- 1Department of Respiratory Medicine, University Hospital Zurich, University of Zurich, Zurich, Switzerland
- 2Swiss-Kyrgyz High-Altitude Medicine and Research Initiative, Bishkek, Kyrgyzstan
- 3National Centre for Cardiology and Internal Medicine, Bishkek, Kyrgyzstan
Introduction: This prospective cohort study assessed the effects of chronic hypoxaemia due to high-altitude residency on the cerebral tissue oxygenation (CTO) and cerebrovascular reactivity.
Methods: Highlanders, born, raised, and currently living above 2,500 m, without cardiopulmonary disease, participated in a prospective cohort study from 2012 until 2017. The measurements were performed at 3,250 m. After 20 min of rest in supine position while breathing ambient air (FiO2 0.21) or oxygen (FiO2 1.0) in random order, guided hyperventilation followed under the corresponding gas mixture. Finger pulse oximetry (SpO2) and cerebral near-infrared spectroscopy assessing CTO and change in cerebral haemoglobin concentration (cHb), a surrogate of cerebral blood volume changes and cerebrovascular reactivity, were applied. Arterial blood gases were obtained during ambient air breathing.
Results: Fifty three highlanders, aged 50 ± 2 years, participated in 2017 and 2012. While breathing air in 2017 vs. 2012, PaO2 was reduced, mean ± SE, 7.40 ± 0.13 vs. 7.84 ± 0.13 kPa; heart rate was increased 77 ± 1 vs. 70 ± 1 bpm (p < 0.05) but CTO remained unchanged, 67.2% ± 0.7% vs. 67.4% ± 0.7%. With oxygen, SpO2 and CTO increased similarly in 2017 and 2012, by a mean (95% CI) of 8.3% (7.5–9.1) vs. 8.5% (7.7–9.3) in SpO2, and 5.5% (4.1–7.0) vs. 4.5% (3.0–6.0) in CTO, respectively. Hyperventilation resulted in less reduction of cHb in 2017 vs. 2012, mean difference (95% CI) in change with air 2.0 U/L (0.3–3.6); with oxygen, 2.1 U/L (0.5–3.7).
Conclusion: Within 5 years, CTO in highlanders was preserved despite a decreased PaO2. As this was associated with a reduced response of cerebral blood volume to hypocapnia, adaptation of cerebrovascular reactivity might have occurred.
1 Introduction
Approximately 140 million people live permanently at altitudes above 2,500 m (Moore et al., 1998). Due to a general growth in world-wide population, the number of people living at such altitudes can also assumed to be growing. Chronic altitude exposure and associated chronic hypoxaemia can have severe pathophysiological effects on the human body leading to chronic altitude related illnesses, such as high-altitude pulmonary hypertension (HAPH) (León-Velarde et al., 2005; Furian et al., 2015). HAPH is associated with excessive pulmonary vasoconstriction, further hypoxaemia, right heart dysfunction and potentially, premature death (León-Velarde et al., 2005). However, whether chronic hypoxaemia due to high-altitude residency or HAPH has an impact on cerebrovascular homeostasis and reactivity, has not been extensively studied.
Oxygen is essential for the brain; not only for its cognitive functions, but also to enable the brain to maintain its governor functions regulating numerous physiologic and metabolic processes. To ensure adequate oxygen delivery, cerebral blood vessels underlie calibre modulating autoregulatory mechanisms. They are activated by triggers such as fluctuations in systemic blood pressure (Paulson et al., 1972) or alterations in the partial pressure of the blood gases (Lassen and Christensen, 1976). Dropping arterial partial pressure of carbon dioxide (PaCO2) and rising arterial partial pressure of oxygen (PaO2), induce cerebral vasoconstriction, while hypercapnia and hypoxia induce cerebral vasodilation (Poulin et al., 2002; Ainslie and Burgess, 2008; Willie et al., 2014).
In 2012, we assessed cerebral tissue oxygenation (CTO), and shift in cerebral haemoglobin concentration (cHb) at baseline and in response to hypocapnia and hyperoxia, as an index of regional cerebral blood volume and cerebrovascular reactivity, in Kyrgyz highlanders and lowlanders at their respective altitude of residence (3,250 and 760 m), using non-invasive near-infrared spectroscopy (NIRS) (Imray et al., 1998; Al-Rawi et al., 2001; McLeod et al., 2003; Ulrich et al., 2014). To stimulate a cerebrovascular response, hyperoxia and hypocapnia, as well as their combination, were experimentally induced (Furian et al., 2015). We found similar CTO and cerebrovascular reactivity in highlanders (with and without HAPH) compared to a control group of healthy lowlanders, while arterial oxygen saturation (SpO2) at rest was significantly lower in highlanders. However, the cross-sectional study design did not allow assessment of possible observed compensation of CTO changes over time, especially in highlanders (Furian et al., 2015). Therefore, it remains unclear whether the similar CTO and cerebrovascular reactivity observed in highlanders and lowlanders are unbiased or due to some sort of natural selection or other causes.
