- 1School of Global Health, Faculty of Health, York University, Toronto, ON, Canada
- 2Department of Family and Community Medicine, St. Michael’s Hospital, Toronto, ON, Canada
- 3Muscle Health Research Center, School of Kinesiology and Health Science, Faculty of Health, York University, Toronto, ON, Canada
- 4Laboratoire des Sciences Pour l’Environnement (SPE), UMR-CNRS 6134, University of Corsica Pasquale Paoli, Campus Grimaldi, Corte, France
Climate change favors weather conditions conducive to wildland fires. The intensity and frequency of forest fires are increasing, and fire seasons are lengthening. Exposure of human populations to smoke emitted by these fires increases, thereby contributing to airborne pollution through the emission of gas and particulate matter (PM). The adverse health outcomes associated with wildland fire exposure represent an important burden on the economies and health systems of societies. Even though cardiovascular diseases (CVDs) are the main of cause of the global burden of diseases attributable to PM exposure, it remains difficult to show reliable associations between exposure to wildland fire smoke and cardiovascular disease risk in population-based studies. Optimal health requires a resilient and adaptable network of small blood vessels, namely, the microvasculature. Often alterations of this microvasculature precede the occurrence of adverse health outcomes, including CVD. Biomarkers of microvascular health could then represent possible markers for the early detection of poor cardiovascular outcomes. This review aims to synthesize the current literature to gauge whether assessing the microvasculature can better estimate the cardiovascular impact of wildland fires.
1 Introduction
Wildland fires are a major threat to human life. They adversely impact the environment, our health, and our economies. Worldwide estimates predict that global warming will generate more fire-prone areas in boreal and temperate regions. Wildland fires in these areas will become more frequent, larger, and more severe, leading to more damage and destruction (Bowman et al, 2020; Touma et al, 2021; Senande-Rivera, Insua-Costa, and Miguez-Macho, 2022). In recent years, some of the worst wildland fire seasons were reported all across the globe, with massive fires raging in Australia, in North America, around the Mediterranean Basin, in the Amazon Rainforest, and in the Siberian Boreal Forest (Lewis, 2020; Mega, 2020; Witze, 2020). In North America, including Eastern Canada (Canadian Shield and Hudson Bay), the length of the wildland fire season has increased by almost 19% over the last 35 years (Jain et al., 2018). If climate change and global warming certainly play a key role in this evolution of wildland fires (frequency, size, and severity), human factors must be acknowledged too. This was well highlighted in a recent communication from the Royal Society (Santin and Doerr, 2022): Population growth across North America increases the overall surface areas where human development intersects with wildland or vegetative fuels, thereby increasing odds of wildland fire smoke exposure to inhabitants (Peterson et al., 2021). Arson and accidental ignitions are mostly involved in highly populated areas (Balch et al, 2017). These risks can also be exacerbated by drastic fire suppression policies that aimed to subtract wildland fires from our ecosystems even though wildland fires contribute to their rejuvenation cycle. This can result in an excessive accumulation of biomass fuels (North et al, 2015).
Until recently, articles retrieved using search engines from new media database (i.e., google news, CBS, CBC, CNN and Reuters) with the keywords “wildland fire” AND “health” often focus on the immediate risks associated with wildland fires, including information about fire forecasts, injuries and casualties, losses and associated financial costs, firefighting activity, wildlife impact, and remodeled landscapes (Weber, 2020; CBS13/CNN, 2020; Canadian Red Cross, 2023; Hill, 2020). A few articles reported the short-term impact on health of chemical hazards acutely released in the air during wildland fires, having mostly an emphasis on the increased vulnerability to respiratory diseases such as asthma or more recently flu and COVID-19 (McFall-Johnsen, 2020a; McFall-Johnsen, 2020b; Kozlov, 2021). The long-term consequences of wildland fire smoke exposure remain even less documented. Long-term exposure studies have in fact essentially focused on the increased risk of developing cancer in professional firefighters (Asher-Schapiro, and Sherfinski, 2021; Kozlov, 2021). In the aftermath of Canadian wildland fires of the Spring and early Summer 2023, news media started discussing more massively the short-term impact on health of chemical hazards acutely released in the air during wildland fires (Christensen, 2023; Gibbens and McKeever, 2023; Lapid, 2023; Margaux, 2023; Migliaccio, 2023). Scientific blogs have also updated their information reporting what acute and adverse effects of wildland fire exposure are (K. Miller, 2023). These news media articles address the short-term health impact of wildland fire smoke, mostly reporting the exacerbations of respiratory symptoms and increased risks to adverse cardiovascular events.
Obviously, inhaled chemical pollutants can lodge in the respiratory tract, eventually reaching the lung alveoli at the deepest (Oberdörster, 1995; Guttenberg et al, 2016). The World Health Organization refers to particulate matters (PM) as a “common proxy indicator for outdoor air pollution”. PM2.5 and ultrafine particulate matters (UFP) with diameters respectively inferior or equal to 2.5 and 0.1 µm represent the smallest pollutants in size. Conversely to larger PM (as PM10) and other chemicals that will remain in the respiratory system, PM2.5 and UFP are small enough to penetrate the blood stream by passing through the lung alveolo-capillary barrier (WHO’s Air Quality and Health Unit, 2022). Subsequently, they can interact with the endothelium, the cellular lining found in our blood vessels and cardiac cavities, and we can then question their impact on cardiovascular health. This question is supported by the fact that cardiovascular disease (CVD) account for about 50% of the global burden posed by PM exposure, representing nearly sixty-one millions of disability-adjusted life years (DALYs) lost in 2019 (‘GBD Compare | IHME Viz Hub’ 2021). In the context of wildland fires, PM levels increase in the immediate vicinity of fires and also up to thousands of kilometers away from the fire center (Meng et al, 2019; Larsen et al, 2020; Matz et al, 2020; Schneider et al, 2021). In Canada between 2013 and 2018, PM originated from wildland fires in the Western and Northern ends of the continent impacted population in Central and Eastern Canada, supporting the notion of a long-range transport of wildland fire related PM.
Despite these facts, only a few recent news outlets and media release have highlighted the role that chemicals and particularly PM might play in increasing cardiovascular events (e.g., heart attacks) in response to an acute exposure to wildland fire smokes (Kozlov, 2021; Gibbens and McKeever, 2023; Lapid, 2023; Margaux, 2023; Migliaccio, 2023). Short-term exposure to wildland fire smoke seems to be a precipitating cause of adverse cardiovascular events in at-risk populations (Chen et al, 2021). Nonetheless, discrepancy exists between these studies and could be attributed to many factors: the dose exposure to PM (concentration x duration), the type of burned biomass, the use of different outcome measurements (Chen et al, 2021). Interestingly, most epidemiological studies examining the relationship between PM exposure during wildland fires and CVD use outcome measurements that are often late markers of cardiovascular conditions with a long prodromal phase, for example, hospital admissions for heart failure, myocardial infarction, pulmonary embolism, stroke. These conditions often share atherosclerosis as a common underlying cause, a pathological and aging process of the vessels developing over decades. Hence, developing effective measures to protect population from smoke exposure during wildland fire seems difficult (Hano et al, 2020; Marfori et al, 2020; Xu et al, 2020; Peterson et al., 2021).
Another important observation from these studies is the potential role played by the microvasculature. Indeed, populations with microvascular dysfunction might be more vulnerable to adverse cardiovascular outcomes in response to an exposure to wildland or biomass fire smoke. Whereas larger blood vessels (arteries, veins and venules) constitute the macrovasculature, arterioles and capillaries represent the microvasculature and the vast majority of our blood vessels. The microvasculature plays key roles in maintaining our cardiovascular health (Sörensen et al, 2016; Wagenmakers et al, 2016; Augustin and Koh, 2017; Stehouwer, 2018; Alam et al, 2019). Alterations of the microvasculature often precedes more adverse cardiovascular outcomes with macrovascular and cardiac dysfunction (Anderson et al, 2011; Lee et al, 2012; Stehouwer, 2018; van Sloten et al, 2020; Loai et al, 2022). Microvascular biomarkers that would allow an early detection of possible cardiovascular risks, when adverse effects are still reversible, could therefore represent a more suitable alternative to indicators of later CVD.
This review analyses the current literature to ascertain whether the microvascular perspective could be beneficial for the early detection of alterations in cardiovascular health during exposure to wildland fire smoke. The first section of this review (see 2.0) will determine if there is any association between microvascular alterations and cardiovascular outcomes after wildland fires based on epidemiological research. The second section of this review (See 3.0) will examine whether smoke components from wildland fires or anthropologic biomass burning impact the microvasculature. Finally, the last section (See 4.0) will identify how the remaining gaps of knowledge could be addressed to ascertain the value of microvascular biomarkers for early detection of the cardiovascular risk posed by wildland fire smoke.
2 What evidence do population-based studies provide regarding the impact of wildland fire on the microvasculature and cardiovascular outcome?
As reviewed in (Soteriades et al, 2011; Chen et al, 2021; Reid et al, 2016; Cascio, 2018), adverse cardiovascular outcomes might be more frequent when wildland fires occur. Yet, many studies failed to demonstrate any strong relationships between PM exposure and cardiovascular risk for populations who live either far or at proximity from wildland fires (Analitis, Georgiadis, and Katsouyanni, 2012; Delfino et al, 2009; Hanigan, Johnston, and Morgan, 2008; Alman et al, 2016; Henderson et al, 2011; Liu et al, 2017; Reid et al, 2016). These equivocal results may arise from the difficulty to clearly establish the exact dose of PM a population is exposed to in the event of a wildland fire or during the entire fire season. It could also indicate that the outcomes measured are too broad to optimally assess the cardiovascular risk. Nonetheless, and noteworthy, positive association emerges more clearly in studies using cardiovascular pathologies associated with microvascular dysfunctions (e.g., diabetes or heart failure) as outcomes to test the relationship between wildland fire smoke exposure and cardiovascular risk (Figure 1). Non-invasive assessments of the microvasculature have emerged as innovative tools to stratify the cardiovascular risk at a population level, and this could be applied to wildland fires to better assess their risk for cardiovascular health.
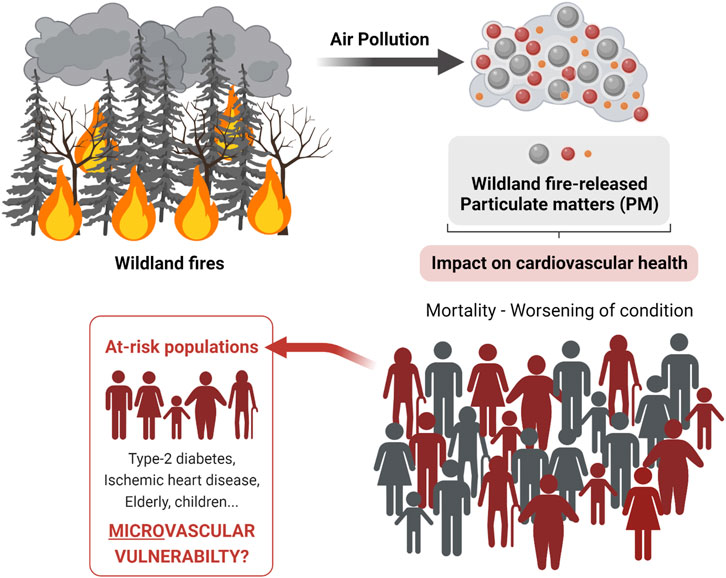
FIGURE 1. Impact of wildland fires-related PM on cardiovascular health. Population-based studies suggest that PM originating from wildland fires might more severely impact individuals with microvascular vulnerability. Created with BioRender.com.
2.1 Air pollution from wildland fires and biomass burning in the general population: Impact on cardiovascular mortality and medical consultations
It remains difficult to demonstrate a strong positive relationship between cardiovascular mortality and exposure to PM during wildland fire season. In the Greater Athens Area between 1998 and 2004, medium and large wildland fires (greater than
Analyzing the impact of wildland fires and biomass burning on medical consultations for cardiovascular events remains also largely inconclusive. When assessing wildland fire exposure based on the smoke density, medium and dense smoke significantly increased the odds of admission to emergency departments for cardiovascular and cerebrovascular diseases in Californian adults during the wildland fire season in 2015 (Wettstein et al, 2022). In Brazil, Ye and colleagues estimated that any rise of PM2.5 by 10 μg/m3 could increase admissions for cardiovascular conditions by 10% 24 h following the start of wildland fires (Ye et al, 2021). Yet, other studies did not report any positive association between PM2.5 and PM10 exposure and hospital admission due to CVD in the general population (Hanigan, Johnston, and Morgan, 2008; Kollanus et al, 2016; Mahsin, Cabaj, and Saini, 2021; Alman et al, 2016; J. C; Liu et al, 2017; Johnston et al, 2014; 2007). In Helsinki, Finland, long-range PM2.5 levels observed during vegetation fires were not related to any change in hospital admissions due to CVD. During the 1996–2005 fire seasons in Darwin, Australia, daily hospital admission due to CVD were not associated with PM10 estimates on the day of admission and up to 3 days pre-admission (Hanigan, Johnston, and Morgan, 2008). Similar observations were made in Australia in Sydney, Wollongong, and Newcastle for the 1996–2007 period, and in Western regions of North America (British Columbia and California) during Summer 2003. During fire seasons across various settings, PM2.5 or/and PM10 levels were not related to hospital admission or physician visits due to overall CVD in the general population (Delfino et al, 2009; Henderson et al, 2011; Martin et al, 2013; Johnston et al, 2014; Hutchinson et al, 2018; Doubleday et al, 2020). Yet, some of these studies reported positive association with respiratory diseases admission (Hutchinson et al, 2018; Doubleday et al, 2020).
