1927-1
Morabito, R., Remigante, A., and Marino, A. (2019a). Protective role of magnesium against oxidative stress on SO(4)(=) uptake through band 3 protein in human erythrocytes. Cell Physiol. Biochem. 52, 1292–1308. doi:10.33594/000000091
Morabito, R., Remigante, A., Spinelli, S., Vitale, G., Trichilo, V., Loddo, S., et al. (2020b). High glucose concentrations affect band 3 protein in human erythrocytes. Antioxidants (Basel) 9, 365. doi:10.3390/antiox9050365
Morabito, R., Romano, O., La Spada, G., and Marino, A. (2016). H2O2-Induced oxidative stress affects SO4= transport in human erythrocytes. PLoS One 11, e0146485. doi:10.1371/journal.pone.0146485
Musolino, V., Gliozzi, M., Nucera, S., Carresi, C., Maiuolo, J., Mollace, R., et al. (2019). The effect of bergamot polyphenolic fraction on lipid transfer protein system and vascular oxidative stress in a rat model of hyperlipemia. Lipids Health Dis. 18, 115–118. doi:10.1186/s12944-019-1061-0
Osseni, R. A., Rat, P., Bogdan, A., Warnet, J. M., and Touitou, Y. (2000). Evidence of prooxidant and antioxidant action of melatonin on human liver cell line HepG2. Life Sci. 68, 387–399. doi:10.1016/s0024-3205(00)00955-3
Pandey, K. B., and Rizvi, S. I. (2011). Biomarkers of oxidative stress in red blood cells. Biomed. Pap. Med. Fac. Univ. Palacky. Olomouc Czech Repub. 155, 131–136. doi:10.5507/bp.2011.027
Pandey, K. B., and Rizvi, S. I. (2010). Markers of oxidative stress in erythrocytes and plasma during aging in humans. Oxid. Med. Cell Longev. 3, 2–12. doi:10.4161/oxim.3.1.10476
Perrone, P., Spinelli, S., Mantegna, G., Notariale, R., Straface, E., Caruso, D., et al. (2023). Mercury chloride affects band 3 protein-mediated anionic transport in red blood cells: Role of oxidative stress and protective effect of olive oil polyphenols. Cells 12, 424. doi:10.3390/cells12030424
Pingitore, A., Lima, G. P., Mastorci, F., Quinones, A., Iervasi, G., and Vassalle, C. (2015). Exercise and oxidative stress: Potential effects of antioxidant dietary strategies in sports. Nutrition 31, 916–922. doi:10.1016/j.nut.2015.02.005
Pisoschi, A. M., and Pop, A. (2015). The role of antioxidants in the chemistry of oxidative stress: A review. Eur. J. Med. Chem. 97, 55–74. doi:10.1016/j.ejmech.2015.04.040
Pretorius, E. (2018). Erythrocyte deformability and eryptosis during inflammation, and impaired blood rheology. Clin. Hemorheol. Microcirc. 69, 545–550. doi:10.3233/CH-189205
Puchulu-Campanella, E., Chu, H., Anstee, D. J., Galan, J. A., Tao, W. A., and Low, P. S. (2013). Identification of the components of a glycolytic enzyme metabolon on the human red blood cell membrane. J. Biol. Chem. 288, 848–858. doi:10.1074/jbc.M112.428573
Radi, E., Formichi, P., Battisti, C., and Federico, A. (2014). Apoptosis and oxidative stress in neurodegenerative diseases. J. Alzheimers Dis. 42, S125–S152. Suppl 3. doi:10.3233/JAD-132738
Rao, A., and Reithmeier, R. (1979). Reactive sulfhydryl groups of the band 3 polypeptide from human erythroycte membranes. Location in the primary structure. J. Biol. Chem. 254, 6144–6150. doi:10.1016/s0021-9258(18)50530-5
Reisz, J. A., Wither, M. J., Dzieciatkowska, M., Nemkov, T., Issaian, A., Yoshida, T., et al. (2016). Oxidative modifications of glyceraldehyde 3-phosphate dehydrogenase regulate metabolic reprogramming of stored red blood cells. Blood 128, e32–e42. doi:10.1182/blood-2016-05-714816
Reithmeier, R. A., Casey, J. R., Kalli, A. C., Sansom, M. S., Alguel, Y., and Iwata, S. (2016). Band 3, the human red cell chloride/bicarbonate anion exchanger (AE1, SLC4A1), in a structural context. Biochim. Biophys. Acta 1858, 1507–1532. doi:10.1016/j.bbamem.2016.03.030
