- 1Department of Physiotherapy, University of Warmia and Mazury in Olsztyn, Olsztyn, Poland
- 2Institute of Health, Angelus Silesius University of Applied Sciences, Wałbrzych, Poland
- 3Doctoral School of Health Sciences, Faculty of Health Sciences, University of Pécs, Pécs, Hungary
- 4Department of Sport Sciences, Faculty of Education and Psychology, Eötvös Loránd University, Szombathely, Hungary
- 5Department Kinesiology and Health Prevention, Jan Dlugosz University in Czestochowa, Czestochowa, Poland
Background: Anthropometric and physiological characteristics are often considered as potential variables that are significantly related with motor performance.
Aim: The aim of this study was to identify and weigh the key anthropometric and physiological characteristics that are associated with 2000-m rowing ergometer performance in male and female athletes.
Methods: The study involved 70 best female and 130 best male rowers from the seven largest Hungarian rowing clubs, classified into one of the following age categories: juniors (36 women and 55 men, age range: 15-16 years), older juniors (26 women and 52 men, age range: 17-18 years), and seniors (8 women and 23 men, age range: over 18 years). Anthropometric and body composition measurements were determined by the bioelectrical impedance method proposed by Weiner and Lourie (1969), and skin fold measurements were conducted to estimate relative body fat content. The countermovement jump test and the 2000-m maximal rowing ergometer test were used for physiological measurements.
Results: An increase in skeletal muscle mass was correlated (r = -.39, p <.001) with a significant decrease in rowing time over a distance of 2000 m, whereas a significant increase in rowing time was noted with an increase in sitting height (only in men, r = .33, p <.001), body mass (in women and men: r = .24, p = .013 and r = .31, p = .009), and body fat percentage (r = .26, p < .030). Rowing time was also bound by a significant correlation with maximal force (r = -.79 and -.90, p <.001) and relative maximal power (r = -.54 and -.78, p <.001) in both sexes, with relative peak power in males (r = -.51, p < .001), and with estimated relative maximal aerobic capacity in females (r = -.43, p <.001).
Conclusion: Rowing performance over a distance of 2000 m is significantly negatively correlated with the skeletal muscle mass, maximal force, relative maximal power, relative peak power, and estimated relative maximal aerobic capacity.
Introduction
Athletes participating in various sports differ in anthropometric and physiological characteristics (APCh) (Stoop et al., 2018; Lau et al., 2020). The performance and APCh of male and female rowers have been studied extensively among both juniors (Bourgois et al., 2000; Claessens et al., 2002; Claessens et al., 2005; Alacid et al., 2011; Giroux et al., 2017) and seniors (Hebbelinck et al., 1980; Jürimäe et al., 2000; Battista et al., 2007; Forjasz, 2011; Durkalec-Michalski et al., 2019; Penichet-Tomás et al., 2019), although significantly more studies have been conducted on male than female rowers. Some researchers compared the APCh of rowers to the general population (Malina, 1994; Shephard, 1998; Bourgois et al., 2000; Schranz et al., 2010). Several studies analyzed the APCh of rowers from different age and rank groups (Mikulić, 2008; Klusiewicz et al., 2014; Podstawski et al., 2022), and they posited that this approach facilitates the selection of individuals with a predisposition for rowing (Alfoldi et al., 2021). However, there is a general scarcity of published studies on sexual dimorphism in rowing (Keenan et al., 2018; Podstawski et al., 2022).
The combination of both APCh is a good predictor of rowing performance (Jurimae and Jurimae, 2002), although some traits may play a greater role in rowing efficiency. Rowing is a strength endurance sport, where body size and body mass undoubtedly affect performance (Secher and Vaage, 1983; Jürimäe et al., 2010). According to Battista et al. (2007) and Penichet-Tomás et al. (2019), fat-free body mass, which is related primarily to skeletal muscle mass (SMM), is undoubtedly the key predictor of rowing performance, which could be attributed to the fact that high-performance rowers engage around 70% of all muscles when rowing a boat (Richter et al., 2010). Anthropometric studies also agree on the importance of body height as a determinant of rowing performance (Forijasz, 2011; Akça, 2014). The laws of physics and biomechanics play a key role in all sports that use oars, and they reveal the possibilities and limitations of the human body at the physical and anthropometric level (Penichet-Tomás et al., 2019). According to Shimoda et al. (2009), stroke power consistency contributes to the maintenance of power during ergometer rowing. However, opinions are divided on the extent to which various parts of the body contribute to rowing. According to Crosgrove et al. (1999), successful elite rowers generate around 75%–80% of power with the lower limbs and around 20%–25% with the arms during the rowing stroke. As regards the involvement of different muscle groups (such as antagonistic muscles) in rowing performance, rowers use mainly extensor muscles which, if lower limb muscles are inadequately trained, generate the force necessary to propel the boat (Ogurkowska et al., 2015). This is probably because that the increase in torso torque is much smaller in extensor muscles than flexor muscles (Podgórski et al., 2020). Therefore, considering both classifications, especially in sliding seat rowing, including the Olympic modality, lower limbs are the most important part of human body used in rowing compared to other rowing categories (Kollias et al., 2004), and the stroke range is greater in rowers with longer limbs (Claessens et al., 2005; Rakovac et al., 2011). Physiological and biomechanical characteristics undoubtedly influence rowing performance (Richter et al., 2010; De Larochelambert et al., 2020), and some of them play a significantly more important role and can be regarded as the main factors.
As regards physiological characteristics, aerobic metabolism covers 75%–80% of the demand for energy in a 2000-m rowing race (Jurišic et al., 2014). In view of the above, the anaerobic threshold and maximal oxygen uptake (VO2max) are most frequently used to diagnose, program, and control the training process in rowing (Mikulić, 2008; Klusiewicz et al., 2014; Martin and Tomescu. 2017). High anaerobic capacity is also crucial for high-level performance (Mäestu et al., 2005).
Models of body constitution and the physiological profiles of competitors participating in various competitive events have been described extensively in the literature (Bourgois et al., 2000; Fell and Gaffney, 2001; Claessens et al., 2002; Jürimäe and Jürimäe, 2002; Claessens et al., 2005; Slater et al., 2006; Mikulic, 2008; Forjasz and Kuchnio, 2009; Forjasz, 2011) and validated in studies of young elite rowers. However, to the best of our knowledge, very few studies have investigated anthropometric and physiological determinants together with the rowing pace in athletes aspiring to become elite rowers. A research methodology combining APCh with racing pace determinants could expand our understanding of rowing performance for the needs of the selection process. Therefore, the aim of this study was to identify and indicate anthropometric and physiological characteristics that are associated with 2000-m rowing ergometer performance in male and female athletes.
