- Estonian University of Life Sciences, Tartu, Estonia
Introduction: Declines in honeybee abundance have been observed worldwide during last decades. This is partly due to plant protection agents used in intensive farming, landscaping and infrastructure maintenance. Another type of factors negatively affecting honeybees is the spread of diseases caused by different pathogens and pests. Lately, more focus has been paid to the interactions between different overlapping stressors affecting honeybee health, the combination of these often being more detrimental compared to individual stressors. The most widely used stress-evaluating methods take into account lethal- or motorial changes of the individuals or colonies. Comparatively little honeybee research has examined changes in initial recovery potential and physiological symptoms of toxification. The aim of this study was to examine the combined effect of Nosema apis and N. ceranae (according to a newer classification Vairimorpha apis and V. ceranae), the common causes of nosemosis in the honeybee Apis mellifera L., with the insecticide dimethoate.
Methods: In this study, honeybee mortality and metabolic rate were used to assess the combined effects interactions of Nosema ssp. and dimethoate.
Results: Our results showed that exposure to the low concentration of either dimethoate, either one or both species of Nosema ssp as single factors or in the combination had no significant effect on honeybee metabolic rate. The mortality increased with the two Nosema spp., as well as with infection by N. ceranae alone. The effect of dimethoate was observed only in combination with N. apis infection, which alone had no effect on individual honeybee mortality.
Conclusion: This study demonstrates that the overlapping exposure to a non-lethal concentration of a pesticide and a pathogen can be hidden by stronger stressor but become observable with milder stressors.
Introduction
Over the past half century, a number of problems have emerged due to agriculture and poor nature conservation practices, to which little attention has been paid. Stressors like disease-causing pathogens and parasites, the use of plant protection products, climate change and their interactions are considered as factors affecting pollinator populations (Hristov et al., 2020; Insolia et al., 2022; Piot et al., 2022). Chemical pesticides used in intensive agriculture often get the most attention in this regard. The consistent and sometimes excessive use of these has exacerbated the problem of pesticide residues accumulating in the environment (Sharma et al., 2019), where they come into contact with non-target organisms like pollinators (Shafeeque, Ahmad, and Kamal, n.d.; Nicholls and Altieri, 2013). Traces of chemical pesticides used in agriculture have been detected in various beekeeping products as well as in bees themselves (Kasiotis et al., 2014; Kiljanek et al., 2017; Böhme et al., 2018). The bee declines have been observed in America, Europe, and many other parts of the world, especially in areas where intensive farming practices are used (Dirzo et al., 2014; Potts et al., 2010; Potts et al., 2016; van Engelsdorp and Meixner, 2010; Osterman et al., 2021).
The majority of honeybee colony losses occur during the winter, but it is difficult to pinpoint the specific cause of the mortality, and it is likely the case that poor bee health often results not from single stressors, but rather combinations of stressors to which bees are exposed (Engelsdorp et al., 2009; Goulson et al., 2015; Insolia et al., 2022; van). While the parasitic varroa mite Varroa destructor is considered the most harmful pest to honeybees, partly because of vectoring other viruses (EPILOBEE Consortium et al., 2016), there are also other diseases which should not remain understudied. The two microsporidian pathogens, Nosema apis and N. ceranae (according Tokarev et al. (2020) Vairimorpha apis and V. ceranae) cause nosemosis, a serious but mostly not deadly disease (Botías et al., 2013). Nosema ceranae, originally a parasite of the Asian honeybee which nowadays causes serious problems to European honeybees all over the world and has largely replaced the Nosema apis, an original parasite of European honeybee (Paxton et al., 2007). Both pathogenic species infect the epithelical cells of the honeybee midgut, causing energetic stress (Mayack and Naug 2009; Zheng et al., 2014). Honeybee nosemosis is a disease that often has no clear clinical symptoms and there is no specific treatment against it (Galajda et al., 2021). Partly because of this, nosemosis is considered to be among the factors seriously affecting the overwintering success of honeybees (Fries, Ekbohm, and Villumstad, 1984).
The interaction of the disease with other stress factors, such as pesticide residues found in bee-collected pollen and nectar (Kasiotis et al., 2014; Karise et al., 2017; Kiljanek et al., 2017; Böhme et al., 2018; Raimets et al., 2020) may be detrimental to honeybees (Goblirsch, 2018). Several pesticides can affect a bee’s ability to cope with various diseases. For example, simultaneous exposure to glyphosate and difenoconazole, in Nosema-infected honeybees, was demonstrated to induce additional physiological stress (Almasri et al., 2021). Furthermore, in an Australian field experiment, combined exposure to thiamethoxam and N. apis substantially increased mortality, as well as reduced immunocompetence, in honeybees (Grassl et al., 2018). Overall, pathogen-pesticide interactions, either overlapping or sequential, can reduce honeybee survival and weaken colonies (Alaux et al., 2010; Sánchez-Bayo et al., 2016; Grassl et al., 2018; Almasri et al., 2021). Negative co-effects of Nosema ceranae infection and pesticides are previously observed over different life stages of worker honeybees (Glavinic et al., 2019; Tesovnik et al., 2020).
Fast and reliable methods for measuring non-invasive endpoints in living bees are needed. Until now, most studies have focused on the effects of one or multiple stress factors on cellular processes, survival and humoral immune response (Hartfelder et al., 2013; Havard, Laurent, and Chauzat, 2019; Trytek et al., 2022). Other studies focus on behavioural changes, brood development, number of mites or spores counted or estimated (Henry et al., 2017; Bird et al., 2021). However, it is difficult to measure the severity of these interactions on living bees. These methods are usually invasive and the bee will be sacrificed during the experiment. One of the methods allows measurements of living and intact insects might be respirometry, which allows to observe changes in metabolic rate in real-time and in living organisms (Muljar et al., 2012; Mänd and Karise, 2015; Karise et al., 2016; Ploomi et al., 2018; Castro et al., 2021). The traditional survival experiments may hide some important toxicological aspects like the process of recovery from toxicosis (El-Seedi et al., 2022) allowing also repeated measurement of treated individuals.
