- 1Division of Genetics, ICAR-Indian Agricultural Research Institute (IARI), Pusa Campus, New Delhi, India
- 2School of Biological Sciences, Universiti Sains Malaysia (USM), Georgetown, Penang, Malaysia
- 3Department of Biosciences, Jamia Millia Islamia, New Delhi, Delhi, India
- 4Department of Botany, Deshbandhu College, University of Delhi, New Delhi, India
- 5Department of Botany, Maitreyi College, University of Delhi, New Delhi, India
- 6Department of Botany, Hansraj College, University of Delhi, New Delhi, India
- 7Faculty of Agricultural Sciences, Institute of Applied Sciences and Humanities, GLA University, Mathura, Uttar Pradesh, India
- 8ICAR- National Institute for Biotechnology, New Delhi, India
- 9Department of Botany, Institute of Science, Banaras Hindu University (BHU), Varanasi, Uttar Pradesh, India
- 10School of Biotechnology, Institute of Science, Banaras Hindu University (BHU), Varanasi, Uttar Pradesh, India
- 11Division of Genetics, Indian Council of Agricultural Research (ICAR)-Indian Agricultural Research Institute (IARI), Regional Research Centre, Dharwad, Karnataka, India
- 12Department of Botany, Sri Venkateswara College, University of Delhi, New Delhi, India
- 13Chemical Centre Biology (CCB), Universiti Sains Malaysia (USM), Bayan Lepas, Penang, Malaysia
- 14Institute of Nano Optoelectronics Research and Technology, Universiti Sains Malaysia (USM), Bayan Lepas, Penang, Malaysia
- 15Department of Pharmaceutical Chemistry, Faculty of Pharmacy, Future University in Egypt, Cairo, Egypt
Cardiovascular diseases (CVDs) are one of the major reasons for deaths globally. The renin–angiotensin–aldosterone system (RAAS) regulates body hypertension and fluid balance which causes CVD. Angiotensin-converting enzyme I (ACE I) is the central Zn-metallopeptidase component of the RAAS playing a crucial role in maintaining homeostasis of the cardiovascular system. The available drugs to treat CVD have many side effects, and thus, there is a need to explore phytocompounds and peptides to be utilized as alternative therapies. Soybean is a unique legume cum oilseed crop with an enriched source of proteins. Soybean extracts serve as a primary ingredient in many drug formulations against diabetes, obesity, and spinal cord-related disorders. Soy proteins and their products act against ACE I which may provide a new scope for the identification of potential scaffolds that can help in the design of safer and natural cardiovascular therapies. In this study, the molecular basis for selective inhibition of 34 soy phytomolecules (especially of beta-sitosterol, soyasaponin I, soyasaponin II, soyasaponin II methyl ester, dehydrosoyasaponin I, and phytic acid) was evaluated using in silico molecular docking approaches and dynamic simulations. Our results indicate that amongst the compounds, beta-sitosterol exhibited a potential inhibitory action against ACE I.
1 Introduction
The angiotensin-converting enzyme (ACE) (EC 3.4.15.1) is a chloride-dependent and zinc-containing peptidyl-dipeptidase A enzyme (Natesh et al., 2003; Sharma et al., 2016). It is a crucial enzyme that regulates the formation of angiotensin I (Ang I) to angiotensin II (Ang II) and, in turn, blood pressure (BP). This enzyme is a part of the renin–angiotensin–aldosterone system (RAAS). ACE inhibitors have a therapeutic role in regulating the level of blood pressure and, thus, preventing cardiovascular diseases (CVDs) (Zhao et al., 2019; Tahir et al., 2020; Kircheva et al., 2021; Li et al., 2022). CVD is one of the major diseases and a leading cause of mass mortality estimating around 17.9 million deaths each year (Roth et al., 2020). Hypertension is one of the primary reasons for CVD affecting the vital organs including the brain and kidneys. Several other pathophysiological processes also occur simultaneously along with hypertension which include the stiffening of large ducts (aorta and carotid artery) and elastic artery, smooth muscle cell proliferation, vasoconstriction, and dysfunction of the endothelium (Agrawal et al., 2016; Margalef et al., 2017). The RAAS helps in the regulation of fluid balance and plays a crucial part in maintaining homeostasis of the cardiovascular system and normalizing BP. The inhibitors of ACE competitively inhibit its conversion to Ang II which is formed by the ACE I from Ang I. Thus, formed Ang II then stimulates the release of aldosterone, which eventually elevates BP (Atlas, 2007; Shimakage et al., 2012; Kircheva et al., 2021) and also simultaneously catalyzes the degradation of bradykinin, a potent vasodilator (Margalef et al., 2017). The process of controlling BP is a complex mechanism involving a cascade of steps and different organs, involving the autonomic nervous system (ANS), vasopressor and vasodepressor hormones, the total volume of body fluid, renal function, and vasculature. The endothelium is directly involved in controlling BP by producing multiple vasodilators and vasoconstrictors such as nitric oxide (NO), which is the most important endothelial vasodilator factor (Margalef et al., 2017; Khan and Kumar, 2019; Chamata et al., 2020).
The commercially available synthetic ACE inhibitors (enalapril, lisinopril, etc.) cause side effects like nausea, hyperkalemia, headache, swelling of the lower portion of the skin, cough, disturbances in taste, and angioneurotic edema (Sharma et al., 2016). Various plants have been reported to have potential ACE inhibition properties as reviewed by Patten et al. (2016). The plant-based bioactive compounds are better alternatives to synthetic drugs because they are non-toxic and easily available and have no side effects (Patten et al., 2016; Khan and Kumar, 2019; Chamata et al., 2020; Zhang et al., 2022). Therefore, there is a need to switch and find alternative natural sources (like medicinal crops) having promising health-promoting benefits with no side effects (Hermida et al., 2011; Sitanggang et al., 2021; Xu et al., 2021; Zhang et al., 2022). One such medicinal crop is soybean.