Materials and methods
Participants
Seventy best female and 130 best male rowers from the seven largest Hungarian rowing clubs participated in this study, which was conducted at Gyor rowing club over a period of four consecutive days in the middle of the racing season (10 days after one rowing regatta and 6 days before the following rowing regatta). The rowers regularly competed at both national and (in some cases) international events. The vast majority of the surveyed subjects were Hungarian Rowing Championship medalists in different categories. Rowers with an international ranking had won medals at European Championship regattas or had qualified for the finals in such regattas. The following inclusion criteria were used in the targeted sampling procedure in all age groups: rowers held a valid competition license and had participated in national and/or international competitions for at least 1 year. All rowers had valid medical certificates. They trained regularly, and their physical activity levels were not limited (for whatever reason) to the extent that could significantly affect their motor fitness. The training program was consistent with the training plan recommended by the Hungarian Rowing Federation: 12–13 h/week for 15- to 16-year-olds, 14–15 h/week for 17- to 18-year-olds, and 16–17 h/week for 19- to 22-year-olds. The aerobic-to-anaerobic training ratio in the above groups was 80:20%, 75:25%, and 70:30%, respectively. In addition, athletes who had received points for participating in international events attended training camps organized by the Hungarian Rowing Federation twice or three times a year (depending on the age group). Each rower was classified into one of the three age categories: juniors (36 women and 55 men, age range: 15–16 years), older juniors (26 women and 52 men, age range: 17–18 years), and seniors (8 women and 23 men, age range: over 18 years). In the senior group, both male and female athletes were relatively young, and the oldest rowers were only 22 years old. The trial was performed in compliance with the guidelines and policies of the Health Science Council, the Hungarian Scientific and Research Ethics Committee (IV/3067-3/2021/EKU), and the Declaration of Helsinki.
Each participant was provided with detailed information about the purpose of the study, potential risks, measurement methods, and motor test techniques that could be practiced during training sessions held directly before the study. All rowers gave voluntary informed consent to participate in the study by signing consent forms.
Procedures, data collection and equipment
At the beginning of the 2022 racing season, all participants attended anthropometric and physiological tests which were performed in the morning. The rowers were informed that they could have a light meal (800–1,200 kcals) consisting mainly of carbohydrates (60%–70%), but no later than 3–4 h before the trial (Williams, 1999). Anthropometric measurements were performed on days 1 and 2; the athletes participated in motor tests on day 3, and they rowed a distance of 2000 m on day 4. The rowers’ coaches in the sports clubs were instructed not to engage the subjects in any strenuous training the day before the trial, and they assisted the authors in performing the measurements. Body height was measured to the nearest 1 mm with a calibrated Soehlne Electronic Height Rod 5,003 (Soehlne Professional, Germany), in accordance with the relevant guidelines. Body mass (measured to the nearest 0.1 kg), BMI and body composition characteristics, including body fat percentage (BFP) and SMM, were determined by bioelectrical impedance with the InBody 720 (Biospace Co. Inc., Seoul, South Korea) Bioelectrical Impedance Analyzer (BIA). This foot-to-foot, hand-to-hand and hand-to-foot contact device features two stainless-steel foot pad electrodes mounted on a platform scale and a tetrapolar 8-point tactile electrode system with two stainless-steel handles. The reliability of bioelectrical-impedance analysis relative to other body composition measurement methods, such as DXA, has been successfully demonstrated (Sun et al., 2005; Gibson et al., 2008; Shafer et al., 2009). The platform scale uses a single load cell to measure body mass (and stature) and calculate the body mass index (BMI). The body fat percentage (PBF) is determined by summing the results of the segmental lean analysis to determine total lean body mass, fat mass, and the proportion of fat mass to total body mass. The muscle mass percentage (M%) is estimated by evaluating water content in the segmental regions with the use of the provided equations. The visceral fat area (VFA) is estimated with the use of regression equations (provided) which, according to the manufacturer, have been derived from a comparison of visceral fat in computerized tomography scans and impedance in the torso region in a segmental lean analysis. The remaining anthropometric characteristics, such as sitting height [cm], arm span [cm], limb length [cm], and BSA [m2], were measured using the methods proposed by Weiner and Lourie (1969). Skin fold measurements (biceps, triceps, scapula, suprailiac, abdomen, thigh, lower leg) were performed using a Harpenden caliper.
Estimation of relative body fat content
Relative body fat content was measured with a caliper based on the method developed by Pařízková (1961). According to this procedure, the thickness of five skinfolds is measured (over the biceps and triceps, subscapular, suprailiac and medial calf), and the sum of the obtained values is multiplied by 2; the product is then used to find the estimated relative body fat content in a table.
Countermovement jumping
The power output of the lower extremities and the height attained by the center of body mass during vertical jumps were measured with a PJS-4P60S force plate (JBA Zb. Staniak, Poland) with a 400 Hz sampling rate (Gajewski et al., 2018; Batra et al., 2021). The force plate was connected via an analog-to-digital converter to a PC with MVJ v.3.4 software (JBA Zb. Staniak, Poland). The amplifier was connected to a PC via an A/D converter. Measurements were performed using the MVJ v. 3.4 software package (JBA Zb. Staniak, Poland). The calculations relied on a physical model where the participants’ body mass served as a point that is affected by the vertical components of two external forces, namely the force of gravity acting on the body, and the platform’s reactive force. Each subject performed three countermovement jumps (CMJ) with maximal force. A CMJ is a vertical jump from a standing erect position, preceded by a counter movement of the upper limbs and lowering of the body mass center before take-off. Each participant was asked to perform a CMJ from the force plate to determine the maximal force [N] and the rate of displacement [m/s]. Jump height [cm] and peak power [W] were determined based on the above measurements. Jump height was calculated from ground reaction forces. Relative peak power [W/kg] was calculated based on each subject’s body mass.
2000-m maximal rowing ergometer test
The participants were asked to perform an all-out 2000-m test on a certified rowing ergometer (Concept 2 D). The ergometer screen was set to display the number of meters remaining, the average 500-m time, and the accumulated time. Power output in watts (W) was measured over 2000 m. Watts were calculated in a two-step procedure. In the first step, distance was defined as (time/number of strokes) × 500. In the next step, a “split” was defined as 500 × (time/distance). Watts were calculated as 2.8/(split/500). There were minor differences in intensity due to individual variations in stroke value and the ability to keep the 500 m split time constant. Prior to each test, the participants performed a 6-min warm-up over 500 m, followed by a 6-min rest that involved stretching exercises. The estimated relative aerobic capacity (ErVO2) was calculated based on the formula developed by McArdle et al. (2006) for men: ErVO2 = (Y × 1,000)/BM, where BM is body mass, and Y = [BM < 75kg; 15.1- (1.5 × time)]; BM => 75 kg; 15.7- (1.5 × time)]. The power delivered over 2000 m was divided by body mass to calculate relative performance (rW 2k).
Neither heart rate (HR) nor acid-base balance indicators, such as the blood concentrations of lactic acid, alkaline deficiency or excess, blood pH, and current molecular pressure of CO2, were measured in this study due to time and logistic constraints. A relatively high number of separate measurements had to be performed over three consecutive days to minimize interference with the athletes’ training program and changes in their overall body condition.
Statistical analysis
The results were processed statistically using Statistica PL v. 13.5 software. n-order parametric partial correlation coefficients were calculated. A partial correlation is a correlation between a pair of variables that accounts for their relationships with another (third) variable (first-order partial correlation) or several n) other variables (n-order partial correlation). This approach is applied to determine whether variables A and B are still correlated when the relationships between the remaining variables are eliminated (which corresponds to the assumption that the remaining variables have constant values). The level of p ≤ 0.005 was assumed as statistically significant values of the correlation coefficient. All examined and correlated features had a normal distribution, which was checked with the Shapiro-Wilk test.
