- 1Key Laboratory of Aquaculture Nutrition and Feed (Ministry of Agriculture and Rural Affairs), Key Laboratory of Mariculture (Ministry of Education), Ocean University of China, Qingdao, China
- 2Laboratory for Marine Fisheries Science and Food Production Processes, Qingdao National Laboratory for Marine Science and Technology, Qingdao, China
A 30-day feeding trial was designed to evaluate the effect of supplemental fulvic acid (FA) on survival, growth performance, digestive ability and immunity of large yellow croaker (Larimichthys crocea) larvae (initial body weight 11.33 ± 0.57 mg). Four isonitrogenous and isolipids diets containing 0.00%, 0.01%, 0.02% and 0.04% FA were formulated, respectively. Results showed that the supplementation of 0.04% FA significantly improved survival rate of large yellow croaker larvae. Meanwhile, supplemental FA significantly increased final body weight and specific growth rate. Based on the specific growth rate, the optimal supplementation was 0.0135% FA. Larvae fed the diet with 0.01% FA had significantly higher villus height than the control. The supplementation of 0.01%–0.02% FA significantly increased the muscular thickness of intestine. Moreover, supplementation of FA significantly increased mRNA expression of intestinal epithelial proliferation and barrier genes (pcna, zo-1 and zo-2). Diets supplemented with 0.02%–0.04% FA significantly increased the activity of trypsin in the intestinal segment, while 0.01%–0.02% FA significantly increased the activity of trypsin in the pancreatic segment. Compared with the control, supplementation of FA remarkably increased activities of alkaline phosphatase and leucine aminopeptidase in the brush border membrane of intestine. Larvae fed the diet with 0.01% FA significantly increased activities of lysozyme and total nitric oxide synthase. Furthermore, the supplementation of 0.01% to 0.02% FA significantly decreased the mRNA expression of pro-inflammatory cytokines (tnf-α and il-6). Concurrently, supplemental FA significantly increased anti-inflammatory cytokine (il-10) mRNA expression level. In conclusion, this study indicated that the supplementation of FA could improve the survival rate and growth performance of larvae by promoting intestinal development, digestive enzymes activities and innate immunity.
1 Introduction
Production of larvae is a critical bottleneck restricting the development of marine fish farming (Zhang et al., 2023). Inadequate digestive system and low immunity are the chief causes of high mortality of larvae (Comabella et al., 2013; Rojo-Cebreros et al., 2018). Therefore, finding ecofriendly feed additives to promote the maturation of digestive function and the development of immune system in larval stage is a powerful way for aquacultural flourish.
Fulvic acid (FA) is an organic substance found in humus with the lowest molecular size (around 2000 Da) but the highest activity (Isam et al., 2005; Plaza et al., 2005). Due to the various functional groups of FA, it has beneficial functions such as anti-inflammatory, antibacterial, stimulating the absorption of microelements, and enhancing immunostimulatory properties (Van Rensburg, 2015; Hullár et al., 2018; Goenadi, 2021). Studies in mammals have shown that FA has potential to prevent or treat diseases, such as diabetes and neurodegenerative disorders (Winkler and Ghosh, 2018; Dominguez-Meijide et al., 2020). In livestock and poultry, FA has been shown to improve lipid metabolism, intestinal morphology and meat quality (Bai et al., 2013; Chang et al., 2014; Taklimi et al., 2012). Researches of FA in aquatic animals have made progress in recent years. Studies proved that dietary FA can promote growth and digestive ability in Carassius auratus (Wu et al., 2016), regulate intestinal microbiota of Paramisgurnus dabryanus (Gao et al., 2017), improve antioxidant capacity of Oreochromis niloticus (Neamatallah et al., 2022), and enhance non-specific immunity of Procambarus clarkia (Zhang, 2018). However, the effects of supplemental FA on intestinal development and immune function of marine fish larvae have not been reported.