Soybean [Glycine max (L.) Merr.] is a multifaceted nutritional and golden legume crop containing proteins, minerals, and other constituents (Rajendran & Lal, 2020; Ramlal et al., 2022a; Kumar et al., 2022; Mandal et al., 2022; Rajendran et al., 2022). Soybean has been widely associated with reducing BP and obesity. It shows an anti-cholesterol activity by lowering both genic and non-genic origin-based hypercholesterolemic and triglycerides, thus reducing the risk of CVD and simultaneously reducing postmenopausal symptoms and the risk of osteoporosis and antimutagenic effects (Chen et al., 2012; Handa et al., 2020). Soy proteins help in the regulation of hypercholesterolemia and improve lipid metabolism (Caponio et al., 2020). It also possesses hypotensive activities like the inhibition of ACE I and anti-microbial and anti-thrombotic activities (Arnoldi, 2013). Soybean acts as an ideal source for the identification of bioactive peptides against hypertension with other effects (Li et al., 2022). Soy proteins such as glycinin along with other products, namely, tofu, soy protein isolates, soy hydrolysate, and fermented products of soybean (douche and tofu), all have shown the presence of an inhibitory activity against the ACE (Handa et al., 2020; Xu et al., 2021; Ramlal et al., 2022c). Recently, soybean isoflavonoids, especially genistein, were shown to be used against ACE (Ramlal et al., 2022b). Inhibitory peptides involved in the inhibition of ACE are summarized by Ramlal et al. (2022c).
To explore and identify the potential inhibitory compounds, screening methods are most useful, such as molecular docking. Here, in this article, with the use of molecular docking and dynamic simulations, soybean compounds that could potentially be involved in the inhibition of ACE are being reported and that eventually would be helpful in the identification and development of novel functional food additives and useful in the design of safer drugs for ACE inhibition.
2 Materials and methods
2.1 Structure retrieval
Protein Data Bank (PDB) coordinates of the C domain (cACE; PDB ID: 1O8A) and the N domain (nACE; PDB ID: 4BZS) of ACE were retrieved from the PDB (https://www.rcsb.org/). Soybean compounds (Supplementary Figure S1) were retrieved from the PubChem (https://pubchem.ncbi.nlm.nih.gov/) database, namely, beta-sitosterol (BS; ID: 222284), soyasaponin I (SSI; ID: 122097), soyasaponin II (SSII; ID: 443614), soyasaponin II methyl ester (SSIIME; ID: 101638318), dehydrosoyasaponin I (DHSSI; ID: 656760), and phytic acid (PA; ID: 890) and other compounds (Supplementary Table S1).
2.2 Protein and ligand preparation
For docking experiments, the preparation of ligands was carried out using the Chimera (V: 1.15; https://www.cgl.ucsf.edu/chimera/download.html) and AutoDock Vina (V: 1.5.7) (http://vina.scripps.edu/download.html) was used for the preparation of a receptor (protein). Protein preparation was performed by using the AutoDock suite. The energy minimizations for both proteins and ligands were carried out (http://www.yasara.org/minimizationserver.htm) (Krieger et al., 2009). Heteroatoms including water were deleted, polar hydrogens were added, non-polar hydrogens were merged, and both Kollman and Gasteiger charges were added. Ligands downloaded from PubChem in an SDF format were converted into the PDB format using Open Babel (http://openbabel.org/wiki/Main_Page). Charges of the ligands were set to neutral, and Gasteiger charge was added. The number of torsions was kept as default.
2.3 Protein–ligand docking
Molecular docking was performed using the AutoDock Vina tool (Trott & Olson, 2010). Blind docking was performed initially followed by precision docking. The spacing angstrom was set to 1, and then, the grid box was adjusted manually to cover all the active-site residues of the receptor. The dimensions of the grid box were set as X = 30, Y = 30, and Z = 30, and the center grid box was set with the coordinates as center x = 49.257, center y = 37.37, and center z = 43.69 for cACE and center x = 28.259, center y = 13.045, and center z = 7.095 for nACE, and the grid box dimensions were X = 30, Y = 30, and Z = 30. Exhaustiveness was set to 8 for both proteins. All the dockings were performed in three replicates (Morris et al., 2009; Cosconati et al., 2010; Forli & Olson, 2012). The molecular docking was also carried out using InstaDock (Mohammad et al., 2020) (Supplementary Appendix S1).
2.4 Standard dynamic simulations
The dynamic simulation studies were performed using Discovery Studio V.4.0 with the enzymes (nACE and cACE) in docked states with reference captopril and BS. Standard dynamic cascades were applied where the first minimization algorithm was set to the steepest descent with maximum steps of 2000 and RMS gradient 1.0. The second minimization algorithm was set to conjugate gradient with maximum steps of 1,000 and RMS gradient 0.1. The heating phase was adjusted to possess a simulation time of 4 ps and an interval of 2 ps. The initial temperature was set to 50 and the target temperature to 300 with a maximum velocity of 2000. On the other hand, the equilibration phase was set with a simulation time of 10 ps and an interval of 2 ps. The target temperature was adjusted similar to the heating phase at 300, with a maximum velocity of 1000. The implicit solvent model was set to generalized born with a simple switching (GBSW), and the dynamics integrator protocol used leapfrog–Verlet (Huang and Leimkuhler, 1997; Im et al., 2003).
2.5 Visualization of poses
PyMoL (https://www.pymol.org/pymol.html) is used for the visualization and analysis of the docked poses and protein structures and for rendering the figures, while the LigPlot+ (V: 2.2.5) and Discovery Studio Visualizer (https://discover.3ds.com/discovery-studio-visualizer-download) were used to get the two-dimensional protein–ligand interactions (Laskowski & Swindells, 2011; Schrodinger, 2021). Cartoons of protein–ligand interactions were rendered in the complexes using Chimera (Pettersen et al., 2004).
