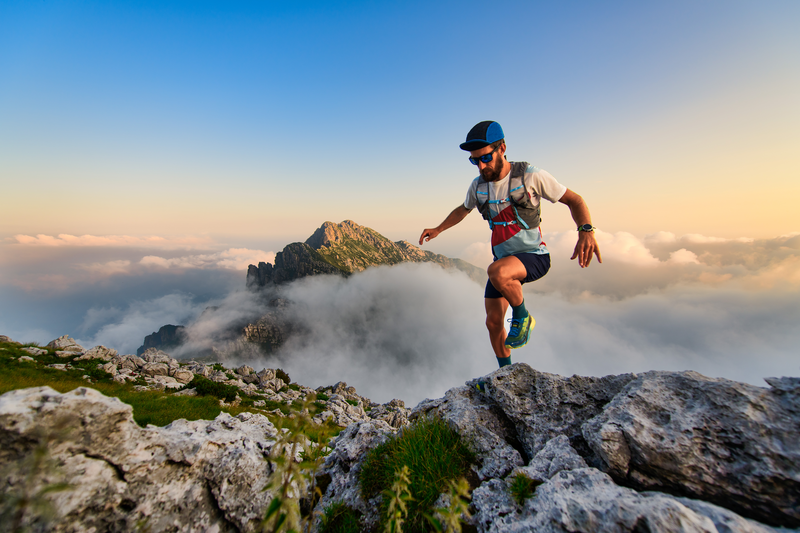
95% of researchers rate our articles as excellent or good
Learn more about the work of our research integrity team to safeguard the quality of each article we publish.
Find out more
PERSPECTIVE article
Front. Physiol. , 18 May 2023
Sec. Gastrointestinal Sciences
Volume 14 - 2023 | https://doi.org/10.3389/fphys.2023.1174655
This article is part of the Research Topic Global Excellence in Cellular Neuropathology: Ukraine View all 20 articles
General anesthesia produces multiple side effects. Notably, it temporarily impairs gastrointestinal motility following surgery and causes the so-called postoperative ileus (POI), a multifactorial and complex condition that develops secondary to neuromuscular failure and mainly affects the small intestine. There are currently limited medication options for POI, reflecting a lack of comprehensive understanding of the mechanisms involved in this complex condition. Notably, although acetylcholine is one of the major neurotransmitters initiating excitation-contraction coupling in the gut, cholinergic stimulation by prokinetic drugs is not very efficient in case of POI. Acetylcholine when released from excitatory motoneurones of the enteric nervous system binds to and activates M2 and M3 types of muscarinic receptors in smooth muscle myocytes. Downstream of these G protein-coupled receptors, muscarinic cation TRPC4 channels act as the major focal point of receptor-mediated signal integration, causing membrane depolarisation accompanied by action potential discharge and calcium influx via L-type Ca2+ channels for myocyte contraction. We have recently found that both inhalation (isoflurane) and intravenous (ketamine) anesthetics significantly inhibit this muscarinic cation current (termed mICAT) in ileal myocytes, even when G proteins are activated directly by intracellular GTPγS, i.e., bypassing muscarinic receptors. Here we aim to summarize Transient Receptor Potential channels and calcium signalling-related aspects of the cholinergic mechanisms in the gut and visceral pain, discuss exactly how these may be negatively impacted by general anaesthetics, while proposing the receptor-operated TRPC4 channel as a novel molecular target for the treatment of POI.
Postoperative ileus (POI) remains one of the most common and costly for the healthcare system complications of surgery, in particular abdominal surgery. POI is a multifactorial problem, whereby administration of general anaesthetics and anxiolytics is certainly one of the multiple risk factors, and is likely especially important during the first neurological phase of POI involving sympathetic and enteric nervous systems (Venara et al., 2016; Wattchow et al., 2021).
General anaesthetics are primarily aimed at targeting specific receptors of the central nervous system (CNS), but these drugs can also affect other molecular off-targets, such as receptors and ion channels outside of the CNS. Understanding how general anaesthetics can negatively affect gut motility would seem to require, in the first place, knowledge of their effects within the enteric nervous system (ENS), as well as on the pacemaker cells of the gastrointestinal (GI) tract - Interstitial Cells of Cajal (ICC cells), which coordinate and regulate the sensory, secretory and motor functions of the gut (Spencer and Hu, 2020). Indeed, one of the pioneer studies in this area has demonstrated that phencyclidine and related drugs including ketamine decreased neurogenic contractions of guinea-pig ileum and shifted the dose-response curve to acetylcholine to higher agonist concentrations. The authors have thus suggested that these drugs interact with both enteric neurones and smooth muscle myocytes (Gintzler et al., 1982). However, there has not been much subsequent progress towards delineating specific molecular and/or cellular pathways involved. On the other hand, among various prokinetic drugs used for POI treatment (Venara et al., 2016), low concentrations of neostigmine, which is an inhibitor of acetylcholinesterase, may be beneficial (results of the recent systematic review and meta-analysis performed by Liao et al., 2021), which calls for a better understanding of the possible dysfunction of the acetylcholine-mediated signal transduction during the impairment of GI motility by general anaesthetics.