Large yellow croaker (Larimichthys crocea) is one of the main economic fish in China (He et al., 2022). Lots of studies in nutrition requirements, digestive physiology and feed manufacturing technology of larvae have been reported (Ai et al., 2008; Zhao et al., 2013; Cai et al., 2017; Liu et al., 2022). However, there is no study on effects of FA on digestive system maturation and immunity of large yellow croaker larvae. Therefore, this experiment was conducted to investigate the effects of supplemental FA on survival, growth performance, digestive capacity and immunity of large yellow croaker larvae.
2 Materials and methods
2.1 Diets formulation
Four isonitrogenous (crude protein 50.4%) and isolipidic (crude lipid 17.2%) experimental diets supplemented with 0.00%, 0.01%, 0.02% and 0.04% FA (purity 85%, Shanghai Macklin Biochemical Co., Ltd), respectively (Table 1). Each experimental feed ingredient was crushed to 150 mesh, then FA was mixed with powdered ingredients in the order of addition from less to more. The remaining ingredients were added to an electric blender and stirred for 30 min until a uniform, viscous pellet was formed. The pellet was made into 1 mm micro-diets, and then oven-baked for about 8 h at 50°C. The dried feed was broken and filtrated to obtain two micro-diets with particle sizes of 280–450 μm and 450–600 μm. The dried feed was stored at −20°C for use in subsequent experiments. Diets of 280–450 μm were fed to larvae 17–32 days after hatching (DAH), and diets of 450–600 μm were fed afterwards.
2.2 Experimental procedures
The experiment was carried out at the Ningbo Marine and Fishery Science and Technology Innovation Base in Zhejiang Province. The experimental objects were artificially hatched large yellow croaker larvae from the same batch. Larvae were fed with rotifers (Brachionus plicatilis) from three to eight DAH. From 6 to 11 DAH, the larvae were fed with brine shrimp (Artemia nauplii). From 10 to 17 DAH, larvae were feed copepod (Calanus sinicus) and micro-diet, with the proportion of diet gradually increasing until 17 DAH, when the experimental diet was fed exclusively. In the experiment, larvae (mean body weight 11.33 ± 0.57 mg) were randomly assigned to 12 cylindrical white barrels (water volume 220 L), with a density of 3,000 individuals per barrel. Larvae were fed the experimental diet, and four treatment groups were set up with three replicates per treatment. During the rearing period (17–47 DAH), the experimental feed was fed to larvae seven times a day (06:00, 09:00, 12:00, 15:00, 18:00, 21:00, 24:00) until satiation. The rearing conditions are strictly controlled and water temperature, pH, and salinity were ranging from 23°C to 26°C, 7.8 to 8.2, 21‰–25‰, respectively. Approximately 150%–200% of the water was replaced daily.
2.3 Sample collection
At the beginning, fifty larvae of 17 DAH were randomly selected from each holding bucket, and initial body weight (IBW) and initial body length (IBL) were measured using an analytical balance and vernier caliper, respectively. At the end of the feeding trial, larvae were fasted for 24 h to empty their digestive tracts. Larvae were counted and survival rates (SR) were calculated for each barrel. Fifty larvae from each tank were randomly collected to measure final body weight (FBW) and final body length (FBL). Fifty larvae were randomly selected from each barrel and dissected under a dissecting microscope at 0°C to get the pancreatic and intestinal segments (PS and IS) for the detection of digestive enzyme activity (Cahu and Infante, 1994). Forty larvae were randomly selected from each barrel and dissected at 0°C to get visceral masses (VM) for analysis immune enzyme activities. Ninety larvae were randomly selected from each barrel and dissected under a dissecting microscope at 0°C in a sterile environment. The whole intestine and liver were separated and stored in RNase-free centrifuge tubes and immediately frozen in liquid nitrogen for real-time quantitative PCR analysis. Fifteen larvae from each treatment group were randomly selected and fixed in 4% paraformaldehyde for 24 h, then transferred to 75% alcohol for HE staining. After sampling, the remaining larvae were divided into 10 mL RNase-free centrifuge tubes and snap-frozen in liquid nitrogen for analysis of body composition.