3 Results
3.1 Molecular modeling studies
The binding of six compounds of soybean, namely, beta-sitosterol, soyasaponin I, soyasaponin II, soyasaponin II methyl ester, dehydrosoyasaponin I, and phytic acid (thereon BS, SSI, SSII, SSIIME, DHSSI, and PA, respectively), to the catalytic sites of both cACE and nACE was investigated using a molecular docking approach. The ACE is the ellipsoidal structure, traversed by a long and deep active-site cleft which is composed primarily of alpha-helices. The cavity is formed at the binding site which contains a
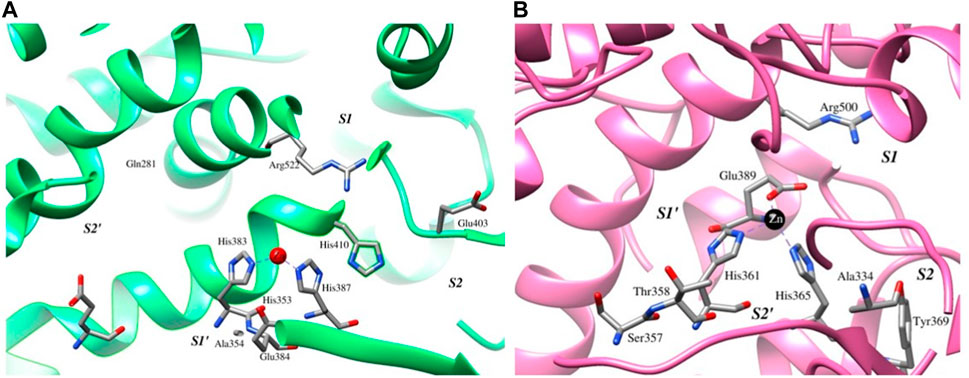
FIGURE 1. Active-site and other conserved residues are represented as sticks, enclosing the catalytic pocket and conserved HEXXH motif depicted as green and pink ribbons, and the catalytic Zn is depicted as the red and black spheres along with the subsites for (A) cACE and (B) nACE, respectively.
3.2 Inhibitor-binding sites and their interactions
The distribution of interacting amino acid residues with the ligands (PA and BS) is shown in Table 1. The docking results for the PA–cACE complex reveal that it formed 11 direct hydrogen bonds with the Asn66 (3.14
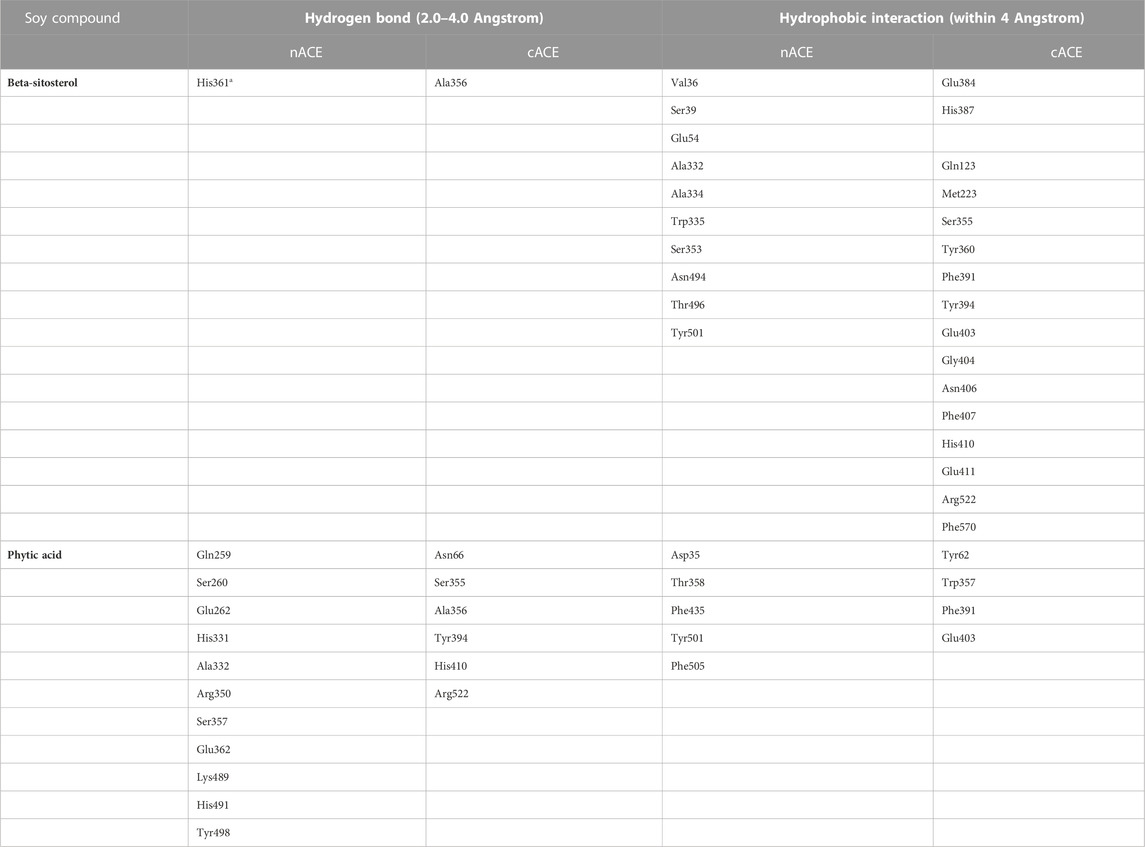
TABLE 1. Amino acid residues involved in ACE interactions with soy compounds forming direct hydrogen bonds and hydrophobic interactions (a—active-site residues).