Therefore, the purpose of the current longitudinal study was to repeat the identical measurements in these highlanders after 5 years of permanent high-altitude residency and investigate the progression of cerebral homeostasis and cerebrovascular reactivity in response to years living at high altitude and potential alterations in blood gases.
Based on the initial findings in 2012, we tested the hypotheses that in highlanders, CTO at rest remains preserved and increases under hyperoxia, while cHb decreases, as vasoconstriction occurs. Hypocapnia would reduce CTO and cHb. The combination of hyperoxia and hypocapnia presumably would cause cHb to decrease while CTO remains unaltered. Additionally, in a subgroup analysis, we investigated whether highlanders with and without HAPH differ in the progression of CTO and cerebral reactivity during the time course of 5 years.
2 Materials and methods
To assure comparability, the experimental design and measurement methods of the current study were identical to those of the previously published study in 2012 (Furian et al., 2015).
2.1 Participants
In 2012, Kyrgyz highlanders, born, raised, and currently living between 2,500 and 3,500 m, aged 18–80 years, were recruited on the Aksay high altitude plateau, and studied at the Aksay health post (3,250 m; barometric pressure 519 mmHg), Kyrgyzstan. The diagnosis of HAPH in 2012 was based upon a mean pulmonary artery pressure (mPAP) > 30 mmHg in the absence of excessive erythrocytosis (haemoglobin concentration in females <19 g/dL, in males <21 g/dL) and other diseases leading to hypoxaemia, such as cardiopulmonary diseases (León-Velarde et al., 2005). Exclusion criteria were other relevant cardiopulmonary disease, heavy smoking (>10 cigarettes per day or >25 pack-years) (Galie et al., 2016).
All participating highlanders from 2012 were invited to join the current study conducted in 2017 at the Aksay health post. The same exclusion criteria were applied. In conformity with a per-protocol analysis only data of subjects participating in both studies were analysed. Informed written consent was obtained, as well as approval by the ethics committee of the National Centre of Cardiology and Internal Medicine, Bishkek, Kyrgyzstan (01-8/433).
2.2 Design and interventions
Examination of the highlanders took place in supine position, breathing through a reservoir bag equipped face mask with a one-way exhalation valve. Ambient air was administered by a continuous positive airway pressure generator (REMstar; Philips Respironics, Zofingen, Switzerland). The fractional inspired oxygen (FiO2) mixtures of 100% (FiO2 1.0), i.e., oxygen, and 21% (FiO2 0.21), i.e., air, at a flow rate 10 L/min, were administered according to a randomized cross-over design. After a 20 min baseline period, a 20 min recording period followed, in which the participants were breathing air or oxygen, respectively. The participants were blinded to the inhaled gas mixture. At the end of each period, the participants were asked to hyperventilate until end-tidal partial pressure of carbon dioxide (PetCO2) was reduced by >10 mmHg for at least 15 s. Another period of 10 min of quiet breathing was affiliated, allowing the effects of hyperventilation, and if given hyperoxia, to wash out. Finally, the hyperventilation procedure was repeated with the alternative gas mixture. During the examination, the participants were not allowed to sleep.
2.3 Measurements
NIRS was performed using a NIRO 200NX device (Hamamatsu, Japan) emitting light at 735, 810 and 850 nm. Optode positioning in both studies followed the same protocol of bilateral placement at Fp1 and Fp2, high on the forehead, 4 cm apart, and secured with adhesive tape (Ulrich et al., 2014). The Hamamatsu NIRO device was chosen because of its use in our previous cross-sectional study performed on these highlanders. Its ability to track changes in CTO adequately when compared to the invasive techniques of jugular venous saturation and direct tissue probe measurement of brain oxygen tension is well established (Al-Rawi et al., 2001; McLeod et al., 2003). The NIRO 200NX device measures the changes in concentrations of oxygenated haemoglobin, ∆O2Hb (µmol/L), and deoxygenated haemoglobin ∆HHb (µmol/L) as well as the tissue oxygenation index (%), representing CTO. Changes in cHb (a surrogate for changes in blood volume) were calculated as ∆O2Hb + ∆HHb. NIRS data were recorded at 2 Hz along with ECG, finger pulse oximetry and capnography of expired air (Capnocheck Sleep; Smiths Medical PM Inc., Waukesha, WI, United States). The final 2 min of stable quiet breathing with FiO2 0.21 and FiO2 1.0 respectively, as well as the final 20–30 s of the hyperventilation plateau, were analysed. The data of the two NIRS channels were averaged. Arterial blood gases and haemoglobin concentration were obtained via a radial artery blood sample, drawn during quiet breathing with air (RapidPoint 405 & 500; Siemens, Zurich, Switzerland). Spirometry (EasyOne; NDD, Zurich, Switzerland) was performed with each participant (Miller et al., 2005) and Doppler echocardiography (SonoSite MicroMaxx; SonoSite Inc., Bothell, WA, United States) was used to measure the acceleration of peak velocity of pulmonary artery outflow, in order to derive mPAP (Kitabatake et al., 1983).