2.4 Analytical methods
2.4.1 Proximate composition analysis
Diets and larvae were oven baked at 105°C for 72 h to constant weight to determine water content. Crude protein content of diets and fish was determined by the Kjeltec nitrogen method (FOSS Kjeltec 8,400 Analyzer Uni, Sweden) and estimated by multiplying nitrogen by 6.25. Crude lipid content of diets and fish was examined by the Soxhlet extraction method (Soxhlet Extraction System B-801, Buchi 36,680, Switzerland).
2.4.2 Intestinal morphology analysis
Intestinal sections were prepared according to the methods described in the published paper (Mai et al., 2005). In brief, larvae intestinal segments were dehydrated in a gradient, embedded in paraffin, sectioned, and immediately stained with hematoxylin and eosin solutions. Sections were observed under a light microscope (Leica DM3000 LED, Germany), and professional image analysis software (Image Pro Plus 6.0, United States) was used to measure the villus height, enterocyte cell height and muscular thickness.
2.4.3 Digestive enzyme activities assay
The PS and IS samples were mixed and homogenized at a 1:9 ratio, centrifuged at 3,500 g for 15 min, and the supernatant was used for index determination. Purified brush border membranes (BBM) were obtained from homogenates of IS according to the method described by Crane et al. (1979). LAP activity was determined according to the method of Maroux et al. (1973). Trypsin, α-amylase, lipase and AKP activities were measured using commercial kits (Nanjing Jiancheng Bio-Engineering Institute, China). Total protein quantification (TP) was determined using the Beyotime BCA kit (P0011). All experimental procedures were performed in strict accordance with the instructions.
2.4.4 Immune enzymes activities assay
The VM sample were mixed and homogenized at a 1:9 ratio, centrifuged at 3,500 g for 15 min, and the supernatant was used for index determination. LZM, TNOS and iNOS activities were measured using commercial kits (Nanjing Jiancheng Bio-Engineering Institute, China).
2.4.5 RNA extraction and real-time quantitative PCR
Total RNA was extracted from the intestine or liver of larvae using RNAiso Plus (Takara Biotech, Japan). cDNA was reverse transcribed from the RNA using the Prime Script-RT kit (Takara, Japan). The primer sequences were designed and synthesized based on the corresponding sequences in published papers (Table 2). Real-time quantitative PCR was performed by referring to Zhang et al. (2023).
2.5 Calculations and statistical analysis
The following calculations were performed:
Where Nt and N0 were the final and initial number of larvae, respectively; Wt and W0 were the final and initial body weights, respectively; d was the experimental period in days.
Statistical analysis was performed in SPSS 26.0 (SPSS Inc., United States). The data were analyzed by the one-way analysis of variance (ANOVA) followed by Tukey’s multiple-range test. Statistically significant differences were determined using p < 0.05. Results were presented as mean ± S.E.M. (Standard error of means).
3 Results
3.1 Survival, growth performance and body composition
After 30 days, the supplementation of FA significantly improved the survival rate and growth performance of larvae (Table 3) (p < 0.05). When the FA supplementation reached 0.04%, the survival rate significantly increased from 20.64% to 23.48% (p < 0.05). Supplementation of 0.01%–0.04% FA increased FBL, FBW and SGR of larvae significantly (p < 0.05). The broken-line analysis for SGR indicated that the maximum growth of larvae appeared in the supplementation of 0.0135% FA (Figure 1). There were no significant differences in moisture, crude lipid and crude protein contents among supplementary treatments (p > 0.05) (Table 4).
