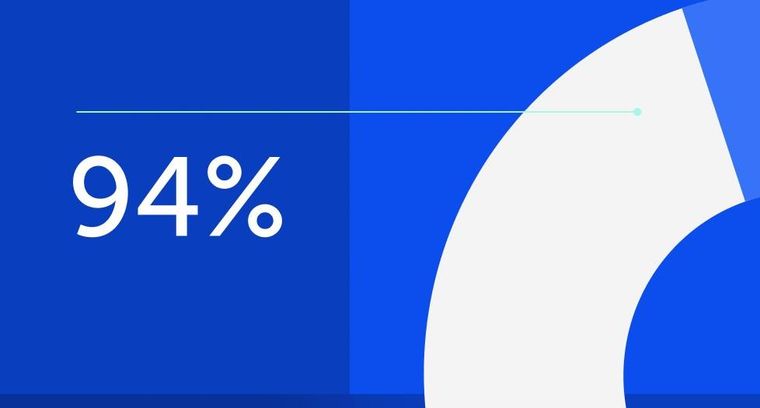
94% of researchers rate our articles as excellent or good
Learn more about the work of our research integrity team to safeguard the quality of each article we publish.
Find out more
EDITORIAL article
Front. Physiol., 11 April 2023
Sec. Mitochondrial Research
Volume 14 - 2023 | https://doi.org/10.3389/fphys.2023.1192759
This article is part of the Research TopicMuscle Plasticity: Regulation of adaptive changes in muscles and mitochondrial dynamicsView all 5 articles
Editorial on the Research Topic
Muscle plasticity: Regulation of adaptive changes in muscles and mitochondrial dynamics
Skeletal muscle comprises long, cylindrical muscle fibers that are multinucleated and striated, i.e., they appear striped under a microscope (Frontera and Ochala, 2015). Skeletal muscles are under voluntary control and mediate walking, running, jumping, breathing, and lifting weights. They work with tendons, which attach muscle to bone, and neurons, which signal the muscle fibers to contract (Widmaier and Vander, 2019). Skeletal muscles also play key roles in glucose, protein, and lipid metabolism, and they contribute approximately 40% of total body weight and 50%–75% of all proteins in the body (Frontera and Ochala, 2015; Saladin et al., 2018). Muscle mass depends on the balance between protein synthesis and degradation. Both processes are sensitive to nutritional status, hormonal equilibrium, physical activity/exercise, and injury or disease (Frontera and Ochala, 2015; Saladin et al., 2018).
Skeletal muscles can modify their size in response to use (Frontera and Ochala, 2015; Jorgenson et al., 2020) and this impressive plasticity in metabolism and function can be linked to organelles, such as mitochondria, peroxisomes, and endoplasmic reticulum. While researchers have made significant strides in understanding the regulation of muscle mass and mitochondrial metabolism in physiological and pathophysiological conditions, further investigation is needed to uncover the mechanisms underlying muscle plasticity. This editorial article introduces our Research Topic, which aims to provide new insights into muscle plasticity and mitochondrial metabolism. The featured articles showcase the role of teneurin C-terminal associated peptide (TCAP-1) in regulating mitochondrial metabolism and muscle contraction (Hogg et al.), introduce a novel method to rapidly isolate functional mitochondria (Younis et al.), and review the role of exercise and mitochondrial mechanisms in sarcopenia (Alizadeh Pahlavani et al.) and the effect of acute exercise on muscle protein turnover (Hajj-Boutros et al.).
Hogg et al. unveiled a new mechanism for regulating skeletal muscle dynamics through TCAP-1 action. TCAP-1 modulates energy metabolism in skeletal muscle via an insulin-independent pathway, which affects contractile kinetics through changes in Ca2+ dynamics and ATP production. The authors identified interactions between TCAP-1 and LPHN-1 and LPHN-3, which activates G-protein-coupled PLC, leading to subsequent changes in intracellular IP3 and DAG levels. Additionally, TCAP-1 increases cellular energy availability by promoting glucose importation into cells, likely due to increased GLUT4 expression.
Younis et al. presented a new method for rapidly and efficiently isolating intact, respiring mitochondria from cultured skeletal muscle cells and murine tissue. This protocol offers high yield and purity of mitochondria with minimal contamination from other cell fractions by using nitrogen cavitation and rapid differential centrifugation to lyse cell membranes and isolate mitochondria. Further, this method allows for low variation in respiratory control ratio, with a ± 0.2 and 0.8 standard error of mean in isolated mitochondria from tissue and cells, respectively, making it highly suitable for high-resolution respiratory studies. This new protocol offers a significant advantage for researchers working with skeletal muscle tissues, particularly for respiratory studies and proteome analysis. The protocol will facilitate downstream mitochondrial evaluation, with important implications for advancing our understanding of human health and disease.
Sarcopenia, the loss of muscle mass and function in the elderly, significantly contributes to reduced quality of life in older individuals (Romanello, 2020). Alizadeh Pahlavani et al. provide a thorough explanation of exercise intervention and mitochondrial mechanisms in sarcopenia muscles. This article reviews the evidence linking physical inactivity, reduced antioxidant defenses and impaired mitochondrial function in sarcopenia. This review also explains that age-associated disruption in multiple mitochondrial quality control processes, including mitochondrial biogenesis, fusion, fission, and mitophagy are important contributors to sarcopenia. This review highlights exercise as the most effective way to improve mitochondrial function and biogenesis, as well as to reduce oxidative stress in aging muscles. Exercise can activate signals that increase the expression of critical genes involved in mitochondrial biogenesis (i.e., PGC-1α, PGC-1β, TFAM, and Nrf-1), thereby improving mitochondrial biogenesis, which is essential for preserving muscle function in the elderly. Alizadeh Pahlavani et al. also review the evidence that exercise positively impacts mitochondrial dynamics (fusion and fission) and mitophagy, which are mechanisms that likely contribute to improving mitochondrial and muscle function in the elderly. Overall, the article describes in detail how sarcopenia is a complex condition involving oxidative stress, impaired mitochondrial function, and impaired mitochondrial quality control processes, and illustrates how exercise can combat sarcopenia, by increasing both mitochondrial and muscle quality, thus improving overall health and wellbeing.