Strong evidence has been accumulated over the recent years indicating a major functional role of various subtypes of Transient Receptor Potential (TRP) channels in smooth muscles and sensory neurons (Venkatachalam and Montell, 2007; Tsvilovskyy et al., 2009). Recent studies (Matta et al., 2008; Abeele et al., 2013; Dryn et al., 2018; Wang et al., 2019; Melnyk et al., 2020) have shown that TRP channels could interact with general anesthetics at subclinical doses, which makes them highly likely primary candidates for the development of side effects produced by local and general anaesthetics. Thus, in recent years we focused our research on the problem of how general anaesthetics, such as isoflurane and ketamine, affect acetylcholine-activated TRPC4 channels, which mediate muscarinic cation current in ileal myocytes, termed mICAT (Tsvilovskyy et al., 2009). Since TRPC4 channels are widely expressed in the CNS, ENS, ICC and GI smooth muscle myocytes they are increasingly proposed as promising pharmacological targets (Boesmans et al., 2011), but their role in GI pathophysiology in general, and specifically in the pathogenesis of POI, remains to be better elucidated, prompting us to summarize the current status of this research in this Perspective.
Chronic pain significantly impairs quality of life. As reviewed by Julius (2013), TRP channels play a significant role in pain signalling (Julius, 2013). TRP channels are known as polymodal sensors of various stimuli, including chemical modulators, reactive oxygen species, changes in pH and temperature, and mechanical forces (Wu et al., 2010). Being a vital protective mechanism, pain perception could be a considerable problem under some pathological conditions, such as inflammation, when chronic pain develops. Among multiple other co-morbidities, severe pain also correlates with POI. Recent studies have highlighted the involvement of several members of the TRP superfamily of ion channels in producing pain. The cold receptor TRPM8 is expressed in various sensory neurons and is involved in cold nociception (Knowlton et al., 2013). Notably, these channels perceive noxious cold, innocuous cooling and TRPM8-mediated analgesia differently (Laing and Dhaka, 2016). These channels are implicated in inflammatory and neuropathic cold allodynia and other cold hypersensitivity (Bautista et al., 2007; Colburn et al., 2007; Moran and Szallasi, 2018). A recent study has identified the efficacy of novel TRPM8 antagonists in treating both inflammatory and neuropathic pain (De Caro et al., 2018). Different pharmaceutical companies have been developing novel TRPM8 antagonists as pharmacological treatment for chronic or inflammatory pain, migraine and chemotherapeutic-induced allodynia (Weyer and Lehto, 2017). However, as of yet there are no ongoing clinical trials of these compounds.
The other member of the TRP superfamily which is expressed in neuronal, smooth muscles cells and other non-neuronal cells is TRPV4, a multimodal sensor which underlies regulation of several important physiological functions such as osmotic, mechanical and warm temperature sensation (Everaerts et al., 2010; Moore et al., 2018). Recent studies revealed the involvement of this channel in nociception, in particular in joint- and skin-mediated inflammatory pain, neuropathic pain and visceral pain (Alessandri-Haber et al., 2004; Brierley et al., 2008; Moore et al., 2013; O’Conor et al., 2016; Qu et al., 2016). These data indicate that TRP channels are promising therapeutic targets for chronic pain relief. Better understanding of the underlying molecular mechanisms of the TRP channels’ role in nociception could promote the search for chemical compounds as prospective and novel pharmacological approaches targeting these channels for effective pain relief.
The GI tract has differentiated sensory afferent innervation, with sensory neurones located in the dorsal root ganglia (DRGs), nodose ganglia and the inferior ganglion of the vagus nerve (Brookes et al., 2013; Spencer and Hu, 2020). Visceral pain can be associated with smooth muscle (SM) spasms, in turn causing irritable bowel syndrome (IBS). Abdominal pain is a primary symptom of IBS (Keszthelyi et al., 2012a; 2012b). Several of the TRP channels (most notably TRPA1, TRPC4, TRPV1 and TRPV4) are expressed in the gut, where they play important roles in multiple pathophysiological processes, including visceral nociception and pain (Boesmans et al., 2011; Holzer, 2011). Thus, TRPC4 channels opened secondary to muscarinic acetylcholine receptor activation trigger excitation and contraction of small intestinal smooth muscles (Bolton et al., 1999; Tsvilovskyy et al., 2009). TRPV4 senses local pressure that can become painful when it exceeds certain threshold level (Liedtke, 2005). Moreover, TRPV4 in afferent nerves can be sensitized via protease-activated receptor 2 thus evoking visceral hyperalgesia (Sipe et al., 2008). The molecular pathophysiology of IBS is not completely understood (Shah et al., 2020), but among other ion channels, TRPV4 and TRPA1 have been suggested as important sensory channels in IBS (Yang et al., 2022).
Currently, three main agent classes are used for SM antispasmodic action: antimuscarinic agents (Tobin et al., 2009), calcium channel inhibitors (Evangelista, 2004), and direct smooth muscle relaxants (Subissi et al., 1983), but their efficiency is less than optimal, making these compounds questionable for their clinical use. There is thus an urgent need to develop other therapies for treating chronic abdominal pain, such as TRP channels modulators.
Inhaled anesthetics rapidly equilibrate between air in the alveoli and capillary blood. Small hydrophobic molecules like anesthetics can first of all affect the membrane lipid bilayer, thus nonspecifically altering the functions of multiple, if not all, transmembrane proteins (Tsuchiya and Mizogami, 2013). The second, much better understood group of their molecular targets includes plasma membrane receptors and ion channels. Among the latter, both voltage-gated (Ca2+, Na+ and K+ channels of different types) and ligand-activated (such as nicotinic acetylcholine receptors, serotonin, glycine and GABAA receptors) received considerable attention in this context (Jenkins et al., 1996; Tassonyi et al., 2002; Hara and Sata, 2007; Alberola-Die et al., 2011; Germann et al., 2016; Liu et al., 2019; Mathie et al., 2021). For example, the inhalational anaesthetic isoflurane, which is a halogenated ether, targets GABAA, glutamate and glycine receptors, while ketamine is best characterised as an antagonist of NMDA receptors, which determines its strong analgesic action (Franks, 2006; Franks, 2008).