For cACE, the amino acid residues His410 and Ala354 are positioned in the
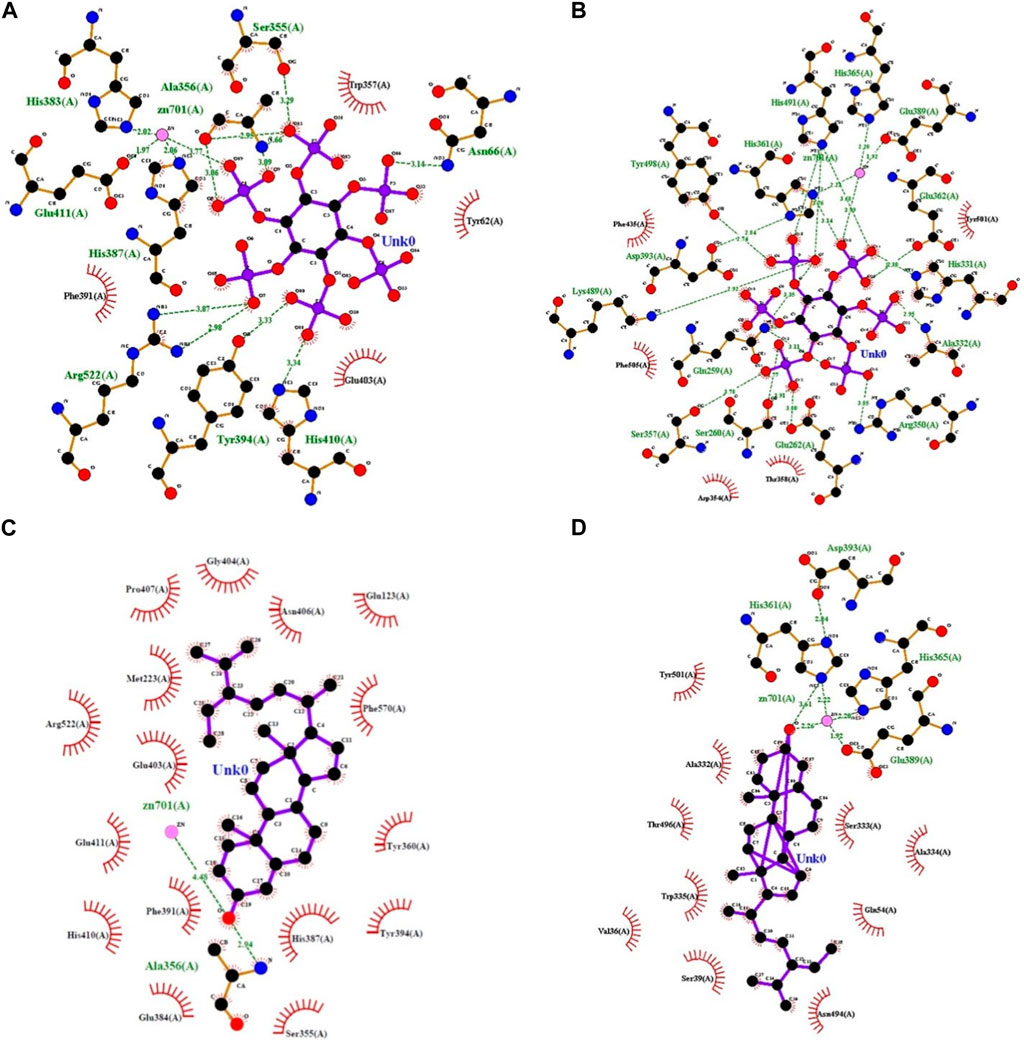
FIGURE 2. LigPlot representations of phytocompound interactions with catalytic site residues of PA-cACE (A), PA-nACE (B), BS-cACE (C), and BS-nACE (D) (H-bond/electrostatic interactions are shown in green, and the residues which are involved only in hydrophobic interactions are represented by red semicircles).
The nACE–PA binding involves fifteen hydrogen bonds with the Gln259 (3.11
In the case of BS, the binding with cACE involves only one hydrogen bond at the site other than catalytic subsites, namely, Ala356 (2.94
For the nACE–BS binding, the ligand formed only one hydrogen bond with a catalytic residue His361 with a 3.61
All 34 compounds and three reference compounds were subjected to docking analysis using InstaDock and presented a binding affinity within the range of −5.4 kcal/mol (Cap) to −15.1 kcal/mol (Urs) toward cACE and for nACE ranged from −5.3 kcal/mol (Cap) to −15.5 kcal/mol (Urs). The binding affinities and other docking parameters of each compound used in this study are shown in Supplementary Tables S2, S3. Both the dockings revealed that the binding affinities of the reference compounds, namely, quinapril, lisinopril, and captopril, with cACE and nACE were −9.5, −8.6, and −5.4 kcal/mol and −8.7, −7.5, and −5.3 kcal/mol, respectively.
3.3 Standard dynamic simulations
To investigate further, standard dynamic simulations (DSs) and analyzed trajectory studies were performed using the Discovery Studio 4.0 to confirm the nature of the stability of both cACE and nACE and the produced conformations after docking with the most active promising compound compared to reference captopril and their free states. The stability could be reflected via total energy calculations versus time and root mean square deviation (RMSD) versus conformations. The total energy decreases with time in the case of BS–cACE, while against the same time frame docked with the enzyme and captopril, the total energy decreases as well (Figure 3). Similarly, for nACE, the simulation studies showed a decrease in the total energy versus time with both BS and captopril and a slight increase after 22 ps in BS vs. nACE while there is a steep increase with captopril vs. nACE (Figure 3) (features of the run are shown in Supplementary Tables S4, S5).