A post-hoc power analysis was also performed for partial correlations in IBM SPSS Statistics for Windows, Version 28.0 (IBM Corp. Released 2021. Armonk, NY: IBM Corp.). The sample size is usually selected and the power analysis is usually conducted before data collection, but in the present study, this was not an intentional approach because the entire “population” of rowers who were eligible and available for the study was examined. Therefore, post-hoc power was calculated based on the sample size for the results of the partial correlation analysis. The calculated power denotes the sensitivity of the analysis. The observed power was calculated based on the size of male (n = 130) and female (n = 70) populations in a two-sided analysis, with an alternative hypothesis of 0.4 at α = 0.05 and α = 0.01. Depending on the type of the analysis, 3 to 8 partialled variables were eliminated (Table 1). The analysis had very high power in all cases.
Results
Analysis 1: Anthropometric characteristics
Only the parameters that were significantly correlated with the time taken to complete a distance of 2000 m on the rowing ergometer were used in the analysis. Skeletal muscle mass [%], which was not significantly correlated in males or females, and BFP, which was correlated only in males, were also considered (Table 2). Interestingly, none of the skinfold measurements were significantly correlated with the time taken to complete a distance of 2000 m in males or females. In both genders, rowing time (p-value ranged from .007 to .001) was significantly negative correlated with an age (r = −.66 and −.57) and anthropometric characteristics such as body height (r = −.70 and −.48), sitting height (r = −.67 and −.38), body mass (r = −.79 and −.68), BMI (r = −.57 and −.46, arm span (r = −.68 and r = −.51), and lower limb length (r = −.47 and −.35). In males, the time taken to complete a distance of 2000 m decreased significantly with a rise in BFP values (r = −.29, p = .002).
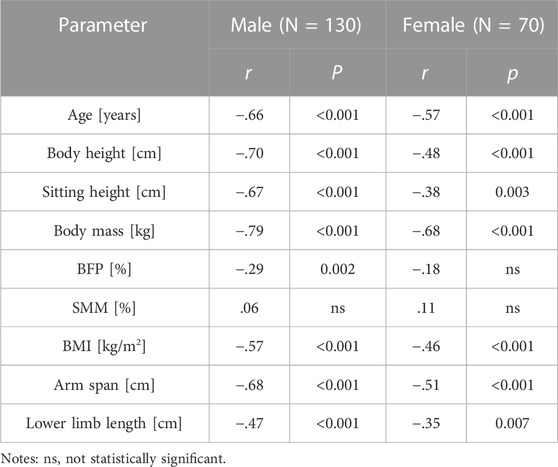
TABLE 2. Raw correlation coefficients between age and the studied anthropometric characteristics vs. the time taken to complete a distance of 2000 m on a rowing ergometer.
In most cases, anthropometric parameters and age were significantly positively correlated, which could considerably affect the real correlations between these factors and rowing time over a distance of 2000 m (Table 3).
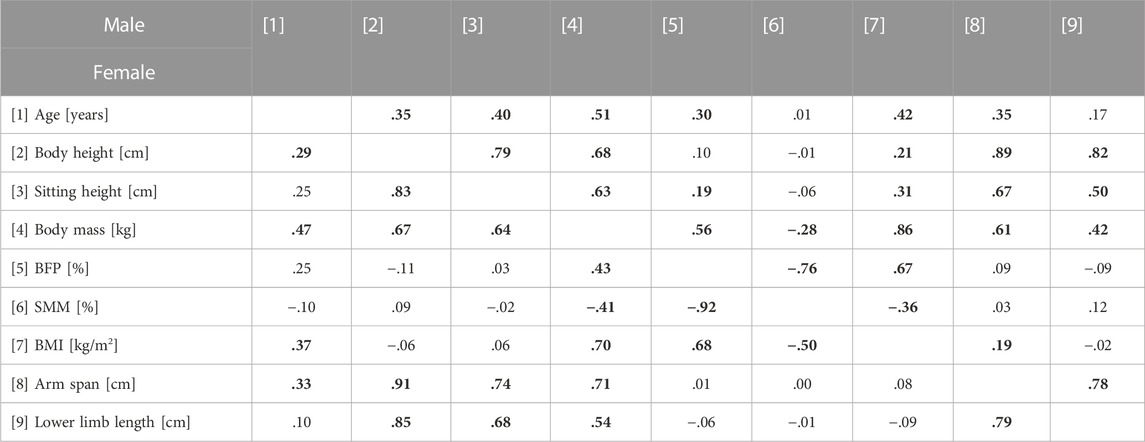
TABLE 3. Correlation coefficients between age and the studied anthropometric characteristics in males and females (values significant at p < 0.05 are marked in bold).
For this reason, partial correlation coefficients were calculated to control for the values of the remaining attributes (Table 4). The correlations between anthropometric parameters and the time taken to complete a distance of 2000 m on a rowing ergometer changed completely in both genders when the effects of the remaining variables were eliminated. In males, rowing time over a distance of 2000 m was significantly negatively correlated with SMM only (r = −.39, p < .001)—an increase in SMM was associated with shorter rowing time. Rowing time over a distance of 2000 m was significantly positively correlated with sitting height (r = .33, p < .001) and body mass (r = .24, p = .013)—an increase in the above anthropometric characteristics was associated with a significant increase in rowing time over a distance of 2000 m. In females, rowing time was significantly positively correlated with body mass (r = .31, p = .009) and BFP (r = .26, p < .030) only, which implies that an increase in the above anthropometric characteristics prolonged the time taken to complete 2000 m on the rowing ergometer. In males, rowing time was also significantly shortened with an increase in age.
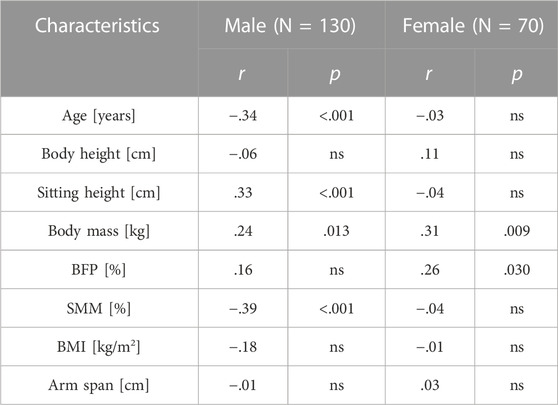
TABLE 4. Further-order partial correlations coefficients between the studied anthropometric characteristics and the time taken to complete a distance of 2000 m.
Analysis 2: Physiological characteristics
All measured physiological characteristics were considered in the analysis. In males, all physiological characteristics were bound by highly significant negative correlations with rowing time over a distance of 2000 m. In females, rowing time was significantly negatively correlated with only five physical characteristics (Power peak, RPP, ERGrelVO2max, ERGVO2max, Force max), whereas no significant correlations were noted for jump height, speed max, or RPM (Table 5). In both genders, the time taken to complete 2000 m on a rowing ergometer was significantly (p < .001) shortened with an increase in Powerpeak (r = −.98 and-.99), RPP (r = −.77 and −.76), ERGrelVO2max (r = −.56 and −.82), ERGVO2max (r = −.99) and forcemax (r = −.59 and −.52). In males, rowing time was also significantly shortened with an increase in jump height (r = −.36, p < .001), speed max (r = −.34, p < .001), and RPmax (r = −.27, p = .003).