The TRP channels discussed above represent yet another group of such targets. There is indeed growing evidence in this area of research showing that, for example, halothane, chloroform, and propofol can inhibit TRPC5 channels (Bahnasi et al., 2008), isoflurane can activate TRPA1 channels in sensory DRG neurones (Matta et al., 2008), while propofol affects TRPA1 and TRPV1 channels as became evident from observing propofol-induced vasorelaxation of coronary arterioles (Wang et al., 2015).
Acetylcholine, a major neurotransmitter that plays multiple important roles in the central and peripheral nervous system, activates muscarinic acetylcholine receptors (mainly of M2 and M3 subtypes), which are the main excitatory receptor subtypes expressed in GI smooth muscles (Bolton, 1972; 1979; Zholos, 2006). This, in turn, results in the openings of two receptor-operated cation channels, TRPC4 and TRPC6, of which TRPC4 is of main importance since it mediates about 85% of mICAT (Tsvilovskyy et al., 2009). Signal transduction pathways leading to TRPC4 are complex, since two receptor subtypes, which are differentially coupled to Gi/o and Gq/11 proteins, are involved (M2 and M3 receptors, respectively). These have been previously extensively studied and reviewed (Zholos and Bolton, 1997; Bolton et al., 1999; Zholos et al., 2004; Zholos, 2006; Sakamoto et al., 2007; Tanahashi et al., 2020), as summarised schematically in Figure 1. In brief, these receptors systems act in synergy, whereby the M2/Gi/o is of primary nature, while the M3/Gq/PLC system and the increase in intracellular Ca2+ concentration it produces by InsP3-evoked Ca2+ release play both permissive (at least in part via PIP2 depletion) and potentiating (via [Ca2+]i elevation) roles. We therefore reasoned that inhibition of mICAT generation as the primary mechanism of cholinergic excitation-contraction coupling in the gut (Bolton et al., 1999) can occur at different levels, ranging from muscarinic receptors and the G-proteins that are coupled to them, and to Ca2+ signalling and TRPC4 channels themselves.
FIGURE 1. The schematic illustration of the possible mechanism of anaesthetics side-effects action on the novel molecular targets in intestinal myocytes. Acting in synergy with muscarinic M2 and M3 receptors coupled to Gi/o- and Gq/11-proteins, respectively, TRPC4 channels are the main molecular component of depolarizing inward current mICAT, which is the principal regulator of cholinergic excitation-contraction coupling in intestinal smooth muscles. Anaesthetics (ketamine and isoflurane) strongly suppressed mICAT, acting more likely on G-proteins, but minimally on M2/M3 receptors. TRPC4 channels direct agonist (−)-englerin A recovered ketamine-induced inhibition of mICAT, suggesting that this anaesthetic is targeting G-proteins rather than TRPC4 channels.
We have addressed these possibilities using a range of experimental techniques, from patch-clamp recordings to in vitro contractile recordings, in our recent studies of isoflurane and ketamine effects on mICAT and spontaneous as well as carbachol-stimulated contractions of ileal smooth muscles (Dryn et al., 2018; Melnyk et al., 2020). To bypass the receptor activation step, GTPγS infusion via patch-pipette was employed for direct activation of all trimeric G-proteins. Intriguingly, both isoflurane and ketamine strongly inhibited both carbachol- and GTPγS-induced mICAT at clinically relevant concentrations, and the inhibitory effects had much in common. Thus, muscarinic receptors are not the major targets of their action, and hence any strategy aimed at the upregulation of mACh receptor activity, such as inhibitors of acetylcholinesterase, would be, in theory, not very efficient. At the same time the effect of ketamine was effectively opposed by the direct TRPC4 agonist (−)-englerin A (EA) indicating that the function of the channel itself was preserved (Melnyk et al., 2020) (Figure 1). We thus concluded that TRPC4 agonists may be used for the correction of GI motility suppression induced by general anesthesia. It is worth noting that there has recently been significant progress in developing nontoxic analogue of EA (Seenadera et al., 2022).
Currently, the most effective and widely used treatments for post-traumatic stress disorder (PTSD) are antidepressants and anxiolytics, but other novel treatments are being considered, especially for the treatment of more refractory and disabling cases of PTSD (Ipser and Stein, 2012; Liriano et al., 2019). Thus, ketamine is not only a widely used anesthetic, but has recently been considered as a potential antidepressant (Duman et al., 2012; Zanos and Gould, 2018; Jeong et al., 2022), in particular as a promising and novel pharmacotherapeutic agent for PTSD patients, especially in more complex cases (Liriano et al., 2019).
Recent studies have shown that some novel modulators of TRP channels possess antidepressant action. A recently identified inhibitor of TRPC4/C5 channels, M084, has demonstrated antidepressant and anxiolytic effects (Yang et al., 2015). HC-070, a new small molecule antagonist of these channels, also possess antidepressant effects and may be proposed as a treatment for a number of symptoms of psychiatric disorders (Just et al., 2018). Antidepressants, in turn, affect TRP channels, and not only in the nervous system, but also in other systems and organs, where they can produce side effects. It was shown that tricyclic antidepressants, which are also used for treating IBS, inhibited TRPC4 channels in colonic myocytes, resulting in suppression of GI motility (Jeong et al., 2022). These results well correlate with our recent studies of the inhibitory action of ketamine and isoflurane on the TRPC4 channel-mediated mICAT in mouse small intestinal myocytes (Dryn et al., 2018; Melnyk et al., 2020). Thus, antidepressants and anxiolytics can modulate the TRP channels function, which in turn could lead to intestinal complications.