Nosemosis does not cause rapid death of colonies (Martín-Hernández et al., 2011) and is detectable throughout several seasons (Naudi et al., 2021). However, in combination with other stressors, the severity increases (Straub et al., 2020). The two Nosema species differ in their virulence (Martín-Hernández et al., 2011; Naudi et al., 2021), but both affect the bee gut by destroying the epithelial cells (Adl et al., 2005). We hypothesise that this could cause a nutritional deficiency that could be detectable with respirometry measurements. For instance the effects of neurotoxic insecticides are usually easily detectable with respirometry (Kestler, 1991; Muljar et al., 2012; Vinha et al., 2021). We also expect that the combination of the low dose of a neurotoxic insecticide and the pathogen might severely affect the overall metabolic rate of honeybees.
Materials and methods
The geographical location and study design
The study was conducted in Estonia in the summer of 2018 in Estonian University of Life Sciences in the insect physiology laboratory of the Chair of Plant Health. The first step was to establish 12 round isolated tents (with diameter of 3 m) covered with insect-proof nets. Each tent accommodated one Italian honeybee (Apis mellifera ligustica) colony. All the colonies were equal in size—the nucleus colonies included five frames (three frames of brood, two frames of honey), and the feeder for sugar solution.
We repeatedly sampled forager bees from the entrance of the colonies 2 weeks before the experiment to ensure no Nosema infection was present. The absence of the Nosema spp. was proven by using Multiplex PCR method. The DNeasy Blood and Tissue Kit (Qiagen, Hilden, Germany) was used to isolate DNA, thereafter a Multiplex PCR (M-PCR) was used to identy the species (described in (Naudi et al., 2021)). Following the assay, electrophoretic separation was done to PCR products and visualized in 2% agarose TAE gel (N. apis: band at 321 bp, N. ceranae: band at 218–219 bp, Figure 1).
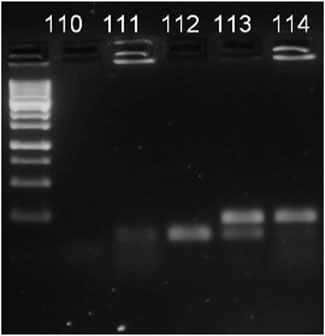
FIGURE 1. Figure 1 Example of visualisation of M-PCR products. 110 indicates negative control, 111—negative result, 112—N. ceranae, 113—mix of both pathogens, 114—Nosema apis.
To obtain the N. apis and N. ceranae spores, honeybee colonies with inherent clinical nosemosis symptoms were identified by local beekeepers. From these colonies, infected worker bees were collected, midguts with entire hind bodies were removed and crushed using a hand homogenizer in a Bioreba universal separation bag (15 × 28.5 cm), and the spore-bearing suspension was collected. The Nosema species were identified by the same Multiplex PCR method. Once the species were identified, centrifugation protocol (Fries et al., 2013a) was used to purify the spores. As the sample purity with this protocol is about 85%, the resulting suspension was cleaned with a 10-micron filter. A flow cytometer (Accuri®C6 flow cytometer (Becton Dickinson, BD, Franklin Lakes, NJ, USA) was used to determine the number of spores (described in (Naudi et al., 2021)).
To infect the nosemosis-free colonies, we used three treatments (N. apis, N. ceranae and their co-infection) with three replications each. Each treatment consisted of five million spores mixed in 1 L of syrup (mix of 40% fructose, 30% glucose, and 30% sucrose dissolved in heated water). The food was provided weekly for 3 weeks, meaning each honeybee colony received a total of 15 million spores.
According to the calculations, each bee acquired about 1200–1500 spores (colony with 5 frames full of bees—roughly 2000–2500 bees per frame). Two weeks after the final inoculation, mortality and physiology experiments were conducted.
Pesticide treatment
As additional stressor, the insecticide dimethoate in its pure form (Sigma-Aldrich, 99.6% pure active ingredient) was used. Dimethoate was chosen for this experiment as a positive control. Dimethoate has known to have a negative effect on honeybees with known and established lethal and non-lethal doses (Schuehly, Riessberger-Gallé, and Hernández López, 2021).
Dimethoate was mixed into sugar syrup (water and sugar 1:1). 20 μg of dimethoate (far below the LD50 to honeybees) was added per 1 L of syrup. The pesticide concentration was chosen according to the survey conducted in Poland where 20 µg of dimethoate per 1 kg of honey was found. (Bargańska, Ślebioda, and Namieśnik, 2013). Both in control and treatment groups, the bees were fed ad libitum. The colonies were monitored daily and empty feeders were replaced with full ones. We did not monitor the exact amount of feed consumed by individual bees. However, based on standard calculations about honeybee daily food intake (Rodney and Purdy, 2020), we calculated the approximate amount of food consumed and thus possible dimethoate intake. The bees received 20 µg of dimethoate per litre through feed syrup. Considering that one worker bee consumes 22 mg of sugar syrup per day, maximum of 0.0003 µg per day of dimethoate was consumed by one bee.
Mortality assessment
To assess the effects of dimethoate and nosemosis on honeybee mortality, we created special micro-colonies in small wooden cages (9 × 7 × 4 cm), with plastic mesh sides. 20 young worker bees, taken from each nucleus colony described above, were placed in each cage. The cages were kept in climate chamber (controlled temperature, humidity and total darkness, Sanyo MLR-351H, Osaka, Japan), where ambient temperature was 34.5 °C and the relative humidity was 60% to mimic the normal conditions of a bee colony. Cages were equipped with two 1.5 ml Eppendorf tubes (one containing feed syrup (1:1 sugar-water) and the other containing distilled water), where the bees had free access. We created two sets of cages per each hive—one to test the effect of the pathogen, the other to test the effect of adding chronical exposure to a non-lethal dose of dimethoate. From each colony, we created three cages, while we had three colonies in every treatment group, which makes 9 cages per treatment group. Treatments were distributed as follows: control, control + dimethoate, N. apis, N. apis + dimethoate, N. ceranae, N. ceranae + dimethoate, Nosema co-infection, Nosema co-infection + dimethoate. The observation lasted 25 days, during which dead bees were counted every 24 h.