Remigante, A., Morabito, R., and Marino, A. (2021a). Band 3 protein function and oxidative stress in erythrocytes. J. Cell Physiol. 236, 6225–6234. doi:10.1002/jcp.30322
Remigante, A., and Morabito, R. (2022). Cellular and molecular mechanisms in oxidative stress-related diseases. Int. J. Mol. Sci. 23, 8017. doi:10.3390/ijms23148017
Remigante, A., Morabito, R., and Marino, A. (2019). Natural antioxidants beneficial effects on anion exchange through band 3 protein in human erythrocytes. Antioxidants (Basel) 9, 25. doi:10.3390/antiox9010025
Remigante, A., Morabito, R., Spinelli, S., Trichilo, V., Loddo, S., Sarikas, A., et al. (2020). D-Galactose decreases anion exchange capability through band 3 protein in human erythrocytes. Antioxidants (Basel) 9, 689. doi:10.3390/antiox9080689
Remigante, A., Spinelli, S., Straface, E., Gambardella, L., Caruso, D., Falliti, G., et al. (2022a). Antioxidant activity of quercetin in a H(2)O(2)-induced oxidative stress model in red blood cells: Functional role of band 3 protein. Int. J. Mol. Sci. 23, 10991. doi:10.3390/ijms231910991
Remigante, A., Spinelli, S., Basile, N., Caruso, D., Falliti, G., Dossena, S., et al. (2022d). Oxidation stress as a mechanism of aging in human erythrocytes: Protective effect of quercetin. Int. J. Mol. Sci. 23, 7781. doi:10.3390/ijms23147781
Remigante, A., Spinelli, S., Pusch, M., Sarikas, A., Morabito, R., Marino, A., et al. (2022e). Role of SLC4 and SLC26 solute carriers during oxidative stress. Acta Physiol. (Oxf) 235, e13796. doi:10.1111/apha.13796
Remigante, A., Spinelli, S., Straface, E., Gambardella, L., Caruso, D., Falliti, G., et al. (2022b). Acai (euterpe oleracea) extract protects human erythrocytes from age-related oxidative stress. Cells 11, 2391. doi:10.3390/cells11152391
Remigante, A., Spinelli, S., Trichilo, V., Loddo, S., Sarikas, A., Pusch, M., et al. (2022c). d-Galactose induced early aging in human erythrocytes: Role of band 3 protein. J. Cell Physiol. 237, 1586–1596. doi:10.1002/jcp.30632
Remigante, A., Zuccolini, P., Barbieri, R., Ferrera, L., Morabito, R., Gavazzo, P., et al. (2021b). NS-11021 modulates cancer-associated processes independently of BK channels in melanoma and pancreatic duct adenocarcinoma cell lines. Cancers (Basel) 13, 6144. doi:10.3390/cancers13236144
Rizvi, S. I., and Maurya, P. K. (2007). Markers of oxidative stress in erythrocytes during aging in humans. Ann. N. Y. Acad. Sci. 1100, 373–382. doi:10.1196/annals.1395.041
Romano, L., and Passow, H. (1984). Characterization of anion transport system in trout red blood cell. Am. J. Physiol. 246, C330–C338. doi:10.1152/ajpcell.1984.246.3.C330
Romano, L., Scuteri, A., Gugliotta, T., Romano, P., de Luca, G., Sidoti, A., et al. (2002). Sulphate influx in the erythrocytes of normotensive, diabetic and hypertensive patients. Cell Biol. Int. 26, 421–426. doi:10.1006/cbir.2002.0874
Russo, C., Maugeri, A., Lombardo, G. E., Musumeci, L., Barreca, D., Rapisarda, A., et al. (2021). The second life of citrus fruit waste: A valuable source of bioactive compounds. Molecules 26, 5991. doi:10.3390/molecules26195991
Russo, M., Arigò, A., Calabrò, M. L., Farnetti, S., Mondello, L., and Dugo, P. (2016). Bergamot (citrus bergamia risso) as a source of nutraceuticals: Limonoids and flavonoids. J. Funct. Foods 20, 10–19. doi:10.1016/j.jff.2015.10.005