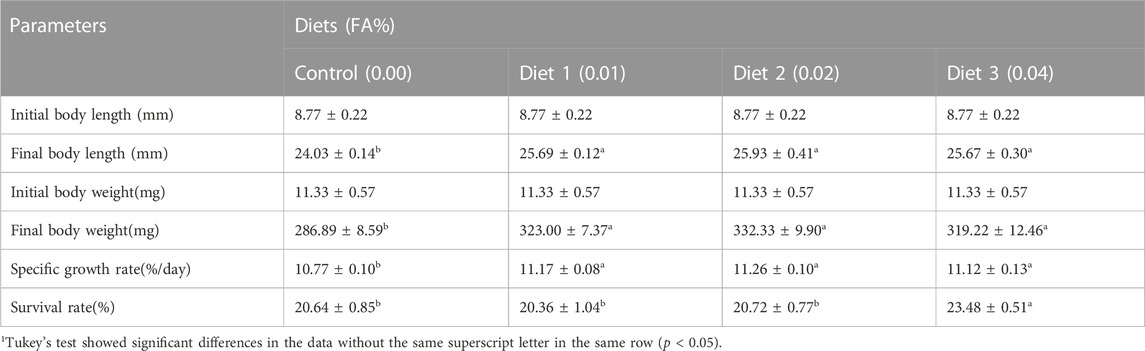
TABLE 3. Effects of supplemental fulvic acid on survival rate and growth performance of large yellow croaker larvae (Means ± S.E.M., n = 3)1
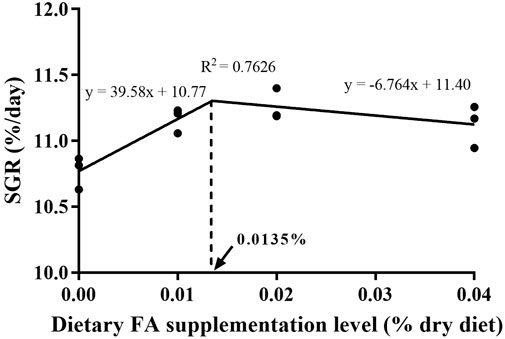
FIGURE 1. Relationship of specific growth rate (SGR, %/d) with supplemental FA levels of large yellow croaker larvae.
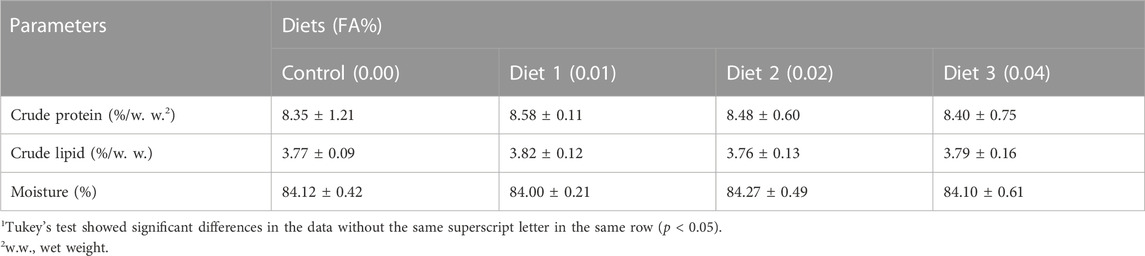
TABLE 4. Effects of supplemental fulvic acid on body composition of large yellow croaker larvae (Means ± S.E.M., n = 3)1.
3.2 Intestinal morphology
The supplementation of FA could improve the intestinal morphology of larvae (Figure 2). With an increase in FA supplementation, the VH, MT and EH increased firstly and then decreased. VH was significantly increased when FA supplementation was 0.01%. Compared with the control, the difference of MT was statistically significant when the supplemental level was 0.01%–0.02% (p < 0.05) (Table 5). However, no significant change of the EH was observed in the intestinal tract of larvae (p > 0.05) (Table 5).