Respiratory measurements
Separate cages with honeybees needed for respiratory measurements were prepared additionally to those used in mortality assessments. 20 bees were collected from each hive, and acclimated for 12 h before the measurements started. Measurements of the 10 individuals lasted about 10 h, thus all the honeybees from all treatments had been exposed to the dimethoate 12–21 h, and this scheme was followed for each treatment.
The bee respiration rate was determined, using flow-through respirometer LI-7000 (LiCor, Lincoln, Nebraska, USA). Measurements were performed at room temperature (22°C ± 1°C). Each bee spent 50 min in respirometer chamber. The air circulating in the analyzer was taken from the laboratory environment and was passed through magnesium perchlorate Mg(ClO4)2 and sodium hydroxide NaOH to remove water vapor and CO2. The volume of air passing through the system in each minute was 600 ml. The measurement of the metabolic rate of a single bee lasted 60 min, which included including a 5-min machine calibration time to achieve 0-level and 5 min for calming down from handling stress of honeybees—both which were excluded from the analysis. Thus the metabolic rate was calculated based on 50 min measurement. During this time, the machine reached its 0-level registration stage.
Statistical analysis
The statistical software Statistica (DellTM StatisticaTM, StatSoft) was used to process and analyze the data of the experiment. The respiratory data was normalized by log-transforming (using logarithmic values of) the data. The effects of the variables ‘Nosema treatment’ and ‘dimethoate treatment’ were analyzed using Main-effect ANOVA followed by Fisher LSD post hoc test. For mortality data we used the General Linear Models Repeated Measures Analyses of Variance using the ‘Time’ as within-effects factor and ‘Nosema treatment’ and ‘dimethoate treatment’ as categorical factors. To understand when the different factors started to affect the honeybee longevity, we allowed the model to calculate Univariate Results for each day.
Results
Effect on honeybees metabolism
By the end of the experiment samples were collected and analysed. The results showed 4872–65940 spores per bee throughout all treatments. In average, N. apis 9417 ± 4544,5, N. ceranae 42966 ± 22974 and Nosema mix 7434 ± 2702 spores.
We did not detect statistically significant changes in metabolic rate of honeybees neither by the Nosema treatment (Figure 2) (F (3, 72) = 0.85, p = 0.47) nor by adding dimethoate at sublethal level (F (1, 72) = 0.80, p = 0.37) (Figure 2). The variations within the treatments were relatively large, ranging from 61.8 µL CO2/L of air to 3456.6 µL CO2/L. The lowest result was recorded in honeybees infected with N. ceranae spores (the difference from the control was nearly significant, Fisher LSD test: p = 0.057) and the highest result in those bees infected with N. apis spores that also had received dimethoate in the feed.
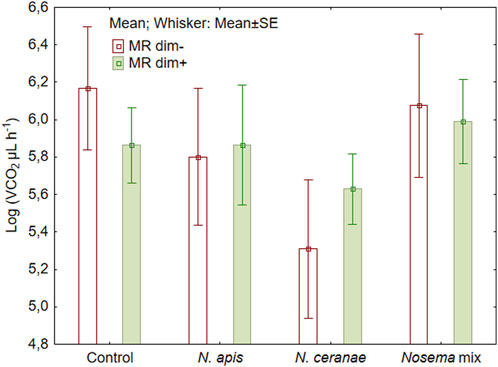
FIGURE 2. Mean metabolic rate (MR) of honeybees (N = 10) during 50 min of measurements after overlapping exposure to Nosema apis, N. ceranae or mix of both with or without a sublethal dose of dimethoate (dim−/dim+).
Mortality
Mortality rate in Nosema infected bees increased significantly on the day 4 of the experiment (F (3, 64) = 17.47, p < 0.001) (see Univariate Results for each day in Supplementary Material Supplementary Table S1). Additional stress caused by dimethoate was generally not significant (F (1, 64) = 0.87, p = 0.35). However we observed significant effect on bee mortality on day 21 in the N. apis treatment group (Figure 3; Supplementary Table S1).
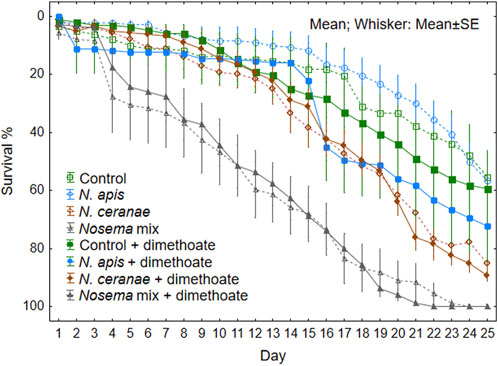
FIGURE 3. Survival rate of honeybees (N = 9 cages, 20 honeybees in each) per treatment group during 25 days of observation after overlapping sposure to Nosema apis, N. ceranae or mix of both with or without a sublethal dose of dimethoate chronically in the feed.
Mortality rate was lowest in the N. apis treatment group during the full extent of the experiment, being even slightly slower than that of the control groups (Figure 3). In the N. ceranae treatment groups, the mortality rate was initially slow similar to the control and N. apis groups, but increased rapidly after 2 weeks from the start of the experiment. The combination of both Nosema spp. resulted in higher mortality rate single pathogens and the difference was obvious already after few days followed by constant increase in mortality rate.
Discussion
Our results suggest that N. apis as a stressor does not significantly affect the respiration rate on individual level in honeybees. Still, the N. ceranae had a greater impact compared to N. apis. Different studies also found the same difference between the two pathogens (Higes et al., 2010; Goblirsch, 2018; Sinpoo et al., 2018). N. ceranae is described as more energy demanding on the host, indicated by higher feed consumption (Martín-Hernández et al., 2011).