The above studies did not identify any clear relationship between exposure to PM from wildland fires or biomass burning and medical consultations for cardiovascular pathologies or cardiovascular mortality in the general population.
2.2 Impact of air pollution from wildland fires and biomass burning in patients presenting microvascular vulnerability
When analyzing specific cardiovascular conditions and age groups, it appears that certain populations with microvascular dysfunction might be more vulnerable to adverse cardiovascular outcomes. Older individuals and patients with cardio-metabolic diseases, such as diabetes, heart failure, and ischemic heart diseases, were more likely to seek medical assistance following exposure to wildland or biomass fire smoke (Delfino et al, 2009; Henderson et al, 2011; Haikerwal et al, 2015; Yao et al, 2020; Mahsin, Cabaj, and Saini, 2021; Wettstein et al, 2022). It is noteworthy that these cardio-metabolic diseases are associated with microvascular alterations that can alter whole-body performance. Heart failure and ischemic heart disease present functional alterations of the heart and skeletal muscle microvasculature (D’Amario, Borovac, and Crea, 2021; Kitzman et al, 2014; D’Amario et al, 2019; Mehta et al, 2022; van de Hoef et al, 2020). Patients unable to maintain a functional microvasculature in the skeletal muscle and cardiac tissues have a greater intolerance to performing daily activities, that could worsen symptoms such as dyspnea and fatigue that are hallmarks of heart failure and main causes of hospitalizations (Kitzman et al, 2014; Del Buono et al, 2019; Mahfouz, Gouda, and Abdelhamid, 2020). As individuals age, microvascular dysfunctions in striated cardiac and skeletal muscles will limit blood flow distribution to these tissues during physical exertion, making them more susceptible to ischemic pathologies (Bearden, 2006; A. J; LeBlanc and Hoying, 2016).
Multiple studies have reported an increased risk to seek medical care due to acute myocardial infarction, ischemic heart disease, and congestive heart failure in the event of wildland fire-related PM exposure (Johnston et al, 2014; 2007; Rappold A. G. et al, 2011; Rappold et al, 2012; Haikerwal et al, 2015; Yao et al, 2020; Mahsin, Cabaj, and Saini, 2021; Wettstein et al, 2022). The risk appears to be even higher for older individuals (Delfino et al, 2009; Johnston et al, 2014). For example, during the Fall 2003 Californian wildland fire season, PM2.5 exposure was positively associated with hospitalization when examining admissions due to congestive heart failure (+11.3%, all age groups) for individuals aged 45–99 years (Delfino et al, 2009). In Australia, during bush fires, PM exposure led to higher odds of emergency department admissions due to ischemic heart diseases in Darwin’s aboriginal population and due to heart failure in Sydney’s inhabitants (Johnston et al, 2014; 2007). During the wildland fire season of Summer 2006–2007 in Victoria (Australia), any increase of PM2.5 by 9 μg/m3 increased the risk of hospital admission due to ischemic heart disease and acute myocardial infarction (Haikerwal et al, 2015). Similar observations were made during the fire seasons of 2010–2015 in British Columbia (Canada) where increased odds of myocardial infarction (+19% after 17 h) and ischemic heart disease (+7% after 28 h) following exposure to PM2.5 were reported (Yao et al, 2020). An increased risk of diabetic outcomes (i.e., hyperglycemia or hypoglycemia) (+20%) was also observed 48 h post-exposure (Yao et al, 2020). Some of the studies cited above indicate that older individuals aged 65 years and older had a higher risk for hospital admission due to CVD (Haikerwal et al, 2015; Mahsin, Cabaj, and Saini, 2021; Wettstein et al, 2022). Every increase in PM2.5 by 10 μg/m3 increased the risk of physician visits due to congestive heart failure and ischemic heart disease by 11% and 19%, respectively, for seniors aged 65 years and older. To add, seniors with diabetes had an increased risk of cardiovascular morbidity (+30%), post fire (Mahsin, Cabaj, and Saini, 2021). In California, older individuals (65+) had a greater risk of admission due to cardiovascular and cerebrovascular diseases when the density of wildfire smoke increased above 10.5 μg/m3 of PM2.5 (Wettstein et al, 2022). While the association between PM exposure and cardiovascular risk in the general population remains uncertain, exposure to wildland fire smoke appears to be linked with worse cardiovascular outcomes in older individuals and in patients with other comorbid cardiometabolic diseases (i.e., heart failure, ischemic heart diseases and diabetes). Since microvascular dysfunction worsens cardiometabolic symptoms, it may be postulated that exposure to wildland fire smoke may directly trigger additional microvascular dysfunction thereby exacerbating pre-existing cardiovascular morbidities.
2.3 Direct evidence of the impact of wildland fires or biomass burning exposure on the microvasculature in the general population
A limited number of studies have investigated whether outdoor air pollution generated by wildland fires could directly impact the microvasculature. Yet, a few studies have non-invasively evaluated the microvasculature, corroborating the notion that biomass burning could indeed impact the microvasculature, both functionally and structurally. In vivo imaging of the retina allows for a non-invasive assessment of microvascular alterations. Changes in the geometry of the retinal microvasculature has indeed emerged as a valuable tool to stratify cardiovascular risk. A reduced diameter of the central retinal arterioles (CRAE), a greater diameter of the central retinal venules (CRVE) and a lower arteriolar-to-venular ratio (AVR = CRAE/CRVE) are associated with adverse cardiovascular events and cardiovascular risk factors (Mutlu et al, 2016; Seidelmann et al, 2016; Rijks et al, 2018). Retinal microvasculature measurement has been used to assess the impact of urban airborne pollution on microvascular function among individuals. This approach has shown that long-term and short-term exposure to black carbon, PM2.5 and PM10, was linked with a decreased central retinal arteriole diameter among adults and children (Adar et al, 2010; Louwies et al, 2013; Provost et al, 2017). Thus, urban air pollution alters the geometry of the retinal microvasculature, potentially suggesting an increased cardiovascular risk. Among children aged 4–6 years, prenatal and early childhood exposure to PM2.5 and nitrogen oxide (NOx) was associated with widening of the retinal venular and arterial diameters (Luyten et al, 2020). Using a similar approach, Korsiak and colleagues assessed the short-term impact (same day to 3 weeks of lag exposure) of outdoor pollution from biomass burning on the retinal microvasculature in children aged 4–12 years living in rural areas (Korsiak et al, 2021). PM2.5 levels and an index of the presence of oxidant gases (Ox) that combines a measure of ozone and nitrogen oxide (O3 and NOx) to assess outdoor pollution. Any increase of Ox by 10 ppm over a period of 7 days was associated with a decrease in retinal arteriole diameter by 2.63 μm (−1.44% of the total arteriolar diameter). Yet, PM2.5 exposure due to biomass burning only impacted the diameter of retinal arterioles when Ox levels were high (Korsiak et al, 2021), suggesting that the interaction between oxidant gases and PM elicits greater impact on the microvasculature. Further investigations will better delineate whether structural changes observed in children’s retinal microvasculature are good predictors of future cardiovascular risks.
Another approach has evaluated whether residential pollution alters cutaneous microvascular functions. Measuring changes in skin blood flow is a promising non-invasive tool to evaluate the microvascular function, and to indirectly assess cardiovascular health. In fact, Witters and colleagues assessed the impact of residential air pollution on the cutaneous microvascular function of children aged 4–6 years using local heat-mediated vasodilation (Witters et al, 2021). In response to local skin heating, vasodilation of the skin’s microvasculature increases local blood flow as measured by laser doppler. Prenatal exposure to black carbon, PM2.5 or PM10 of residential origin during the last trimester of pregnancy lowered the cutaneous microvascular function in children aged 4–6 years (Witters et al, 2021). Yet, post-natal exposure had no impact on the microvascular function and did not modify the association between prenatal exposure and microvascular function (Witters et al, 2021). To our knowledge, the impact of PM exposure on skin microvascular function has not been investigated in the specific context of wildland or biomass burning.
As conclusion of this first section, future studies combining an accurate dosimetry of PM with a non-invasive assessment of the microvascular function would contribute to better evaluate the cardiovascular risk of wildland fires PM exposure.
3 Could chemicals present in wildland fire smoke impact the microvascular endothelium?
3.1 Overview of the microvasculature
The vasculature is a vast system of blood vessels composed of arteries, arterioles, capillaries, venules, and veins. All these vessels are primarily composed of a layer of endothelial cells forming the endothelium. Arterioles and capillaries represent together the microvasculature whereas larger diameters vessels constitute the macrovasculature. Arterioles are located downstream of resistance arteries. In a simplistic representation, arterioles can be seen as vascular tubes made of endothelial cells and supported by vascular smooth muscle cells (VSMCs) that confer to arterioles their vasomotricity (vasoconstriction and vasodilation). Such vasomotricity allows arterioles to play a central role in controlling peripheral vascular resistance and blood pressure (Jackson, 2021). For example, in the skeletal muscle, which represent about 40% of the body mass, arterioles contributes for about 50% of tissue vascular resistance (Fronek and Zweifach, 1975). These arterioles largely determines the blood flow into downstream capillaries, which are the most abundant and smallest blood vessels in the human body. Capillaries are constituted of a single layer of endothelial cells, supported by mural cells such as pericytes. In a healthy adult, the capillary endothelium with a surface of 600 m2 represents more than 85% of the total vascular surface (Wagenmakers et al, 2016). The capillary endothelium supports key homeostatic functions: delivery of oxygen and nutrients to tissues, capacity of tissues to respond to infection, metabolic tissular adaptation, thermoregulation, endocrine communication, tissue regeneration after injury (Augustin and Koh, 2017). The microvascular endothelium found in arterioles and capillaries also senses local vasodilatory molecules present in the tissue, relaying the information to the arteriolar VSMCs. The coordinated action of the microvascular endothelium and smooth muscle tissue regulates the process of arteriolar vasodilation ensuring an appropriate blood supply to the tissue as well as an optimal control of blood pressure (Murrant and Sarelius, 2015; Dora, 2016; Wagenmakers et al, 2016). From this standpoint, it is obvious to comprehend why health, and particularly cardiovascular health, requires an optimal microvasculature (Figure 3). Recent studies have suggested that atmospheric pollution and PM could dysregulate blood pressure. Wen and colleagues have reported a positive association between atmospheric pollution and diastolic blood pressure in post-menopausal women (Wen et al, 2023). And Marrone and colleagues have described a potential positive correlation with systolic blood pressure in adolescents (Marrone et al, 2023). Based on these observations, studying whether exposure to wildland fire smoke has a short-term or long-term impact on the microvasculature has become more critical than ever.
3.2 Presence of inhaled pollutants in the blood circulation and interaction with the endothelium
To impact the endothelium, and more particularly the microvascular endothelium, wildland fire pollutants must enter the blood stream (Figure 2). Biomass burning and wildland fire emit smoke into the atmosphere that contain a complex mixture of chemicals under the form of gases, volatile organic and semi-volatiles compounds (VOC and SVOC), polycyclic hydrocarbons, and particulate matter (Barboni et al, 2011; 2010; Romagnoli et al, 2014) (Baxter et al, 2014; Navarro et al, 2019; 2017; Cherry et al, 2021). The main pollutants released are carbon dioxide (CO2), carbon monoxide (CO), nitrogen oxides (NOx), and aerosols (i.e., particulate matter). Aerosols consist of solid particles, such as soot, with a diameter less than or equal to 1 µm (UFP and PM1), and liquid particles in the form of tar, with a diameter less than or equal to 2.5 µm (PM2.5). Aerosols are molecules with long-range transportability that can be inhaled during breathing and absorbed through skin deposition during or in the aftermath of a wildland fire (Meng et al, 2019; Cherry et al, 2021).
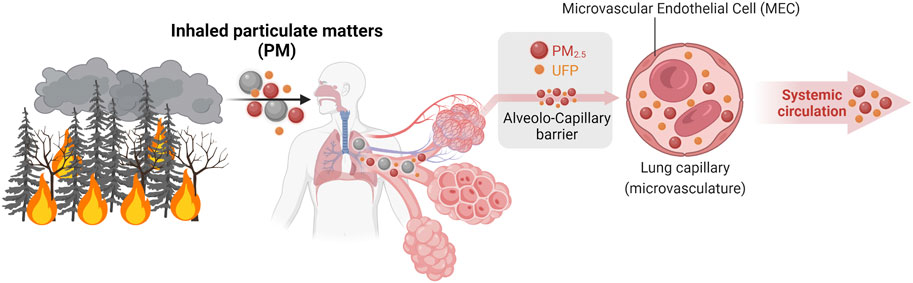
FIGURE 2. Wildland fire-related PM can enter the bloodstream. Being the smallest pollutants, PM2.5 and ultrafine PM (UFP) penetrate the alveolo-capillary barrier and then enter the bloodstream. Created with BioRender.com.