Currently, continuation of the studies of the side effects of anaesthetics and antidepressants on TRP channels, in particular in intestinal myocytes, remains an important task. War and military actions are the most significant factors of PTSD development, and also of the increase in the number and complexity of surgical interventions. According to the latest medical reports from the Ministry of Health of Ukraine, as a result of the Russian invasion of Ukraine in 2022 and the ongoing war, the risk of PTSD occurrence can be very high and will amount from 4.5 to 15 million people, including both military personnel and civilians (Bryant et al., 2022; Cai et al., 2022; Chaaya et al., 2022; Zaliska et al., 2022). The results of such studies as outlined in this Perspective can propose some recommendations for optimizing protocols for the use and dosage of certain types of anaesthetics and antidepressants in medical practice, in particular in the treatment of PTSD. Thus, our own future studies will be aimed at revealing ion channel mechanisms of side effects of other widely used anaesthetics, as well as anxiolytics and antidepressants, and their combinations. Moreover, other members of the TRP family, in particular TRPV4 and TRPM8 channels, which are important for the regulation of blood vessel tone, need to be more fully characterized in this context (Melanaphy et al., 2016; Dryn et al., 2018). There is accumulating evidence that some anaesthetics can affect these two types of channels (Abeele et al., 2013; Wang et al., 2019), as well as vascular tone (Liu et al., 2009; Gille et al., 2012; Sakai et al., 2014), therefore this research area is also relevant and promising.
The original contributions presented in the study are included in the article/Supplementary Material, further inquiries can be directed to the corresponding author.
The animal study was reviewed and approved. This Perspective refers to some results in our two papers published previously. These are referenced, and the original papers include relevant Ethics information (Dryn et al., 2018; Melnyk et al., 2020).
AZ supervised the project; AZ, DD, and MM designed the studies outlined in this paper; DD, MM drafted the manuscript; AZ, DD, and MM finalized and approved the final version of the manuscript. All authors listed have made a substantial, direct, and intellectual contribution to the work and approved it for publication.
Supported by the Ministry of Education and Science of Ukraine (Grant No. 0122U001535 to AZ and MM) and National Academy of Sciences of Ukraine (Grant No. 0122U002126 to DD and MM).
We are grateful to Professor David J. Beech for a gift of (−)-englerin A and to Dmytro O. Dziuba for his consultations and discussion of the clinical significance/implications of our research findings.
The authors declare that the research was conducted in the absence of any commercial or financial relationships that could be construed as a potential conflict of interest.
All claims expressed in this article are solely those of the authors and do not necessarily represent those of their affiliated organizations, or those of the publisher, the editors and the reviewers. Any product that may be evaluated in this article, or claim that may be made by its manufacturer, is not guaranteed or endorsed by the publisher.
CNS, central nervous system; DRG, dorsal root ganglia; EA, (−)-englerin A; ENS, enteric nervous system; GI, gastrointestinal; IBS, irritable bowel syndrome; mAChR, muscarinic acetylcholine receptor; mICAT, muscarinic receptor cation current; POI, postoperative ileus; PTSD, post-traumatic stress disorder; SM, smooth muscle; TRP, Transient Receptor Potential.
Abeele, F. V., Kondratskyi, A., Dubois, C., Shapovlov, G., Gkika, D., Busserolles, J., et al. (2013). Complex modulation of the cold receptor TRPM8 by volatile anaesthetics and its role in complications of general anaesthesia. J. Cell Sci. 126, 4479–4489. doi:10.1242/JCS.131631
Alberola-Die, A., Martinez-Pinna, J., González-Ros, J. M., Ivorra, I., and Morales, A. (2011). Multiple inhibitory actions of lidocaine on Torpedo nicotinic acetylcholine receptors transplanted to Xenopus oocytes. J. Neurochem. 117, 1009–1019. doi:10.1111/J.1471-4159.2011.07271.X
Alessandri-Haber, N., Dina, O. A., Yeh, J. J., Parada, C. A., Reichling, D. B., and Levine, J. D. (2004). Transient receptor potential vanilloid 4 is essential in chemotherapy-induced neuropathic pain in the rat. J. Neurosci. 24, 4444–4452. doi:10.1523/JNEUROSCI.0242-04.2004