Russo, M., Bonaccorsi, I., Torre, G., Sarò, M., Dugo, P., and Mondello, L. (2014). Underestimated sources of flavonoids, limonoids and dietary fibre: Availability in lemon's by-products. J. Funct. foods 9, 18–26. doi:10.1016/j.jff.2014.04.004
Russo, M., Dugo, P., Marzocco, S., Inferrera, V., and Mondello, L. (2015). Multidimensional preparative liquid chromatography to isolate flavonoids from bergamot juice and evaluation of their anti-inflammatory potential. J. Sep. Sci. 38, 4196–4203. doi:10.1002/jssc.201500878
Sahebkar, A. (2015). Dual effect of curcumin in preventing atherosclerosis: The potential role of pro-oxidant-antioxidant mechanisms. Nat. Prod. Res. 29, 491–492. doi:10.1080/14786419.2014.956212
Schwartz, R. S., Madsen, J. W., Rybicki, A. C., and Nagel, R. L. (1991). Oxidation of spectrin and deformability defects in diabetic erythrocytes. Diabetes 40, 701–708. doi:10.2337/diab.40.6.701
Shiga, T., Maeda, N., and Kon, K. (1990). Erythrocyte rheology. Crit. Rev. Oncol. Hematol. 10, 9–48. doi:10.1016/1040-8428(90)90020-s
Silva-Herdade, A. S., Andolina, G., Faggio, C., Calado, A., and Saldanha, C. (2016). Erythrocyte deformability - a partner of the inflammatory response. Microvasc. Res. 107, 34–38. doi:10.1016/j.mvr.2016.04.011
Singh, B., Singh, J. P., Kaur, A., and Singh, N. (2020). Phenolic composition, antioxidant potential and health benefits of citrus peel. Food Res. Int. 132, 109114. doi:10.1016/j.foodres.2020.109114
Sompong, W., Cheng, H., and Adisakwattana, S. (2015). Protective effects of ferulic acid on high glucose-induced protein glycation, lipid peroxidation, and membrane ion pump activity in human erythrocytes. PLoS One 10, e0129495. doi:10.1371/journal.pone.0129495
Spinelli, S., Straface, E., Gambardella, L., Caruso, D., Falliti, G., Remigante, A., et al. (2023). Aging injury impairs structural properties and cell signaling in human red blood cells; acai berry is a keystone. Antioxidants (Basel) 12, 848. doi:10.3390/antiox12040848
Straface, E., Gambardella, L., Mattatelli, A., Canali, E., Boccalini, F., Agati, L., et al. (2011). The red blood cell as a gender-associated biomarker in metabolic syndrome: A pilot study. Int. J. Cell Biol. 2011, 1–7. doi:10.1155/2011/204157
Subasinghe, W., and Spence, D. M. (2008). Simultaneous determination of cell aging and ATP release from erythrocytes and its implications in type 2 diabetes. Anal. Chim. Acta 618, 227–233. doi:10.1016/j.aca.2008.04.061
Tan, B. L., Norhaizan, M. E., and Liew, W. P. (2018). Nutrients and oxidative stress: Friend or foe? Oxid. Med. Cell Longev. 2018, 1–24. doi:10.1155/2018/9719584
Teti, D., Crupi, M., Busa, M., Valenti, A., Loddo, S., Mondello, M., et al. (2005). Chemical and pathological oxidative influences on band 3 protein anion-exchanger. Cell Physiol. Biochem. 16, 77–86. doi:10.1159/000087734
Turk, Z., Mesić, R., and Benko, B. (1998). Comparison of advanced glycation endproducts on haemoglobin (Hb-AGE) and haemoglobin A1c for the assessment of diabetic control. Clin. Chim. acta 277, 159–170. doi:10.1016/s0009-8981(98)00128-4
Ulanczyk, Z., Grabowicz, A., Cecerska-Heryc, E., Sleboda-Taront, D., Krytkowska, E., Mozolewska-Piotrowska, K., et al. (2020). Dietary and lifestyle factors modulate the activity of the endogenous antioxidant system in patients with age-related macular degeneration: Correlations with disease severity. Antioxidants (Basel) 9, 954. doi:10.3390/antiox9100954
Vallese, F., Kim, K., Yen, L. Y., Johnston, J. D., Noble, A. J., Cali, T., et al. (2022). Architecture of the human erythrocyte ankyrin-1 complex. Nat. Struct. Mol. Biol. 29, 706–718. doi:10.1038/s41594-022-00792-w