As previously mentioned, the smallest PM (PM2.5 and UFP) can penetrate through the lung alveolo-capillary barrier into the blood stream (Figure 2). Once in the circulation, these PM promote systemic inflammation, affecting both circulating immune cells and endothelial cells (Pope et al, 2016; Finch et al, 2019). Limited studies have specifically investigated whether PM2.5 and UFP directly interact with the microvascular endothelium. Yet, a few studies support this notion. First, inhaled nanoparticles were found to circulate in the blood stream and accumulate in diseased arteries in both preclinical rodent models and in human subjects (Miller et al, 2017; Raftis and Miller, 2019; Nemmar et al, 2002). A few studies have also shown that after inhalation, nanoparticles circulate in the microvascular beds of multiple organs. For example, inhaled silica nanoparticles were found in capillaries from lung, lymph nodes, spleen, and kidney tissues from male F344 rats (Guttenberg et al, 2016). Additionally, an accumulation of PM2.5 originating from organophosphate flame retardants was observed in the heart, brain, and skeletal muscle tissues from C57B6J mice (M. Chen et al, 2020). The endothelia in these tissues are continuous. To reach tissue cells, these circulating pollutants must therefore interact with and then cross the microvascular endothelium to penetrate and accumulate into tissues. The interaction between these circulating PM and endothelial cells could also modify the communication between endothelial cells and VSMCs and alter the arteriolar vasomotricity with consequences on the blood flow feeding downstream capillaries (Figure 3). The impact of PM on the vasomotricy might vary depending on the size of PM inhaled. Using calibrated titanium oxide PM, Nurkiewicz et colleagues reported that the microvascular impairment (i.e., capacity of the arterioles to dilate) increases as the size of PM decreases (Nurkiewicz et al, 2011). Once in these tissues, PM can promote cell damages and oxidative stress that trigger inflammation (Farina et al, 2019; Hameed et al, 2022). This can feed a vicious circle where tissue inflammation could promote further microvascular dysfunction (Nurkiewicz et al, 2011; 2008). Circulating PM could also interact with the capillary endothelium to impair the capacity of the capillary bed to remodel or grow through angiogenesis, the formation of new capillaries from pre-existing ones (Abe et al, 1990; Capitão and Soares, 2016; Fuchs et al, 2017; Shi and Vanhoutte, 2017) (Figure 3). Pope and colleagues have analyzed the impact of urban-related PM and UFP on the blood composition (Pope et al, 2016). They reported that PM2.5 concentration correlated positively with the number of circulating endothelial microparticle, a marker of endothelial cell damage, and negatively with the concentration of circulating cytokines known to promote angiogenesis (the formation of new capillaries from existing ones). To date, it remains unknown whether PM from wildland fire origin have similar impact on the microvasculature. While it remains unclear if a similar effect (tissue accumulation) prevails with PM specifically released from wildland fire smoke, these findings suggest that ultrafine and fine PM from airborne pollution could circulate through the microvasculature, interact with microvascular endothelia, and accumulate in various tissues (Figure 3). If confirmed, these PM-induced microvascular alterations could have important repercussions on cardiovascular health since arteriolar vasomotricity and capillary angiogenesis could de facto support the restoration of blood flow and tissue repair post-ischemic events (e.g., myocardial infarction, peripheral arterial disease). Indeed the alteration of these microvascular processes can participate to the pathogenesis and progression of CVD (Lähteenvuo and Rosenzweig, 2012; Crea, Montone, and Rinaldi, 2021; D’Amario, Borovac, and Crea, 2021; Dean et al, 2015; Annex and Cooke, 2021; Coker et al, 2019; Chen et al, 2016).
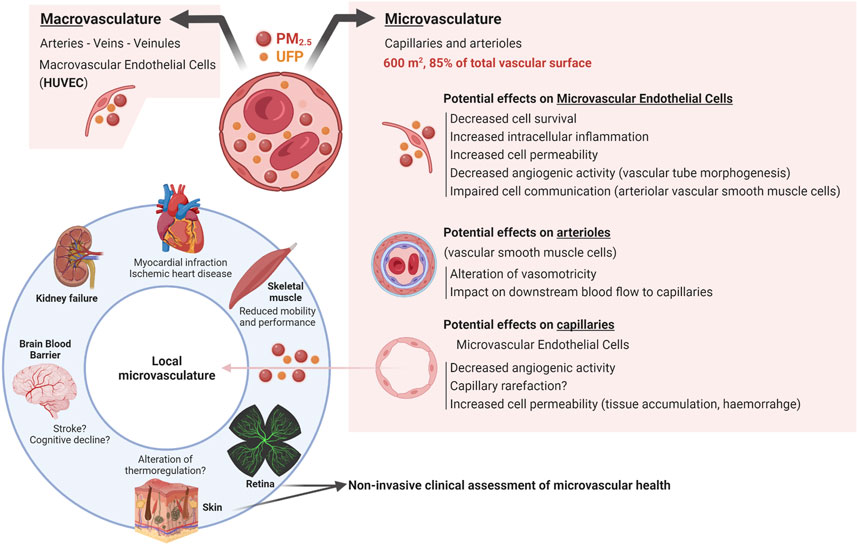
FIGURE 3. Circulating PM interacts with macro- and micro-vascular endothelia potentially impacting multiple tissues. Once in the bloodstream, PM2.5 and ultrafine PM (UFP) might impair the biology of macro- and micro-endothelial cells with consequences on vessel integrity (arterioles, capillaries), angiogenic capacity, and inflammation. The microvasculature of various tissues and organs (kidneys, cardiac and skeletal muscle, brain, skin, retina) could be altered with consequences on disease progression and functionality (cognitive aging, fatigue, worsening of cardiac conditions). Non-invasive assessments of microvasculature could represent a new avenue for cardiovascular risk assessment in the context of wildland fire smoke exposure. Created with BioRender.com.
3.3 Assessing the impact of wildland fire smoke on the microvascular smooth muscle
Limited literature exists regarding the impact of PM from wildland fire smoke on vascular smooth muscle cells (VSMCs). As reviewed by Ming and colleagues, the impact of PM2.5 on VSMCs has been mainly investigated for particles originating from cigarette smoke, diesel exhaust, or urban airborne pollution but not wildland fires and other biomass burning (Ming et al, 2022). In addition, most of these studies have used smooth muscle cells from macrovascular origin (i.e., isolated from aorta) with an interest in studying whether PM could promote atherosclerosis through the activation of smooth muscle hypertrophy (Chang et al, 2017; Wan et al., 2018; Ho et al, 2019; Gu et al, 2021; Ho et al, 2021; Tian et al, 2021; Ju et al, 2022; Ming et al, 2022; Bao et al, 2023). Similarly to cigarette smoke-related PM, particles from urban pollution increase mitogenic and hypertrophic signals (e.g., PDGFR-β, JAK2/STAT3) in macrovascular VSMCs, promoting the thickening of the media, an contributing to atherosclerosis and calcification in the aorta (Bao et al, 2023; Schroeter et al, 2008; R; Li et al, 2013). Exposure to PM from cigarette smoke and urban airborne pollution also induce an important oxidative stress in these macrovascular VSMCs, enhancing pro-inflammatory signals (i.e., NFκB), the expression of metalloproteinase (MMPs), cell proliferation and migration (Chang et al, 2017; Ho et al, 2021; Ju et al, 2022). These studies indicate that PM can significantly alter the phenotype of VSMCs. PM exposure has been reported to significantly impair the microvascular function, with frequent report of endothelial dysfunction (Pope et al, 2016; Nurkiewicz et al, 2004; A; LeBlanc et al, 2009). Yet, nanoparticles formed of titanium oxide altered the endothelial function in sub-epicardial arterioles without apparent changes in the sensitivity of the vascular smooth muscle to nitric oxide, the most potent local vasodilator (LeBlanc et al, 2009). We could therefore hypothesize that PM produced by wildland fires could have a similar impact of the phenotype of microvascular VSMCs. To our knowledge, there is no study that has investigated the impact of PM from wildland fire smoke on VSMCs from microvascular origin. It remains unknown what the impact of wildland fire related PM on the capacity of arterioles to dilate could be. This highlight the need to perform further investigation on whether wildland fire smoke could alter the control of blood flow by damaging the microvascular smooth muscle.
3.4 Assessing in vitro the impact of wildland fire smoke pollutants on microvascular endothelial cells
Despite the importance of the microvasculature in cardiovascular health, Microvascular Endothelial Cells (MECs) remain largely understudied as an in vitro model to investigate the effect of airborne pollutants on endothelial cells. The most frequently used endothelial cells to investigate the toxicity of nanoparticles and air pollution are macrovascular Human Umbilical Vein Endothelial Cells (HUVECs) (Ding et al, 2020; S; Chen et al, 2017; He et al, 2021; Setyawati et al, 2015; Jung et al, 2022; Su et al, 2020; Hu et al, 2017; Y; Liu et al, 2021; Wang et al, 2017), which are phenotypically different from MECs, and therefore might respond differently to stressors (Lang et al, 2003; Suphasiriroj, Mikami, and Sato, 2013; Lau et al, 2021). This might hold true for PM, as nanotoxicity is known to vary depending on the phenotype and shape of endothelial cells (Setyawati et al, 2015). When comparing the impact of urban PM2.5 on HUVECs and human microvascular ECs (HMECs), Chen and colleagues reported a decrease in cell survival, an increase in intracellular inflammation, and reduced angiogenic capacity for high concentrations of PM2.5 (100–800 μg/mL, 24 h treatment) in both microvascular (HMECs) and macrovascular (HUVECs) endothelial cells (Chen et al, 2017). Yet, the effects of PM2.5 were less severe in HMECs. In another study, exposure to PM10 (5–50 μg/mL for 24 h) altered cell permeability in human brain MECs (HBMECs) and led to the acquisition of pro-senescent and pro-inflammatory profiles with high levels of ICAM and vascular adhesion molecule-1 (VCAM) in these cells (Park et al, 2021).
In spite of the limitation of being of macrovascular and venous origin, HUVECs have nonetheless proven useful to assess chemicals toxicity (Cao et al, 2017; Cao, 2018). Nanoparticles and PM2.5 can enter HUVECs through endocytosis and micropinocytosis and can be released by exocytosis (Cao, 2018; Su et al, 2020). The mechanism of cell internationalization varies depending on the chemical composition of the PM2.5. For example, metal-rich PM2.5 enter HUVECs through micropinocytosis; PAH-rich PM2.5 require clathrin-dependent endocytosis, and water-soluble PM2.5 are internalized through a caveolin-dependent endocytosis (Su et al, 2020). Once inside the cells, PM2.5 can alter the cytoskeleton structure and reduce HUVECs survival via apoptosis in a time and dose dependent manner (Wang et al, 2017; Su et al, 2020). Beyond inducing cell apoptosis, PM2.5 can also alter endothelial cell functions such as cell permeability, inflammation, and angiogenesis. In a tridimensional culture model, HUVECs exposed to PM2.5 showed an increased expression of the pro-inflammatory markers interleukin-1 (IL-1), Nuclear Factor Kappa-light-chain-enhancer of activated B cells (NF-κB), and intercellular adhesion molecule-1 (ICAM-1) (Y. Li et al, 2019). PM2.5 composed of organic carbon and element carbon were shown to increase vascular permeability in HUVECs (Long et al, 2020).
PM2.5 exposure can also slow down the process of autophagy and increase activity of the tumor suppressor gene p53 (TP53) in HUVECs (Liu et al, 2021; Wang et al, 2017). Autophagy is an important intracellular process that identifies and degrades unnecessary or dysfunctional proteins and organelles. Autophagy has protective functions against cytotoxic actions of microenvironmental stressors promoted by metabolic risk factors (e.g., high glucose; oxidized low density protein, ox-LDL) (Jiang, 2016). An overactivation of tumor suppressor protein 53 (TP53) in the endothelium can challenge cardiovascular homeostasis, making arteries more prone to atherosclerosis (Warboys et al, 2014). Angiogenesis, the formation of new capillaries from pre-existing ones, supports the expansion of the microvascular network to restore oxygen and nutrient supply to tissues during cardiac hypertrophy and after post-ischemic events (e.g., myocardial infarction, peripheral arterial diseases) (Li, Zhao, and Zhu, 2022; Annex and Cooke, 2021; Dorn, 2007; Sano et al, 2007). Some of the identified cellular effects of PM2.5 could alter the angiogenic process. For example, previous reports indicate that an increase in TP53 during ischemia limits angiogenesis during cardiac hypertrophy and in preclinical model of peripheral arterial diseases (Gogiraju et al, 2015; Pfaff et al, 2018).