Dimethoate has been demonstrated to be lethal to honeybees when administered at concentrations of 0.2 µg per bee (Baskar, Sudha, and Tamilselvan, 2016). Sublethal concentration of dimethoate may increase the bumblebee’s food consumption (Cornement et al., 2017), it is possible that similar increase took place in our study. However, as the feed consumption was not monitored, the consumed amount of feed is based on average nutritional needs of 0.0003 µg per day.
Different stressors can result in different responses in honeybees, including altered metabolism (Even, Devaud, and Barron, 2012). In the present study, the absence of any significant effect may have resulted from high variability within the data accompanied with relatively low spore loads (Figure 2.). Such variability can be due to the individual physiological characteristics of bees, as each individual has a different ability to withstand stress. In addition to the treatments, further stress is induced by handling the bees when putting them in the analyser and limiting their movement. To reduce the impact of handling stress, it could be helpful to extend the period of analysis, during which the individuals could get used to new and more unpleasant conditions (Mänd et al., 2005). In the case of acclimated bees, the variability in the dataset may somewhat decrease (Even, Devaud, and Barron, 2012). However, based on our experiences, honeybees do not tolerate long detainment in the analyser.
Individual stress factors (N. apis, N. ceranae and dimethoate) had no individual impact on honeybee mortality. The authors suggest that this was because the bees used in the experiments were mainly adult bees. The adult honeybees can be more resistant to stress induced by pathogens (Roberts and Hughes, 2014). The selective breeding throughout the years has also resulted in more tolerant bees that are more capable to maintain close to normal functionality even when infected with Nosema spp (Kurze et al., 2016).
Spore counts have often been used to describe the severity of the disease (Botías et al., 2013; Vidau et al., 2014; Kurze et al., 2016). It is proven that 10–33 000 spores per bee is sufficient to induce infection (Fries et al., 2013). In an experiment carried out in Sweden, where mixture of N. apis and N. ceranae spores were used. There the number of spores used to infect honey bees ranged from 10 to 10,000 per bee. Regardless of the mixed pathogen spore ratio used, the number of spores equalized by day 12 of the experiment and infection occurred in both cases (Forsgren and Fries, 2010). From the collected and analyzed bees, after the experiment, we conclude that the amount of spores used in this work were sufficient to induce infection, cause stress and alter the metabolic rate. Natural resistance to nosemosis of the bees used in our experiment is unknown. It is demonstrated that in artificial laboratory conditions can result in a longer lifespan of bees (Human et al., 2013). In this regard, the physical capacity to tolerate stress in laboratory conditions can be greater than in the field conditions.
The combined effect of multiple stressors, in the form of N. apis and N. ceranae, resulted in a significantly higher stress response, expressed by higher honeybee mortality. Control groups, both with and without dimethoate, and also infection with solely N. apis, resulted in 50% of the specimens still alive at the end of the experiment (25 days). Approximately half of the bees (53%) were alive on day 20. This is similar to the results of Vidau (2011), where specimens were infected only with N. ceranae, but the additionally exposure to fipronil and thiacloprid caused the mortality of 82% and 71% respectively (Vidau et al., 2011). In our study, N. ceranae and the overlapping exposure to dimethoate, resulted both in the 60% mortality of individuals on the day 20 of observation (Figure 2). But with N. apis, our results clearly demonstrate the additive stress from the dimethoate—already at Day15, the mortality increased rapidly being similar to the mortality level honeybees of N. ceranae treatment groups. Given that the 24 h LD50 of dimethoate for honey bee workers is 0.126 µg/bee, dimethoate alone in the concentrations chosen in our experiment, is unlikely to cause significant mortality.
The maximum amount of dimethoate that one bee can take per day in this experiment remained significantly lower, as mentioned above. The negative interaction of different pesticides and nosemosis has been proven in various researches. The interaction is often manifested in the fact that a sublethal dose of pesticide causes faster growth of Nosema spores in the nosemosis honeybees (Alaux et al., 2010; Vidau et al., 2011; Aufauvre et al., 2012; Pettis et al., 2012). According to the present study, the combined effects of stressors results in shorter lifespan of worker bees even at very low doses, which hardly impact the test results at shorter time scale as shown by our respirometry experiment. Therefore it is important to pay attention to the honeybee colony health. Beekeepers should be aware about combined effects of stressors and try to avoid adding any synthetic pesticides as parasite or disease treatment. Even if it would be hard to avoid agricultural pesticides, avoiding synthetic veterinary medicines in beehives, is something, every beekeeper can do to protect their honeybees.
Data availability statement
The raw data supporting the conclusions of this article will be made available by the authors, without undue reservation.
Author contributions
Conceptualization: KP, SN, and RR; Writing KP; Reading and correcting: KP, SN, RR, MJ, EL, and RK; Methodology: KP, SN, and RR; experimenting KP, SN, and MJ; Data curation: KP, MJ, EL, and RK; Funding RK. All authors contributed to the article and approved the submitted version.
Funding
This research was supported by Baseline funding for supporting the activities related to the project “Honey bee (Apis mellifera) sensitivity to food quality and diseases: explained by collected field data and subsequent individual lab testing (P200192PKTE)” of the Estonian Ministry of Education.
Acknowledgments
We thank the beekeeper, who supplied us with the honey bee hives and to Madis Järvis for his help with setting up the experiment. Also, we are grateful to Dr. Jonathan M. Willow for his help in improving the English.
Conflict of interest
The authors declare that the research was conducted in the absence of any commercial or financial relationships that could be construed as a potential conflict of interest.
Publisher’s note
All claims expressed in this article are solely those of the authors and do not necessarily represent those of their affiliated organizations, or those of the publisher, the editors and the reviewers. Any product that may be evaluated in this article, or claim that may be made by its manufacturer, is not guaranteed or endorsed by the publisher.