2.4 Statistics
All data were analysed using linear mixed regression models. Model fitting was tested with fitted residuals and random intercept QQ-plots. Due to the affirmed robustness of mixed models to violations of distributional assumptions, the normality of the data was considered irrelevant in the given data set (Arnau et al., 2013; Arnau et al., 2014; Schielzeth et al., 2020). In the mixed linear regression models, outcomes of interest were defined as dependent variable, whereas year of study (2017 vs. 2012) and intervention (air–rest, air–hyperventilation, oxygen–rest, oxygen–hyperventilation) were defined as fixed effects and participants as random effect. Data are presented as means ± SE. Differences among the tested conditions are expressed as mean difference with 95% confidence intervals. Further subgroup analysis was performed to evaluate variations taking the diagnosis of HAPH in 2012 into account. A probability of p < 0.05 was considered statistically significant. All statistical analyses were performed with R Studio (version 1.3.1056, R Studio Inc., San Francisco, United States) using the lmerTest package (version 3.1-2).
3 Results
Of 90 highlanders who participated in 2012, 13 moved to low altitude, 10 refused to participate, 7 had died in the past 5 years, 6 were excluded due to the onset of new co-morbidities and 1 due to technical failure, resulting in 53 highlanders included in the final analysis (Figure 1).
Data for all baseline measurements are summarized in Table 1 and Figure 2. Measurements during the interventions are summarized in Table 2, Table 3 and Figure 3.
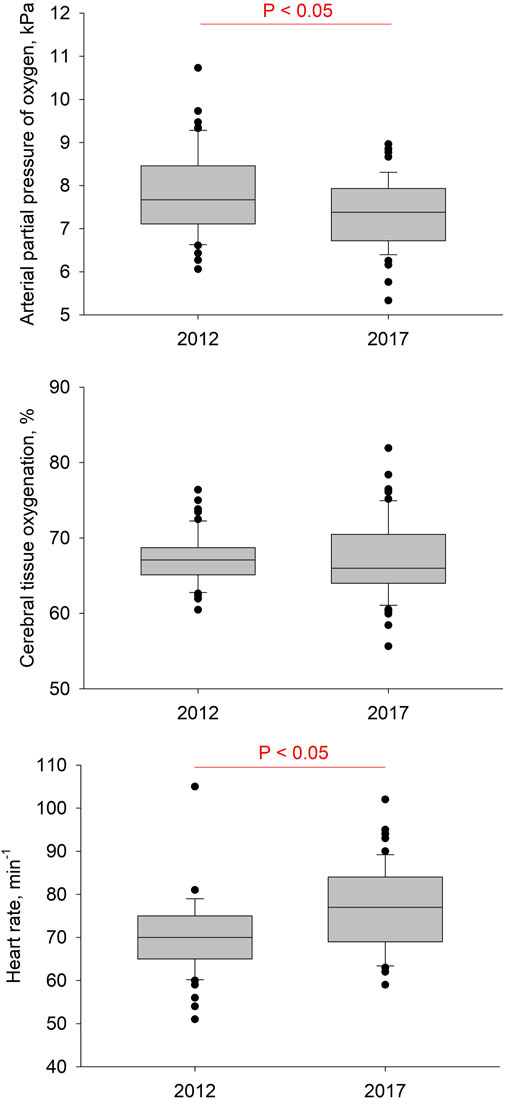
FIGURE 2. Results of baseline measurements at rest with ambient air. Boxes with lines represent medians and quartiles, whiskers represent the 10th and 90th percentiles, and dots represent individual values that fall outside the 10th–90th percentile range. Significant differences between years are indicated.