Willekens, F. L., Werre, J. M., Groenen-Dopp, Y. A., Roerdinkholder-Stoelwinder, B., de Pauw, B., and Bosman, G. J. (2008). Erythrocyte vesiculation: A self-protective mechanism? Br. J. Haematol. 141, 549–556. doi:10.1111/j.1365-2141.2008.07055.x
Wu, F., Satchwell, T. J., and Toye, A. M. (2011). Anion exchanger 1 in red blood cells and kidney: Band 3’s in a podThis paper is one of a selection of papers published in a Special Issue entitled CSBMCB 53rd Annual Meeting — membrane Proteins in Health and Disease, and has undergone the Journal’s usual peer review process. Biochem. Cell Biol. 89, 106–114. doi:10.1139/o10-146
Xu, D., Hu, M. J., Wang, Y. Q., and Cui, Y. L. (2019). Antioxidant activities of quercetin and its complexes for medicinal application. Molecules 24, 1123. doi:10.3390/molecules24061123
Zabinski, Z., Dabrowski, Z., Moszczynski, P., and Rutowski, J. (2000). The activity of erythrocyte enzymes and basic indices of peripheral blood erythrocytes from workers chronically exposed to mercury vapours. Toxicol. Ind. Health 16, 58–64. doi:10.1191/074823300678827663
Zhang, Y., Manning, L. R., Falcone, J., Platt, O., and Manning, J. M. (2003). Human erythrocyte membrane band 3 protein influences hemoglobin cooperativity. J. Biol. Chem. 278, 39565–39571. doi:10.1074/jbc.M303352200
Zhao, H., Dong, J., Lu, J., Chen, J., Li, Y., Shan, L., et al. (2006). Effects of extraction solvent mixtures on antioxidant activity evaluation and their extraction capacity and selectivity for free phenolic compounds in barley (Hordeum vulgare L). J. Agric. Food Chem. 54, 7277–7286. doi:10.1021/jf061087w
Keywords: flavonoid fraction, bergamot (citrus bergamia), peel and/or juice extract, oxidative stress, aging, red blood cells, band 3 anion exchanger
Citation: Remigante A, Spinelli S, Straface E, Gambardella L, Russo M, Cafeo G, Caruso D, Falliti G, Dugo P, Dossena S, Marino A and Morabito R (2023) Mechanisms underlying the anti-aging activity of bergamot (Citrus bergamia) extract in human red blood cells. Front. Physiol. 14:1225552. doi: 10.3389/fphys.2023.1225552
Received: 19 May 2023; Accepted: 22 June 2023;
Published: 30 June 2023.
Edited by:
Andrea Gerbino, University of Bari Aldo Moro, ItalyReviewed by:
Francisco O. Silva, University of Texas Southwestern Medical Center, United StatesCopyright © 2023 Remigante, Spinelli, Straface, Gambardella, Russo, Cafeo, Caruso, Falliti, Dugo, Dossena, Marino and Morabito. This is an open-access article distributed under the terms of the Creative Commons Attribution License (CC BY). The use, distribution or reproduction in other forums is permitted, provided the original author(s) and the copyright owner(s) are credited and that the original publication in this journal is cited, in accordance with accepted academic practice. No use, distribution or reproduction is permitted which does not comply with these terms.
*Correspondence: Alessia Remigante, YXJlbWlnYW50ZUB1bmltZS5pdA==
†These authors have contributed equally to this work
‡These authors share senior authorship
Disclaimer: All claims expressed in this article are solely those of the authors and do not necessarily represent those of their affiliated organizations, or those of the publisher, the editors and the reviewers. Any product that may be evaluated in this article or claim that may be made by its manufacturer is not guaranteed or endorsed by the publisher.
Research integrity at Frontiers
Learn more about the work of our research integrity team to safeguard the quality of each article we publish.