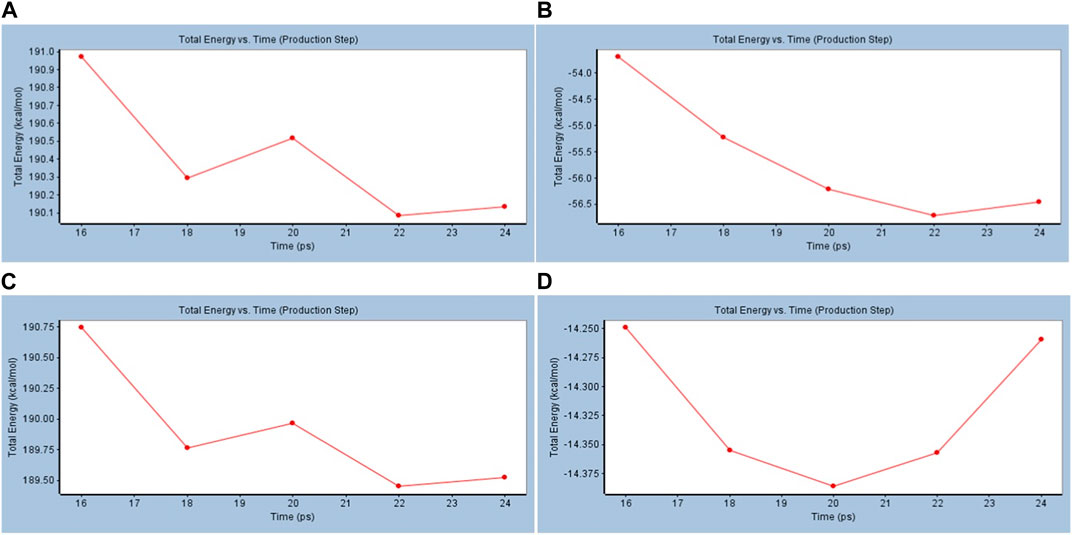
FIGURE 3. Standard dynamic simulation depicting the total energy versus time in the production step using beta-sitosterol. (A) BS vs. cACE, (B) captopril vs. cACE, (C) BS vs. nACE, and (D) captopril vs. nACE.
3.4 RMSD analysis
The value of RMSD is an indicator of the stability of the receptor–ligand complex. The RMSD versus conformation results showed that in both BS and captopril with cACE, the RMSD increased (Figure 4). Similarly, in the simulations with the nACE, studies showed an increase in the RMSD with both BS and captopril (Figure 4).
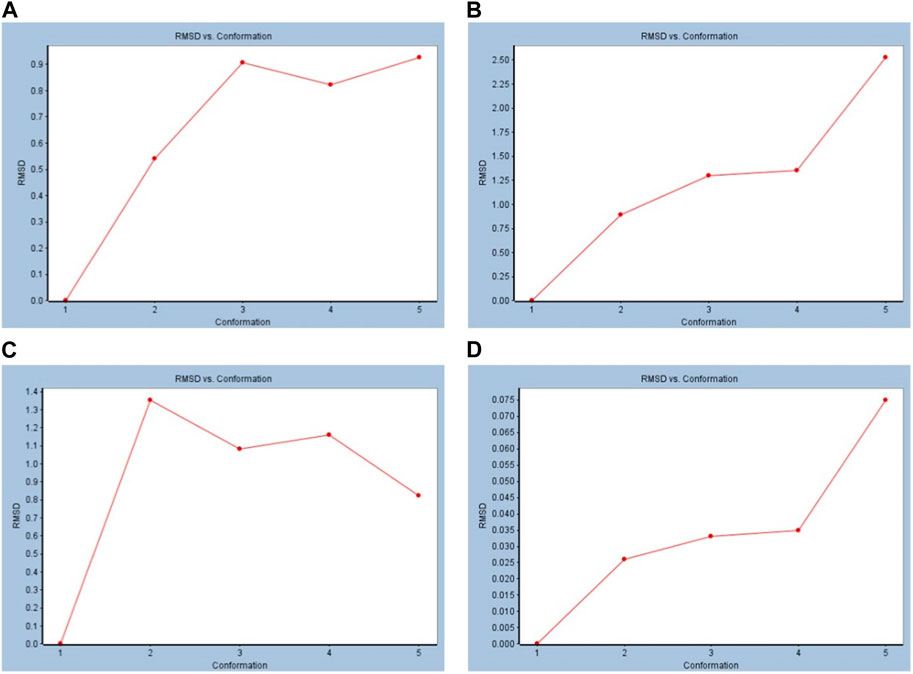
FIGURE 4. Standard dynamic simulation depicting the RMSD versus time in the production step using beta-sitosterol. (A) BS vs. cACE, (B) captopril vs. cACE, (C) BS vs. nACE, and (D) captopril vs. nACE.
4 Discussion
Despite the availability of commercial drugs for ACE inhibition, the lower success rates of drugs and prolonged treatment procedures with persistent side effects (angioedema and cough) and with no one-time remedy (Sharma et al., 2016) necessitate the search for natural solutions against it. Moreover, since the outbreak of the pandemic and even before its occurrence, herbal medicines were the preferred choice over synthetic drugs due to their side effects (Karimi et al., 2015; Farooq and Ngaini, 2021). Therefore, phytocompounds are being searched for their inhibitory activity against ACE as an alternative therapy. In the current study, different compounds of soybean were used to investigate and study the interactions in the search of the inhibitory activity for selected compounds and predicting binding affinities with both the ACE domains, namely, cACE and nACE, through the in silico approach. The compounds studied, namely, beta-sitosterol, soyasaponin I, soyasaponin II, soyasaponin II methyl ester, dehydrosoyasaponin I, and phytic acid, were found to be effective in controlling hypertension and cardiovascular diseases (Isanga and Zhang, 2008; Swallah et al., 2022). The compounds presented in this study are a small subset and were randomly chosen from the bioactive compounds (Supplementary Figure S1) of soybean known to be involved in controlling CVDs.