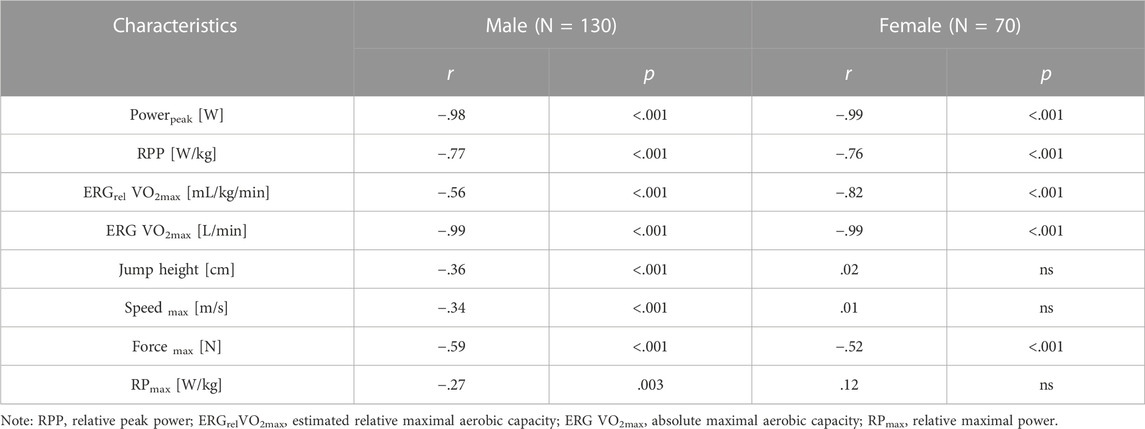
TABLE 5. Raw correlation coefficients between age and the studied physiological characteristics vs. the time taken to complete a distance of 2000 m.
In most cases, significant positive correlations were also observed between physiological characteristics (in particular in men), which could affect the real correlations between these attributes and the time taken to complete a distance of 2000 m (Table 6).
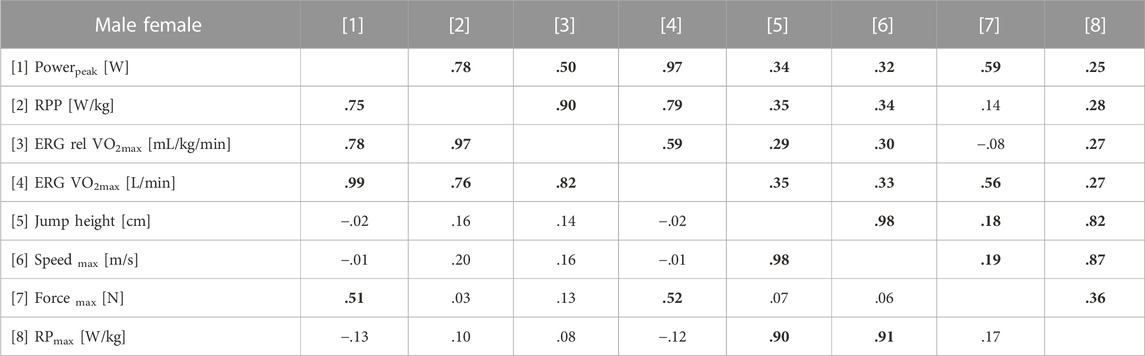
TABLE 6. Correlation coefficients between age and the studied physiological characteristics in males and females (values significant at p < 0.05 are marked in bold).
Therefore, partial correlation coefficients were calculated to control for the values of the remaining attributes (Table 7). It should be stressed that Powerpeak [W] and ERG VO2max [L/min] were not considered in the analysis because they were significantly correlated (r approximated 1.0) with rowing time over a distance of 2000 m. The above observation can be attributed to the fact that Powerpeak and ERG VO2max values were estimated based on rowing time over a distance of 2000 m (in particular ERG VO2max) and were highly correlated (Table 5). The calculated partial correlations would be completely different if these variables were included in the analysis. The correlations between the analyzed parameters and the time taken to complete a distance of 2000 m on a rowing ergometer changed completely in both genders when the effects of the remaining variables were eliminated. In both sexes (more so in women), rowing time over a distance of 2000 m was most significantly correlated with an increase in force max (r = −.79 and −.90, p < .001) and RPmax (r = −.54 and −.78, p < .001). In addition, the time needed to complete 2000 m on a rowing ergometer was significantly shortened with an increase in RPP in males (r = −.51, p < .001), and with an increase in ERGrel VO2max in females (r = −.43, p < .001).
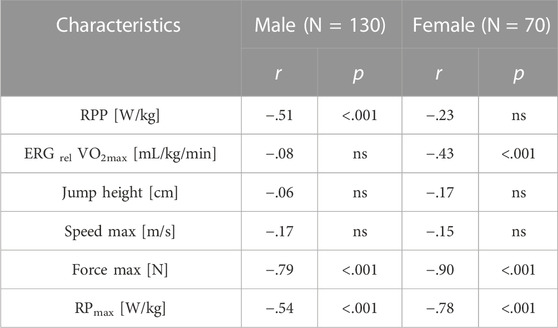
TABLE 7. Further-order partial correlations coefficients between the studied physiological characteristics and the time taken to complete a distance of 2000 m.
Discussion
The present study involved an original approach to interpreting the relationships between 2000-m rowing performance and APCh. The main limitation in research examining the correlations between APCh and motor performance stems from the fact that all variables are related to a certain degree, and the final result in a motor test is affected by numerous factors. The characteristics that significantly correlate with performance during a 2000-m rowing test are even more difficult to identify in young athletes who aspire to become elite rowers. The male and female rowers examined in this study were relatively young, and some of them had already achieved success in European regattas (including European championship), but none had participated in international championships or the Olympics. Young rowers aspiring to become elite athletes constitute a less homogeneous group than, for example, the finalists of the most prestigious regattas, and their performance can also be affected by factors that are unrelated to training (e.g., socioeconomic factors) (Alföldi et al., 2021; Podstawski et al., 2022). Such athletes train in different rowing clubs under the supervision of various trainers, which can also significantly affect their psychomotor development (Shipherd et al., 2019). Moreover, the influence of developmental factors is difficult to eliminate in rowers younger than 18, because the central and peripheral nervous systems are not yet fully anatomically and functionally developed at that age, and a certain imbalance can exist between excitatory and inhibitory neurons (van de Lagemaat et al., 2014; Tornero-Aguilera et al., 2022). Therefore, the procedure of selecting the most promising rowers is highly complex (Podstawski et al., 2022). During training practice, coaches play a key role in identifying the most talented athletes, and their judgement is often regarded as the “gold standard” against which scientific methods of talent identification are compared (Roberts et al., 2021).
In the present study, many variables were strongly correlated with the time of completing the 2000-m rowing performance test; therefore, factors that directly affect performance in rowing, a hybrid effort that requires high levels of both strength and endurance abilities, were difficult to identify (Lawton et al., 2013). The factors that exert the greatest impact on motor performance had been first identified in the 3-Minute Burpee Test (3-MBT) which evaluates endurance-strength abilities (Podstawski et al., 2016a; Podstawski et al., 2016b). In the group of the analyzed variables, only physical activity levels significantly correlated with the strength and endurance of moderately physically active young women and men during the 3-MBT (Borysławski et al., 2020). Despite the fact that 3-MBT and the rowing ergometer test involve similar types of effort, the results of these tests cannot be compared directly because the evaluated rowers, despite their relatively young age, are a more homogeneous and better trained group than university students. Rowers participate in strenuous training programs, most of which are developed for teams and groups in preparation for competitive events, and they are characterized by high and very similar PA levels (Mikulić, 2008; Alfoldi et al., 2021).