Two toxicogenomic studies bring further evidence that PM2.5 could alter the angiogenic capacity of HUVECs (Hu et al, 2017; Jung et al, 2022). After 24 h of exposure to 50 μg/mL of urban airborne PM2.5, 501 genes were differentially regulated, 177 genes were downregulated and 417 genes were upregulated (Hu et al, 2017). In the second study, 24 h of exposure to PM2.5 originated from gasoline engine exhaustion (59.0 μg/mL) changed the expression of 1081 genes associated with cardiovascular system morphogenesis, vascular tube formation, receptor signaling (cytokine-related signaling), or cell adhesion (Jung et al, 2022). Both studies suggest that PM2.5 exposure could in fact alter important processes for angiogenesis such as morphogenesis, vascular tube formation, receptor signaling. Yet, many of the top differentially regulated genes differs between the two studies (Hu et al, 2017; Jung et al, 2022). The observed discrepancy might result from the difference in chemical compositions of the PM2.5. One study used PM2.5 originated for gasoline exhaustion (100%) (Jung et al, 2022), while the second versus has PM2.5 of mixed origin from both coal combustion and vehicle exhaustion (Hu et al, 2017; Y; Liu et al, 2021). Altogether, these endothelial cell alterations induced by PM2.5 and other pollutant nanoparticles could make the vasculature more vulnerable to cardiovascular risk factors and therefore, prone to atherosclerosis development.
Finally, if the observations made in HUVECs were all verified in MECs, it would be tangible that airborne PM could alter the biology of MECs (Figure 3). In conclusion, these in vitro studies have limitations: 1) PM with different chemical characteristics may elicit different responses in ECs. 2) The most extensively used endothelial cells to study air pollution are macrovascular HUVECs, with limited information on microvascular endothelial cells. 3) MECs have themselves a broad range of phenotypes depending on their tissue of origin, potentially eliciting different responses to environmental stressors (Aird, 2007a; Augustin and Koh, 2017).
3.5 Direct in vivo and ex vivo evidence that wildland fire smoke could impact the microvasculature
As discussed in the above section, in vitro studies have shown that PM could impair the structure and function of cultured endothelial cells. Yet, limited evidence exists regarding the direct impact of exposure to wildland fire smoke on the microvasculature per se (i.e., arterioles and capillaries). A few pre-clinical studies have investigated the impact of airborne pollution from vehicle exhaust and biomass burning on the mouse microvasculature in vivo, suggesting that exposure to wildland fire smoke or air pollution secondary to residential fire overhaul activity post residential fires could induce microvascular alterations (Gainey et al, 2018; Adivi et al, 2021; Scieszka et al, 2021).
Mice exposed to firefighting overhauling activity after a fire that modelled residential fire presented significant changes in the genes expressed within the pulmonary tissue (Gainey et al, 2018). While the authors essentially focused on identifying genes associated with lung carcinogenesis, the KEGG gene ontology analysis also revealed that exposure to the overhaul environment led to a significant over-representation of genes regulated by the Forkhead box O (FoxO) pathway (Gainey et al, 2018). We and others have shown that FoxO signaling pathway is crucial to microvascular homeostasis, where an increased activity of FoxO reduces the capacity of tissues to initiate angiogenesis in response to ischemia or in presence of cardiovascular risk factors (Paik et al, 2007; Milkiewicz et al, 2011; Roudier et al, 2013; Nwadozi et al, 2016; Rudnicki et al, 2018). Further investigations would need to confirm whether exposure to wildland fire smoke could elicit similar effects on the FoxO signaling pathway and impact the capacity of the microvasculature to remodel through angiogenesis.
In another study, Kim and colleagues have exposed mice to PM collected in the vicinity of peat bog fires that took place in the summer 2008 in the rural counties of North Carolina (Kim et al, 2014). Interestingly, the impact of these peat burning events on cardiovascular health was also investigated at the level of the general population by other research groups, and some positive associations between smoke and haze density and the risk of hospital admission for congestive heart failure were identified (Rappold A. G. et al, 2011; Rappold et al, 2012). In their animal study, Kim and colleagues used the Langendorff model to assess the impact of PM exposure on cardiac function. The Langedorff model enables a controlled ex vivo perfusion of the heart where ischemia-reperfusion is performed to mimic acute myocardial infarction. It has been previously demonstrated that microvascular alterations were part of the underlying mechanisms leading to ventricular dysfunction and myocardial infarction in the Langendorff model (Hollander et al, 2016). Using this model, Kim and colleagues have shown that female mice exposed to 154 ng/cm2 of inhaled ultrafine PM (≤0.1 μm) extracted from peat burning sites had greater infarct size and left ventricular dysfunction post-ischemia when compared to control mice (Kim et al, 2014). These results suggest that inhaling UFP and PM from peat burning smoke could increase the vulnerability of murine hearts to cardiac dysfunction following myocardial infarction, potentially due to greater microvascular injuries (Kim et al, 2014).
The impact of air pollution on the microvasculature has also been investigated at the cerebral level. The brain microvasculature is unique; astroglial cells surround endothelial cells to form a very tight barrier where the exchange of molecules is strictly controlled to protect neurons for toxic substances (Augustin and Koh, 2017). Exposure to vehicle exhaust PM altered the brain’s microvasculature integrity in Apo E−/− mice highly prone to atherosclerosis (Oppenheim et al, 2013; Adivi et al, 2021). The acquisition of a pro-inflammatory profile, an increased microvascular permeability and the associated changes in the expression of tight junction proteins could support the loss of brain microvascular integrity (Oppenheim et al, 2013; Adivi et al, 2021). Exposure to PM from wildland fire origin might also impact the brain microvasculature. Indeed, using a mobile laboratory, Scieszka and colleagues exposed healthy mice to concentrated PM2.5 (4 h/day over 20 days) from smoke of wildland fires naturally occurring in California, Arizona, and Washington states (Scieszka et al, 2021). In this study, mice were exposed to an average concentration of 104 μg/m3 of PM2.5. Animal exposed to these wildland fire related PM2.5 had an increased deposition of amyloid β (Aβ-42), a hallmark of cerebrovascular aging, an infiltration of peripheral immune cells, and a shift in the inflammatory profile of the cerebral microvascular endothelium (Scieszka et al, 2021). These changes were also associated to a greater coverage of capillaries by astroglial cells. Thus, PM2.5 originating from wildland fire might trigger neuroinflammation and an adverse remodeling of the microvascular blood brain barrier. Further investigations would be required to determine whether these alterations contribute to cerebrovascular aging (Figure 3).
The mentioned studies have investigated the acute or short-term effects of exposure to airborne pollutants from structural fires, biomass burning, and wildland fires. Further studies are however needed to elucidate whether repeated exposure to wildland fire smoke could initiate maladaptive responses of the microvasculature, potentially contributing to or worsening airborne pollution-driven CVD.
4 Remaining gaps of knowledge and future directions
Current evidence suggests that inhaled PM2.5 and UFP originated from biomass burning or wildland fire smokes could cross the gas-blood barrier to reach microvascular endothelia. Yet, gaps of knowledge remain, and further studies are required to delineate their biological impact on the microvasculature.
First, the chemical composition and size of PM varies depending on the fuel consumed (e.g., biomass vs. gasoline) and the conditions of combustion (Lighty, Veranth, and Sarofim, 2000). To date, most toxicology-based in vitro and animal-based in vivo studies have investigated the impact of PM from traffic pollution and vehicle exhaust, with only a few studies focusing on PM derived from wildland fire smoke or biomass burning. Most in vitro studies also utilized high-to-very high doses of PM to evaluate their toxicity, often far from doses that the general population would be exposed to.
Second, most in vitro studies were performed on macrovascular HUVECs, which are phenotypically very different from microvascular MECs (Aird, 2007a; 2007b; Nakato et al, 2019). In mammalians, the vast majority of the endothelia is microvascular (Wagenmakers et al, 2016). The response of MECs to wildland fire-related PM might be considerably different than the responses induced by urban-related PM measured in HUVECs (S. Chen et al, 2017). It appears now crucial to design in vitro studies that examine the toxicity of wildland fire-related PM directly on MECs.
Third, very limited data are available regarding the impact of PM exposure from wildland fires on the microvasculature per se in animal models. To our knowledge, only two studies have exposed mice to PM naturally collected from forest and peat wildfires (Kim et al, 2014; Scieszka et al, 2021).
Fourth, population-based studies revealed that individuals with conditions or diseases associated to microvascular alterations are more inclined to seek medical assistance due to the worsening of their health status (Johnston et al, 2014; 2007; Rappold A. G. et al, 2011; Rappold et al, 2012; Haikerwal et al, 2015; Yao et al, 2020; Mahsin, Cabaj, and Saini, 2021; Wettstein et al, 2022). Non-invasive assessments of the microvasculature emerge as innovative tools to stratify the cardiovascular risk at a population level. Despite this emergence of these new approaches (Adar et al, 2010; Louwies et al, 2013; Provost et al, 2017; Rijks et al, 2018; Luyten et al, 2020; Korsiak et al, 2021; Witters et al, 2021), the number of studies that investigated the direct impact of outdoor airborne pollution on human microvasculature in the context of wildland fires and biomass burning are insufficient.
In the future, it will be crucial that population-based studies inform the design of preclinical experimental studies to mimic real-world exposure as close as possible. To better determine the vulnerability of microvascular endothelium to wildland fire smoke exposure, experiments must take in consideration the doses of circulating PM, the chemical formulation of PM and their bioavailability in real-world conditions, and the duration of exposure (Lewtas, 2007; Thepnuan et al, 2020). Pre-clinical and clinical studies might help ascertaining whether microvascular alterations are accountable for the adverse cardiovascular outcomes observed in vulnerable populations. Verifying whether wildland fire-related PM directly causes microvascular alterations in these populations requires more intensive work. Undeniably, more population-based studies directly assessing the impact of wildland fire smoke on the microvasculature are needed. Yet, due to the phenotypic differences that exist within the microvasculature, it appears crucial to confirm whether non-invasive assessments of the cutaneous and retinal microvascular beds could correlate with other microvascular impairment observed in other tissues or organs after exposure to PM originated from wildland fire smoke. Careful design of pre-clinical animal studies where the impact of wildland fire PM is tested in multiple microvascular beds (e.g., brain, skeletal muscle, cardiac tissue, retina, and skin) might help in bridging these current gaps of knowledge.
5 Conclusive remarks
In a retrospective analysis of the wildland fire seasons 2013, 2015 and 2017–2018 from published Canadian studies (Crouse Dan L. et al, 2012; Stieb et al, 2020), Matz and colleagues estimated the impact on Canadians’ health due to short-term and long term-exposure to wildfire-PM2.5 (Matz et al, 2020). They concluded that, each year, there were 50–240 premature deaths due to acute health impact of wildland fire related PM2.5, and 570 to 2,500 premature deaths due to chronic health impact. This represents a significant burden on the Canadian health system estimated to cost $410M-$1.8B for the acute impact and $4.3B-$19B for chronic health impact. Most of these health-related costs were attributable to respiratory and cardiovascular conditions (Matz et al, 2020). With the anticipated expansion in size, severity, and number of wildland fires and the predicted lengthening of the fire seasons globally (Bowman et al, 2020; Touma et al, 2021; Senande-Rivera, Insua-Costa, and Miguez-Macho, 2022), better tools are required for cardiovascular health risk assessment. Exploring the biology of the microvasculature in this context could open new avenues for earlier and better detection of adverse cardiovascular outcomes posed by exposure to wildland fire smoke. This will improve knowledge regarding underlying mechanisms of wildland fires’ role in health and will pave the way for better risk management and prevention.
Author contributions
ER, NN, and CC have contributed to the conception or design of this review article. ER, NN, and CC have performed in depth analysis of the population studies. OB and ER have analysed the literature related to preclinical studies. TB provided information related to wildland fire smoke, particulate matter and climate change. ER and NN have drafted the first version of the manuscript. OB has generated the first versions of all figures. All authors contributed to writing, reviewing and editing the final version of the article and approved the submitted version.
Funding
Supported by the Natural Sciences and Engineering Research Council of Canada (NSERC) Discovery Grants Program, grant # RGPIN-2020-07142DG.
Conflict of interest
The authors declare that the research was conducted in the absence of any commercial or financial relationships that could be construed as a potential conflict of interest.
Publisher’s note
All claims expressed in this article are solely those of the authors and do not necessarily represent those of their affiliated organizations, or those of the publisher, the editors and the reviewers. Any product that may be evaluated in this article, or claim that may be made by its manufacturer, is not guaranteed or endorsed by the publisher.
