- 1Center of Calcium and Bone Research (COCAB), Faculty of Science, Mahidol University, Bangkok, Thailand
- 2Department of Physiology, Faculty of Science, Mahidol University, Bangkok, Thailand
- 3Institute of Molecular Biosciences, Mahidol University, Nakhon Pathom, Thailand
- 4The Academy of Science, The Royal Society of Thailand, Bangkok, Thailand
- 5National Center for Genetic Engineering and Biotechnology (BIOTEC), National Science and Technology Development Agency, Pathum Thani, Thailand
- 6Department of Physiology, Faculty of Medical Science, Naresuan University, Phitsanulok, Thailand
- 7Faculty of Allied Health Sciences, Burapha University, Chonburi, Thailand
Editorial on the Research Topic
Crosstalk between bone and other cells
Communications between osseous tissues and other organs are very common and essential for physiology of overall body system. For example, osteoblasts and osteocytes—now as endocrine cells (Figure 1)—are able to produce and release fibroblast growth factor (FGF)-23, which, in turn, regulates renal phosphate reabsorption (Vervloet, 2019; Agoro and White, 2023) and calcium transport across the intestinal epithelium (Khuituan et al., 2012; Rodrat et al., 2018; Wongdee et al., 2018; Wongdee et al., 2021). FGF-23 also downregulates the expression and activity of 25-hydroxyvitamin D 1α-hydroxylase (CYP27B1) in the renal proximal tubular cells, thereby reducing the action of 1,25-dihydroxyvitamin D3 (Perwad et al., 2007). During acute inflammation, osteocytes release certain mediators including C-terminal FGF-23 peptides to modulate hepatic hepcidin production and serum iron (Courbon et al., 2023). Moreover, osteocalcin or γ-carboxyglutamic acid-containing protein—as an osteoblast-derived endocrine factor—is capable of regulating pancreatic insulin production (Lee et al., 2007), adiponectin release from adipocytes (Lee et al., 2007) or testicular androgen biosynthesis (Karsenty and Oury, 2014).
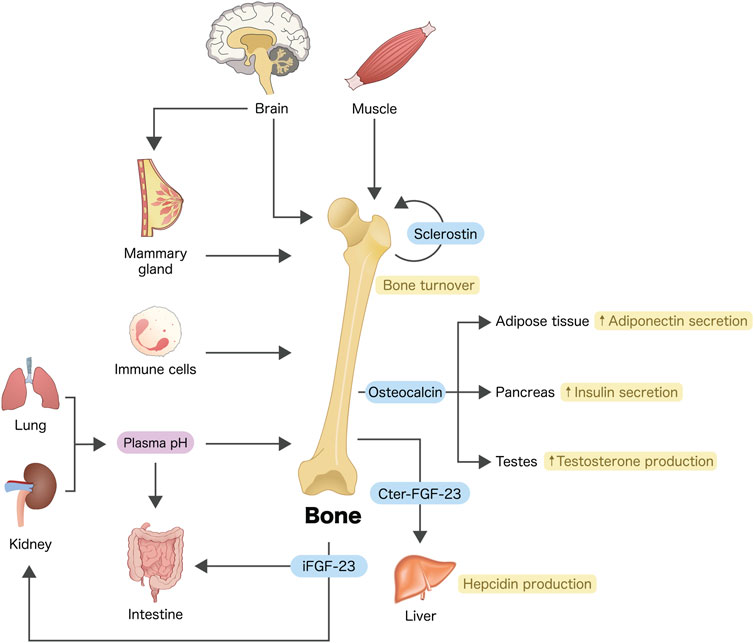
FIGURE 1. Crosstalks between bone and other organs or cells. Osteoblasts and/or osteocytes are considered endocrine cells that produce a number of bioactive molecules, e.g., osteocalcin, FGF-23 and sclerostin (see text for details). Some tissues or cells such as skeletal muscle, brain, immune cells, etc., also produce bioactive molecules to modulate bone turnover. Arrows indicate communication, modulation and/or regulation. iFGF-23, intact fibroblast growth factor-23; Cter-FGF-23, C-terminal FGF-23 peptide.
On the other hand, several tissues, such as muscle and brain, also send signals to modulate bone remodeling (Rousseaud et al., 2016; Gomarasca et al., 2020). For instance, myokines (e.g., interleukin-6 and irisin) have been reported to positively regulate bone formation (Gomarasca et al., 2020), whereas central sympathetic outflow probably enhances bone resorption through β2-adrenergic receptor (Bonnet et al., 2008; Huang et al., 2009). Certain organs such as lung and kidney are principal regulators of plasma pH, a disturbance of which (e.g., metabolic acidosis) profoundly affects intestinal calcium absorption (Charoenphandhu et al., 2007) and osteoblast-mediated bone formation (Bushinsky and Krieger, 2022).