Anthropometric characteristics
In the current study, an increase in SMM in males was associated with a shorter rowing time over a distance of 2000 m. In contrast, greater sitting height and body mass were associated with a significant increase in rowing time over the same distance. The observation that greater body mass and sitting height can compromise rowing performance may seem surprising, as it is generally believed that heavier and taller individuals perform better in this sports discipline (Russel et al., 1998; Forjasz, 2011; Lawton et al., 2013). Despite the fact that sitting height is a component of body height, junior rowers had lower sitting height relative to stature (51.6%) and greater leg length relative to stature (48.4%) in comparison with the normative reference group (52.1% and 47.9% respectively) (Bourgois et al., 2000). Long lower limbs mainly increase the drive phase of the rowing stroke and generate the greatest power during rowing (Secher and Vaage, 1983). In females, higher body mass and BFP prolonged the time taken to complete 2000 m on the rowing ergometer. These results are consistent with the observations made by Battista et al. (2007) and Penichet-Tomás et al. (2019) who found that an increase in SMM with a simultaneous decrease in BFP are needed to improve 2000-m rowing performance. The significance of low BFP in conjunction with increased muscle mass had been previously emphasized in research conducted on elite and sub-elite juniors and seniors (Russel et al., 1998; Cosgrove et al., 1999; Yoshiga et al., 2000; Mikulić, 2008). Rowing is a hybrid sport that requires high strength and endurance abilities, which is why low BFP is essential for high-level rowing performance. Moreover, greater fat-free mass is associated with higher aerobic capacity which is crucial for successful rowing performance (Yoshiga and Higuchi, 2003). In studies designed to determine the optimal predictors of athletic performance (Ingham et al., 2002; Riechman et al., 2002), fat-free mass emerged as one of the strongest predictors of performance when anthropometric characteristics were measured. Therefore, long-term rowing-specific training programs combined with weight training significantly contribute to aerobic capacity, increased metabolic efficiency, as well as low BFP and increased muscle mass (Russel et al., 1998; Mikulić, 2008). Body fat expressed as both fat mass and fat percentage tends to be lower in juniors than in elite and sub-elite seniors, although it appears that BFP also decreases in elite rowers (Mikulić, 2008).
Physiological characteristics
The identified correlations indicate that Powerpeak [W] and ERGrel VO2max [L/min] were highly correlated with the time taken to complete the 2000-m rowing ergometer test by both women and men. These parameters were not considered in the partial correlation analysis presented in the Results section, but they will be discussed in this section because they considerably influence rowing performance (Jurišić et al., 2014; Egan-Shuttler et al., 2019). The results of basic analyses confirmed the well-known fact that an increase in total rowing power and VO2max expressed in L/min shortens the time to complete a 2000-m rowing ergometer test by both females (Battista et al., 2007; Klusiewicz et al., 2014; De Larochelambert et al., 2020) and males (Russel et al., 1998; Yoshiga and Higuchi, 2000; Fell and Gaffney, 2001; Ingham et al., 2002; Lawton et al., 2013). However, special attention should be paid to the relationships that emerged after the effects of the remaining variables had been eliminated in a partial correlation analysis. In both sexes, the time of completing the distance of 2000 m was most significantly shortened by an increase in Forcemax and RPmax (relative maximal power). Rowing time was also significantly shortened by an increase in relative peak power in males and an increase in ERGrel VO2max (in mL/min/kg) in females.
These findings indicate that relative values and their relationships with rowing performance should be also considered in statistical analyses to facilitate the selection of athletes with the optimal and relatively lowest BFP. Most research conducted on rowers analyzed absolute values, but the significance of relative peak power (Alfoldi et al., 2021; Podstawski et al., 2022) and realative VO2max (Mikulić, 2008; Alfoldi et al., 2021; Almeida-Neto et al., 2022; Papadakis et al., 2022; Podstawski et al., 2022) is increasingly recognized. During progressive exercise, individuals with higher BFP are characterized by higher oxygen demand, more rapid and shallow breathing, higher ventilatory demand, but a lower anaerobic threshold than athletes with higher lean body mass (Li et al., 2001; Vargas et al., 2018). As a result, lean body mass is closely related with the aerobic and anaerobic capacity of rowers (Durkalec-Michalski et al., 2019), which may be demonstrated during successive races in a regatta. During the finals, the performance of a rower with a higher BFP can decrease significantly relative to the results achieved during the qualifications. The phases of the rowing cycle and the differences between aerobic and anaerobic metabolism also play an important role in analyses of 2000-m rowing performance because these factors affect the production of cellular energy during physical effort. During the start, athletes use high stroke rates to increase the vessel’s displacement speed. Thus, speed is achieved and maintained mainly with the energy generated by the glycolytic pathway (Martin and Tomescu, 2017). During the central phase, the stroke rate decreases proportionally to the metabolic rate; the supply of energy from the glycolytic pathway is reduced, and most of the supplied energy is generated by aerobic pathways (between 65% and 75%). In the finishing phase, the stroke rate increases, and most energy is produced by anaerobic metabolic pathways (Held et al., 2020). Regardless of age and gender, total body mass and motor fitness levels can promote changes in the efficiency of cellular energy production in the human body (McNarry and Barker, 2018). However, biological maturation processes peak during adolescence, and the functioning of biological systems, including musculoskeletal and metabolic systems, is optimized during that period (Scheffler and Hermanussen, 2018). Therefore, the biological age is not always in sync with the chronological age and may be delayed or advanced (Moore et al., 2015). In metabolic terms, the efficiency with which cellular energy is produced by the oxidative pathway during physical exercise increases in advanced stages of biological maturation (Rowland, 2008; Ratel and Blazevich, 2017).
Almeida-Neto et al. (2022) found that advanced biological maturation in young elite rowers increased anaerobic power and decreased the time to complete the 2000-m rowing test both indoors and during competitive events. Dantas et al. (2018) found a significant relationship between the advancement of biological maturation and increased upper and lower limb power in rowers of both sexes. In the present study, higher chronological age was associated with a significantly shorter time of completing the 2000-m rowing test because the analysis involved both female and male rowers from different age groups (juniors to seniors).
Strengths and limitations
One of the strengths of this study was that a partial correlation analysis was used to identify the APCh that exert the greatest impact on the performance of young rowers during a 2000-m rowing ergometer test. The relatively small number of senior female rowers was a potential limitation. Mikulić (2008) observed that the collection of extensive data on well-trained subjects is seldom practical or possible due to the limited access to such subjects. Moreover, performance measurements were obtained in a controlled laboratory environment, and it is debatable whether a maximal performance test can be carried out on a rowing ergometer, and whether the results acquired in different age groups can be used to predict real-world ergometer performance (Mikulić and Ružić, 2008; Almeida-Neto et al., 2020). In the present study, only the participants’ chronological age was considered, but the correlations between biological age and the time to complete the 2000-m rowing ergometer test were not determined.
Conclusion
The results of this study, which involved a partial correlation analysis, suggest that the relative values of motor fitness indicators should be taken into account when describing the physiological profiles of rowers. The performance of both male and female rowers, including junior athletes, is not determined by a single factor, but several variables. Research has shown that rowing performance over a distance of 2000 m is significantly correlated by SMM, maximal force, relative maximal power, relative peak power, and estimated relative maximal aerobic capacity. In males, rowing time also decreased significantly with an increase in age, which confirms that older and more experienced athletes are more successful than juniors.
Data availability statement
The raw data supporting the conclusion of this article will be made available by the authors, without undue reservation.