Among the docked compounds, phytic acid was found to be more potent as compared to the rest of the compounds in terms of more hydrogen bonds (10 and 17 with cACE and nACE, respectively) than BS (two each) interactions with residues at ACE catalytic subsites ensuring a stronger binding with respect to molecular docking. It is observed that phytic acid is a moderate cACE and nACE inhibitor, while the other beta-sitosterol exhibited selective inhibition profiles for the N domain of ACE. However, BS is observed to be stable with the DS analysis and formed 83 new hydrogen bonds ranging from 1.413–1.09
5 Conclusion and future prospects
ACE is a key enzyme in the RAAS which helps in the regulation of hypertension. The overproduction of angiotensin by the activity of ACE leads to a medical condition known as hypertension, and consumption of synthetic drugs causes many side effects and sometimes even death. Therefore, it becomes very important to control and inhibit hypertension and ACE using natural compounds such as phytocompounds like saponins, terpenes, and isoflavonoids. In the current study, the molecular basis of the selectivity of some soy compounds as candidate ACE inhibitors was determined through in silico molecular-docking approaches. The rest of the compounds (Supplementary Table S1) need to be evaluated in detail. Overall, among the interactions studied using the in silico and MD approaches, the BS confirmed the stability and indicated its potential to be developed as an ACE drug to combat hypertension and cardiovascular diseases. The results obtained in this study need to be confirmed and validated through experiments and in vivo studies, and also, they must go through proper preclinical and clinical trials for further scientific validation. Moreover, natural compounds and other phytoconstituents should be searched for their inhibitory activity against ACE for a safer alternative and future drug design, and this study will serve as a starting point in this direction.
Data availability statement
The original contributions presented in the study are included in the article/Supplementary Material; further inquiries can be directed to the corresponding author.
Author contributions
AyR and AmR contributed to the conception and design of the study. AyR wrote the first draft, curated the data and performed the analysis. IB assisted in performing the dockings and helped to develop the manuscript. AyR, AN, PB, SM, SrS, AmR, VK, ST, MS, BPM, and ShS contributed to the manuscript revision, editing and fund acquisition. AyR, IB, and IMF analysed the results. IMF, SKL, and AmR have supervised and guided the study. All authors contributed to the article and approved the submitted version.
Acknowledgments
AyR and AmR would like to thank Dr. Urvashi Sharma, Ramalingaswami Fellow, Institute of Bioinformatics and Applied Biotechnology (IBAB), Bengaluru, India, for the preparation of the manuscript and figures and Dr Dhandapani Raju, Senior Scientist, Division of Plant Physiology, ICAR-Indian Agricultural Research Institute, Pusa Campus, New Delhi, India, for his helpful advice in draft preparation.
Conflict of interest
The authors declare that the research was conducted in the absence of any commercial or financial relationships that could be construed as a potential conflict of interest.
Publisher’s note
All claims expressed in this article are solely those of the authors and do not necessarily represent those of their affiliated organizations, or those of the publisher, the editors, and the reviewers. Any product that may be evaluated in this article, or claim that may be made by its manufacturer, is not guaranteed or endorsed by the publisher.
Supplementary material
The Supplementary Material for this article can be found online at: https://www.frontiersin.org/articles/10.3389/fphys.2023.1172684/full#supplementary-material
References
Agrawal, V., Gupta, J. K., Qureshi, S. S., and Vishwakarma, V. K. (2016). Role of cardiac renin angiotensin system in ischemia reperfusion injury and preconditioning of heart. Indian heart J. 68 (6), 856–861. doi:10.1016/j.ihj.2016.06.010
Atlas, S. A. (2007). The renin-angiotensin aldosterone system: Pathophysiological role andpharmacologic inhibition. J. Manag. Care Pharm. 13 (8), 9–20. doi:10.18553/jmcp.2007.13.s8-b.9
Caballero, J. (2020). Considerations for docking of selective angiotensin-converting enzyme inhibitors. Molecules 25 (2), 295. doi:10.3390/molecules25020295
Caponio, G. R., Wang, D. Q. H., Di Ciaula, A., De Angelis, M., and Portincasa, P. (2020). Regulation of cholesterol metabolism by bioactive components of soy proteins: Novel translational evidence. Int. J. Mol. Sci. 22 (1), 227. doi:10.3390/ijms22010227
Chamata, Y., Watson, K. A., and Jauregi, P. (2020). Whey-derived peptides interactions with ACE by molecular docking as a potential predictive tool of natural ACE inhibitors. Int. J. Mol. Sci. 21 (3), 864. doi:10.3390/ijms21030864
Chen, K. I., Erh, M. H., Su, N. W., Liu, W. H., Chou, C. C., and Cheng, K. C. (2012). Soyfoods and soybean products: From traditional use to modern applications. Appl. Microbiol. Biotechnol. 96 (1), 9–22. doi:10.1007/s00253-012-4330-7
Cosconati, S., Forli, S., Perryman, A. L., Harris, R., Goodsell, D. S., and Olson, A. J. (2010). Virtual screening with AutoDock: Theory and practice. Expert Opin. Drug Discov. 5 (6), 597–607. doi:10.1517/17460441.2010.484460
Cozier, G. E., Arendse, L. B., Schwager, S. L., Sturrock, E. D., and Acharya, K. R. (2018b). Molecular basis for multiple omapatrilat binding sites within the ACE C-domain: Implications for drug design. J. Med. Chem. 61 (22), 10141–10154. doi:10.1021/acs.jmedchem.8b01309
Cozier, G. E., Schwager, S. L., Sharma, R. K., Chibale, K., Sturrock, E. D., and Acharya, K. R. (2018a). Crystal structures of sampatrilat and sampatrilat-Asp in complex with human ACE–a molecular basis for domain selectivity. FEBS J. 285 (8), 1477–1490. doi:10.1111/febs.14421
Farooq, S., and Ngaini, Z. (2021). Natural and synthetic drugs as potential treatment for coronavirus disease 2019 (COVID-2019). Chem. Afr. 4 (1), 1–13. doi:10.1007/s42250-020-00203-x
Forli, S., and Olson, A. J. (2012). A force field with discrete displaceable waters and desolvation entropy for hydrated ligand docking. J. Med. Chem. 55 (2), 623–638. doi:10.1021/jm2005145
Handa, C. L., Zhang, Y., Kumari, S., Xu, J., Ida, E. I., and Chang, S. K. (2020). Comparative study of angiotensin I-converting enzyme (ACE) inhibition of soy foods as affected by processing methods and protein isolation. Processes 8 (8), 978. doi:10.3390/pr8080978
Hermida, R. C., Ayala, D. E., Fernández, J. R., Portaluppi, F., Fabbian, F., and Smolensky, M. H. (2011). Circadian rhythms in blood pressure regulation and optimization of hypertension treatment with ACE inhibitor and ARB medications. Am. J. Hypertens. 24 (4), 383–391. doi:10.1038/ajh.2010.217
Huang, W., and Leimkuhler, B. (1997). The adaptive Verlet method. SIAM J. Sci. Comput. 18 (1), 239–256. doi:10.1137/s1064827595284658
Im, W., Lee, M. S., and Brooks, C. L. (2003). Generalized born model with a simple smoothing function. J. Comput. Chem. 24 (14), 1691–1702. doi:10.1002/jcc.10321
Isanga, J., and Zhang, G. N. (2008). Soybean bioactive components and their implications to health—A review. Food Rev. Int. 24 (2), 252–276. doi:10.1080/87559120801926351
Karimi, A., Majlesi, M., and Rafieian-Kopaei, M. (2015). Herbal versus synthetic drugs; beliefs and facts. J. Nephropharmacology 4 (1), 27–30.