Supplementary material
The Supplementary Material for this article can be found online at: https://www.frontiersin.org/articles/10.3389/fphys.2023.1198070/full#supplementary-material
References
Adl, S. M., Simpson, A. G. B., Farmer, M. A., Andersen, R. A., Anderson, O. R., Barta, J. R., et al. (2005). The new higher level classification of eukaryotes with emphasis on the taxonomy of protists. J. Eukaryot. Microbiol. 52 (nr 5), 399–451. doi:10.1111/j.1550-7408.2005.00053.x
Alaux, Cédric, Brunet, Jean-Luc, Dussaubat, Claudia, Mondet, Fanny, Tchamitchan, Sylvie, Cousin, Marianne, et al. (2010). Interactions between Nosema microspores and a neonicotinoid weaken honeybees (Apis mellifera). Environ. Microbiol. 12 (3), 774–782. doi:10.1111/j.1462-2920.2009.02123.x
Almasri, Hanine, Tavares, Daiana Antonia, Diogon, Marie, Pioz, Maryline, Alamil, Maryam, Sené, Déborah, et al. (2021). Physiological effects of the interaction between Nosema ceranae and sequential and overlapping exposure to glyphosate and difenoconazole in the honey bee Apis mellifera. Ecotoxicol. Environ. Saf. 217 (July), 112258. doi:10.1016/j.ecoenv.2021.112258
Aufauvre, Julie, Biron, David G., Vidau, Cyril, Fontbonne, Régis, Roudel, Mathieu, Diogon, Marie, et al. (2012). Parasite-insecticide interactions: A case study of Nosema ceranae and fipronil synergy on honeybee. Sci. Rep. 2 (1), 326. doi:10.1038/srep00326
Böhme, Franziska, Bischoff, Gabriela, Claus, P., Zebitz, W., Peter, Rosenkranz, and Wallner, Klaus. 2018. “Pesticide residue survey of pollen loads collected by honeybees (Apis mellifera) in daily intervals at three agricultural sites in south Germany.” edited by nicolas desneux. PLOS ONE 13 (7): e0199995. doi:10.1371/journal.pone.0199995
Bargańska, Żaneta, Ślebioda, Marek, and Namieśnik, Jacek (2013). Pesticide residues levels in honey from apiaries located of northern Poland. Food control 31 (1), 196–201. doi:10.1016/j.foodcont.2012.09.049
Baskar, Kathirvelu, Venkatesan, Sudha, and Tamilselvan, Chidambaram. 2016. “Acute oral toxicity of dimethoate 30% ec on honey bee (Apis mellifera)” 1 (1): 5, https://www.academia.edu/32493047/Acute_Oral_Toxicity_of_Dimethoate_30_Ec_on_Honey_Bee_Apis_mellifera.
Bird, Gwendolyn, Wilson, Alan E., Williams, Geoffrey R., and Hardy, Nate B. 2021, “Parasites and pesticides act antagonistically on honey bee health” edited by lorenzo marini, J. Appl. Ecol. 58 (5): 997–1005. doi:10.1111/1365-2664.13811
Botías, Cristina, Martín-Hernández, Raquel, Barrios, Laura, Meana, Aránzazu, and Higes, Mariano (2013). Nosema spp. Infection and its negative effects on honey bees (Apis mellifera iberiensis) at the colony level. Veterinary Res. 44 (1), 25. doi:10.1186/1297-9716-44-25
Castro, Bárbara Monteiro de Castro e., Martínez, Luis Carlos, Plata-Rueda, Angelica, Soares, Marcus Alvarenga, Wilcken, Carlos Frederico, Zanuncio, Antônio José Vinha, et al. (2021). Exposure to chlorantraniliprole reduces locomotion, respiration, and causes histological changes in the midgut of velvetbean caterpillar anticarsia gemmatalis (Lepidoptera: Noctuidae). Chemosphere 263 (January), 128008. doi:10.1016/j.chemosphere.2020.128008
Cornement, Magdaléna, Hodapp, Bettina, Schmidt, Thomas, Pardo, Pablo, Höger, Stefan, and Kimmel, Stefan. 2017. “Bumble bee acute testing: Why not testing more than one endpoint?,”Proceedings of the European SETAC Meeting 2017, July 2017, Brussels, Belgium, 1.
Dirzo, R., Young, H. S., Galetti, M., Ceballos, G., Isaac, N. J. B., Collen, B., et al. (2014). Defaunation in the anthropocene. Science 345 (6195), 401–406. doi:10.1126/science.1251817
El-Seedi, Hesham R., Ahmed, Hanan R., Abd El-Wahed, Aida A., Saeed, Aamer, Algethami, Ahmed F., Attia, Nour F., et al. (2022). Bee stressors from an immunological perspective and strategies to improve bee health. Veterinary Sci. 9 (5), 199. doi:10.3390/vetsci9050199
Even, Naïla, Devaud, Jean-Marc, and Barron, Andrew (2012). General stress responses in the honey bee. Insects 3 (4), 1271–1298. doi:10.3390/insects3041271
Forsgren, Eva, and Fries, Ingemar (2010). Comparative virulence of Nosema ceranae and Nosema Apis in individual European honey bees. Veterinary Parasitol. 170 (3–4), 212–217. doi:10.1016/j.vetpar.2010.02.010
Fries, Ingemar, Ekbohm, Gunnar, and Villumstad, Egil (1984). Nosema Apis, sampling techniques and honey yield. J. Apic. Res. 23 (2), 102–105. doi:10.1080/00218839.1984.11100617
Fries, Ingemar, Chauzat, Marie-Pierre, Chen, Yan-Ping, Vincent, Doublet, Genersch, Elke, Gisder, Sebastian, et al. (2013a). Standard methods for Nosema research. J. Apic. Res. 52 (1), 1–28. doi:10.3896/IBRA.1.52.1.14
Galajda, Richard, Valenčáková, Alexandra, Sučik, Monika, and Kandráčová, Petra (2021). Nosema disease of European honey bees. J. Fungi 7 (9), 714. doi:10.3390/jof7090714