In the present Research Topic, there are publications that clearly point out the crosstalk between bone and some other tissues and cells, including the respiratory tissues, adipose tissue, mammary tissue, brain, and immune cells (e.g., monocytes, T-cells, etc.). Specifically, in an original article by Ivanova et al., the results corroborated that a disruption of Wnt-sclerostin pathway contributed to osteopathy (i.e., osteopenia and osteoporosis) in patients with Gaucher’s disease, a common lysosomal storage disease caused by acid β-glucocerebrosidase gene mutation with cellular accumulation of glucocerebroside. Another original article by Kurgan et al. demonstrated the effects of treadmill exercise on serum sclerostin levels in male mice. Sclerostin has been recognized as a secretory glycoprotein that strongly negates bone formation (Delgado-Calle et al., 2017; Liao et al., 2022), especially during mechanical unloading (Lin et al., 2009). It is predominantly expressed by osteocytes in order to suppress osteogenesis by regulating proliferation, differentiation, mineralization and apoptosis of osteoblasts (Winkler et al., 2003; van Bezooijen et al., 2004; Liao et al., 2022). Sclerostin is also expressed in some other tissues, e.g., cartilage, heart, kidney, and liver as well (Brunkow et al., 2001; Weivoda et al., 2017). Since Kurgan and others (Kurgan et al.) assessed serum sclerostin in exercising male mice and found a decrease in serum sclerostin in exercising group, mechanical loading during exercise is probably an efficient way to restrict sclerostin secretion from both osteocytes and adipose tissue (Kurgan et al.; Oniszczuk et al., 2022).
Generally, Wnt/β-catenin signaling plays a critical role in bone homeostasis by promoting osteoblastogenesis and bone formation. On the other hand, sclerostin—known as a Wnt signaling antagonist—binds to Wnt co-receptors, i.e., low-density lipoprotein receptor-related proteins 5 and 6 (LRP5 and LRP6), thereby preventing Wnt from binding to these co-receptor proteins. As a consequence, sclerostin blocks the Wnt downstream signaling pathway and downregulates the expression of genes involved in osteoblast commitment, such as RUNX2 (Delgado-Calle et al., 2017). Several factors have been reported to suppress the expression of sclerostin including parathyroid hormone, estrogen as well as mechanical loading (Drake and Khosla, 2017). These factors thus act in concert with the Wnt/β-catenin signaling pathway to modulate bone homeostasis.
This Research Topic also contains review and mini-review articles that elaborate the crosstalk between bone and other tissues. Specifically, Ma and others (Ma et al.) explained how pulmonary tuberculosis, lung cancers, pollutant exposure [including particulate matter 2.5 (PM2.5)], asthma and chronic obstructive pulmonary disease are able to cause or aggravate osteoporosis. In a mini-review by He and Jiang, the interdependent interactions between immune cells (e.g., T cells, macrophages, NK cells, and dendritic cells) and cancer cells in bone microenvironment are well delineated. Lastly, Athonvarangkul and Wysolmerski discussed the physiological significances of the brain–breast–bone axis and maternal skeletal changes. A maternal pathological condition known as pregnancy/lactation-associated osteoporosis was also mentioned. Maternal central nervous system—particularly hypothalamus—controls bone metabolism by altering a number of humoral factors, e.g., prolactin, oxytocin and gonadotropin-releasing hormone, the latter of which is the salient regulator of circulating gonadotropins and estrogen. Prolactin not only enhances the intestinal calcium absorption (Charoenphandhu et al., 2009) but also modulates the expression of osteoblast-derived osteoclastogenic factors (Wongdee et al., 2011) and sclerostin production (Athonvarangkul and Wysolmerski).
Therefore, the present Research Topic is certainly able to shed some light on the crosstalks between bone and other distant cells, especially in lung and adipose tissue as well as cells in the brain–breast–bone axis (Figure 1). Indeed, interactions between bone cells and neighboring hematopoietic cells or stem cells within marrow tissue microenvironment are not uncommon and their detailed signaling networks are worth exploring.
Author contributions
All authors listed have made a substantial, direct, and contribution to the work and approved it for publication.
Funding
NC is supported by Mahidol University [Fundamental Fund: fiscal year 2022 by National Science Research and Innovation Fund (NSRF)], and the National Research Council of Thailand–Mahidol University Grant (Distinguished Research Professor Grant). KW is supported by Burapha University, Thailand Science Research and Innovation (TSRI), and National Science Research and Innovation Fund (NSRF) (Fundamental Fund: Grant no. 41/2566).
Acknowledgments
We thank Thitapha Kiattisirichai for artwork.