References
Abe, M., Ono, J., Sato, Y., Okeda, T., and Takaki, R. (1990). Effects of glucose and insulin on cultured human microvascular endothelial cells. Diabetes Res. Clin. Pract. 9, 287–295. doi:10.1016/0168-8227(90)90058-2
Adar, S. D., Klein, R., Klein, B. E. K., Szpiro, A. A., Cotch, M. F., Wong, T. Y., et al. (2010). Air pollution and the microvasculature: A cross-sectional assessment of in vivo retinal images in the population-based multi-ethnic study of atherosclerosis (MESA). PLoS Med. 7, e1000372. doi:10.1371/journal.pmed.1000372
Adivi, A., Lucero, J., Simpson, N., McDonald, J. D., and Lund, A. K. (2021). Exposure to traffic-generated air pollution promotes alterations in the integrity of the brain microvasculature and inflammation in female ApoE-/- mice. Toxicol. Lett. 339, 39–50. doi:10.1016/j.toxlet.2020.12.016
Aird, W. C. (2007a). Phenotypic heterogeneity of the endothelium: I. Structure, function, and mechanisms. Circ. Res. 100, 158–173. doi:10.1161/01.RES.0000255691.76142.4a
Aird, W. C. (2007b). Phenotypic heterogeneity of the endothelium: II. Representative vascular beds. Circ. Res. 100, 174–190. doi:10.1161/01.RES.0000255690.03436.ae
Alam, S., Lang, J. J., Drucker, A. M., Gotay, C., Kozloff, N., et al. (2019). Assessment of the burden of diseases and injuries attributable to risk factors in Canada from 1990 to 2016: An analysis of the global burden of disease study. CMAJ Open 7, E140–E148. doi:10.9778/cmajo.20180137
Alman, B. L., Pfister, G., Hao, H., Stowell, J., Hu, X., Liu, Y., et al. (2016). The association of wildfire smoke with respiratory and cardiovascular emergency department visits in Colorado in 2012: A case crossover study. Environ. Health Glob. Access Sci. Source 15, 64. doi:10.1186/s12940-016-0146-8
Analitis, A., Georgiadis, I., and Katsouyanni, K. (2012). Forest fires are associated with elevated mortality in a dense urban setting. Occup. Environ. Med. 69, 158–162. doi:10.1136/oem.2010.064238
Anderson, T. J., Charbonneau, F., Title, L. M., Buithieu, J., Rose, M. S., Conradson, H., et al. (2011). Microvascular function predicts cardiovascular events in primary prevention: Long-term results from the firefighters and their endothelium (FATE) study. Circulation 123, 163–169. doi:10.1161/CIRCULATIONAHA.110.953653
Annex, B. H., and Cooke, J. P. (2021). New directions in therapeutic angiogenesis and arteriogenesis in peripheral arterial disease. Circ. Res. 128, 1944–1957. doi:10.1161/CIRCRESAHA.121.318266
Asher-Schapiro, A., and Sherfinski, D. (2021). U.S. firefighters on climate frontline face “broken” health system. Available at http://longreads.trust.org/item/USA-firefighters-climate-frontlines-broken-health-system.
Augustin, H. G., and Koh, G. Y. (2017). Organotypic vasculature: From descriptive heterogeneity to functional pathophysiology. Science 357, eaal2379. doi:10.1126/science.aal2379
Balch, J. K., Bradley, B. A., Abatzoglou, J. T., Nagy, R. C., Fusco, E. J., and Mahood, A. L. (2017). Human-started wildfires expand the fire niche across the United States. Proc. Natl. Acad. Sci. U. S. A. 114, 2946–2951. doi:10.1073/pnas.1617394114
Bao, H., Li, B., You, Q., Dun, X., Zhang, Z., et al. (2023). Exposure to real-ambient particulate matter induced vascular hypertrophy through activation of PDGFRβ. J. Hazard. Mat. 449, 130985. doi:10.1016/j.jhazmat.2023.130985
Barboni, T., Cannac, M., Leoni, E., and Chiaramonti, N. (2011). Emission of biogenic volatile organic compounds involved in eruptive fire: Implications for the safety of firefighters. Int. J. Wildland Fire 20, 152. doi:10.1071/WF08122
Barboni, T., Cannac, M., Pasqualini, V., Simeoni, A., Leoni, E., and Chiaramonti, N. (2010). Volatile and semi-volatile organic compounds in smoke exposure of firefighters during prescribed burning in the Mediterranean region. Int. J. Wildland Fire 19, 606. doi:10.1071/WF08121
Baxter, C. S., Hoffman, J. D., Knipp, M. J., Reponen, T., and Haynes, E. N. (2014). Exposure of firefighters to particulates and polycyclic aromatic hydrocarbons. J. Occup. Environ. Hyg. 11, D85–D91. doi:10.1080/15459624.2014.890286
Bearden, S. E. (2006). Effect of aging on the structure and function of skeletal muscle microvascular networks. Microcirculation 13, 279–288. doi:10.1080/10739680600618892
Bowman, D. M. J. S., Kolden, C. A., Abatzoglou, J. T., Johnston, F. H., van der Werf, G. R., and Flannigan, M. (2020). Vegetation fires in the anthropocene. Nat. Rev. Earth Environ. 1, 500–515. doi:10.1038/s43017-020-0085-3
Canadian Red Cross , (2023). Wildfires: Before, during & after - Canadian red cross. Available at: https://www.redcross.ca/how-we-help/emergencies-and-disasters-incanada/types-of-emergencies/wildfires (ONG Red Cross Canada 2023).
Cao, Y., Gong, Y., Liu, L., Zhou, Y., Fang, X., Zhang, C., et al. (2017). The use of human umbilical vein endothelial cells (HUVECs) as an in vitro model to assess the toxicity of nanoparticles to endothelium: A review. J. Appl. Toxicol. 37, 1359–1369. doi:10.1002/jat.3470
Cao, Y. (2018). The toxicity of nanoparticles to human endothelial cells. Adv. Exp. Med. Biol. 1048, 59–69. doi:10.1007/978-3-319-72041-8_4
Capitão, M., and Soares, R. (2016). Angiogenesis and inflammation crosstalk in diabetic retinopathy. J. Cell. Biochem. 117, 2443–2453. doi:10.1002/jcb.25575
Cascio, W. E. (2018). Wildland fire smoke and human health. Sci. Total Environ. 1, 586–595. doi:10.1016/j.scitotenv.2017.12.086
Cbs13/Cnn, (2020). California wildfires: Rain and cooler temperatures could help fire crews this week - CNN CBS News Sacram. URL https://www.cnn.com/2020/10/05/us/california-wildfires-monday-weather-relief/index.html.
Chang, K.-H., Park, J.-M., Lee, C. H., Kim, B., Choi, K.-C., Choi, S.-J., et al. (2017). NADPH oxidase (NOX) 1 mediates cigarette smoke-induced superoxide generation in rat vascular smooth muscle cells. Toxicol. Vitro Int. J. Publ. Assoc. BIBRA 38, 49–58. doi:10.1016/j.tiv.2016.10.013
Chen, H., Samet, J. M., Bromberg, P. A., and Tong, H. (2021). Cardiovascular health impacts of wildfire smoke exposure. Fibre Toxicol. 18, 2. doi:10.1186/s12989-020-00394-8
Chen, M., Liao, X., Yan, S.-C., Gao, Y., Yang, C., Song, Y., et al. (2020). Uptake, accumulation, and biomarkers of pm2.5-associated organophosphate flame retardants in C57bl/6 mice after chronic exposure at real environmental concentrations. Environ. Sci. Technol. 54, 9519–9528. doi:10.1021/acs.est.0c02237
Chen, S., Wu, X., Hu, J., Dai, G., Rong, A., and Guo, G. (2017). PM2.5 exposure decreases viability, migration and angiogenesis in human umbilical vein endothelial cells and human microvascular endothelial cells. Mol. Med. Rep. 16, 2425–2430. doi:10.3892/mmr.2017.6877
Chen, Z., Salam, M. T., Toledo-Corral, C., Watanabe, R. M., Xiang, A. H., Buchanan, T. A., et al. (2016). Ambient air pollutants have adverse effects on insulin and glucose homeostasis in Mexican Americans. Diabetes Care 39, 547–554. doi:10.2337/dc15-1795
Cherry, N., Galarneau, J.-M., Kinniburgh, D., Quemerais, B., Tiu, S., and Zhang, X. (2021). Exposure and absorption of PAHs in wildland firefighters: A field study with pilot interventions. Ann. Work Expo. Health 65, 148–161. doi:10.1093/annweh/wxaa064
Christensen, J. (2023). How to minimize the health risks from wildfire smoke. Available at: https://www.cnn.com/2023/06/07/health/wildfire-smoke-health-tips-wellness/index.html.
Coker, R. H., Murphy, C. J., Johannsen, M., Galvin, G., and Ruby, B. C. (2019). Wildland firefighting: Adverse influence on indices of metabolic and cardiovascular health. J. Occup. Environ. Med. 61, e91–e94. doi:10.1097/JOM.0000000000001535
Crea, F., Montone, R. A., and Rinaldi, R. (2021). Pathophysiology of coronary microvascular dysfunction. Circ. J. Off. J. Jpn. Circ. Soc. 86, 1319–1328. doi:10.1253/circj.CJ-21-0848
Crouse, D. L., Peters, P. A., van Donkelaar, A., Goldberg, M. S., Villeneuve, P. J., Brion, O., et al. (2012). Risk of nonaccidental and cardiovascular mortality in relation to long-term exposure to low concentrations of fine particulate matter: A Canadian national-level cohort study. Environ. Health Perspect. 120, 708–714. doi:10.1289/ehp.1104049
D’Amario, D., Borovac, J. A., and Crea, F. (2021). Coronary microvascular dysfunction in heart failure with preserved ejection fraction: Not the end but the end of the beginning. Eur. J. Heart Fail. 23, 773–775. doi:10.1002/ejhf.2069
D’Amario, D., Migliaro, S., Borovac, J. A., Restivo, A., Vergallo, R., Galli, M., et al. (2019). Microvascular dysfunction in heart failure with preserved ejection fraction. Front. Physiol. 10, 1347. doi:10.3389/fphys.2019.01347
Dean, J., Cruz, S. D., Mehta, P. K., and Merz, C. N. B. (2015). Coronary microvascular dysfunction: Sex-specific risk, diagnosis, and therapy. Nat. Rev. Cardiol. 12, 406–414. doi:10.1038/nrcardio.2015.72
Del Buono, M. G., Arena, R., Borlaug, B. A., Carbone, S., Canada, J. M., Kirkman, D. L., et al. (2019). Exercise intolerance in patients with heart failure: JACC state-of-the-art review. J. Am. Coll. Cardiol. 73, 2209–2225. doi:10.1016/j.jacc.2019.01.072
Delfino, R. J., Brummel, S., Wu, J., Stern, H., Ostro, B., Lipsett, M., et al. (2009). The relationship of respiratory and cardiovascular hospital admissions to the southern California wildfires of 2003. Occup. Environ. Med. Lond. 66, 189–197. doi:10.1136/oem.2008.041376
Ding, L., Sui, X., Yang, M., Zhang, Q., Sun, S., Zhu, F., et al. (2020). Toxicity of cooking oil fume derived particulate matter: Vitamin D3 protects tubule formation activation in human umbilical vein endothelial cells. Ecotoxicol. Environ. Saf. 188, 109905. doi:10.1016/j.ecoenv.2019.109905
Dora, K. A. (2016). Endothelial-smooth muscle cell interactions in the regulation of vascular tone in skeletal muscle. Microcirc. N. Y. N. 23, 626–630. doi:10.1111/micc.12322
Dorn, G. W. (2007). Myocardial angiogenesis: Its absence makes the growing heart founder. Cell Metab. 5, 326–327. doi:10.1016/j.cmet.2007.04.003
Doubleday, A., Schulte, J., Sheppard, L., Kadlec, M., Dhammapala, R., Fox, J., et al. (2020). Mortality associated with wildfire smoke exposure in Washington state, 2006-2017: A case-crossover study. Environ. Health Glob. Access Sci. Source 19, 4. doi:10.1186/s12940-020-0559-2
Farina, F., Lonati, E., Milani, C., Massimino, L., Ballarini, E., Donzelli, E., et al. (2019). In vivo comparative study on acute and sub-acute biological effects induced by ultrafine particles of different anthropogenic sources in BALB/c mice. Int. J. Mol. Sci. 20, 2805. doi:10.3390/ijms20112805
Finch, J., Riggs, D. W., O’Toole, T. E., Pope, C. A., Bhatnagar, A., and Conklin, D. J. (2019). Acute exposure to air pollution is associated with novel changes in blood levels of endothelin-1 and circulating angiogenic cells in young, healthy adults. AIMS Environ. Sci. 6, 265–276. doi:10.3934/environsci.2019.4.265
Fronek, K., and Zweifach, B. (1975). Microvascular pressure distribution in skeletal muscle and the effect of vasodilation. Am. J. Physiol.-Leg. Content 228, 791–796. doi:10.1152/ajplegacy.1975.228.3.791
Fuchs, D., Dupon, P. P., Schaap, L. A., and Draijer, R. (2017). The association between diabetes and dermal microvascular dysfunction non-invasively assessed by laser Doppler with local thermal hyperemia: A systematic review with meta-analysis. Cardiovasc. Diabetol. 16, 11. doi:10.1186/s12933-016-0487-1
Gainey, S. J., Horn, G. P., Towers, A. E., Oelschlager, M. L., Tir, V. L., Drnevich, J., et al. (2018). Exposure to a firefighting overhaul environment without respiratory protection increases immune dysregulation and lung disease risk. PloS One 13, e0201830. doi:10.1371/journal.pone.0201830
Gbd Compare Ihme Viz Hub, (2021). GBD compare | IHME viz hub. Available at: http://vizhub.healthdata.org/gbd-compare.