Glavinic, Uros, Tesovnik, Tanja, Stevanovic, Jevrosima, Zorc, Minja, Cizelj, Ivanka, Stanimirovic, Zoran, et al. (2019). Response of adult honey bees treated in larval stage with prochloraz to infection with Nosema ceranae. PeerJ 7 (February), e6325. doi:10.7717/peerj.6325
Goblirsch, Mike (2018). Nosema ceranae disease of the honey bee (Apis mellifera). Apidologie 49 (1), 131–150. doi:10.1007/s13592-017-0535-1
Goulson, Dave, Nicholls, Elizabeth, Botías, Cristina, Ellen, L., and Rotheray, (2015). Bee declines driven by combined stress from parasites, pesticides, and lack of flowers. Science 347 (6229), 1255957. doi:10.1126/science.1255957
Grassl, Julia, Shannon, Holt, Cremen, Naomi, Peso, Marianne, Hahne, Dorothee, and Baer, Boris (2018). Synergistic effects of pathogen and pesticide exposure on honey bee (Apis mellifera) survival and immunity. J. Invertebr. Pathology 159 (November), 78–86. doi:10.1016/j.jip.2018.10.005
Hartfelder, Klaus, Bitondi, Márcia M. G., Brent, Colin S., Guidugli-Lazzarini, Karina R., Simões, Zilá L. P., Stabentheiner, Anton, et al. (2013). Standard methods for physiology and biochemistry research in Apis mellifera. J. Apic. Res. 52 (1), 1–48. doi:10.3896/IBRA.1.52.1.06
Havard, Tiphaine, Laurent, Marion, and Chauzat, Marie-Pierre (2019). Impact of stressors on honey bees (Apis mellifera; hymenoptera: Apidae): Some guidance for research emerge from a meta-analysis. Diversity 12 (1), 7. doi:10.3390/d12010007
Henry, Mickaël, Becher, Matthias A., Osborne, Juliet L., Kennedy, Peter J., Aupinel, Pierrick, Vincent, Bretagnolle, et al. (2017). Predictive systems models can help elucidate bee declines driven by multiple combined stressors. Apidologie 48 (3), 328–339. doi:10.1007/s13592-016-0476-0
Higes, Mariano, García-Palencia, P., Botías, C., and Martín-Hernández, R.The differential development of microsporidia infecting worker honey bee (Apis mellifera) at increasing incubation temperature. 2010. “the differential development of microsporidia infecting worker honey bee (Apis mellifera) at increasing incubation temperature: Nosema Apis infection is inhibited at high temperature.” Environ. Microbiol. Rep. 2 (6): 745–748. doi:10.1111/j.1758-2229.2010.00170.x
Hristov, Peter, Shumkova, Rositsa, Palova, Nadezhda, and Neov, Boyko (2020). Factors associated with honey bee colony losses: A mini-review. Veterinary Sci. 7 (4), 166. doi:10.3390/vetsci7040166
Human, Hannelie, Brodschneider, Robert, Vincent, Dietemann, Dively, Galen, Ellis, James D., Forsgren, Eva, et al. (2013). Miscellaneous standard methods for Apis mellifera research. J. Apic. Res. 52 (4), 1–53. doi:10.3896/IBRA.1.52.4.10
Fries, Ingemar, Chauzat, Marie-Pierre, Chen, Yan-Ping, Doublet, Vincent, Genersch, Elke, Gisder, Sebastian, et al. (2013b). Standard methods for Nosema research. J. Apic. Res. 52 (1), 1–28. doi:10.3896/IBRA.1.52.1.14
Insolia, Luca, Molinari, Roberto, Rogers, Stephanie R., Williams, Geoffrey R., Chiaromonte, Francesca, and Calovi, Martina (2022). Honey bee colony loss linked to parasites, pesticides and extreme weather across the United States. Sci. Rep. 12 (1), 20787. doi:10.1038/s41598-022-24946-4
Karise, Reet, Muljar, Riin, Guy, Smagghe, Kaart, Tanel, Kuusik, Aare, Dreyersdorff, Gerit, et al. (2016). Sublethal effects of kaolin and the biopesticides prestop-mix and BotaniGard on metabolic rate, water loss and longevity in bumble bees (bombus terrestris). J. Pest Sci. 89 (1), 171–178. doi:10.1007/s10340-015-0649-z
Karise, Reet, Raimets, Risto, Bartkevics, Vadims, Pugajeva, Iveta, Pihlik, Priit, Keres, Indrek, et al. (2017). Are pesticide residues in honey related to oilseed rape treatments? Chemosphere 188 (December), 389–396. doi:10.1016/j.chemosphere.2017.09.013
Kasiotis, Konstantinos M., Anagnostopoulos, Chris, Anastasiadou, Pelagia, and Machera, Kyriaki (2014). Pesticide residues in honeybees, honey and bee pollen by LC–MS/MS screening: Reported death incidents in honeybees. Sci. Total Environ. 485–486 (July), 633–642. doi:10.1016/j.scitotenv.2014.03.042
Kestler, Paul (1991). Cyclic CO2 release as a physiological stress indicator in insects. Comp. Biochem. Physiology Part C Comp. Pharmacol. 100 (1–2), 207–211. doi:10.1016/0742-8413(91)90155-M
Kiljanek, Tomasz, Niewiadowska, Alicja, Gaweł, Marta, Semeniuk, Stanisław, Borzęcka, Milena, Posyniak, Andrzej, et al. (2017). Multiple pesticide residues in live and poisoned honeybees – preliminary exposure assessment. Chemosphere 175 (May), 36–44. doi:10.1016/j.chemosphere.2017.02.028
Kurze, Christoph, Mayack, Christopher, Frank, Hirche, Stangl, Gabriele I., Conte, Yves le, Kryger, Per, and Moritz, Robin F. A. (2016). Nosema spp. Infections cause No energetic stress in tolerant honeybees. Parasitol. Res. 115 (6), 2381–2388. doi:10.1007/s00436-016-4988-3
Mänd, Marika, Kuusik, Aare, Martin, Ants-Johannes, Williams, Ingrid H., Luik, Anne, Karise, Reet, et al. (2005). Discontinuous gas exchange cycles and active ventilation in pupae of the bumblebee bombus terrestris. Apidologie 36 (4), 561–570. doi:10.1051/apido:2005045