Khan, M. Y., and Kumar, V. (2019). Mechanism & inhibition kinetics of bioassay-guided fractions of Indian medicinal plants and foods as ACE inhibitors. J. traditional complementary Med. 9 (1), 73–84. doi:10.1016/j.jtcme.2018.02.001
Kircheva, N., Dobrev, S., Yakimova, B., Stoineva, I., and Angelova, S. (2021). Molecular insights into the interaction of angiotensin I-converting enzyme (ACE) inhibitors and HEXXH motif. Biophys. Chem. 276, 106626. doi:10.1016/j.bpc.2021.106626
Krieger, E., Joo, K., Lee, J., Lee, J., Raman, S., Thompson, J., et al. (2009). Improving physical realism, stereochemistry, and side-chain accuracy in homology modeling: Four approaches that performed well in CASP8. Proteins Struct. Funct. Bioinforma. 77 (S9), 114–122. doi:10.1002/prot.22570
Kumar, R., Saini, M., Taku, M., Debbarma, P., Mahto, R. K., Ramlal, A., et al. (2022). Identification of quantitative trait loci (QTLs) and candidate genes for seed shape and 100-seed weight in soybean [Glycine max (L) Merr]. Front. Plant Sci. 13, 1074245. doi:10.3389/fpls.2022.1074245
Laskowski, R. A., and Swindells, M. B. (2011). LigPlot+: Multiple ligand–protein interaction diagrams for drug discovery. J. Chem. Inf. Model. 51 (10), 2778–2786. doi:10.1021/ci200227u
Li, T., Zhang, X., Ren, Y., Zeng, Y., Huang, Q., and Wang, C. (2022). Antihypertensive effect of soybean bioactive peptides: A review. Curr. Opin. Pharmacol. 62, 74–81. doi:10.1016/j.coph.2021.11.005
Mandal, A., Cho, H., and Chauhan, B. S. (2022). Experimental investigation of multiple fry waste soya bean oil in an agricultural CI engine. Energies 15 (9), 3209. doi:10.3390/en15093209
Margalef, M., Bravo, F. I., Arola-Arnal, A., and Muguerza, B. (2017). Natural angiotensin converting enzyme (ACE) inhibitors with antihypertensive properties. Nat. Prod. Target. Clin. Relev. Enzym., 45–67. doi:10.1002/9783527805921.ch3
Mohammad, T., Mathur, Y., and Hassan, M. I. (2020). InstaDock: A single-click graphical user interface for molecular docking-based virtual high-throughput screening. Briefings Bioinforma. 22 (4), bbaa279–8. doi:10.1093/bib/bbaa279
Morris, G. M., Huey, R., Lindstrom, W., Sanner, M. F., Belew, R. K., Goodsell, D. S., et al. (2009). AutoDock4 and AutoDockTools4: Automated docking with selective receptor flexibility. J. Comput. Chem. 30 (16), 2785–2791. doi:10.1002/jcc.21256
Natesh, R., Schwager, S. L., Sturrock, E. D., and Acharya, K. R. (2003). Crystal structure of the human angiotensin-converting enzyme–lisinopril complex. Nature 421, 551–554. doi:10.1038/nature01370
Patten, G. S., Abeywardena, M. Y., and Bennett, L. E. (2016). Inhibition of angiotensin converting enzyme, angiotensin II receptor blocking, and blood pressure lowering bioactivity across plant families. Crit. Rev. Food Sci. Nutr. 56 (2), 181–214. doi:10.1080/10408398.2011.651176
Pettersen, E. F., Goddard, T. D., Huang, C. C., Couch, G. S., Greenblatt, D. M., Meng, E. C., et al. (2004). UCSF Chimera—A visualization system for exploratory research and analysis. J. Comput. Chem. 25 (13), 1605–1612. doi:10.1002/jcc.20084
Rajendran, A., and Lal, S. K. (2020). Assessing the need of pre-germination anaerobic stress-tolerant varieties in Indian Soybean (Glycine max (L) Merrill). Natl. Acad. Sci. Lett. 43, 593–597. doi:10.1007/s40009-020-00937-9
Rajendran, A., Lal, S. K., Raju, D., and Ramlal, A. (2022). Associations of direct and indirect selection for pregermination anaerobic stress tolerance in soybean (Glycine max). Plant Breed. 141, 634–643. doi:10.1111/pbr.13048
Ramlal, A., Nautiyal, A., Baweja, P., Mahto, R. K., Mehta, S., Mallikarjun, B. P., et al. (2022a). Harnessing heterosis and male sterility in soybean (Glycine max (L) Merr): A critical revisit. Front. Plant Sci. 13, 981768. doi:10.3389/fpls.2022.981768/
Ramlal, A., Dey, N., Sharma, U., and Rajendran, A. (2022b). “In-silico studies to reveal the potential inhibitory capacity of soy isoflavonoids against angiotensin-converting enzyme,” in Coreference Proceedings: Sustainable future for humanity: The new learning curve. Editors H. Chopra, V. Ghuriani, P. M. Arora, S. Babbar, and P. Baweja (Mumbai: Imperial Publications), 155–167.