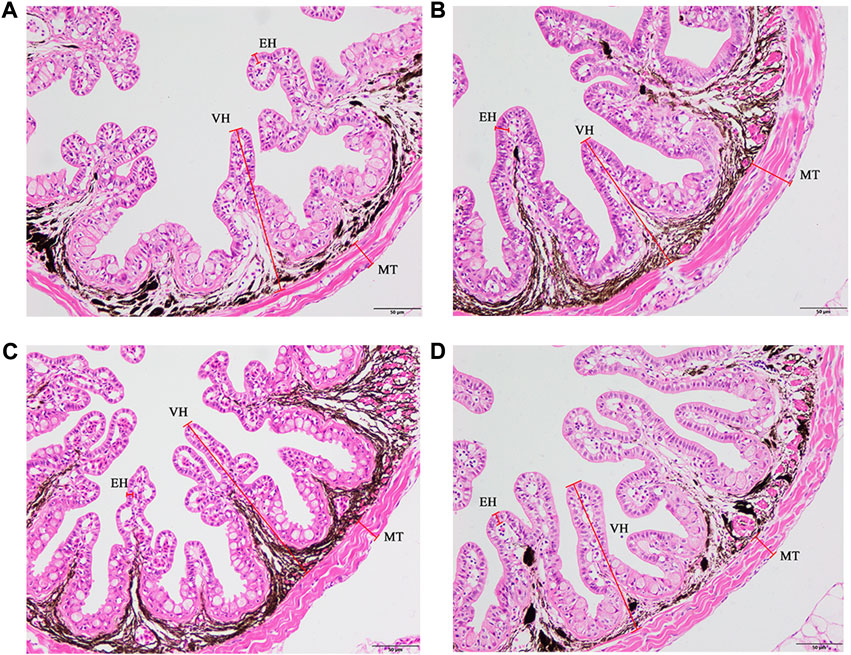
FIGURE 2. Effects of supplemental fulvic acid on intestinal morphology of large yellow croaker larvae. Control group (0.00%) (A); 0.01% FA group (B); 0.02% FA group (C); 0.04% FA group (D). MT, muscular thickness; VH, villus height; EH, enterocyte height (HE staining; Scale bar = 50 μm).
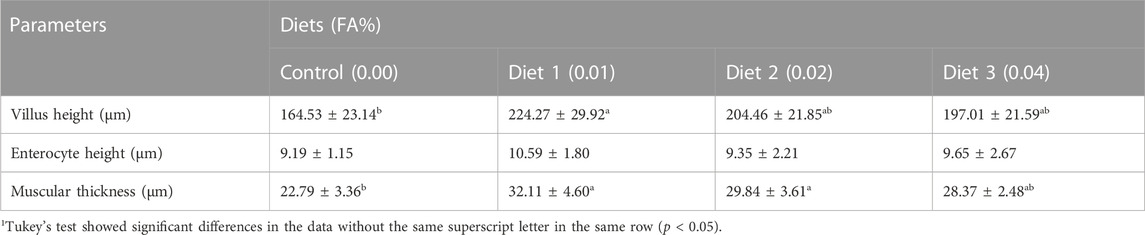
TABLE 5. Effects of supplemental fulvic acid on micromorphology of the intestine of large yellow croaker larvae (Means ± S.E.M., n = 3)1.
3.3 Expression of proliferation-related and barrier-related genes in intestinal cell
Compared to the control, supplementation of 0.02%–0.04% FA had notably higher mRNA expression of zo-1 (p < 0.05) (Figure 3). The zo-2 mRNA expression of larvae with 0.01%–0.02% FA supplementation was significantly higher than the control (p < 0.05) (Figure 3). Meanwhile, supplementation of 0.01% FA significantly increased the pcna mRNA expression (p < 0.05) (Figure 3). However, supplemental FA had no significant effect on the mRNA expression of occludin and odc (p > 0.05) (Figure 3).
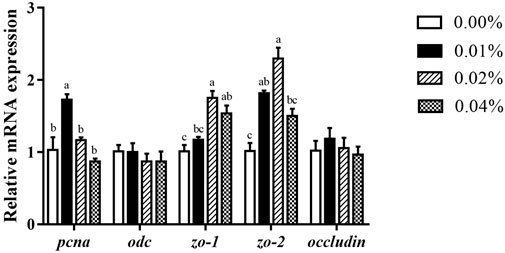
FIGURE 3. Effects of supplemental fulvic acid on intestinal cell proliferation-related and barrier-related genes mRNA expression in intestine of large yellow croaker larvae (mean ± S.E.M, n = 3). Pcna, proliferating cell nuclear antigen; odc, ornithine decarboxylase; zo-1, zonula occludens-1; zo-2, zonula occludens-2. Bars with different letters showed a significant difference (p < 0.05, Tukey’s test).