Conflict of interest
The authors declare that the research was conducted in the absence of any commercial or financial relationships that could be construed as a potential conflict of interest.
Publisher’s note
All claims expressed in this article are solely those of the authors and do not necessarily represent those of their affiliated organizations, or those of the publisher, the editors and the reviewers. Any product that may be evaluated in this article, or claim that may be made by its manufacturer, is not guaranteed or endorsed by the publisher.
References
Agoro, R., and White, K. E. (2023). Regulation of FGF23 production and phosphate metabolism by bone-kidney interactions. Nat. Rev. Nephrol. 19 (3), 185–193. doi:10.1038/s41581-022-00665-x
Bonnet, N., Benhamou, C. L., Malaval, L., Goncalves, C., Vico, L., Eder, V., et al. (2008). Low dose beta-blocker prevents ovariectomy-induced bone loss in rats without affecting heart functions. J. Cell Physiol. 217 (3), 819–827. doi:10.1002/jcp.21564
Brunkow, M. E., Gardner, J. C., Van Ness, J., Paeper, B. W., Kovacevich, B. R., Proll, S., et al. (2001). Bone dysplasia sclerosteosis results from loss of the SOST gene product, a novel cystine knot-containing protein. Am. J. Hum. Genet. 68 (3), 577–589. doi:10.1086/318811
Bushinsky, D. A., and Krieger, N. S. (2022). Effects of acid on bone. Kidney Int. 101 (6), 1160–1170. doi:10.1016/j.kint.2022.02.032
Charoenphandhu, N., Nakkrasae, L. I., Kraidith, K., Teerapornpuntakit, J., Thongchote, K., Thongon, N., et al. (2009). Two-step stimulation of intestinal Ca2+ absorption during lactation by long-term prolactin exposure and suckling-induced prolactin surge. Am. J. Physiol. Endocrinol. Metab. 297 (3), E609–E619. doi:10.1152/ajpendo.00347.2009
Charoenphandhu, N., Wongdee, K., Tudpor, K., Pandaranandaka, J., and Krishnamra, N. (2007). Chronic metabolic acidosis upregulated claudin mRNA expression in the duodenal enterocytes of female rats. Life Sci. 80 (19), 1729–1737. doi:10.1016/j.lfs.2007.01.063
Courbon, G., Thomas, J. J., Martinez Calle, M., Wang, X., Spindler, J., Von Drasek, J., et al. (2023). Bone-derived C-terminal FGF23 cleaved peptides increase iron availability in acute inflammation. Blood 2022, 18475. doi:10.1182/blood.2022018475
Delgado-Calle, J., Sato, A. Y., and Bellido, T. (2017). Role and mechanism of action of sclerostin in bone. Bone 96, 29–37. doi:10.1016/j.bone.2016.10.007
Drake, M. T., and Khosla, S. (2017). Hormonal and systemic regulation of sclerostin. Bone 96, 8–17. doi:10.1016/j.bone.2016.12.004
Gomarasca, M., Banfi, G., and Lombardi, G. (2020). Myokines: The endocrine coupling of skeletal muscle and bone. Adv. Clin. Chem. 94, 155–218. doi:10.1016/bs.acc.2019.07.010
Huang, H. H., Brennan, T. C., Muir, M. M., and Mason, R. S. (2009). Functional α1-and β2-adrenergic receptors in human osteoblasts. J. Cell Physiol. 220 (1), 267–275. doi:10.1002/jcp.21761
Karsenty, G., and Oury, F. (2014). Regulation of male fertility by the bone-derived hormone osteocalcin. Mol. Cell Endocrinol. 382 (1), 521–526. doi:10.1016/j.mce.2013.10.008
Khuituan, P., Teerapornpuntakit, J., Wongdee, K., Suntornsaratoon, P., Konthapakdee, N., Sangsaksri, J., et al. (2012). Fibroblast growth factor-23 abolishes 1,25-dihydroxyvitamin D3-enhanced duodenal calcium transport in male mice. Am. J. Physiol. Endocrinol. Metab. 302 (8), E903–E913. doi:10.1152/ajpendo.00620.2011
Lee, N. K., Sowa, H., Hinoi, E., Ferron, M., Ahn, J. D., Confavreux, C., et al. (2007). Endocrine regulation of energy metabolism by the skeleton. Cell 130 (3), 456–469. doi:10.1016/j.cell.2007.05.047
Liao, C., Liang, S., Wang, Y., Zhong, T., and Liu, X. (2022). Sclerostin is a promising therapeutic target for oral inflammation and regenerative dentistry. J. Transl. Med. 20 (1), 221. doi:10.1186/s12967-022-03417-4