Ramlal, A., Nautiyal, A., Baweja, P., Kumar, V., Mehta, S., Mahto, R. K., et al. (2022c). Angiotensin-converting enzyme inhibitory peptides and isoflavonoids from soybean [Glycine max (L) Merr]. Front. Nutr. 9, 1068388. doi:10.3389/fnut.2022.1068388
Roth, G. A., Mensah, G. A., Johnson, C. O., Addolorato, G., Ammirati, E., Baddour, L. M., et al. GBD-NHLBI-JACC Global Burden of Cardiovascular Diseases Writing Group (2020). Global burden of cardiovascular diseases and risk factors, 1990–2019: Update from the GBD 2019 study. J. Am. Coll. Cardiol. 76 (25), 2982–3021. doi:10.1016/j.jacc.2020.11.010
Sharma, R. K., Espinoza-Moraga, M., Poblete, H., Douglas, R. G., Sturrock, E. D., Caballero, J., et al. (2016). The dynamic nonprime binding of sampatrilat to the C-domain of angiotensin-converting enzyme. J. Chem. Inf. Model. 56 (12), 2486–2494. doi:10.1021/acs.jcim.6b00524
Shimakage, A., Shinbo, M., and Yamada, S. (2012). ACE inhibitory substances derived from soy foods. J. Biol. Macromol. 12, 72–80. doi:10.14533/jbm.12.72
Sitanggang, A. B., Putri, J. E., Palupi, N. S., Hatzakis, E., Syamsir, E., and Budijanto, S. (2021). Enzymatic preparation of bioactive peptides exhibiting ACE inhibitory activity from soybean and velvet bean: A systematic review. Molecules 26 (13), 3822. doi:10.3390/molecules26133822
Swallah, M. S., Yang, X., Li, J., Korese, J. K., Wang, S., Fan, H., et al. (2022). The pros and cons of soybean bioactive compounds: An overview. Food Rev. Int., 1–28. doi:10.1080/87559129.2022.2062763
Tahir, R. A., Bashir, A., Yousaf, M. N., Ahmed, A., Dali, Y., Khan, S., et al. (2020). In silico identification of angiotensin-converting enzyme inhibitory peptides from MRJP1. PloS one 15 (2), e0228265. doi:10.1371/journal.pone.0228265
Trott, O., and Olson, A. J. (2010). AutoDock vina: Improving the speed and accuracy of docking with a new scoring function, efficient optimization, and multithreading. J. Comput. Chem. 31 (2), 455–461. doi:10.1002/jcc.21334
Xu, Z., Wu, C., Sun-Waterhouse, D., Zhao, T., Waterhouse, G. I., Zhao, M., et al. (2021). Identification of post-digestion angiotensin-I converting enzyme (ACE) inhibitory peptides from soybean protein Isolate: Their production conditions and in silico molecular docking with ACE. Food Chem. 345, 128855. doi:10.1016/j.foodchem.2020.128855
Zhang, B., Liu, J., Wen, H., Jiang, F., Wang, E., and Zhang, T. (2022). Structural requirements and interaction mechanisms of ACE inhibitory peptides: Molecular simulation and thermodynamics studies on LAPYK and its modified peptides. Food Sci. Hum. Wellness 11 (6), 1623–1630. doi:10.1016/j.fshw.2022.06.021
Keywords: angiotensin-converting enzyme I, beta-sitosterol, cardiovascular diseases, natural drugs, renin–angiotensin–aldosterone system, phytocompounds, phytic acid, soybean
Citation: Ramlal A, Bhat I, Nautiyal A, Baweja P, Mehta S, Kumar V, Tripathi S, Mahto RK, Saini M, Mallikarjuna BP, Saluja S, Lal SK, Subramaniam S, Fawzy IM and Rajendran A (2023) In silico analysis of angiotensin-converting enzyme inhibitory compounds obtained from soybean [Glycine max (L.) Merr.]. Front. Physiol. 14:1172684. doi: 10.3389/fphys.2023.1172684
Received: 23 February 2023; Accepted: 02 May 2023;
Published: 31 May 2023.
Edited by:
Grazia Tamma, University of Bari Aldo Moro, ItalyReviewed by:
Giusy Rita Caponio, University of Bari Aldo Moro, ItalyMariangela Centrone, University of Bari Aldo Moro, Italy
Zhipeng Yu, Hainan University, China
Copyright © 2023 Ramlal, Bhat, Nautiyal, Baweja, Mehta, Kumar, Tripathi, Mahto, Saini, Mallikarjuna, Saluja, Lal, Subramaniam, Fawzy and Rajendran. This is an open-access article distributed under the terms of the Creative Commons Attribution License (CC BY). The use, distribution or reproduction in other forums is permitted, provided the original author(s) and the copyright owner(s) are credited and that the original publication in this journal is cited, in accordance with accepted academic practice. No use, distribution or reproduction is permitted which does not comply with these terms.
*Correspondence: Iten M. Fawzy, Iten.mamdouh@fue.edu.eg; Ambika Rajendran, rambikarajendran@gmail.com
†ORCID: Ayyagari Ramlal, orcid.org/0000-0002-1093-9877; Aparna Nautiyal, orcid.org/0000-0002-4731-1581; Ambika Rajendran, orcid.org/0000-0002-2223-9309