3.4 Digestive enzyme activity
The supplementation of FA increased the activity of digestive enzyme (Table 6). Supplemental FA increased activities of trypsin in IS and PS significantly (p < 0.05). However, supplemental FA did not affect try-IS/(PS + IS) ratio of larvae significantly (p > 0.05). Meanwhile, compared with the control, activities of amylase and lipase were increased but the differences among treatments were not significant (p > 0.05).
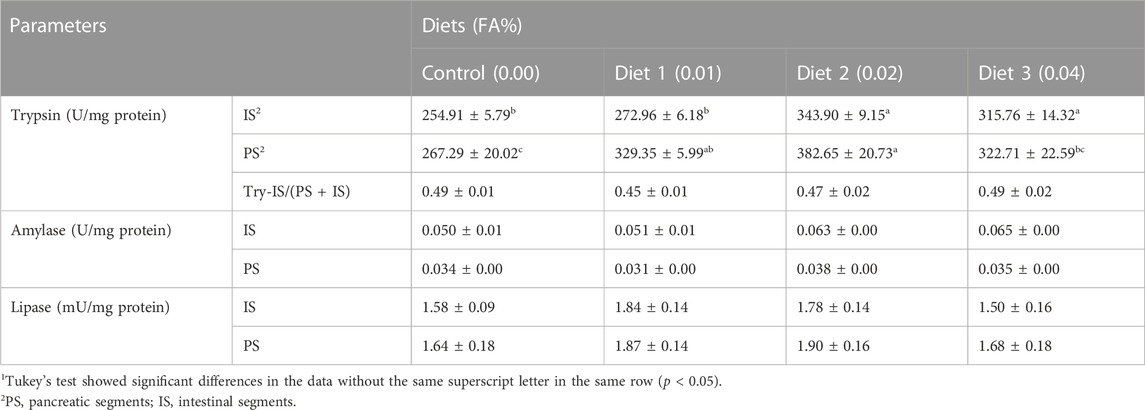
TABLE 6. Effects of supplemental fulvic acid on activities of digestive enzymes of large yellow croaker larvae (Means ± S.E.M, n = 3)1.
The results suggested that activities of AKP and LAP were significantly higher when supplemented with 0.01%–0.04% FA than in the control (p < 0.05) (Table 7). With an increase in FA supplemental level, AKP and LAP activities initially increased and then decreased, with the highest activity observed when the supplemental amount was 0.01%.

TABLE 7. Effects of supplemental fulvic acid on activities of AKP and LAP in BBM2 of large yellow croaker larvae (Means ± S.E.M, n = 3)1.
3.5 Non-specific immune-related enzyme activity
Supplementation of 0.01% FA significantly increased the enzyme activities of LZM and TNOS (p < 0.05) (Figure 4A, B). The supplemental FA increased iNOS activity, but the change was not statistically significant (p > 0.05) (Figure 4C).
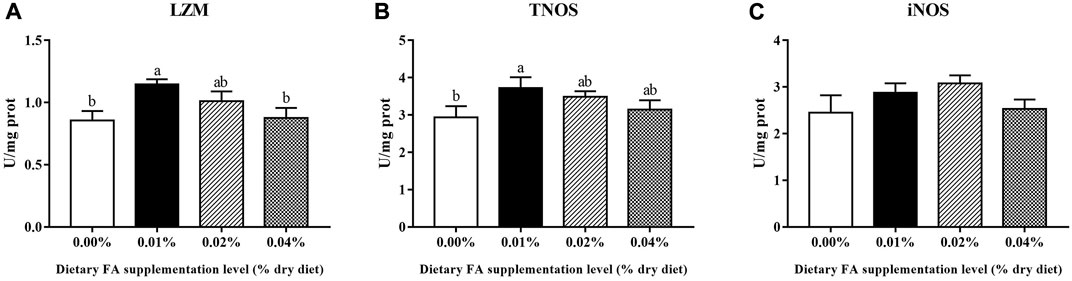
FIGURE 4. Effects of supplemental fulvic acid on LZM (A), TNOS (B) and iNOS (C) activities in the visceral mass of large yellow croaker larvae (mean ± S.E.M, n = 3). LZM, lysozyme; TNOS, total nitric oxide synthase; iNOS, inducible nitric oxide synthase. Bars with different letters showed a significant difference (p < 0.05, Tukey’s test).