Mänd, Marika, and Karise, Reet. 2015. “Recent insights into sublethal effects of pesticides on insect respiratory physiology.” Open Access Insect Physiol., November, 5, 31. doi:10.2147/OAIP.S68870
EPILOBEE Consortium Chauzat, Marie-Pierre, Antoine, Jacques, Laurent, Marion, Bougeard, Stéphanie, Pascal, Hendrikx, and Ribière-Chabert, Magali (2016). Risk indicators affecting honeybee colony survival in Europe: One year of surveillance. Apidologie 47 (3), 348–378. doi:10.1007/s13592-016-0440-z
Martín-Hernández, Raquel, Botías, Cristina, Barrios, Laura, Martínez-Salvador, Amparo, Meana, Aránzazu, Mayack, Christopher, et al. (2011). Comparison of the energetic stress associated with experimental Nosema ceranae and Nosema Apis infection of honeybees (Apis mellifera). Parasitol. Res. 109 (3), 605–612. doi:10.1007/s00436-011-2292-9
Mayack, Christopher, and Naug, Dhruba (2009). Energetic stress in the honeybee Apis mellifera from Nosema ceranae infection. J. Invertebr. Pathology 100 (3), 185–188. doi:10.1016/j.jip.2008.12.001
Muljar, Riin, Karise, Reet, Viik, Eneli, Kuusik, Aare, Williams, Ingrid, Metspalu, Luule, et al. (2012). Effects of fastac 50 EC on bumble bee bombus terrestris L. Respiration: DGE disappearance does not lead to increasing water loss. J. Insect Physiology 58 (11), 1469–1476. doi:10.1016/j.jinsphys.2012.08.014
Naudi, Sigmar, Šteiselis, Juris, Jürison, Margret, Raimets, Risto, Tummeleht, Lea, Praakle, Kristi, et al. (2021). Variation in the distribution of Nosema species in honeybees (Apis mellifera linnaeus) between the neighboring countries Estonia and Latvia. Veterinary Sci. 8 (4), 58. doi:10.3390/vetsci8040058
Nicholls, Clara I., and Altieri, Miguel A. (2013). Plant biodiversity enhances bees and other insect pollinators in agroecosystems. A review. Agron. Sustain. Dev. 33 (2), 257–274. doi:10.1007/s13593-012-0092-y
Osterman, Julia, Aizen, Marcelo A., Biesmeijer, Jacobus C., Bosch, Jordi, Howlett, Brad G., Inouye, David W., et al. (2021). Global trends in the number and diversity of managed pollinator species. Agric. Ecosyst. Environ. 322 (December), 107653. doi:10.1016/j.agee.2021.107653
Paxton, Robert J., Klee, Julia, Korpela, Seppo, and Fries, Ingemar (2007). Nosema ceranae has infected Apis mellifera in Europe since at least 1998 and may Be more virulent than Nosema Apis. Apidologie 38 (6), 558–565. doi:10.1051/apido:2007037
Pettis, Jeffery S., vanEngelsdorp, Dennis, Johnson, Josephine, and Dively, Galen (2012). Pesticide exposure in honey bees results in increased levels of the gut pathogen Nosema. Naturwissenschaften 99 (2), 153–158. doi:10.1007/s00114-011-0881-1
Piot, Niels, Oliver, Schweiger, Meeus, Ivan, Orlando, Yañez, Straub, Lars, Villamar-Bouza, Laura, et al. (2022). Honey bees and climate explain viral prevalence in wild bee communities on a continental scale. Sci. Rep. 12 (1), 1904. doi:10.1038/s41598-022-05603-2
Ploomi, Angela, Kuusik, Aare, Jõgar, Katrin, Metspalu, Luule, Hiiesaar, Külli, Karise, Reet, et al. (2018). Variability in metabolic rate and gas exchange patterns of the Colorado potato beetle of winter and prolonged diapauses: Gas exchange patterns of Colorado potato beetle. Physiol. Entomol. 43 (4), 251–258. doi:10.1111/phen.12254
Potts, Simon G., Biesmeijer, Jacobus C., Kremen, Claire, Neumann, Peter, Oliver, Schweiger, William, E., et al. (2010). Global pollinator declines: Trends, impacts and drivers. Trends Ecol. Evol. 25 (6), 345–353. doi:10.1016/j.tree.2010.01.007
Potts, Simon G., Vera Imperatriz-Fonseca, Hien T. Ngo, Aizen, Marcelo A., Biesmeijer, Jacobus C., Breeze, Thomas D., Dicks, Lynn V., et al. (2016). Safeguarding pollinators and their values to human well-being. Nature 540 (7632), 220–229. doi:10.1038/nature20588
Raimets, Risto, Anna, Bontšutšnaja, Bartkevics, Vadims, Pugajeva, Iveta, Kaart, Tanel, Puusepp, Liisa, et al. (2020). Pesticide residues in beehive matrices are dependent on collection time and matrix type but independent of proportion of foraged oilseed rape and agricultural land in foraging territory. Chemosphere 238 (January), 124555. doi:10.1016/j.chemosphere.2019.124555
Roberts, Katherine E., and Hughes, William O. H. (2014). Immunosenescence and resistance to parasite infection in the honey bee, Apis mellifera. J. Invertebr. Pathology 121 (September), 1–6. doi:10.1016/j.jip.2014.06.004
Rodney, Sara, and Purdy, John (2020). Dietary requirements of individual nectar foragers, and colony-level pollen and nectar consumption: A review to support pesticide exposure assessment for honey bees. Apidologie 51 (2), 163–179. doi:10.1007/s13592-019-00694-9
Sánchez-Bayo, Francisco, Goulson, Dave, Pennacchio, Francesco, Nazzi, Francesco, Goka, Koichi, and Desneux, Nicolas. 2016. “Are bee diseases linked to pesticides? — a brief review.” Environ. Int. 89-90, 7–11. (April): 7–11. doi:10.1016/j.envint.2016.01.009