Bahnasi, Y. M., Wright, H. M., Milligan, C. J., Dedman, A. M., Zeng, F., Hopkins, P. M., et al. (2008). Modulation of TRPC5 cation channels by halothane, chloroform and propofol. Br. J. Pharmacol. 153, 1505–1512. doi:10.1038/SJ.BJP.0707689
Bautista, D. M., Siemens, J., Glazer, J. M., Tsuruda, P. R., Basbaum, A. I., Stucky, C. L., et al. (2007). The menthol receptor TRPM8 is the principal detector of environmental cold. Nature 448, 204–208. doi:10.1038/NATURE05910
Boesmans, W., Owsianik, G., Tack, J., Voets, T., and Vanden Berghe, P. (2011). TRP channels in neurogastroenterology: Opportunities for therapeutic intervention. Br. J. Pharmacol. 162, 18–37. doi:10.1111/j.1476-5381.2010.01009.x
Bolton, T. B. (1979). Mechanisms of action of transmitters and other substances on smooth muscle. Physiol. Rev. 59, 606–718. doi:10.1152/PHYSREV.1979.59.3.606
Bolton, T. B., Prestwich, S. A., Zholos, A. V., and Gordienko, D. V. (1999). Excitation-contraction coupling in gastrointestinal and other smooth muscles. Annu. Rev. Physiol. 61, 85–115. doi:10.1146/annurev.physiol.61.1.85
Bolton, T. B. (1972). The depolarizing action of acetylcholine or carbachol in intestinal smooth muscle. J. Physiol. 220, 647–671. doi:10.1113/JPHYSIOL.1972.SP009728
Brierley, S. M., Page, A. J., Hughes, P. A., Adam, B., Liebregts, T., Cooper, N. J., et al. (2008). Selective role for TRPV4 ion channels in visceral sensory pathways. Gastroenterol 134, 2059–2069. doi:10.1053/J.GASTRO.2008.01.074
Brookes, S. J. H., Spencer, N. J., Costa, M., and Zagorodnyuk, V. P. (2013). Extrinsic primary afferent signalling in the gut. Nat. Rev. Gastroenterol. Hepatol. 10, 286–296. doi:10.1038/NRGASTRO.2013.29
Bryant, R. A., Schnurr, P. P., and Pedlar, D.5-Eyes Mental Health Research and Innovation Collaboration in military and veteran mental health (2022). Addressing the mental health needs of civilian combatants in Ukraine. Lancet. Psychiatry 9, 346–347. doi:10.1016/S2215-0366(22)00097-9
Cai, H., Bai, W., Zheng, Y., Zhang, L., Cheung, T., Su, Z., et al. (2022). International collaboration for addressing mental health crisis among child and adolescent refugees during the Russia-Ukraine war. Asian J. Psychiatr. 72, 103109. doi:10.1016/J.AJP.2022.103109
Chaaya, C., Thambi, V. D., Sabuncu, Ö., Abedi, R., Osman, A. O. A., Uwishema, O., et al. (2022). Ukraine – Russia crisis and its impacts on the mental health of Ukrainian young people during the COVID-19 pandemic. Ann. Med. Surg. 79, 104033. doi:10.1016/J.AMSU.2022.104033
Colburn, R. W., Lubin, M. L., Stone, D. J., Wang, Y., Lawrence, D., D’Andrea, M. R. R., et al. (2007). Attenuated cold sensitivity in TRPM8 null mice. Neuron 54, 379–386. doi:10.1016/J.NEURON.2007.04.017
De Caro, C., Russo, R., Avagliano, C., Cristiano, C., Calignano, A., Aramini, A., et al. (2018). Antinociceptive effect of two novel transient receptor potential melastatin 8 antagonists in acute and chronic pain models in rat. Br. J. Pharmacol. 175, 1691–1706. doi:10.1111/BPH.14177
Dryn, D., Luo, J., Melnyk, M., Zholos, A., and Hu, H. (2018). Inhalation anaesthetic isoflurane inhibits the muscarinic cation current and carbachol-induced gastrointestinal smooth muscle contractions. Eur. J. Pharmacol. 820, 39–44. doi:10.1016/j.ejphar.2017.11.044
Duman, R. S., Li, N., Liu, R. J., Duric, V., and Aghajanian, G. (2012). Signaling pathways underlying the rapid antidepressant actions of ketamine. Neuropharmacol 62, 35–41. doi:10.1016/J.NEUROPHARM.2011.08.044
Evangelista, S. (2004). Quaternary ammonium derivatives as spasmolytics for irritable bowel syndrome. Curr. Pharm. Des. 10, 3561–3568. doi:10.2174/1381612043382972
Everaerts, W., Nilius, B., and Owsianik, G. (2010). The vanilloid transient receptor potential channel TRPV4: From structure to disease. Prog. Biophys. Mol. Biol. 103, 2–17. doi:10.1016/J.PBIOMOLBIO.2009.10.002
Franks, N. P. (2008). General anaesthesia: From molecular targets to neuronal pathways of sleep and arousal. Nat. Rev. Neurosci. 9, 370–386. doi:10.1038/NRN2372
Franks, N. P. (2006). Molecular targets underlying general anaesthesia. Br. J. Pharmacol. 147 (1), S72–S81. doi:10.1038/SJ.BJP.0706441