Ethics statement
The studies involving human participants were reviewed and approved by Health Science Council, the Hungarian Scientific and Research Ethics Committee (IV / 3067-3 / 2021 / EKU). Written informed consent to participate in this study was provided by the participants’ legal guardian/next of kin.
Author contributions
RP and FI designed this study. Methodology: KB and RP. ZA and FI contributed to data collection. Software and validation were carried out under KB supervision. KB, FI, and RP analyzed and interpreted the data. RP, FI, ZA, and JW drafted the primary manuscript. RP, JW, and KB drafted the final version. All authors contributed to the article and approved the submitted version.
Funding
The funding for this research received from the University of Warmia and Mazury in Olsztyn.
Acknowledgments
The authors would like to thank all rowers who volunteered for the study.
Conflict of interest
The authors declare that the research was conducted in the absence of any commercial or financial relationships that could be construed as a potential conflict of interest.
Publisher’s note
All claims expressed in this article are solely those of the authors and do not necessarily represent those of their affiliated organizations, or those of the publisher, the editors and the reviewers. Any product that may be evaluated in this article, or claim that may be made by its manufacturer, is not guaranteed or endorsed by the publisher.
References
Akça, F. (2014). Prediction of rowing ergometer performance from functional anaerobic power, strength and anthropometric components. J. Hum. Kinet. 41, 133–142. doi:10.2478/hukin-2014-0041
Alacid, F., Marfell-Jones, M., López-Miñarro, P. A., Martínez, I., and Muyor, J. M. (2011). Morphological characteristics of young elite paddlers. J. Hum. Kinet. 27, 95–110. doi:10.2478/v10078-011-0008-y
Alföldi, Z., Borysławski, K., Ihasz, F., Soos, I., and Podstawski, R. (2021). Differences in the anthropometric and physiological profiles of Hungarian male rowers of various age categories, rankings and career lengths: Selection problems. Front. Physiol. 12, 1–11. doi:10.3389/fphys.2021.747781
Almeida-Neto, P. Fd., Silva, L. Fd., Matos, D. Gd., Jeffreys, I., Cesário, Td. M., Neto, R. B., et al. (2020). Equation for analyzing the peak power in aquatic environment: An alternative for olympic rowing athletes. PLoS ONE 15 (12), e0243157. doi:10.1371/journal.pone.0243157
Almeida-Neto, P. Fd., Silva, L. F. D., Miarka, B., De Medeiros, J. A., de Medeiros, R. Cd. S. C., Teixeira, R. P. A., et al. (2022). Influence of advancing biological maturation on aerobic and anaerobic power and on sport performance of junior rowers: A longitudinal study. Front. Physiol. 13, 892966. doi:10.3389/fphys.2022.892966
Batra, A., Wetmore, A. B., Hornsby, W. G., Lipinska, P., Staniak, Z., Surala, O., et al. (2021). Strength, Endocrine, and Body Composition Alterations across Four Blocks of Training in an Elite 400 m Sprinter. J. Funct. Morphol. Kinesiol. 25. doi:10.3390/jfmk6010025
Battista, R. A., Pivarnik, J. M., Dummer, G. M., Sauer, N., and Malina, R. M. (2007). Comparisons of physical characteristics and performances among female collegiate rowers. J. Sports Sci. 25 (6), 651–657. doi:10.1080/02640410600831781
Borysławski, K., Podstawski, R., Ihasz, F., and Żurek, P. (2020). The real determinants of power generation and maintenance during extreme strength endurance efforts: The 3-minute burpee test. T. S. S. 27 (2), 57–62. doi:10.23829/TSS.2020.27.2-2
Bourgois, J., Claessens, A. L., Vrijens, J., Philipaerts, R., Van Renterghem, B., Thomis, M., et al. (2000). Anthropometric characteristics of elite male junior rowers. Brit. J. Sports Med. 34, 213–217.
Claessens, A. L., Bourgois, J., Pintens, K., Lefevre, J., Van Renterghem, B., Philippaerts, R., et al. (2002). Body composition and somatotype characteristics of elite female junior rowers in relation to competition level, rowing style and boat type. Hum. Biol. Budapestinesis. Editor O. G. Eiben, and É. B. Bodzsár, Children and youth at the beginning of the 21st century 27, 159–165.
Claessens, A. L., Bourgois, J., Van Aken, K., Van der Auwera, R., Philippaerts, R., Thomis, M., et al. (2005). Body proportions of elite male junior rowers in relation to competition level, rowing style and boat type. Kinesiology 37(2), 123–132.
Cosgrove, M. J., Wilson, J., Watt, D., and Grant, S. F. (1999). The relationship between selected physiological variables of rowers and rowing performance as determined by a 2000 m ergometer test. J. Sport. Sci. 17, 845–852.
Dantas, M. P., Silva, L. F., Gantois, P., Silva, L. M., Dantas, R. N., and Cabral, B. T. (2018). Relationship between maturation and explosive strength in young rowers. Motricidade 14 (1), 112–121.
De Larochelambert, Q., Del Vecchio, S., Leroy, A., Duncombe, S., Toussaint, J.-F., and Sedeaud, A. (2020). Body and boat: Significance of morphology on elite rowing performance. Front. Sports Act. Living 2, 597676. doi:10.3389/fspor.2020.59767
Durkalec-Michalski, K., Nowaczyk, P. M., Podgórski, T., Kusy, K., Osiński, W., and Jeszka, J. (2019). Relationship between body composition and the level of aerobic and anaerobic capacity in highly trained male rowers. J. Sports Med. Phys. Fit. 59 (9), 1526–1535. doi:10.23736/S0022-4707.19.08951-5
Egan-Shuttler, J. D., Edmonds, R., Eddy, C., O’Neill, V., and Ives, S. J. (2019). Beyond peak, a simple approach to assess rowing power and the impact of training: A technical report. Int. J. Exerc Sci. 12 (6), 233–244.
Fell, J. W., and Gaffney, P. T. (2001). Physiological profiles of Australian surf boat rowers. J. Sci. Med. Sport. 4 (2), 188–195. doi:10.1016/S1440-2440(01)80029-3
Forjasz, J. (2011a). Somatic build of rowers in the period from 1995 to 2005. Hum. Mov. 12 (1), 47–57. doi:10.2478/v100038-011-0004-z
Forjasz, J. (2011b). Anthropometric typology of male and female rowers using K-means clustering. J. Hum. Kinet. 28, 155–164. doi:10.2478/v10078-011-0032-y
Forjasz, J., and Kuchnio, M. (2009). Factors structure of morphological variability in male and female rowers. Studies in Physical Culture and Tourism 16 (3), 249–257.
Gajewski, J., Michalski, R., Buśko, K., Mazur-Różycka, J., and Staniak, Z. (2018). Countermovement depth – a variable which clarifies the relationship between the maximum power output and height of a vertical jump. Acta Bioeng. Biomech 20, 1. doi:10.5277/ABB-01058-2017-02
Gibson, A. L., Holmes, J. C., Desautels, R. L., Edmonds, L. B., and Nuudi, L. (2008). Ability of new octapolar bioimpedance spectroscopy analyzers top redict 4-component-model percentage body fat in Hispanic, black, and white adults. Am. J. Clin. Nutr. 87 (2), 332–338. doi:10.1093/ajcn/87.2.332
Giroux, C., Maciejewski, H., Ben-Abdessamie, A., Chorin, F., Lardy, J., Ratel, S., et al. (2017). Relationship between force-velocity profiles and 1,500-m ergometer performance in young rowers. Int. J. Sports Med. 38 (13), 992–1000. doi:10.1055/s-0043-117608
Hebbelinck, M., Ross, W. D., Carter, J. E. L., and Morms, J. (1980). Anthropometric characteristics of female Olympic rowers. Can. J. Appl. Sci. 5, 255–262.