Gibbens, S., and McKeever, A. (2023). How wildfire smoke affects your body — and how you can protect yourself. Available at: https://www.nationalgeographic.com/science/article/how-breathing-wildfire-smoke-affects-the-body.
Gogiraju, R., Xu, X., Bochenek, M. L., Steinbrecher, J. H., Lehnart, S. E., Wenzel, P., et al. (2015). Endothelial p53 deletion improves angiogenesis and prevents cardiac fibrosis and heart failure induced by pressure overload in mice. J. Am. Heart Assoc. 4, e001770. doi:10.1161/JAHA.115.001770
Gu, Y., Xiao, Z. H., Wu, J., Guo, M., Lv, P., and Dou, N. (2021). Anti-atherosclerotic effect of afrocyclamin A against vascular smooth muscle cells is mediated via p38 mapk signaling pathway. Cell J. 23, 191–198. doi:10.22074/cellj.2021.7148
Guttenberg, M., Bezerra, L., Neu-Baker, N. M., del Pilar Sosa Peña, M., Elder, A., Oberdörster, G., et al. (2016). Biodistribution of inhaled metal oxide nanoparticles mimicking occupational exposure: A preliminary investigation using enhanced darkfield microscopy. J. Biophot. 9, 987–993. doi:10.1002/jbio.201600125
Haikerwal, A., Akram, M., Del Monaco, A., Smith, K., Sim, M. R., Meyer, M., et al. (2015). Impact of fine particulate matter (PM2.5) exposure during wildfires on cardiovascular health outcomes. J. Am. Heart Assoc. 4, e001653. doi:10.1161/JAHA.114.001653
Hameed, S., Pan, K., Su, W., Trupp, M., Mi, L., and Zhao, J. (2022). Label-free detection and quantification of ultrafine particulate matter in lung and heart of mouse and evaluation of tissue injury. Part. Fibre Toxicol. 19, 51. doi:10.1186/s12989-022-00493-8
Hanigan, I. C., Johnston, F. H., and Morgan, G. G. (2008). Vegetation fire smoke, indigenous status and cardio-respiratory hospital admissions in Darwin, Australia, 1996–2005: A time-series study. Environ. Health 7, 42–12. doi:10.1186/1476-069X-7-42
Hano, M. C., Prince, S. E., Wei, L., Hubbell, B. J., and Rappold, A. G. (2020). Knowing your audience: A typology of smoke sense participants to inform wildfire smoke health risk communication. Front. Public Health 8, 143. doi:10.3389/fpubh.2020.00143
He, J., Pang, Q., Huang, C., Xie, J., Hu, J., Wang, L., et al. (2021). Environmental dose of 16 priority-controlled PAHs mixture induce damages of vascular endothelial cells involved in oxidative stress and inflammation. Toxicol. Vitro Int. J. Publ. Assoc. BIBRA 79, 105296. doi:10.1016/j.tiv.2021.105296
Henderson, S. B., Brauer, M., Macnab, Y. C., and Kennedy, S. M. (2011). Three measures of forest fire smoke exposure and their associations with respiratory and cardiovascular health outcomes in a population-based cohort. Environ. Health Perspect. 119, 1266–1271. doi:10.1289/ehp.1002288
Hill, A. (2020). Opinion: Wildfires are increasingly destructive. We need to reevaluate how and where we build our homes | CNN's news. Available at: https://edition.cnn.com/2020/10/05/opinions/wildfires-prevention-climate-change-land-use-hill/index.html.
Ho, C.-C., Chen, Y.-C., Tsai, M.-H., Tsai, H.-T., Weng, C.-Y., Yet, S.-F., et al. (2021). Ambient particulate matter induces vascular smooth muscle cell phenotypic changes via NOX1/ROS/NF-κB dependent and independent pathways: Protective effects of polyphenols. Antioxid. Basel Switz. 10, 782. doi:10.3390/antiox10050782
Ho, C.-C., Wu, W.-T., Chen, Y.-C., Liou, S.-H., Yet, S.-F., Lee, C.-H., et al. (2019). Identification of osteopontin as a biomarker of human exposure to fine particulate matter. Environ. Pollut. Barking Essex 245, 975–985. doi:10.1016/j.envpol.2018.11.071
Hollander, M. R., Waard, G. A. de, Konijnenberg, L. S. F., Putten, R. M. E. M., Brom, C. E. van den, Paauw, N., et al. (2016). Dissecting the effects of ischemia and reperfusion on the coronary microcirculation in a rat model of acute myocardial infarction. PLOS ONE 11, e0157233. doi:10.1371/journal.pone.0157233
Hu, H., Asweto, C. O., Wu, J., Shi, Y., Feng, L., Yang, X., et al. (2017). Gene expression profiles and bioinformatics analysis of human umbilical vein endothelial cells exposed to PM2.5. Chemosphere 183, 589–598. doi:10.1016/j.chemosphere.2017.05.153
Hutchinson, J. A., Vargo, J., Milet, M., French, N. H. F., Billmire, M., Johnson, J., et al. (2018). The San Diego 2007 wildfires and Medi-Cal emergency department presentations, inpatient hospitalizations, and outpatient visits: An observational study of smoke exposure periods and a bidirectional case-crossover analysis. PLoS Med. Public Libr. Sci. 15, e1002601. doi:10.1371/journal.pmed.1002601
Jackson, W. F. (2021). Myogenic tone in peripheral resistance arteries and arterioles: The pressure is on. Front. Physiol. 12, 699517. doi:10.3389/fphys.2021.699517
Jain, P., Wang, X., and Flannigan, M. D. (2018). Trend analysis of fire season length and extreme fire weather in North America between 1979 and 2015. Int. J. Wildland Fire 26, 1009–1020. doi:10.1071/WF17008
Jiang, F. (2016). Autophagy in vascular endothelial cells. Clin. Exp. Pharmacol. Physiol. 43, 1021–1028. doi:10.1111/1440-1681.12649
Johnston, F. H., Bailie, R. S., Pilotto, L. S., and Hanigan, I. C. (2007). Ambient biomass smoke and cardio-respiratory hospital admissions in Darwin, Australia. BMC Public Health 7, 240–248. doi:10.1186/1471-2458-7-240
Johnston, F. H., Purdie, S., Jalaludin, B., Martin, K. L., Henderson, S. B., and Morgan, G. G. (2014). Air pollution events from forest fires and emergency department attendances in sydney, Australia 1996-2007: A case-crossover analysis. Environ. Health Glob. Access Sci. Source 1, 105. doi:10.1186/1476-069X-13-105
Ju, S., Lim, L., Ki, Y.-J., Choi, D.-H., and Song, H. (2022). Oxidative stress generated by polycyclic aromatic hydrocarbons from ambient particulate matter enhance vascular smooth muscle cell migration through MMP upregulation and actin reorganization. Part. Fibre Toxicol. 19, 29. doi:10.1186/s12989-022-00472-z
Jung, I., Park, M., Jeong, M.-H., Park, K., Kim, W.-H., and Kim, G.-Y. (2022). Transcriptional analysis of gasoline engine exhaust particulate matter 2.5-exposed human umbilical vein endothelial cells reveals the different gene expression patterns related to the cardiovascular diseases. Biochem. Biophys. Rep. 29, 101190. doi:10.1016/j.bbrep.2021.101190
Kim, Y. H., Tong, H., Daniels, M., Boykin, E., Krantz, Q. T., McGee, J., et al. (2014). Cardiopulmonary toxicity of peat wildfire particulate matter and the predictive utility of precision cut lung slices. Fibre Toxicol. Electron. Resour. 1, 29. doi:10.1186/1743-8977-11-29
Kitzman, D. W., Nicklas, B., Kraus, W. E., Lyles, M. F., Eggebeen, J., Morgan, T. M., et al. (2014). Skeletal muscle abnormalities and exercise intolerance in older patients with heart failure and preserved ejection fraction. Am. J. Physiol. Heart Circ. Physiol. 306, H1364–H1370. doi:10.1152/ajpheart.00004.2014
Kollanus, V., Tiittanen, P., Niemi, J. V., and Lanki, T. (2016). Effects of long-range transported air pollution from vegetation fires on daily mortality and hospital admissions in the Helsinki metropolitan area, Finland. Environ. Res. 1, 351–358. doi:10.1016/j.envres.2016.08.003
Korsiak, J., Perepeluk, K.-L., Peterson, N. G., Kulka, R., and Weichenthal, S. (2021). Air pollution and retinal vessel diameter and blood pressure in school-aged children in a region impacted by residential biomass burning. Sci. Rep. 11, 12790. doi:10.1038/s41598-021-92269-x
Kozlov, M. (2021). How record wildfires are harming human health. Nature 599, 550–552. doi:10.1038/d41586-021-03496-1
Lähteenvuo, J., and Rosenzweig, A. (2012). Effects of aging on angiogenesis. Circ. Res. 110, 1252–1264. doi:10.1161/CIRCRESAHA.111.246116
Lang, I., Pabst, M. A., Hiden, U., Blaschitz, A., Dohr, G., Hahn, T., et al. (2003). Heterogeneity of microvascular endothelial cells isolated from human term placenta and macrovascular umbilical vein endothelial cells. Eur. J. Cell Biol. 82, 163–173. doi:10.1078/0171-9335-00306
Lapid, N. (2023). What are the health risks from wildfire smoke? Reuters. https://www.reuters.com/world/americas/what-are-health-risks-wildfire-smoke-2023-06-07/#:∼:text=Wildfires%20have%20also%20been%20linked,years%2C%20researchers%20reported%20in%20April.