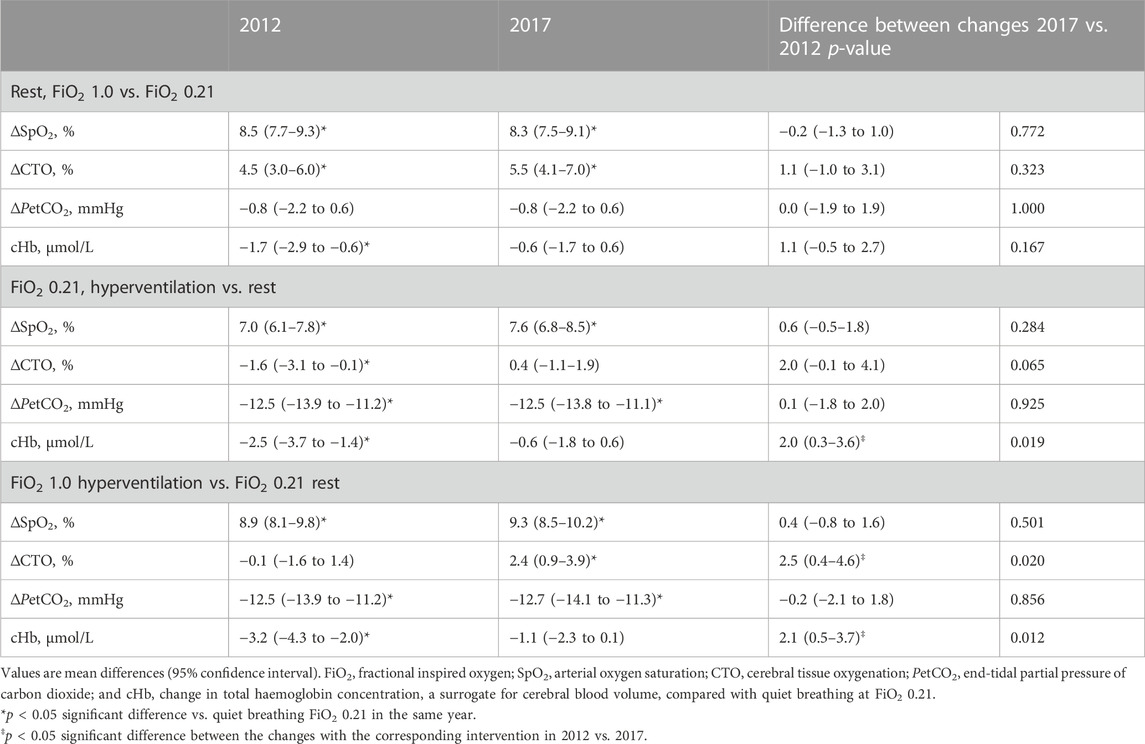
TABLE 3. Response in cerebral tissue oxygenation and cerebrovascular reactivity to induced hypocapnia and hyperoxia.
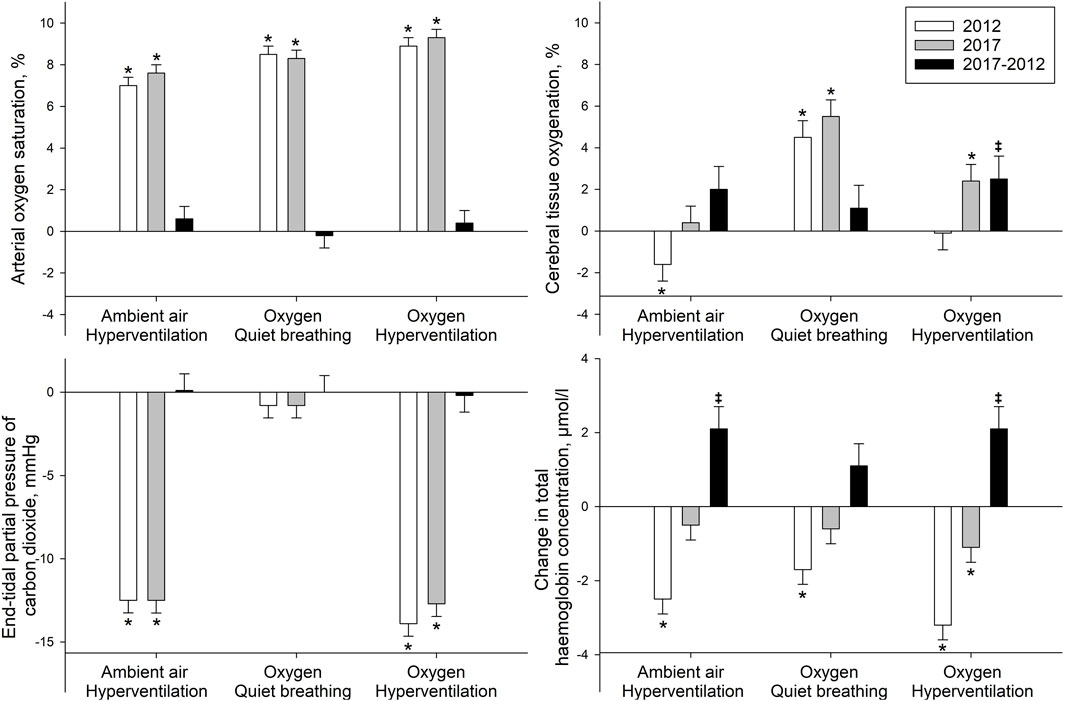
FIGURE 3. Change in physiological variables in response to hyperventilation and hyperoxia. Length of the bars show the given mean difference, whiskers show standard error, in comparison to quiet breathing under ambient air conditions (zero line, FiO2 0.21). *p < 0.05 significant difference versus breathing room air in same year; and ‡p < 0.05 significant difference between the differences within the same intervention in 2017 compared to 2012.
While breathing ambient air in 2017 compared to 2012, SpO2, CTO, PetCO2, PaCO2 and pH did not significantly differ, whereas PaO2 significantly decreased. Blood pressure did not significantly change, while mPAP, heart rate and breathing frequency rose significantly.