Held, S., Siebert, T., and Donath, L. (2020). Changes in mechanical power output in rowing by varying stroke rate and gearing. Eur. J. Sport Sci. 20 (3), 357–365. doi:10.1080/17461391.2019.1628308
Ingham, S. A., Whyte, G. P., Jones, K., and Nevill, A. M. (2002). Determinants of 2,000 m rowing ergometer performance in elite rowers. Eur. J. Appl. Physiol. 88, 243–246. doi:10.1007/s00421-002-0699-9
Jürimäe, J., and Jürimäe, T. A. (2002). Comparison of selected anthropometric, metabolic and hormone parameters in lightweight and open class rowers. Biol. Sport. Zugriff unter. Available at: http://www.ausport.gov.au/fulltext/2000/preoly/abs541.htm 19, 149–161.
Jürimäe, J., Mäestu, J., and Jürimäe, T. (2000). Prediction of rowing performance on single sculls from metabolic and anthropometric variables. J. Hum. Mov. Stud. 38, 126–138.
Jürimäe, T., Perez-Turpin, J. A., Cortell-Tormo, J. M., Chinchilla-Mira, I. J., Cejuela-Anta, R., Mäestu, J., et al. (2010). Relationship between rowing ergometer performance and physiological responses to upper and lower exercises in rowers. J. Sci. Med. Sport 13, 434–437. doi:10.1016/j.jsams.2009.06.003
Jurišić, D., Donadić, Z., and Lozovina, M. (2014). Relationship between maximum oxygen uptake and anaerobic threshold, and the rowing ergometer results in senior rowers. Acta Kinesiol. 8 (2), 55–61.
Keenan, K. G., Senefeld, J. W., and Hunter, S. K. (2018). Girls in the boat: Sex differences in rowing performance and participation. PloS One 13 (1), e0191504. doi:10.1371/journal.pone.0191504
Klusiewicz, A., Starczewski, M., Ładyga, M., Długołęcka, B., Braksator, W., Mamcarz, A., et al. (2014). Reference values of maximal oxygen uptake for Polish rowers. J. Hum. Kinet. 14, 121–127. doi:10.2478/hukin-2014-0117
Kollias, I., Panoutsakopoulos, V., and Papaiakovou, G. (2004). Comparing jumping ability among athletes of various sports: Vertical drop jumping from 60 centimeters. J. Strength Cond. Res. 18, 546–550. doi:10.1519/1533-4287(2004)18<546:CJAAAO>2.0.CO;2
Lau, J. S., Ghafar, R., Hashim, H. A., and Zulkifli, E. Z. (2020). Anthropometric and physical fitness components comparison between high- and low-performance archers. Ann. Appl. Sport Sci. 8 (2). doi:10.29252/aassjournal.897
Lawton, T. W., Cronin, J. B., and McGuigan, M. R. (2013). Strength, power, and muscular endurance exercise and elite rowing ergometer performance. J. Strength Cond. Res. 27 (7), 1928–1935. doi:10.1519/JSC.0b013e3182772f27
Li, J., Li, S., Feuers, R. J., Buffington, C. K., and Cowan, G. S. (2001). Influence of body fat distribution on oxygen uptake and pulmonary performance in morbidly obese females during exercise. Respirology 6 (1), 9–13. doi:10.1046/j.1440-1843.2001.00290.x
Mäestu, J., Jürimäe, J., and Jürimäe, T. (2005). Monitoring of performance and training in rowing. Sports Med. 35 (7), 597–617. doi:10.112-1642/05/0007-0597/$34.95/0
Malina, R. M. (1994). Physical activity and training: e vects on stature and the adolescent growth spurt. Med. Sci. Sport. Exerc. 26, 759–766.
Martin, S. A., and Tomescu, V. (2017). Energy systems efficiency influences the results of 2,000 m race simulation among elite rowers. Clujul. Med. 290 (1), 60–65. doi:10.15386/cjmed-675
McArdle, W. D., Katch, F. I., and Katch, V. L. (2007). Exercise physiology energy, nutrition, and human performance. 7th Edn. (Philadelphia: Lippincott).
Mc Narry, M. A., and Barker, A. R. (2018). “Aerobic and anaerobic metabolism,” in Elite youth cycling (England, UK: Routledge Press), 49–69. doi:10.4324/9781315110776-3
Mikulić, P. (2008). Anthropometric and physiological profiles of rowers of varying ages and ranks. Kinesiology 40, 80–88.
Mikulić, P., and Ružić, L. (2008). Predicting the 1000 m rowing ergomneter performance in 12 – 13-year-old rowers: The basis for selection process? J. Sci. Med. Sport. 11, 218–226. doi:10.1016/j.jsams.2007.01.008
Moore, S. A., McKay, H. A., Macdonald, H., Nettlefold, L., Baxter-Jones, A. D. G., Cameron, N., et al. (2015). Enhancing a somatic maturity prediction model. Med. Sci. Sports Exerc. 47 (8), 1755–1764. doi:10.1249/MSS.0000000000000588
Ogurkowska, M. B., Kawałek, K., and Zygmańska, M. (2015). Biomechanical characteristics of rowing. T. S. S. 2, 61–69.
Papadakis, Z., Etchebaster, M., and Garcia-Retortillo, S. (2022). Cardiorespiratory coordination in collegiate rowing: A network approach to cardiorespiratory exercise testing. Int. J. Environ. Res. Public Health 19, 13250. doi:10.3390/ijerph192013250
Penichet-Tomás, A., Pueo, B., and Jiménez-Olmedo, M. (2019). Physical performance indicators in traditional rowing championships. J. Sports Med. Phys. Fit. 59 (5), 767–773. doi:10.23736/s0022-4707.18.08524-9
Podgórski, T., Nowak, A., Domaszewska, K., Mączyński, J., Jabłońska, M., Janowski, J., et al. (2020). Muscle strength and inflammatory response to the training load in rowers. Peer J. 8, e10355. doi:10.7717/peerj.10355
Podstawski, R., Markowski, P., Choszcz, D., and Żurek, P. (2016a). Correlations between anthropometric indicators, heart rate and endurance-strength abilities during high-intensity exercise of young women. Arch. Budo Sci. Martial Art. Extreme Sport 12, 17–24.
Podstawski, R., Borysławski, K., Ihasz, F., Pomianowski, A., Wąsik, J., and Gronek, P. (2022). Comparison of anthropometric and physiological profiles of Hungarian female rowers across age categories, rankings, and stages of sports career. Appl. Sciences-Basel 12 (5), 1–11. doi:10.3390/app12052649
Podstawski, R., Borysławski, K., Ihasz, F., Pomianowski, A., Wąsik, J., and Gronek, P. (2022). Comparison of anthropometric and physiological profiles of Hungarian female rowers across age categories, rankings, and stages of sports career. Appl. Sci. 12, 2649. doi:10.3390/app12052649
Podstawski, R., Borysławski, K., Katona, Z. B., Alfoldi, Z., Boraczyński, M., Jaszczur-Nowicki, J., et al. (2022). Sex differences in anthropometric and physiological profiles of Hungarian rowers of different ages. Int. J. Environ. Res. Public Health 19 (13), 1–15. doi:10.3390/ijerph19138115
Podstawski, R., Markowski, P., Choszcz, D., Klimczak, J., Romero Ramos, O., and Merino Marban, R. (2016b). Methodological aspect of evaluation of the reliability the 3-Minute Burpee Test. Arch. Budo Sci. Martial Art. Extreme Sport 12, 137–144. AoBID: 11441.