Schuehly, Wolfgang, Riessberger-Gallé, Ulrike, and López, Javier Hernández (2021). Sublethal pesticide exposure induces larval removal behavior in honeybees through chemical cues. Ecotoxicol. Environ. Saf. 228 (December), 113020. doi:10.1016/j.ecoenv.2021.113020
Shafeeque, Mohd Amir, Ahmad, Farhan, and Kamal, Aisha. n.d. “Toxicity of pesticides to plants and non-target organism: A comprehensive review.”https://ijpp.saveh.iau.ir/article_676795_9b346074d816b6175cffc49dfefc5052.pdf, 2020
Sharma, Anket, Kumar, Vinod, Shahzad, Babar, Tanveer, Mohsin, Sidhu, Gagan Preet Singh, Handa, Neha, et al. (2019). Worldwide pesticide usage and its impacts on Ecosystem. SN Appl. Sci. 1 (11), 1446. doi:10.1007/s42452-019-1485-1
Sinpoo, Chainarong, Paxton, Robert J., Disayathanoowat, Terd, Krongdang, Sasiprapa, and Chantawannakul, Panuwan (2018). Impact of Nosema ceranae and Nosema Apis on individual worker bees of the two host species (Apis cerana and Apis mellifera) and regulation of host immune response. J. Insect Physiology 105 (February), 1–8. doi:10.1016/j.jinsphys.2017.12.010
Straub, Lars, Minnameyer, Angela, Strobl, Verena, Kolari, Eleonora, Friedli, Andrea, Kalbermatten, Isabelle, et al. (2020). From antagonism to synergism: Extreme differences in stressor interactions in one species. Sci. Rep. 10 (1), 4667. doi:10.1038/s41598-020-61371-x
Tesovnik, Tanja, Zorc, Minja, Ristanić, Marko, Glavinić, Uroš, Stevanović, Jevrosima, Narat, Mojca, et al. (2020). Exposure of honey bee larvae to thiamethoxam and its interaction with Nosema ceranae infection in adult honey bees. Environ. Pollut. 256 (January), 113443. doi:10.1016/j.envpol.2019.113443
Trytek, Mariusz, Buczek, Katarzyna, Zdybicka-Barabas, Agnieszka, Wojda, Iwona, Borsuk, Grzegorz, Cytryńska, Małgorzata, et al. (2022). Effect of amide protoporphyrin derivatives on immune response in Apis mellifera. Sci. Rep. 12 (1), 14406. doi:10.1038/s41598-022-18534-9
vanEngelsdorp, Dennis, Evans, Jay D., Saegerman, Claude, Mullin, Chris, Haubruge, Eric, Nguyen, Bach Kim, et al. 2009. “Colony collapse disorder: A descriptive study.” Edited by Justin Brown. PLoS ONE 4 (8): e6481. doi:10.1371/journal.pone.0006481
vanEngelsdorp, Dennis, and Meixner, Marina Doris (2010). A historical review of managed honey bee populations in Europe and the United States and the factors that may affect them. J. Invertebr. Pathology 103 (January), S80–S95. doi:10.1016/j.jip.2009.06.011
Vidau, Cyril, Panek, Johan, Texier, Catherine, Biron, David G., Belzunces, Luc P., Le Gall, Morgane, et al. (2014). Differential proteomic analysis of midguts from Nosema ceranae-infected honeybees reveals manipulation of key host functions. J. Invertebr. Pathology 121 (September), 89–96. doi:10.1016/j.jip.2014.07.002
Vidau, Cyril, Diogon, Marie, Aufauvre, Julie, Fontbonne, Régis, Bernard, Viguès, Brunet, Jean-Luc, et al. 2011. “Exposure to sublethal doses of fipronil and thiacloprid highly increases mortality of honeybees previously infected by Nosema ceranae” edited by elizabeth didier. PLoS ONE 6 (6): e21550. doi:10.1371/journal.pone.0021550
Vinha, Lopes Germano, Plata-Rueda, Angelica, Soares, Marcus Alvarenga, Zanuncio, José Cola, Serrão, José Eduardo, Martinez, Luis Carlos, et al. (2021). Deltamethrin-mediated effects on locomotion, respiration, feeding, and histological changes in the midgut of spodoptera frugiperda caterpillars. Insects 12 (6), 483. doi:10.3390/insects12060483
Zheng, Huo-Qing, Lin, Zhe-Guang, Huang, Shao-Kang, Sohr, Alex, Wu, Lyman, and Chen, Yan Ping (2014). Spore loads may not Be used alone as a direct indicator of the severity of Nosema ceranae infection in honey bees Apis mellifera (Hymenoptera:Apidae). J. Econ. Entomology 107 (6), 2037–2044. doi:10.1603/EC13520
Keywords: owerlapping exposure, nosemosis, dimethoate (PubChem CID, 3082), metabolic rate, longevity, honeybee
Citation: Pent K, Naudi S, Raimets R, Jürison M, Liiskmann E and Karise R (2023) Overlapping exposure effects of pathogen and dimethoate on honeybee (Apis mellifera Linnaeus) metabolic rate and longevity. Front. Physiol. 14:1198070. doi: 10.3389/fphys.2023.1198070
Received: 31 March 2023; Accepted: 26 May 2023;
Published: 06 June 2023.
Edited by:
Priyadarshini Chakrabarti Basu, Mississippi State University, United StatesReviewed by:
Jevrosima Stevanovic, University of Belgrade, SerbiaPetar Hristov, Bulgarian Academy of Sciences, Bulgaria
Copyright © 2023 Pent, Naudi, Raimets, Jürison, Liiskmann and Karise. This is an open-access article distributed under the terms of the Creative Commons Attribution License (CC BY). The use, distribution or reproduction in other forums is permitted, provided the original author(s) and the copyright owner(s) are credited and that the original publication in this journal is cited, in accordance with accepted academic practice. No use, distribution or reproduction is permitted which does not comply with these terms.
*Correspondence: Kaarel Pent, a2FhcmVsLnBlbnRAZW11LmVl