Breathing oxygen increased CTO and SpO2 similarly in 2017 and 2012. PetCO2 did not show significant changes neither compared to air, between 2017 and 2012. cHb decreased significantly in 2012 compared to breathing air, whereas it remained unchanged in 2017 (Table 3).
Hyperventilation while breathing ambient air decreased CTO significantly in 2012 while it slightly but insignificantly rose in 2017. The significant rise in SpO2 did not change over 5 years, as well as the reduction of PetCO2 by >10 mmHg did not change. cHb decreased significantly in 2012 but remained stable in 2017 with a significant difference between the years (Table 3).
Hyperventilation while breathing oxygen had no impact on CTO in 2012 but showed a significant increase in 2017 and also a significant difference between years. The significant rise in SpO2 did not change over the 5 years, nor did the reduction of PetCO2 by >10 mmHg did not change, however, like hyperventilation while breathing ambient air, cHb did not decrease as seen in 2012.
The interventions revealed that the overall increase in CTO during hyperventilation (FiO2 1.0) in 2017 vs. 2012 was mainly attributable to the HAPH group (71.7% ± 1.2% vs. 67.9% ± 1.2% HAPH; 68.6% ± 0.9% vs. 66.6% ± 0.9% highlanders without initially diagnosed HAPH) (data not shown), whereas the reduction in cHb during the same interventions followed a similar pattern in both groups (Table 5). The most evident difference in both groups was the uniformly higher SpO2 value in the highlanders without initially diagnosed HAPH group throughout all measurements.
4 Discussion
This prospective cohort study in highlanders permanently living above 2,500 m showed that after 5 years at high altitude we observe a reduction in PaO2 and increased HR and mPAP (Table 1) while CTO (FiO2 0.21 during quiet breathing) remained preserved (Figure 2). However, after 5 years, cerebrovascular reactivity in response to hypocapnia and hyperoxia revealed less stringent cerebrovascular vasoconstriction, which resulted in an increase of CTO compared to the same interventions in 2012. Whether these observed alterations in the cerebrovascular responsiveness should be interpreted as beneficial, due to maintenance of CTO, or deleterious, due to increased vascular stiffness and therefore less vasoconstriction, remains to be elucidated.
Known triggers of cerebral vasoconstriction, namely, hyperoxia and hypocapnia as well as their combination, were experimentally induced (Tables 2, 3; Figure 3). Hypocapnia was induced by hyperventilation and hyperoxia by breathing oxygen. Hyperventilation while breathing ambient air decreased CTO in 2012, but CTO remained unaffected in 2017. Hyperoxia increased CTO in both studies significantly. In 2017, we saw a trend for an even greater increase. The combination of hyperoxia and hyperventilation had little or no impact on CTO in 2012, but significantly increased CTO in 2017. cHb, which indicates changes of regional cerebral blood volume, displayed similar alterations in all three interventions. In 2012, it was significantly reduced, whereas in 2017, it remained unchanged or was reduced to a much lesser extent. Therefore, all changes reflecting cerebrovascular reactivity in response to the applied interventions indicate a mitigated response of cerebral blood vessels to the tested stimuli after 5 years living at high altitude. These results confirm findings from our previous cross-sectional study performed on these highlanders. Furian et al. (2015) reported preserved CTO in highlanders compared to healthy lowlanders at their respective altitude during resting conditions. However, during hypocapnia CTO in highlanders was essentially preserved, unlike the CTO reductions observed in healthy lowlanders. These observations are in accordance with the suggestion, that altitude-related adaptations of the cerebrovascular reactivity took place in these highlanders over the course of 5 years.
To gain further insight on whether these alterations in cerebrovascular reactivity were related to the presence of HAPH, exploratory subgroup analysis in highlanders diagnosed with HAPH in 2012 compared to highlanders without HAPH was performed. It revealed a similar pattern of reduced cerebrovascular reactivity in highlanders with and without HAPH. Therefore, the observed cerebrovascular changes were promoted by chronic hypoxaemia and age, rather than by differences in haemodynamic (Table 4).