3.6 Expression of inflammation cytokines genes
With an increase in FA supplementation, the mRNA expression of pro-inflammation cytokines tnf-α, and il-6 decreased initially, then increased (Figure 5). When FA supplementation achieved 0.04%, no significant difference was found in the mRNA expression of tnf-α (p < 0.05). Supplementation of 0.02% FA significantly decreased the mRNA expression of il-6 (p < 0.05). Simultaneously, the supplementation of FA significantly increased the mRNA expression of il-10 (p < 0.05) (Figure 5). There was no significant difference in the mRNA expression of ifn-γ and il-1β among all groups (p > 0.05) (Figure 5).
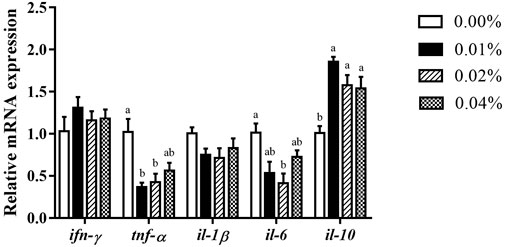
FIGURE 5. Effects of supplemental fulvic acid on genes related to inflammation mRNA expression in liver of large yellow croaker larvae (mean ± S.E.M, n = 3). Ifn-γ, interferon γ; tnf-α, tumor necrosis factor α; il-1β, interleukin-1β; il-6, interleukin-6; il-10, interleukin-10. Bars with different letters showed a significant difference (p < 0.05, Tukey’s test).
4 Discussion
FA is one of innocuous and eco-friendly organic substances, which has been proved to effectively reduce feed conversion rate and promote the growth of livestock and aquatic animals as a feed additive (Bai et al., 2013; Gao et al., 2018; Mao, 2019). In this study, the supplementation of FA could increase the survival and growth of larvae. The similar results have also been found in Nile tilapia (Jusadi et al., 2020) and Pacific white shrimp (Gao et al., 2018). These positive effects are related to the ability of FA to improve digestive capacity and immunity with its multiple active functional groups.
Intestinal development of marine fish larvae is important for improving digestive capacity (Pérez-Sirkin et al., 2020). In the present study, the intestinal VH and MT of larvae were significantly increased, indicating a more developed intestinal structure than that of the control group. Intestinal maturation is related to the proliferation and barrier function of epithelial cells (Liu et al., 2020). The mRNA expression of epithelial cell proliferation related gene (pcna) was significantly upregulated by supplementation of 0.01% FA. The permeability of tight junctions plays a crucial role in regulating the barrier function of the intestinal epithelium. This barrier allows for the absorption of nutrients and water while effectively blocking the entry of pathogens (Turner, 2006; Elsabagh et al., 2018; Li et al., 2021). In the present study, we found that the mRNA expression of intestinal zo-1 and zo-2 was significantly increased in the treatment group. Gildea et al. (2017) found that 20% lignite extract (the main raw material for FA extraction) was able to alleviate damage to the tight junction protein ZO-1 caused by glyphosate. Consequently, supplemental FA could improve intestinal maturation of larvae by ameliorating intestinal morphology, stimulating intestinal epithelial cell proliferation and maintaining intestinal barrier function.