Lin, C., Jiang, X., Dai, Z., Guo, X., Weng, T., Wang, J., et al. (2009). Sclerostin mediates bone response to mechanical unloading through antagonizing Wnt/beta-catenin signaling. J. Bone Min. Res. 24 (10), 1651–1661. doi:10.1359/jbmr.090411
Oniszczuk, A., Kaczmarek, A., Kaczmarek, M., Ciałowicz, M., Arslan, E., Silva, A. F., et al. (2022). Sclerostin as a biomarker of physical exercise in osteoporosis: A narrative review. Front. Endocrinol. (Lausanne). 13, 954895. doi:10.3389/fendo.2022.954895
Perwad, F., Zhang, M. Y., Tenenhouse, H. S., and Portale, A. A. (2007). Fibroblast growth factor 23 impairs phosphorus and vitamin D metabolism in vivo and suppresses 25-hydroxyvitamin D-1alpha-hydroxylase expression in vitro. Am. J. Physiol. Ren. Physiol. 293 (5), F1577–F1583. doi:10.1152/ajprenal.00463.2006
Rodrat, M., Wongdee, K., Panupinthu, N., Thongbunchoo, J., Teerapornpuntakit, J., Krishnamra, N., et al. (2018). Prolonged exposure to 1,25(OH)2D3 and high ionized calcium induces FGF-23 production in intestinal epithelium-like caco-2 monolayer: A local negative feedback for preventing excessive calcium transport. Arch. Biochem. Biophys. 640, 10–16. doi:10.1016/j.abb.2017.12.022
Rousseaud, A., Moriceau, S., Ramos-Brossier, M., and Oury, F. (2016). Bone-brain crosstalk and potential associated diseases. Horm. Mol. Biol. Clin. Investig. 28 (2), 69–83. doi:10.1515/hmbci-2016-0030
van Bezooijen, R. L., Roelen, B. A., Visser, A., van der Wee-Pals, L., de Wilt, E., Karperien, M., et al. (2004). Sclerostin is an osteocyte-expressed negative regulator of bone formation, but not a classical BMP antagonist. J. Exp. Med. 199 (6), 805–814. doi:10.1084/jem.20031454
Vervloet, M. (2019). Renal and extrarenal effects of fibroblast growth factor 23. Nat. Rev. Nephrol. 15 (2), 109–120. doi:10.1038/s41581-018-0087-2
Weivoda, M. M., Youssef, S. J., and Oursler, M. J. (2017). Sclerostin expression and functions beyond the osteocyte. Bone 96, 45–50. doi:10.1016/j.bone.2016.11.024
Winkler, D. G., Sutherland, M. K., Geoghegan, J. C., Yu, C., Hayes, T., Skonier, J. E., et al. (2003). Osteocyte control of bone formation via sclerostin, a novel BMP antagonist. EMBO J. 22 (23), 6267–6276. doi:10.1093/emboj/cdg599
Wongdee, K., Chanpaisaeng, K., Teerapornpuntakit, J., and Charoenphandhu, N. (2021). Intestinal calcium absorption. Compr. Physiol. 11 (3), 2047–2073. doi:10.1002/cphy.c200014
Wongdee, K., Rodrat, M., Keadsai, C., Jantarajit, W., Teerapornpuntakit, J., Thongbunchoo, J., et al. (2018). Activation of calcium-sensing receptor by allosteric agonists cinacalcet and AC-265347 abolishes the 1,25(OH)2)D3-induced Ca2+ transport: Evidence that explains how the intestine prevents excessive Ca2+ absorption. Arch. Biochem. Biophys. 657, 15–22. doi:10.1016/j.abb.2018.09.004
Keywords: bone, calcium, immune cells, maternal bone metabolism, myokine
Citation: Charoenphandhu N, Chanpaisaeng K, Teerapornpuntakit J and Wongdee K (2023) Editorial: Crosstalk between bone and other cells. Front. Physiol. 14:1209053. doi: 10.3389/fphys.2023.1209053
Received: 20 April 2023; Accepted: 02 May 2023;
Published: 09 May 2023.
Edited by:
Laurence Vico, Institut National de la Santé et de la Recherche Médicale (INSERM), FranceReviewed by:
Guillaume Courbon, Northwestern Medicine, United StatesCopyright © 2023 Charoenphandhu, Chanpaisaeng, Teerapornpuntakit and Wongdee. This is an open-access article distributed under the terms of the Creative Commons Attribution License (CC BY). The use, distribution or reproduction in other forums is permitted, provided the original author(s) and the copyright owner(s) are credited and that the original publication in this journal is cited, in accordance with accepted academic practice. No use, distribution or reproduction is permitted which does not comply with these terms.
*Correspondence: Kannikar Wongdee, a2FubmlrYXJAZ28uYnV1LmFjLnRo