Germann, A. L., Shin, D. J., Manion, B. D., Edge, C. J., Smith, E. H., Franks, N. P., et al. (2016). Activation and modulation of recombinant glycine and GABA A receptors by 4-halogenated analogues of propofol. Br. J. Pharmacol. 173, 3110–3120. doi:10.1111/BPH.13566
Gille, J., Seyfarth, H. J., Gerlach, S., Malcharek, M., Czeslick, E., and Sablotzki, A. (2012). Perioperative anesthesiological management of patients with pulmonary hypertension. Anesthesiol. Res. Pract. 2012, 356982. doi:10.1155/2012/356982
Gintzler, A. R., Zukin, R. S., and Zukin, S. R. (1982). Effects of phencyclidine and its derivatives on enteric neurones. Br. J. Pharmacol. 75, 261–267. doi:10.1111/j.1476-5381.1982.tb08782.x
Hara, K., and Sata, T. (2007). The effects of the local anesthetics lidocaine and procaine on glycine and gamma-aminobutyric acid receptors expressed in Xenopus oocytes. Anesth. Analg. 104, 1434–1439. doi:10.1213/01.ANE.0000261509.72234.A6
Holzer, P. (2011). TRP channels in the digestive system. Curr. Pharm. Biotechnol. 12, 24–34. doi:10.2174/138920111793937862
Ipser, J. C., and Stein, D. J. (2012). Evidence-based pharmacotherapy of post-traumatic stress disorder (PTSD). Int. J. Neuropsychopharmacol. 15, 825–840. doi:10.1017/S1461145711001209
Jenkins, A., Franks, N. P., and Lieb, W. R. (1996). Actions of general anaesthetics on 5-HT3 receptors in N1E-115 neuroblastoma cells. Br. J. Pharmacol. 117, 1507–1515. doi:10.1111/J.1476-5381.1996.TB15314.X
Jeong, B., Sung, T. S., Jeon, D., Park, K. J., Jun, J. Y., So, I., et al. (2022). Inhibition of TRPC4 channel activity in colonic myocytes by tricyclic antidepressants disrupts colonic motility causing constipation. J. Cell. Mol. Med. 26 (19), 4911–4923. doi:10.1111/JCMM.17348
Julius, D. (2013). TRP channels and pain. Annu. Rev. Cell Dev. Biol. 29, 355–384. doi:10.1146/ANNUREV-CELLBIO-101011-155833
Just, S., Chenard, B. L., Ceci, A., Strassmaier, T., Chong, J. A., Blair, N. T., et al. (2018). Treatment with HC-070, a potent inhibitor of TRPC4 and TRPC5, leads to anxiolytic and antidepressant effects in mice. PLoS One 13 (1), e0191225. doi:10.1371/JOURNAL.PONE.0191225
Keszthelyi, D., Troost, F. J., and Masclee, A. A. (2012a). Irritable bowel syndrome: Methods, mechanisms, and pathophysiology. Methods to assess visceral hypersensitivity in irritable bowel syndrome. Am. J. Physiol. Gastrointest. Liver Physiol. 303 (2), G141–G154. doi:10.1152/AJPGI.00060.2012
Keszthelyi, D., Troost, F. J., Simrén, M., Ludidi, S., Kruimel, J. W., Conchillo, J. M., et al. (2012b). Revisiting concepts of visceral nociception in irritable bowel syndrome. Eur. J. Pain 16, 1444–1454. doi:10.1002/J.1532-2149.2012.00147.X
Knowlton, W. M., Palkar, R., Lippoldt, E. K., McCoy, D. D., Baluch, F., Chen, J., et al. (2013). A sensory-labeled line for cold: TRPM8-expressing sensory neurons define the cellular basis for cold, cold pain, and cooling-mediated analgesia. J. Neurosci. 33, 2837–2848. doi:10.1523/JNEUROSCI.1943-12.2013
Laing, R. J., and Dhaka, A. (2016). ThermoTRPs and pain. Neuroscientist 22, 171–187. doi:10.1177/1073858414567884
Liao, Y., Li, Y., and Ouyang, W. (2021). Effects and safety of neostigmine for postoperative recovery of gastrointestinal function: A systematic review and meta-analysis. Ann. Palliat. Med. 10, 12507–12518. doi:10.21037/apm-21-3291
Liedtke, W. (2005). TRPV4 as osmosensor: A transgenic approach. Pflugers Arch. 451, 176–180. doi:10.1007/S00424-005-1449-8
Liriano, F., Hatten, C., and Schwartz, T. L. (2019). Ketamine as treatment for post-traumatic stress disorder: A review. Drugs Context 8, 212305. doi:10.7573/DIC.212305
Liu, Q. Z., Hao, M., Zhou, Z. Y., Ge, J. L., Wu, Y. C., Zhao, L. L., et al. (2019). Propofol reduces synaptic strength by inhibiting sodium and calcium channels at nerve terminals. Protein Cell 10, 688–693. doi:10.1007/S13238-019-0624-1
Liu, Y., Chang, H., Niu, L., Xue, W., Zhang, X., Liang, Y., et al. (2009). Effects of propofol on responses of rat isolated renal arteriole to vasoactive agents. Vasc. Pharmacol. 51, 182–189. doi:10.1016/J.VPH.2009.06.003
Mathie, A., Veale, E. L., Cunningham, K. P., Holden, R. G., and Wright, P. D. (2021). Two-pore domain potassium channels as drug targets: Anesthesia and beyond. Annu. Rev. Pharmacol. Toxicol. 61, 401–420. doi:10.1146/ANNUREV-PHARMTOX-030920-111536
Matta, J. A., Cornett, P. M., Miyares, R. L., Abe, K., Sahibzada, N., and Ahern, G. P. (2008). General anesthetics activate a nociceptive ion channel to enhance pain and inflammation. Proc. Natl. Acad. Sci. U. S. A. 105, 8784–8789. doi:10.1073/PNAS.0711038105