Rakovac, M., Smoljanović, T., Bojanić, I., Hannafin, J. A., Hren, D., and Thomas, P. (2011). Body size changes in elite junior rowers: 1997 to 2007. Coll. Antropol. 35, 127–131.
Ratel, S., and Blazevich, A. J. (2017). Are prepubertal children metabolically comparable to well-trained adult endurance athletes? Sports Med. 47 (8), 1477–1485. doi:10.1007/s40279-016-0671-1
Richter, C., Hamilton, S., and Roemer, K. (2010). “Influence of body mass index on rowing kinematics,” in 28th international symposium on biomechanics in sports. Section: Coaching and Sports Activities, June 30-July 6, 1999.
Riechman, S. E., Zoeller, R. F., Balasekaran, G., Goss, F. L., and Robertson, R. J. (2002). Prediction of 2000 m indoorrowing performance using a 30 s sprint and maximal oxygen uptake. J. Sports Sci. 20, 681–687. doi:10.1080/026404102320219383
Roberts, A. H., Greenwood, D., Stanley, M., Humberstone, C., Iredale, F., and Raynor, A. (2021). Understanding the “gut instinct” of expert coaches during talent identification. J. Sports Sci. 39 (4), 359–367. doi:10.1080/02640414.2020.1823083
Russel, A. P., le Rossignol, P. F., and Sparrow, W. A. (1998). Prediction of elite schoolboy 2000 m rowing ergometer performance from metabolic, anthropometric and strength variables. J. Sports Sci. 16, 749–754.
Scheffler, C., and Hermanussen, M. (2018). Growth in childhood and adolescence. Int. Encycl. Biol. Anthropol. 2018, 1–11. doi:10.1002/9781118584538.ieba0537
Schranz, N., Tomkinson, G., Olds, T., and Daniell, N. (2010). Three-dimensional anthropometric analysis: Differences between elite Australian rowers and the general population. J. Sports Sci. 28, 459–469. doi:10.1080/02640411003663284
Secher, N. H., and Vaage, O. (1983). Rowing performance, a mathematical model based on analysis of body dimensions as exemplified by body weight. Eur. J. Appl. Physiol. 52, 88–93.
Shafer, K. J., Siders, W. A., Johnson, L. K., and Lukaski, H. C. (2009). Validity of segmental multiple-frequency bioelectrical impedance analysis to estimate body composition of adults across a range of body mass indexes. Nutrition 25 (1), 25–32. doi:10.1016/j.nut.2008.07.004
Shimoda, M., Fukunaga, T., Higuchi, M., and Kawakami, Y. (2009). Stroke power consistency and 2000 m rowing performance in varsity rowers. Scand. J. Med. Sci. Sports 19, 83–86. doi:10.1111/j.1600-0838.2007.00754.x
Shipherd, A. M., Wakefield, J. C., Stokowski, S., and Filho, E. (2019). The influence of coach turnover on student-athletes’ affective states and team dynamics: An exploratory study in collegiate sports. Int. J. Sports Sci. Coach. 14 (1). doi:10.1177/1747954118766465
Slater, G. J., Rice, A. J., Jenkins, D., Gulbin, J., and Hahn, A. G. (2006). Preparation of former heavyweight oarsmen to compete as lightweight rowers over 16 weeks: Three case studies. Int. J. Sport Nutr. Exerc. Metab. 16, 108–121. doi:10.1123/ijsnem.16.1.108
Stoop, R., Hohenauer, E., Rucker, A. M. L., and Clijsen, R. (2018). Anthropometric properties versus physical performance in rugby:union: Forwards and backs - a systematic review. Appl. Sport Sci. 6 (2), 1–13. doi:10.29252/aassjournal.6.2.1
Sun, G., French, C. R., Martin, G. R., Younghusband, B., Green, R. C., Xie, Y. G., et al. (2005). Comparison of multifrequency bioelectrical impedance analysis with dual-energy X-ray absorptiometry for assessment of percentage body fat in a large, healthy population. Am. J. Clin. Nutr. 81 (1), 74–78. doi:10.1093/ajcn/81.1.74
Tornero-Aguilera, J. F., Jimenez-Morcillo, J., Rubio-Zarapuz, A., and Clemente-Suárez, V. J. (2022). Central and peripheral fatigue in physical exercise explained: A narrative review. Int. J. Environ. Res. Public Health 19, 3909. doi:10.3390/ijerph19073909
van de Lagemaat, L. N., Nijhof, B., Bosch, D. G. M., Kohansal-Nodehi, M., Keerthikumar, S., and Heimel, J. A. (2014). Age-related decreased inhibitory vs. excitatory gene expression in the adult autistic brain. Front. Neurosci. Sec. Neurogenomics 2014, 8. doi:10.3389/fnins.2014.00394
Vargas, V. Z., de Lira, C. A. B., Rayes, A. B. R., Vancini, R. L., and Andrade, M. S. (2018). Fat mass is negatively associated with the physiological ability of tissue to consume oxygen. Mot. Rio Claro 24 (4), e101808. doi:10.1590/S1980-6574201800040010
Weiner, J. E. S. S., and Lourie, J. A. (Editors) (1969). Human Biology. A Guide to Field Methods. IBDP Handbook, No. 9. Oxford: Blackwell Scientific Publishers.
Williams, M. H. (1999). Nutrition for Health, Fitness, and Sport. 5th Edn. (New York, NY: McGraw - Hill).
Yoshiga, C. C., and Higuchi, M. (2003). Oxygen uptake and ventilation during rowing and running in females and males. Scand. J. Med. Sci. Sports 13, 359–363. doi:10.1046/j.1600-0838.2003.00324.x
Keywords: rowing performance, different age, somatic features, physiological parameters, sex categorization
Citation: Podstawski R, Borysławski K, Alföldi Z, Ferenc I and Wąsik J (2023) The effect of confounding variables on the relationship between anthropometric and physiological features in 2000-m rowing ergometer performance. Front. Physiol. 14:1195641. doi: 10.3389/fphys.2023.1195641
Received: 28 March 2023; Accepted: 16 May 2023;
Published: 30 May 2023.
Edited by:
Stevo Popovic, University of Montenegro, MontenegroReviewed by:
Seyed Morteza Tayebi, Allameh Tabataba’i University, IranJarosław Domaradzki, Wroclaw University of Health and Sport Sciences, Poland
Copyright © 2023 Podstawski, Borysławski, Alföldi, Ferenc and Wąsik. This is an open-access article distributed under the terms of the Creative Commons Attribution License (CC BY). The use, distribution or reproduction in other forums is permitted, provided the original author(s) and the copyright owner(s) are credited and that the original publication in this journal is cited, in accordance with accepted academic practice. No use, distribution or reproduction is permitted which does not comply with these terms.
*Correspondence: Robert Podstawski, cG9kc3Rhd3NraXJvYmVydEBnbWFpbC5jb20=