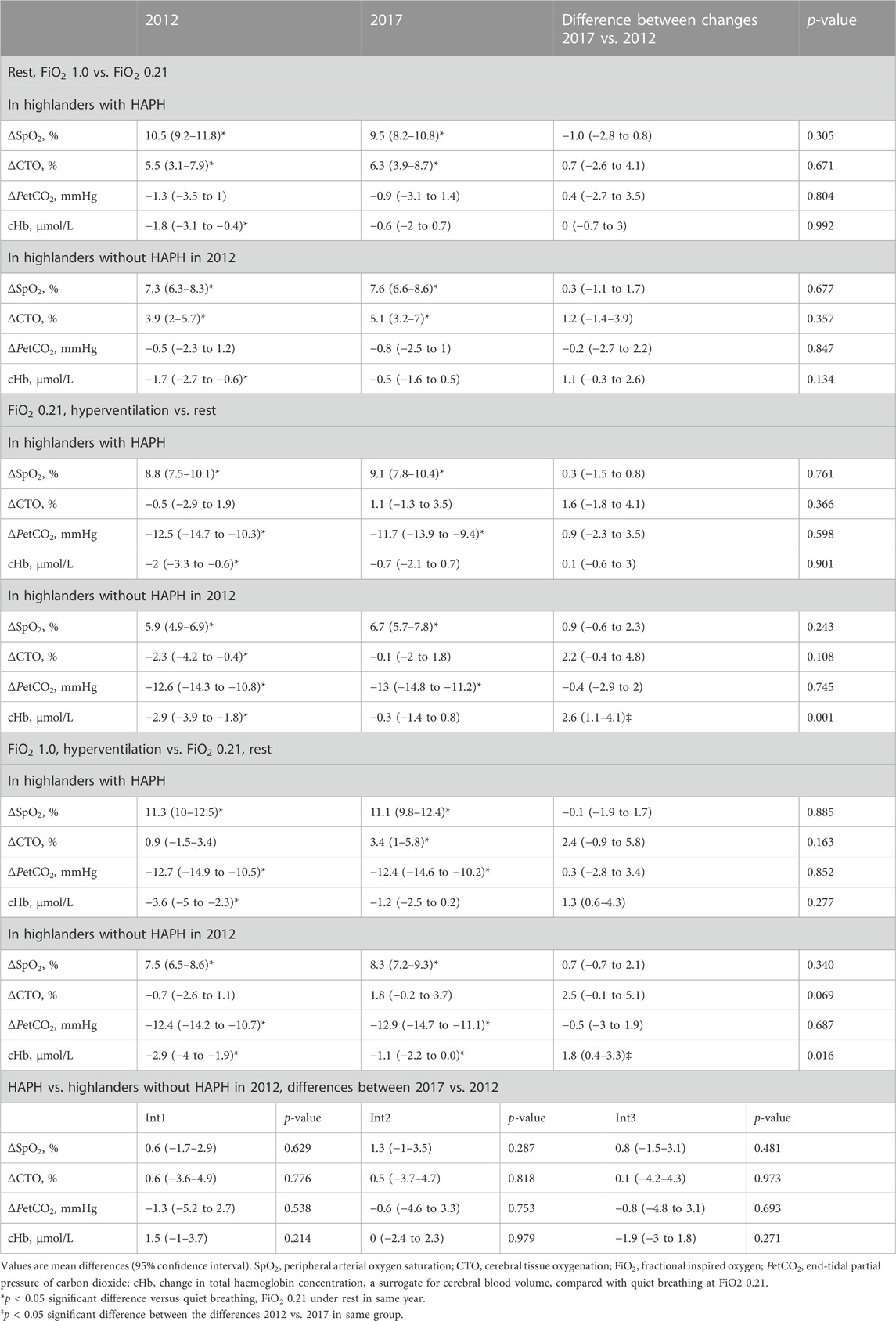
TABLE 5. Response in cerebral tissue oxygenation and cerebrovascular reactivity to induced hypocapnia and hyperoxia in subgroups.
When elaborating the effect of chronic hypoxaemia, we saw worsening of arterial oxygenation over 5 years, therefore, a hypocapnic manoeuvre results in a higher increase in arterial oxygenation vs. decreased CO2. This balance towards higher elevation in SpO2 with hypocapnia would induce a larger increase in CTO compared to the induced cerebral vasoconstriction. This agrees with previous findings by Furian et al. (2015). Another mechanism might be progression of hypoxaemia-related arterial blood vessel stiffness resulting in lower blood vessel reactivity in response to hypocapnia and hypoxaemia. This might have promoted less vasoconstriction and therefore, higher CTO values in response to better oxygenated arterial blood under hyperventilation. It remains to be elucidated whether the potential blood vessel stiffness was induced by aging (Kucharska-Newton et al., 2019) or hypoxaemia. Further, the elevated pulmonary artery pressure in highlanders may compromise venous drainage in the brain, causing increased cerebral blood volume, which may additionally interfere with the regulation of cerebral blood flow (Ainslie and Ogoh, 2010).
This longitudinal cohort study was carried out on Kyrgyz highlanders living between 2,500 and 3,500 m. Whether the cerebrovascular adaptations to protect brain metabolism are preserved in highlanders living at higher altitudes or with different ethnic backgrounds remains to be confirmed. Further, since chronic hypoxaemia might have induced vasodilatation of the cerebral blood vessels, changes in cHb (a surrogate for blood volume) might not represent the same degree of cerebral vasoconstriction in 2017 compared to 2012. Additional measurements to assess the cerebrovascular reactivity (i.e., cold pressor test) would have provided further insights into the physiology and adaptation of highlanders to chronic hypoxia. However, due to the paired comparisons, highlanders served as their own control group, so this potential bias was minimized. Due to the collinear change in age, mPAP and hypoxaemia, the likely principal mechanism underlying the observed cerebrovascular changes needs further study (Lovallo, 1975).