Melanaphy, D., Johnson, C. D., Kustov, M. V., Watson, C. A., Borysova, L., Burdyga, T. V., et al. (2016). Ion channel mechanisms of rat tail artery contraction-relaxation by menthol involving, respectively, TRPM8 activation and L-type Ca2+ channel inhibition. Am. J. Physiol. Heart Circ. Physiol. 311, H1416–H1430. doi:10.1152/AJPHEART.00222.2015
Melnyk, M. I., Dryn, D. O., Al Kury, L. T., Dziuba, D. O., and Zholos, A. V. (2020). Suppression of mICAT in mouse small intestinal myocytes by general anaesthetic ketamine and its recovery by TRPC4 agonist (-)-englerin A. Front. Pharmacol. 11, 594882. doi:10.3389/fphar.2020.594882
Moore, C., Cevikbas, F., Pasolli, H. A., Chen, Y., Kong, W., Kempkes, C., et al. (2013). UVB radiation generates sunburn pain and affects skin by activating epidermal TRPV4 ion channels and triggering endothelin-1 signaling. Proc. Natl. Acad. Sci. U. S. A. 110 (34), E3225–E3234. doi:10.1073/PNAS.1312933110
Moore, C., Gupta, R., Jordt, S. E., Chen, Y., and Liedtke, W. B. (2018). Regulation of pain and itch by TRP channels. Neurosci. Bull. 34, 120–142. doi:10.1007/S12264-017-0200-8
Moran, M. M., and Szallasi, A. (2018). Targeting nociceptive transient receptor potential channels to treat chronic pain: Current state of the field. Br. J. Pharmacol. 175, 2185–2203. doi:10.1111/BPH.14044
O’Conor, C. J., Ramalingam, S., Zelenski, N. A., Benefield, H. C., Rigo, I., Little, D., et al. (2016). Cartilage-specific knockout of the mechanosensory ion channel TRPV4 decreases age-related osteoarthritis. Sci. Rep. 6, 29053. doi:10.1038/SREP29053
Qu, Y. J., Zhang, X., Fan, Z. Z., Huai, J., Teng, Y. B., Zhang, Y., et al. (2016). Effect of TRPV4-p38 MAPK pathway on neuropathic pain in rats with chronic compression of the dorsal root ganglion. Biomed. Res. Int. 2016, 6978923. doi:10.1155/2016/6978923
Sakai, Y., Kawahito, S., Takaishi, K., Mita, N., Kinoshita, H., Hatakeyama, N., et al. (2014). Propofol-induced relaxation of rat aorta is altered by aging. J. Med. Invest. 61, 278–284. doi:10.2152/JMI.61.278
Sakamoto, T., Unno, T., Kitazawa, T., Taneike, T., Yamada, M., Wess, J., et al. (2007). Three distinct muscarinic signalling pathways for cationic channel activation in mouse gut smooth muscle cells. J. Physiol. 582, 41–61. doi:10.1113/jphysiol.2007.133165
Seenadera, S. P. D., Long, S. A., Akee, R., Bermudez, G., Parsonage, G., Strope, J., et al. (2022). Biological effects of modifications of the englerin A glycolate. ACS Med. Chem. Lett. 13, 1472–1476. doi:10.1021/ACSMEDCHEMLETT.2C00258
Shah, A., Talley, N. J., Jones, M., Kendall, B. J., Koloski, N., Walker, M. M., et al. (2020). Small intestinal bacterial overgrowth in irritable bowel syndrome: A systematic review and meta-analysis of case-control studies. Am. J. Gastroenterol. 115, 190–201. doi:10.14309/AJG.0000000000000504
Sipe, W. E. B., Brierley, S. M., Martin, C. M., Phillis, B. D., Cruz, F. B., Grady, E. F., et al. (2008). Transient receptor potential vanilloid 4 mediates protease activated receptor 2-induced sensitization of colonic afferent nerves and visceral hyperalgesia. Am. J. Physiol. Gastrointest. Liver Physiol. 294 (5), G1288–G1298. doi:10.1152/AJPGI.00002.2008
Spencer, N. J., and Hu, H. (2020). Enteric nervous system: Sensory transduction, neural circuits and gastrointestinal motility. Nat. Rev. Gastroenterol. Hepatol. 17, 338–351. doi:10.1038/s41575-020-0271-2
Subissi, A., Brunori, P., and Bachi, M. (1983). Effects of spasmolytics on K+-induced contraction of rat intestine in vivo. Eur. J. Pharmacol. 96, 295–301. doi:10.1016/0014-2999(83)90320-5
Tanahashi, Y., Katsurada, T., Inasaki, N., Uchiyama, M., Sakamoto, T., Yamamoto, M., et al. (2020). Further characterization of the synergistic activation mechanism of cationic channels by M 2 and M 3 muscarinic receptors in mouse intestinal smooth muscle cells. Am. J. Physiol. Cell Physiol. 318, C514–C523. doi:10.1152/AJPCELL.00277.2019
Tassonyi, E., Charpantier, E., Muller, D., Dumont, L., and Bertrand, D. (2002). The role of nicotinic acetylcholine receptors in the mechanisms of anesthesia. Brain Res. Bull. 57, 133–150. doi:10.1016/S0361-9230(01)00740-7
Tobin, G., Giglio, D., and Lundgren, O. (2009). Muscarinic receptor subtypes in the alimentary tract. J. Physiol. Pharmacol. 60 (3–21), 3–21. PMID: 19439804.