Larsen, A., Hanigan, I., Reich, B. J., Qin, Y., Cope, M., Morgan, G., et al. (2020). A deep learning approach to identify smoke plumes in satellite imagery in near-real time for health risk communication. J. Expo. Sci. Environ. Epidemiol. 31, 170–176. doi:10.1038/s41370-020-0246-y
Lau, S., Gossen, M., Lendlein, A., and Jung, F. (2021). Venous and arterial endothelial cells from human umbilical cords: Potential cell sources for cardiovascular research. Int. J. Mol. Sci. 22, 978. doi:10.3390/ijms22020978
LeBlanc, A., Cumpston, J., Chen, B., Frazer, D., Castranova, V., and Nurkiewicz, T. (2009). Nanoparticle inhalation impairs endothelium-dependent vasodilation in subepicardial arterioles. J. Toxicol. Environ. Health A 72, 1576–1584. doi:10.1080/15287390903232467
LeBlanc, A. J., and Hoying, J. B. (2016). Adaptation of the coronary microcirculation in aging. Microcirculation 23, 157–167. doi:10.1111/micc.12264
Lee, V., Martin, B.-J., Fung, M., and Anderson, T. J. (2012). The optimal measure of microvascular function with velocity time integral for cardiovascular risk prediction. Vasc. Med. Lond. Engl. 17, 287–293. doi:10.1177/1358863X12451337
Lewis, D. (2020). ‘Deathly silent’: Ecologist describes Australian wildfires’ devastating aftermath. Nature 577, 304. doi:10.1038/d41586-020-00043-2
Lewtas, J. (2007). Air pollution combustion emissions: Characterization of causative agents and mechanisms associated with cancer, reproductive, and cardiovascular effects. Mutat. Res. Mutat. Res., Sources Potential Hazards Mutagens Complex Environ. Matrices - Part II 636, 95–133. doi:10.1016/j.mrrev.2007.08.003
Li, J., Zhao, Y., and Zhu, W. (2022). Targeting angiogenesis in myocardial infarction: Novel therapeutics (Review). Exp. Ther. Med. 23, 64. doi:10.3892/etm.2021.10986
Li, R., Mittelstein, D., Kam, W., Pakbin, P., Du, Y., Tintut, Y., et al. (2013). Atmospheric ultrafine particles promote vascular calcification via the NF-κB signaling pathway. Am. J. Physiol. Cell Physiol. 304, C362–C369. doi:10.1152/ajpcell.00322.2012
Li, Y., Wu, Y., Liu, Y., Deng, Q.-H., Mak, M., and Yang, X. (2019). Atmospheric nanoparticles affect vascular function using a 3D human vascularized organotypic chip. Nanoscale 11, 15537–15549. doi:10.1039/c9nr03622a
Lighty, J. S., Veranth, J. M., and Sarofim, A. F. (2000). Combustion aerosols: Factors governing their size and composition and implications to human health. J. Air Waste Manag. Assoc. 50, 1565–1618. doi:10.1080/10473289.2000.10464197
Liu, J. C., Wilson, A., Mickley, L. J., Dominici, F., Ebisu, K., Wang, Y., et al. (2017). Wildfire-specific fine particulate matter and risk of hospital admissions in urban and rural counties. Epidemiology 28, 77–85. doi:10.1097/EDE.0000000000000556
Liu, Y., Zhao, D., Peng, W., Xue, P., Jiang, X., Chen, S., et al. (2021). Atmospheric PM2.5 blocking up autophagic flux in HUVECs via inhibiting Sntaxin-17 and LAMP2. Ecotoxicol. Environ. Saf. 208, 111450. doi:10.1016/j.ecoenv.2020.111450
Loai, S., Sun, X., Husain, M., Laflamme, M. A., Yeger, H., Nunes, S. S., et al. (2022). Microvascular dysfunction in skeletal muscle precedes myocardial vascular changes in diabetic cardiomyopathy: Sex-dependent differences. Front. Cardiovasc. Med. 9, 886687. doi:10.3389/fcvm.2022.886687
Long, Y.-M., Yang, X.-Z., Yang, Q.-Q., Clermont, A. C., Yin, Y.-G., Liu, G.-L., et al. (2020). PM2.5 induces vascular permeability increase through activating MAPK/ERK signaling pathway and ROS generation. J. Hazard. Mat. 386, 121659. doi:10.1016/j.jhazmat.2019.121659
Louwies, T., Panis, L. I., Kicinski, M., De, B. P., and Nawrot, T. S. (2013). Retinal microvascular responses to short-term changes in particulate air pollution in healthy adults. Environ. Health Perspect. 121, 1011–1016. doi:10.1289/ehp.1205721
Luyten, L. J., Dockx, Y., Provost, E. B., Madhloum, N., Sleurs, H., Neven, K. Y., et al. (2020). Children’s microvascular traits and ambient air pollution exposure during pregnancy and early childhood: Prospective evidence to elucidate the developmental origin of particle-induced disease. BMC Med. 18, 128. doi:10.1186/s12916-020-01586-x
Mahfouz, R. A., Gouda, M., and Abdelhamid, M. (2020). Relation of microvascular dysfunction and exercise tolerance in patients with heart failure with preserved ejection fraction. Echocardiography 37, 1192–1198. doi:10.1111/echo.14799
Mahsin, M. D., Cabaj, J., and Saini, V. (2021). Respiratory and cardiovascular condition-related physician visits associated with wildfire smoke exposure in calgary, Canada, in 2015: A population-based study. Int. J. Epidemiol. dyab206 51, 166–178. doi:10.1093/ije/dyab206
Marfori, M. T., Campbell, S. L., Garvey, K., McKeown, S., Veitch, M., Wheeler, A. J., et al. (2020). Public health messaging during extreme smoke events: Are we hitting the mark? Front. Public Health 8, 465. doi:10.3389/fpubh.2020.00465
Margaux, L. (2023). Feux de forêt au Canada: Un risque majeur pour la santé ? URL https://www.liberation.fr/environnement/climat/feux-de-foret-au-canada-un-risque-majeur-pour-la-sante-20230609_OYWHQSJYE5EKBM2LAV3HNTDWA4/.
Marrone, E., Longo, C., O’Loughlin, J., Villeneuve, P. J., Zappitelli, M., Bartlett-Esquilant, G., et al. (2023). Association between annual exposure to air pollution and systolic blood pressure among adolescents in Montréal, Canada. Chronic Dis. Prev. Can. Res. Policy Pract. 43, 191–198. doi:10.24095/hpcdp.43.4.04
Martin, K. L., Hanigan, I. C., Morgan, G. G., Henderson, S. B., and Johnston, F. H. (2013). Air pollution from bushfires and their association with hospital admissions in Sydney, Newcastle and Wollongong, Australia 1994–2007. Aust. N. Z. J. Public Health 37, 238–243. doi:10.1111/1753-6405.12065
Matz, C. J., Egyed, M., Xi, G., Racine, J., Pavlovic, R., Rittmaster, R., et al. (2020). Health impact analysis of PM2.5 from wildfire smoke in Canada (2013-2015, 2017-2018). Sci. Total Environ. 725, 138506. doi:10.1016/j.scitotenv.2020.138506
McFall-Johnsen, M. (2020b). Wildfire smoke could leave lungs vulnerable to COVID-19 and the flu, warn scientists: Science alert. Available at: https://www.sciencealert.com/all-this-wildfire-smoke-is-going-to-leave-lungs-vulnerable-to-the-flu-and-covid-19.
McFall-Johnsen, M. (2020a). Wildfire smoke likely to make flu and coronavirus worse in fall - business Insider. Available at: https://www.businessinsider.com/wildfire-smoke-makes-flu-coronavirus-worse-2020-9.
Mega, E. R. (2020). ‘Apocalyptic’ fires are ravaging the world’s largest tropical wetland. Nature 586, 20–21. doi:10.1038/d41586-020-02716-4
Mehta, P. K., Quesada, O., Al-Badri, A., Fleg, J. L., Volgman, A. S., Pepine, C. J., et al. (2022). Ischemia and no obstructive coronary arteries in patients with stable ischemic heart disease. Int. J. Cardiol. 348, 1–8. doi:10.1016/j.ijcard.2021.12.013
Meng, J., Martin, R. V., Li, C., van Donkelaar, A., Tzompa-Sosa, Z. A., Yue, X., et al. (2019). Source contributions to ambient fine particulate matter for Canada. Environ. Sci. Technol. 53, 10269–10278. doi:10.1021/acs.est.9b02461
Migliaccio, C. T. (2023). How wildfire smoke can threaten human health, even when the fire is hundreds of miles away. Available at: https://www.pbs.org/newshour/science/how-wildfire-smoke-can-threaten-human-health-even-when-thefire-is-hundreds-of-miles-away.
Milkiewicz, M., Roudier, E., Doyle, J. L., Trifonova, A., Birot, O., and Haas, T. L. (2011). Identification of a mechanism underlying regulation of the anti-angiogenic forkhead transcription factor FoxO1 in cultured endothelial cells and ischemic muscle. Am. J. Pathol. 178, 935–944. doi:10.1016/j.ajpath.2010.10.042
Miller, K. (2023). EPA and doctors say wildfire smoke can cause these health risks. Available at https://www.prevention.com/health/a44122630/health-effects-wildfire-smoke/.
Miller, M. R., Raftis, J. B., Langrish, J. P., McLean, S. G., Samutrtai, P., Connell, S. P., et al. (2017). Inhaled nanoparticles accumulate at sites of vascular disease. ACS Nano 11, 4542–4552. doi:10.1021/acsnano.6b08551
Ming, Y., Liu, G., Li, J., Lai, H., Wang, C., and Zhu, K. (2022). Adverse effects of ambient fine particulate matter (PM2.5) on vascular smooth muscle cells. J. Appl. Toxicol. JAT. 43, 1108–1118. doi:10.1002/jat.4418
Murrant, C. L., and Sarelius, I. H. (2015). Local control of blood flow during active hyperaemia: What kinds of integration are important? J. Physiol. 593, 4699–4711. doi:10.1113/JP270205
Mutlu, U., Ikram, M. K., Wolters, F. J., Hofman, A., Klaver, C. C. W., and Ikram, M. A. (2016). Retinal microvasculature is associated with long-term survival in the general adult Dutch population. Hypertension 67, 281–287. doi:10.1161/HYPERTENSIONAHA.115.06619
Nakato, R., Wada, Y., Nakaki, R., Nagae, G., Katou, Y., Tsutsumi, S., et al. (2019). Comprehensive epigenome characterization reveals diverse transcriptional regulation across human vascular endothelial cells. Epigenetics Chromatin 12, 77. doi:10.1186/s13072-019-0319-0
Navarro, K. M., Cisneros, R., Noth, E. M., Balmes, J. R., and Hammond, S. K. (2017). Occupational exposure to polycyclic aromatic hydrocarbon of wildland firefighters at prescribed and wildland fires. Environ. Sci. Technol. 51, 6461–6469. doi:10.1021/acs.est.7b00950
Navarro, K. M., Cisneros, R., Schweizer, D., Chowdhary, P., Noth, E. M., Balmes, J. R., et al. (2019). Incident command post exposure to polycyclic aromatic hydrocarbons and particulate matter during a wildfire. J. Occup. Environ. Hyg. 16, 735–744. doi:10.1080/15459624.2019.1657579
Nemmar, A., Hoet, P. H. M., Vanquickenborne, B., Dinsdale, D., Thomeer, M., Hoylaerts, M. F., et al. (2002). Passage of inhaled particles into the blood circulation in humans. Circulation 105, 411–414. doi:10.1161/hc0402.104118
North, M. P., Stephens, S. L., Collins, B. M., Agee, J. K., Aplet, G., Franklin, J. F., et al. (2015). ENVIRONMENTAL SCIENCE. Reform forest fire management. Science 349, 1280–1281. doi:10.1126/science.aab2356
Nurkiewicz, T. R., Porter, D. W., Barger, M., Castranova, V., and Boegehold, M. A. (2004). Particulate matter exposure impairs systemic microvascular endothelium-dependent dilation. Environ. Health Perspect. 112, 1299–1306. doi:10.1289/ehp.7001
Nurkiewicz, T. R., Porter, D. W., Hubbs, A. F., Cumpston, J. L., Chen, B. T., Frazer, D. G., et al. (2008). Nanoparticle inhalation augments particle-dependent systemic microvascular dysfunction. Part. Fibre Toxicol. 5, 1. doi:10.1186/1743-8977-5-1
Nurkiewicz, T. R., Porter, D. W., Hubbs, A. F., Stone, S., Moseley, A. M., Cumpston, J. L., et al. (2011). Pulmonary particulate matter and systemic microvascular dysfunction. Res. Rep. Health Eff. Inst. 164, 3–48.
Nwadozi, E., Roudier, E., Rullman, E., Tharmalingam, S., Liu, H.-Y., Gustafsson, T., et al. (2016). Endothelial FoxO proteins impair insulin sensitivity and restrain muscle angiogenesis in response to a high-fat diet. FASEB J. Off. Publ. Fed. Am. Soc. Exp. Biol. 30, 3039–3052. doi:10.1096/fj.201600245R
Oberdörster, G. (1995). Lung particle overload: Implications for occupational exposures to particles. Regul. Toxicol. Pharmacol. RTP 21, 123–135. doi:10.1006/rtph.1995.1017
Oppenheim, H. A., Lucero, J., Guyot, A.-C., Herbert, L. M., McDonald, J. D., Mabondzo, A., et al. (2013). Exposure to vehicle emissions results in altered blood brain barrier permeability and expression of matrix metalloproteinases and tight junction proteins in mice. Part. Fibre Toxicol. 10, 62. doi:10.1186/1743-8977-10-62
Paik, J.-H., Kollipara, R., Chu, G., Ji, H., Xiao, Y., Ding, Z., et al. (2007). FoxOs are lineage-restricted redundant tumor suppressors and regulate endothelial cell homeostasis. Cell 128, 309–323. doi:10.1016/j.cell.2006.12.029
Park, J. H., Choi, J.-Y., Lee, H.-K., Jo, C., and Koh, Y. H. (2021). Notch1-mediated inflammation is associated with endothelial dysfunction in human brain microvascular endothelial cells upon particulate matter exposure. Arch. Toxicol. 95, 529–540. doi:10.1007/s00204-020-02942-9
Peterson, G. C. L., Prince, S. E., and Rappold, A. G. (2021). Trends in fire danger and population exposure along the wildland-urban interface. Environ. Sci. Technol. 55, 16257–16265. doi:10.1021/acs.est.1c03835
Pfaff, M. J., Mukhopadhyay, S., Hoofnagle, M., Chabasse, C., and Sarkar, R. (2018). Tumor suppressor protein p53 negatively regulates ischemia-induced angiogenesis and arteriogenesis. J. Vasc. Surg. 68, 222S–233S.e1. doi:10.1016/j.jvs.2018.02.055
Pope, C. A., Bhatnagar, A., McCracken, J. P., Abplanalp, W., Conklin, D. J., and O’Tool, T. (2016). Exposure to fine particulate air pollution is associated with endothelial injury and systemic inflammation. Circ. Res. 119, 1204–1214. doi:10.1161/CIRCRESAHA.116.309279
Provost, E. B., Int Panis, L., Saenen, N. D., Kicinski, M., Louwies, T., Vrijens, K., et al. (2017). Recent versus chronic fine particulate air pollution exposure as determinant of the retinal microvasculature in school children. Environ. Res. 159, 103–110. doi:10.1016/j.envres.2017.07.027
Raftis, J. B., and Miller, M. R. (2019). Nanoparticle translocation and multi-organ toxicity: A particularly small problem. Nano Today 26, 8–12. doi:10.1016/j.nantod.2019.03.010
Rappold, A. G., Cascio, W. E., Kilaru, V. J., Stone, S. L., Neas, L. M., Devlin, R. B., et al. (2012). Cardio-respiratory outcomes associated with exposure to wildfire smoke are modified by measures of community health. Environ. Health Glob. Access Sci. Source 11, 71. doi:10.1186/1476-069X-11-71
Rappold, A. G., Stone, S. L., Cascio, W. E., Neas, L. M., Kilaru, V. J., Carraway, M. S., et al. (2011). Peat bog wildfire smoke exposure in rural North Carolina is associated with cardiopulmonary emergency department visits assessed through syndromic surveillance. Environ. Health Perspect. 119, 1415–1420. doi:10.1289/ehp.1003206
Reid, C. E., Brauer, M., Johnston, F. H., Jerrett, M., Balmes, J. R., and Elliott, C. T. (2016). Critical review of health impacts of wildfire smoke exposure. Environ. Health Perspect. 124, 1334–1343. doi:10.1289/ehp.1409277
Rijks, J., Vreugdenhil, A., Dorenbos, E., Karnebeek, K., Joris, P., Berendschot, T., et al. (2018). Characteristics of the retinal microvasculature in association with cardiovascular risk markers in children with overweight, obesity and morbid obesity. Sci. Rep. 8, 16952. doi:10.1038/s41598-018-35279-6
Romagnoli, E., Barboni, T., Santoni, P.-A., and Chiaramonti, N. (2014). Quantification of volatile organic compounds in smoke from prescribed burning and comparison with occupational exposure limits. Nat. Hazards Earth Syst. Sci. 14, 1049–1057. doi:10.5194/nhess-14-1049-2014
Roudier, E., Milkiewicz, M., Birot, O., Slopack, D., Montelius, A., Gustafsson, T., et al. (2013). Endothelial FoxO1 is an intrinsic regulator of thrombospondin 1 expression that restrains angiogenesis in ischemic muscle. Angiogenesis 16, 759–772. doi:10.1007/s10456-013-9353-x
Rudnicki, M., Abdifarkosh, G., Nwadozi, E., Ramos, S. V., Makki, A., Sepa-Kishi, D. M., et al. (2018). Endothelial-specific FoxO1 depletion prevents obesity-related disorders by increasing vascular metabolism and growth. eLife 7, e39780. doi:10.7554/eLife.39780
Sano, M., Minamino, T., Toko, H., Miyauchi, H., Orimo, M., Qin, Y., et al. (2007). p53-induced inhibition of Hif-1 causes cardiac dysfunction during pressure overload. Nature 446, 444–448. doi:10.1038/nature05602
Santin, C., and Doerr, S. (2022). Global trends in wildfire and its impacts | Royal Society. Available at: https://royalsociety.org/blog/2020/10/global-trends-wildfire/.