The review article by Hajj-Boutros et al. details the mechanisms involved in muscle protein turnover after acute exercise, focusing on the acute effects of resistance exercise (RE) and high-intensity interval training (HIIT) on muscle protein synthesis and breakdown. The review notes that older adults generally have a reduced response to exercise compared with younger individuals, with a subtle increase in their muscle protein synthesis (MPS) rate following an acute bout of exercise. The review describes the effect of RE on MPS and the signaling pathways involved, such as insulin-like growth factor-1 (IGF-1) and its downstream targets, which increase MPS. RE is also a potential activator of the integrin pathway and is involved in MPS through extracellular signal-regulated kinase (ERK1/2). Reduced stimulation of both pathways might underlie diminished or delayed MPS in elderly adults. Elderly adults can still gain muscle mass through RE, but the process is slower due to a decreased ability to respond to exercise stimuli. RE and endurance exercise (EE) result in different muscular and cellular adaptations and the duration of exercise determines the positive effects induced by RE and EE. In older adults, HIIT can induce muscle hypertrophy to a certain degree by potentially stimulating MPS, while RE + HIIT can improve muscle strength, functional capacity, and aerobic capacity. Further, the combination of RE + HIIT significantly increased markers of mitochondrial biogenesis compared to RE alone, but downstream signaling molecules were not affected, indicating attenuated protein synthesis. Overall, examining the individual response to exercise has become more complex, especially in aged populations, and predicting how people will respond to exercise is challenging. This review suggests further research is needed to examine the effects of RE + HIIT on changes in muscle mass.
This Research Topic provides fundamental insights into the determinants of muscle plasticity and mitochondrial activity. These research findings hold significant promise for developing therapeutic strategies to promote and enhance muscle health. Furthermore, this Research Topic contributes to understanding the intricate molecular mechanisms that govern healthy mitochondrial function in muscle and the complex processes underlying sarcopenia and protein turnover in skeletal muscle.
PK wrote the manuscript, PK, AH, NS, and GG edited the manuscript. All authors contributed significantly to critically revising it for intellectual content and have approved its publication.
The authors declare that the research was conducted in the absence of any commercial or financial relationships that could be construed as a potential conflict of interest.
All claims expressed in this article are solely those of the authors and do not necessarily represent those of their affiliated organizations, or those of the publisher, the editors and the reviewers. Any product that may be evaluated in this article, or claim that may be made by its manufacturer, is not guaranteed or endorsed by the publisher.
Frontera, W. R., and Ochala, J. (2015). Skeletal muscle: A brief review of structure and function. Calcif. Tissue Int. 96, 183–195. doi:10.1007/s00223-014-9915-y
Jorgenson, K. W., Phillips, S. M., and Hornberger, T. A. (2020). Identifying the structural adaptations that drive the mechanical load-induced growth of skeletal muscle: A scoping review. Cells 9. doi:10.3390/cells9071658
Romanello, V. (2020). The interplay between mitochondrial morphology and myomitokines in aging sarcopenia. Int. J. Mol. Sci. 22, 91. doi:10.3390/ijms22010091
Saladin, K. S., Gan, C. A., and Cushman, H. N. (2018), Anatomy and physiology: The unity of form and function. Eighth edition. edn (McGraw-Hill Education).
Keywords: mitochondria, sarcopenia, TCAP-1, HIIT, resistant exercise, muscles
Citation: Katti P, Kim Y, Sundaresan NR, Gouspillou G and Hinton A Jr.(2023) Editorial: Muscle plasticity: Regulation of adaptive changes in muscles and mitochondrial dynamics. Front. Physiol. 14:1192759. doi: 10.3389/fphys.2023.1192759
Received: 23 March 2023; Accepted: 04 April 2023;
Published: 11 April 2023.
Edited and reviewed by:
Amadou K. S. Camara, Medical College of Wisconsin, United StatesCopyright © 2023 Katti, Kim, Sundaresan, Gouspillou and Hinton. This is an open-access article distributed under the terms of the Creative Commons Attribution License (CC BY). The use, distribution or reproduction in other forums is permitted, provided the original author(s) and the copyright owner(s) are credited and that the original publication in this journal is cited, in accordance with accepted academic practice. No use, distribution or reproduction is permitted which does not comply with these terms.
*Correspondence: Prasanna Katti, cHJhc2FubmFrYXR0aS5rYXR0aUBuaWguZ292, cHJhc2FubmEua3R0QGdtYWlsLmNvbQ==
Disclaimer: All claims expressed in this article are solely those of the authors and do not necessarily represent those of their affiliated organizations, or those of the publisher, the editors and the reviewers. Any product that may be evaluated in this article or claim that may be made by its manufacturer is not guaranteed or endorsed by the publisher.
Research integrity at Frontiers
Learn more about the work of our research integrity team to safeguard the quality of each article we publish.