This first longitudinal cohort study on a large number of highlanders revealed decreased arterial oxygenation but preserved CTO after 5 years of living at high altitude. CTO was preserved in contempt of blunted cerebrovascular reactivity in response to hypocapnia and hyperoxia. These findings were observed in both, highlanders with and without initially diagnosed HAPH, despite unique mPAP progression in highlanders without initially diagnosed HAPH and preserved mPAP in highlanders with HAPH. These findings suggest that the altered cerebrovascular reactivity might be caused by progression in cerebral vessel stiffness due to chronic hypoxaemia or aging, or the combination of both. Whether the observed alterations in the cerebrovascular reactivity are beneficial, by maintaining CTO, or deleterious, remains to be elucidated.
Data availability statement
The original contributions presented in the study are included in the article/Supplementary Material, further inquiries can be directed to the corresponding author.
Ethics statement
The studies involving humans were approved by the Ethics committee of the National Centre of Cardiology and Internal Medicine, Bishkek, Kyrgyzstan. The studies were conducted in accordance with the local legislation and institutional requirements. The participants provided their written informed consent to participate in this study.
Author contributions
TS, SU, KB, and MF contributed to conception and design of the study; all authors contributed to acquisition, analysis, or interpretation of data for the work; MCL, KB, and MF drafted the final manuscript. All authors contributed to the article and approved the submitted version.
Funding
This work was supported by Swiss National Science Foundation (197706), the OPO Foundation and the Zurich-Lung-League.
Conflict of interest
The authors declare that the research was conducted in the absence of any commercial or financial relationships that could be construed as a potential conflict of interest.
Publisher’s note
All claims expressed in this article are solely those of the authors and do not necessarily represent those of their affiliated organizations, or those of the publisher, the editors and the reviewers. Any product that may be evaluated in this article, or claim that may be made by its manufacturer, is not guaranteed or endorsed by the publisher.
Abbreviations
Bf, Breathing frequency; cHb, Change in cerebral haemoglobin concentration; COPD, Chronic obstructive pulmonary disease; CTO, Cerebral tissue oxygenation; FiO2, Fractional of inspired oxygen; HAPH, High-altitude pulmonary hypertension; HR, Heart rate; mPAP, Mean pulmonary artery pressure; NIRS, Near-infrared spectroscopy; PaCO2, Arterial partial pressure of carbon dioxide; PaO2, Arterial partial pressure of oxygen; PetCO2, End-tidal partial pressure of carbon dioxide; SaO2, Arterial oxygen saturation; SpO2, Saturation of oxygen.
References
Ainslie P. N., Burgess K. R. (2008). Cardiorespiratory and cerebrovascular responses to hyperoxic and hypoxic rebreathing: effects of acclimatization to high altitude. Respir. physiology Neurobiol. 161 (2), 201–209. doi:10.1016/j.resp.2008.02.003
Ainslie P. N., Ogoh S. (2010). Regulation of cerebral blood flow in mammals during chronic hypoxia: A matter of balance. Exp. Physiol. 95 (2), 251–262. doi:10.1113/expphysiol.2008.045575
Al-Rawi P. G., Smielewski P., Kirkpatrick P. J. (2001). Evaluation of a near-infrared spectrometer (niro 300) for the detection of intracranial oxygenation changes in the adult head. Stroke 32 (11), 2492–2500. doi:10.1161/hs1101.098356
Arnau J., Bendayan R., Blanca M. J., Bono R. (2014). The effect of skewness and kurtosis on the kenward-roger approximation when group distributions differ. Psicothema 26 (2), 279–285. doi:10.7334/psicothema2013.174
Arnau J., Bendayan R., Blanca M. J., Bono R. (2013). The effect of skewness and kurtosis on the robustness of linear mixed models. Behav. Res. methods 45 (3), 873–879. doi:10.3758/s13428-012-0306-x
Furian M., Latshang T. D., Aeschbacher S. S., Ulrich S., Sooronbaev T., Mirrakhimov E. M., et al. (2015). Cerebral oxygenation in highlanders with and without high-altitude pulmonary hypertension. Exp. Physiol. 100 (8), 905–914. doi:10.1113/ep085200