Schneider, S. R., Lee, K., Santos, G., and Abbatt, J. P. D. (2021). Air quality data approach for defining wildfire influence: Impacts on PM2.5, NO2, CO, and O3 in western Canadian cities. Environ. Sci. Technol. 55, 13709–13717. doi:10.1021/acs.est.1c04042
Schroeter, M. R., Sawalich, M., Humboldt, T., Leifheit, M., Meurrens, K., Berges, A., et al. (2008). Cigarette smoke exposure promotes arterial thrombosis and vessel remodeling after vascular injury in apolipoprotein E-deficient mice. J. Vasc. Res. 45, 480–492. doi:10.1159/000127439
Scieszka, D., Hunter, R., Begay, J., Bitsui, M., Lin, Y., Galewsky, J., et al. (2021). Neuroinflammatory and neurometabolomic consequences from inhaled wildfire smoke-derived particulate matter in the Western United States. Toxicol. Sci. Off. J. Soc. Toxicol. Kfab147. 186, 149–162. doi:10.1093/toxsci/kfab147
Seidelmann, S. B., Claggett, B., Bravo, P. E., Gupta, A., Farhad, H., Klein, B. E., et al. (2016). Retinal vessel calibers in predicting long-term cardiovascular outcomes: The atherosclerosis risk in communities study. Circulation 134, 1328–1338. doi:10.1161/CIRCULATIONAHA.116.023425
Senande-Rivera, M., Insua-Costa, D., and Miguez-Macho, G. (2022). Spatial and temporal expansion of global wildland fire activity in response to climate change. Nat. Commun. 13, 1208. doi:10.1038/s41467-022-28835-2
Setyawati, M. I., Tay, C. Y., Docter, D., Stauber, R. H., and Leong, D. T. (2015). Understanding and exploiting nanoparticles’ intimacy with the blood vessel and blood. Chem. Soc. Rev. 44, 8174–8199. doi:10.1039/C5CS00499C
Shi, Y., and Vanhoutte, P. M. (2017). Macro- and microvascular endothelial dysfunction in diabetes. J. Diabetes 9, 434–449. doi:10.1111/1753-0407.12521
Sörensen, B. M., Houben, A. J. H. M., Berendschot, T. T. J. M., Schouten, J. S. A. G., Kroon, A. A., van der Kallen, C. J. H., et al. (2016). Prediabetes and type 2 diabetes are associated with generalized microvascular dysfunction: The maastricht study. Circulation 134, 1339–1352. doi:10.1161/CIRCULATIONAHA.116.023446
Soteriades, E. S., Smith, D. L., Tsismenakis, A. J., Baur, D. M., and Kales, S. N. (2011). Cardiovascular disease in US firefighters: A systematic review. Cardiol. Rev. 19, 202–215. doi:10.1097/CRD.0b013e318215c105
Stehouwer, C. D. A. (2018). Microvascular dysfunction and hyperglycemia: A vicious cycle with widespread consequences. Diabetes 67, 1729–1741. doi:10.2337/dbi17-0044
Stieb, D. M., Evans, G. J., To, T. M., Brook, J. R., and Burnett, R. T. (2020). An ecological analysis of long-term exposure to PM2.5 and incidence of COVID-19 in Canadian health regions. Environ. Res. 191, 110052. doi:10.1016/j.envres.2020.110052
Su, R., Jin, X., Li, H., Huang, L., and Li, Z. (2020). The mechanisms of PM2.5 and its main components penetrate into HUVEC cells and effects on cell organelles. Chemosphere 241, 125127. doi:10.1016/j.chemosphere.2019.125127
Suphasiriroj, W., Mikami, M., and Sato, S. (2013). Comparative studies on microvascular endothelial cells isolated from periodontal tissue. J. Periodontol. 84, 1002–1009. doi:10.1902/jop.2012.120453
Thepnuan, D., Yabueng, N., Chantara, S., Prapamontol, T., and Tsai, Y. I. (2020). Simultaneous determination of carcinogenic PAHs and levoglucosan bound to PM2.5 for assessment of health risk and pollution sources during a smoke haze period. Chemosphere 257, 127154. doi:10.1016/j.chemosphere.2020.127154
Tian, M., Zhao, J., Mi, X., Wang, K., Kong, D., Mao, H., et al. (2021). Progress in research on effect of PM2.5 on occurrence and development of atherosclerosis. J. Appl. Toxicol. JAT 41, 668–682. doi:10.1002/jat.4110
Touma, D., Stevenson, S., Lehner, F., and Coats, S. (2021). Human-driven greenhouse gas and aerosol emissions cause distinct regional impacts on extreme fire weather. Nat. Commun. 12, 212. doi:10.1038/s41467-020-20570-w
van de Hoef, T. P., Echavarria-Pinto, M., Meuwissen, M., Stegehuis, V. E., Escaned, J., and Piek, J. J. (2020). Contribution of age-related microvascular dysfunction to abnormal coronary: Hemodynamics in patients with ischemic heart disease. JACC Cardiovasc. Interv. 13, 20–29. doi:10.1016/j.jcin.2019.08.052
van Sloten, T. T., Sedaghat, S., Carnethon, M. R., Launer, L. J., and Stehouwer, C. D. A. (2020). Cerebral microvascular complications of type 2 diabetes: Stroke, cognitive dysfunction, and depression. Lancet Diabetes Endocrinol. 8, 325–336. doi:10.1016/S2213-8587(19)30405-X
Wagenmakers, A. J. M., Strauss, J. A., Shepherd, S. O., Keske, M. A., and Cocks, M. (2016). Increased muscle blood supply and transendothelial nutrient and insulin transport induced by food intake and exercise: Effect of obesity and ageing. J. Physiol. 594, 2207–2222. doi:10.1113/jphysiol.2014.284513
Wan, Q., Liu, Z., and Yang, Y. (2018). Puerarin inhibits vascular smooth muscle cells proliferation induced by fine particulate matter via suppressing of the p38 MAPK signaling pathway. BMC Complement. Altern. Med. 18, 146. doi:10.1186/s12906-018-2206-9
Wang, W., Deng, Z., Feng, Y., Liao, F., Zhou, F., Feng, S., et al. (2017). PM2.5 induced apoptosis in endothelial cell through the activation of the p53-bax-caspase pathway. Chemosphere 177, 135–143. doi:10.1016/j.chemosphere.2017.02.144
Warboys, C. M., de Luca, A., Amini, N., Luong, L., Duckles, H., Hsiao, S., et al. (2014). Disturbed flow promotes endothelial senescence via a p53-dependent pathway. Arterioscler. Thromb. Vasc. Biol. 34, 985–995. doi:10.1161/ATVBAHA.114.303415
Weber, C. (2020). ‘Stubborn’ California wildfire fueled by lack of firefighting resources: Officials - national | Globalnews.ca. News. Global news. Available at: https://globalnews.ca/news/7352445/california-bobcat-wildfire-spread/.
Wen, T., Liao, D., Wellenius, G. A., Whitsel, E. A., Margolis, H. G., Tinker, L. F., et al. (2023). Short-term air pollution levels and blood pressure in older women. Epidemiol. Camb. Mass 34, 271–281. doi:10.1097/EDE.0000000000001577
Wettstein, Z. S., Hoshiko, S., Fahimi, J., Harrison, R. J., Cascio, W. E., and Rappold, A. G. (2022). Cardiovascular and cerebrovascular emergency department visits associated with wildfire smoke exposure in California in 2015. J. Am. Heart Assoc. 7, e007492. doi:10.1161/JAHA.117.007492
Who’s Air Quality and Health Unit, (2022). Ambient (outdoor) air pollution. Available at: https://www.who.int/news-room/fact-sheets/detail/ambient-(outdoor)-air-quality-and-health.
Witters, K., Dockx, Y., Op’t Roodt, J., Lefebvre, W., Vanpoucke, C., Plusquin, M., et al. (2021). Dynamics of skin microvascular blood flow in 4-6-year-old children in association with pre- and postnatal black carbon and particulate air pollution exposure. Environ. Int. 157, 106799. doi:10.1016/j.envint.2021.106799
Witze, A. (2020). The arctic is burning like never before — And that’s bad news for climate change. Nature 585, 336–337. doi:10.1038/d41586-020-02568-y
Xu, R., Yu, P., Abramson, M. J., Johnston, F. H., Samet, J. M., Bell, M. L., et al. (2020). Wildfires, global climate change, and human health. N. Engl. J. Med. 383, 2173–2181. doi:10.1056/NEJMsr2028985
Yao, J., Brauer, M., Wei, J., McGrail, K. M., Johnston, F. H., and Henderson, S. B. (2020). Sub-Daily exposure to fine particulate matter and ambulance dispatches during wildfire seasons: A case-crossover study in British columbia, Canada. Environ. Health Perspect. 128, 67006. doi:10.1289/EHP5792
Ye, T., Guo, Y., Chen, G., Yue, X., Xu, R., Coêlho, M. de S. Z. S., et al. (2021). Risk and burden of hospital admissions associated with wildfire-related PM2·5 in Brazil, 2000–15: A nationwide time-series study. Lancet Planet Health 5, e599–e607. doi:10.1016/S2542-5196(21)00173-X
Glossary
Keywords: wildland fire smoke, cardiovascular health, microvascular endothelial cells, microvascular geometry, particulate matter, endothelial function, population-health, airborne pollution
Citation: Naserinejad N, Costanian C, Birot O, Barboni T and Roudier E (2023) Wildland fire, air pollution and cardiovascular health: is it time to focus on the microvasculature as a risk assessment tool?. Front. Physiol. 14:1225195. doi: 10.3389/fphys.2023.1225195
Received: 18 May 2023; Accepted: 06 July 2023;
Published: 19 July 2023.
Edited by:
Antonia Kaltsatou, University of Thessaly, GreeceReviewed by:
Matthew A. Nystoriak, University of Louisville, United StatesTieci Yi, Peking University, China
Copyright © 2023 Naserinejad, Costanian, Birot, Barboni and Roudier. This is an open-access article distributed under the terms of the Creative Commons Attribution License (CC BY). The use, distribution or reproduction in other forums is permitted, provided the original author(s) and the copyright owner(s) are credited and that the original publication in this journal is cited, in accordance with accepted academic practice. No use, distribution or reproduction is permitted which does not comply with these terms.
*Correspondence: Emilie Roudier, ZXJvdWRpZXJAeW9ya3UuY2E=