Tsuchiya, H., and Mizogami, M. (2013). Interaction of local anesthetics with biomembranes consisting of phospholipids and cholesterol: Mechanistic and clinical implications for anesthetic and cardiotoxic effects. Anesthesiol. Res. Pract. 2013, 297141. doi:10.1155/2013/297141
Tsvilovskyy, V. V., Zholos, A. V., Aberle, T., Philipp, S. E., Dietrich, A., Zhu, M. X., et al. (2009). Deletion of TRPC4 and TRPC6 in mice impairs smooth muscle contraction and intestinal motility in vivo. Gastroenterol 137, 1415–1424. doi:10.1053/j.gastro.2009.06.046
Venara, A., Neunlist, M., Slim, K., Barbieux, J., Colas, P. A., Hamy, A., et al. (2016). Postoperative ileus: Pathophysiology, incidence, and prevention. J. Visc. Surg. 153, 439–446. doi:10.1016/J.JVISCSURG.2016.08.010
Venkatachalam, K., and Montell, C. (2007). TRP channels. Annu. Rev. Biochem. 76, 387–417. doi:10.1146/ANNUREV.BIOCHEM.75.103004.142819
Wang, B., Wu, Q., Liao, J., Zhang, S., Liu, H., Yang, C., et al. (2019). Propofol induces cardioprotection against ischemia-reperfusion injury via suppression of Transient Receptor Potential Vanilloid 4 channel. Front. Pharmacol. 10, 1150. doi:10.3389/FPHAR.2019.01150
Wang, Y., Zhou, H., Wu, B., Zhou, Q., Cui, D., and Wang, L. (2015). Protein kinase C isoforms distinctly regulate propofol-induced endothelium-dependent and endothelium-independent vasodilation. J. Cardiovasc. Pharmacol. 66, 276–284. doi:10.1097/FJC.0000000000000275
Wattchow, D., Heitmann, P., Smolilo, D., Spencer, N. J., Parker, D., Hibberd, T., et al. (2021). Postoperative ileus—an ongoing conundrum. Neurogastroenterol. Motil. 33, e14046. doi:10.1111/nmo.14046
Weyer, A. D., and Lehto, S. G. (2017). Development of TRPM8 antagonists to treat chronic pain and migraine. Pharm. (Basel) 10 (2), 37. doi:10.3390/PH10020037
Wu, L. J., Sweet, T. B., and Clapham, D. E. (2010). International Union of Basic and Clinical Pharmacology. LXXVI. Current progress in the mammalian TRP ion channel family. Pharmacol. Rev. 62, 381–404. doi:10.1124/PR.110.002725
Yang, H., Hou, C., Xiao, W., and Qiu, Y. (2022). The role of mechanosensitive ion channels in the gastrointestinal tract. Front. Physiol. 13, 904203. doi:10.3389/fphys.2022.904203
Yang, L. P., Jiang, F. J., Wu, G. S., Deng, K., Wen, M., Zhou, X., et al. (2015). Acute treatment with a novel TRPC4/C5 channel inhibitor produces antidepressant and anxiolytic-like effects in mice. PLoS One 10 (8), e0136255. doi:10.1371/journal.pone.0136255
Zaliska, O., Oleshchuk, O., Forman, R., and Mossialos, E. (2022). Health impacts of the Russian invasion in Ukraine: Need for global health action. Lancet 399, 1450–1452. doi:10.1016/S0140-6736(22)00615-8
Zanos, P., and Gould, T. D. (2018). Mechanisms of ketamine action as an antidepressant. Mol. Psychiatry 23, 801–811. doi:10.1038/MP.2017.255
Zholos, A. V., and Bolton, T. B. (1997). Muscarinic receptor subtypes controlling the cationic current in Guinea-pig ileal smooth muscle. Br. J. Pharmacol. 122, 885–893. doi:10.1038/SJ.BJP.0701438
Zholos, A. V. (2006). Regulation of TRP-like muscarinic cation current in gastrointestinal smooth muscle with special reference to PLC/InsP3/Ca2+ system. Acta Pharmacol. Sin. 27, 833–842. doi:10.1111/J.1745-7254.2006.00392.X
Zholos, A. V., Tsytsyura, Y. D., Gordienko, D. V., Tsvilovskyy, V. V., and Bolton, T. B. (2004). Phospholipase C, but not InsP3 or DAG, -dependent activation of the muscarinic receptor-operated cation current in Guinea-pig ileal smooth muscle cells. Br. J. Pharmacol. 141, 23–36. doi:10.1038/SJ.BJP.0705584
Keywords: smooth muscles, DRG neurons, visceral pain/visceral nociception/visceral hypersensitivity, TRP channels, G proteins, neurotransmission, intracellular calcium, patch-clamp
Citation: Zholos AV, Dryn DO and Melnyk MI (2023) General anaesthesia-related complications of gut motility with a focus on cholinergic mechanisms, TRP channels and visceral pain. Front. Physiol. 14:1174655. doi: 10.3389/fphys.2023.1174655
Received: 28 February 2023; Accepted: 09 May 2023;
Published: 18 May 2023.
Edited by:
Dirk M. Hermann, University of Duisburg-Essen, GermanyReviewed by:
Jiusheng Yan, University of Texas MD Anderson Cancer Center, United StatesCopyright © 2023 Zholos, Dryn and Melnyk. This is an open-access article distributed under the terms of the Creative Commons Attribution License (CC BY). The use, distribution or reproduction in other forums is permitted, provided the original author(s) and the copyright owner(s) are credited and that the original publication in this journal is cited, in accordance with accepted academic practice. No use, distribution or reproduction is permitted which does not comply with these terms.
*Correspondence: Alexander V. Zholos, YXZ6aG9sb3NAa251LnVh
†These authors have contributed equally to this work
Disclaimer: All claims expressed in this article are solely those of the authors and do not necessarily represent those of their affiliated organizations, or those of the publisher, the editors and the reviewers. Any product that may be evaluated in this article or claim that may be made by its manufacturer is not guaranteed or endorsed by the publisher.
Research integrity at Frontiers
Learn more about the work of our research integrity team to safeguard the quality of each article we publish.