Galie N., Humbert M., Vachiery J. L., Gibbs S., Lang I., Torbicki A., et al. (2016). 2015 esc/ers guidelines for the diagnosis and treatment of pulmonary hypertension: the joint task Force for the diagnosis and treatment of pulmonary hypertension of the European society of Cardiology (esc) and the European respiratory society (ers): endorsed by: association for European paediatric and congenital Cardiology (aepc), international society for heart and lung transplantation (ishlt). Eur. Heart J. 37 (1), 67–119. doi:10.1093/eurheartj/ehv317
Imray C. H. E., Barnett N. J., Walsh S., Clarke T., Morgan J., Hale D., et al. (1998). Near-infrared spectroscopy in the assessment of cerebral oxygenation at high altitude. Wilderness Environ. Med. 9 (4), 198–203. doi:10.1580/1080-6032(1998)009[0198:nisita]2.3.co;2
Kitabatake A., Inoue M., Asao M., Masuyama T., Tanouchi J., Morita T., et al. (1983). Noninvasive evaluation of pulmonary hypertension by a pulsed Doppler technique. Circulation 68 (2), 302–309. doi:10.1161/01.CIR.68.2.302
Kucharska-Newton A. M., Stoner L., Meyer M. L. (2019). Determinants of vascular age: an epidemiological perspective. Clin. Chem. 65 (1), 108–118. doi:10.1373/clinchem.2018.287623
Lassen N., Christensen M. (1976). Physiology of cerebral blood flow. Br. J. Anaesth. 48 (8), 719–734. doi:10.1093/bja/48.8.719
León-Velarde F., Maggiorini M., Reeves J. T., Aldashev A., Asmus I., Bernardi L., et al. (2005). Consensus statement on chronic and subacute high altitude diseases. High. Alt. Med. Biol. 6 (2), 147–157. doi:10.1089/ham.2005.6.147
Lovallo W. (1975). The cold pressor test and autonomic function: A review and integration. Psychophysiology 12 (3), 268–282. doi:10.1111/j.1469-8986.1975.tb01289.x
McLeod A. D., Igielman F., Elwell C., Cope M., Smith M. (2003). Measuring cerebral oxygenation during normobaric hyperoxia: A comparison of tissue microprobes, near-infrared spectroscopy, and jugular venous oximetry in head injury. Anesth. Analgesia 97, 851–856. doi:10.1213/01.ane.0000072541.57132.ba
Miller M. R., Hankinson J., Brusasco V., Burgos F., Casaburi R., Coates A., et al. (2005). Standardisation of spirometry. Eur. Respir. J. 26 (2), 319–338. doi:10.1183/09031936.05.00034805
Moore L. G., Niermeyer S., Zamudio S. (1998). Human adaptation to high altitude: regional and life-cycle perspectives. Am. J. Phys. Anthropol. 107 (27), 25–64. doi:10.1002/(sici)1096-8644(1998)107:27+<25:aid-ajpa3>3.0.co;2-l
Paulson O. B., Olesen J., Christensen M. S. (1972). Restoration of autoregulation of cerebral blood flow by hypocapnia. Neurology 22 (3), 286–293. doi:10.1212/wnl.22.3.286
Poulin M. J., Fatemian M., Tansley J. G., O'Connor D. F., Robbins P. A. (2002). Changes in cerebral blood flow during and after 48 H of both isocapnic and poikilocapnic hypoxia in humans. Exp. Physiol. 87 (5), 633–642. doi:10.1113/eph8702437
Schielzeth H., Dingemanse N. J., Nakagawa S., Westneat D. F., Allegue H., Teplitsky C., et al. (2020). Robustness of linear mixed-effects models to violations of distributional assumptions. Methods Ecol. Evol. 11 (9), 1141–1152. doi:10.1111/2041-210x.13434
Ulrich S., Nussbaumer-Ochsner Y., Vasic I., Hasler E., Latshang T. D., Kohler M., et al. (2014). Cerebral oxygenation in patients with osa: effects of hypoxia at altitude and impact of acetazolamide. Chest 146 (2), 299–308. doi:10.1378/chest.13-2967
Keywords: altitude, highlanders, near-infrared spectroscopy, cerebral oxygenation, hyperoxia, hypobaric hypoxia, hypocapnia
Citation: Luyken MC, Appenzeller P, Scheiwiller PM, Lichtblau M, Mademilov M, Muratbekova A, Sheraliev U, Abdraeva A, Marazhapov N, Sooronbaev TM, Ulrich S, Bloch KE and Furian M (2023) Time course of cerebral oxygenation and cerebrovascular reactivity in Kyrgyz highlanders. A five-year prospective cohort study. Front. Physiol. 14:1160050. doi: 10.3389/fphys.2023.1160050
Received: 06 February 2023; Accepted: 26 September 2023;
Published: 10 October 2023.
Edited by:
Siwang Wang, Air Force Medical University, ChinaReviewed by:
Kambiz Pourrezaei, Drexel University, United StatesPranav Prasoon, University of Pittsburgh, United States
Copyright © 2023 Luyken, Appenzeller, Scheiwiller, Lichtblau, Mademilov, Muratbekova, Sheraliev, Abdraeva, Marazhapov, Sooronbaev, Ulrich, Bloch and Furian. This is an open-access article distributed under the terms of the Creative Commons Attribution License (CC BY). The use, distribution or reproduction in other forums is permitted, provided the original author(s) and the copyright owner(s) are credited and that the original publication in this journal is cited, in accordance with accepted academic practice. No use, distribution or reproduction is permitted which does not comply with these terms.
*Correspondence: Michael Furian, bWljaGFlbC5mdXJpYW5AdXN6LmNo