Digestive enzyme activities are widely used as the marker to assess the digestive capacity of larvae (Yao et al., 2020; Yin et al., 2022). The relatively low activity of protease enzymes is thought to be one of the causes of the poor growth of larvae fed on a micro-diets (Dabrowski, 1984; Segner et al., 1989; Kolkovski, 2001). The present study showed that supplemental FA significantly increased the trypsin activities of IS and PS, which was consistent with the results in P. clarkia (Zhang, 2018), P. dabryanus (Gao et al., 2017) and C. auratus (Wu et al., 2016). The increased and stable activity of intestinal BBM enzymes such as aminopeptidase and AKP indicates that the gut may fully mature and achieve full function at this stage of development (Kolkovski et al., 2009; Ma et al., 2005; Lallès, 2020). The present study found that supplemental FA could significantly improve AKP and LAP activities in the intestinal BBM, which means that FA promote the intestine maturation of larvae (Liu et al., 2020; Xu et al., 2022). Thus, larvae fed diets with FA improved digestive enzyme activity and promoted intestinal development, facilitating adequate digestion and absorption of nutrients in the feed sufficiently.
The innate immune system plays a vital role in the immune defense of fish. Effective prophylactic measures to enhance the innate immune system prior to infection are of special value in larviculture (Magnadottir, 2010; Rojo-Cebreros et al., 2018). Oral immunostimulants can increase stress resistance and stimulate potential killing activity of macrophages to defend themselves against pathogens (Geng et al., 2011). Our research found that supplemental FA effectively improved activities of non-specific immune enzymes. LZM is involved in an extensive range of defense mechanisms and can act on the peptidoglycan layer of bacterial cell walls leading to bacterial lysis (Saurabh and Sahoo, 2008). Therefore, the increased activity of LZM indicates an improved protection against bacterial invasion. In vertebrates, Nitric oxide (NO) produced by immune cells is synthesized and released for over time by iNOS, which has unique flexibility in responding to disease (Huang et al., 2020; Pederzoli and Mola, 2016). In RAW246.7 cells, FA regulate iNOS production and stimulate NO production by activating the NF-κB pathway (Jayasooriya et al., 2016). In our study, the increased activity of iNOS indicated that FA had an immunostimulatory effect on larvae. Moreover, FA acts as an immunomodulator and has anti-inflammation effect (Sherry et al., 2013; Van Rensburg et al., 2001). Cytokines, as signaling molecules for cellular communication, are key participants in the innate immune response (Magnadottir, 2010). In the present study, FA stimulation led to upregulation of anti-inflammatory cytokine il-10 and downregulation of pro-inflammatory cytokines tnf-α and il-6. Therefore, our research suggested that supplemental FA can enhance innate immunity of larvae.
In conclusion, the present study showed that the supplementation of FA can improve the survival rate and growth performance of large yellow croaker larvae by improving intestinal morphology, promoting intestinal barrier function, increasing digestive enzymes activities, and enhancing non-specific immunity. Based on the specific growth rate, the supplementation of 0.0135% FA was optimal for the growth performance of large yellow croaker larvae under the current experimental conditions.
Data availability statement
The original contributions presented in the study are included in the article/supplementary material, further inquiries can be directed to the corresponding author.
Ethics statement
The animal study was reviewed and approved by this study was executed in strict compliance with the Chinese Order NO. 676 of the State Council, revised 1 March 2017.
Author contributions
CZ designed the research. CZ, JZ, YW, and JL conducted the research. CZ, YL, and JZ analyzed the data. CZ wrote the manuscript. YL, CY, and YH reviewed manuscript. KM and QA instructed the research. All authors reviewed and approved the submitted manuscript.
Funding
This research is financially supported by the earmarked fund for CARS-47 and Ten-thousand Talents Program (grant number: 2018-29).
Acknowledgments
We thank Zhen Wang, Jichang Zheng, Yuliang He, Zhijie Shi, Xiuneng Wang, Fan Chen, Manxi Zhao and Guobin Liu for their help during the experiment.
Conflict of interest
The authors declare that the research was conducted in the absence of any commercial or financial relationships that could be construed as a potential conflict of interest.
Publisher’s note
All claims expressed in this article are solely those of the authors and do not necessarily represent those of their affiliated organizations, or those of the publisher, the editors and the reviewers. Any product that may be evaluated in this article, or claim that may be made by its manufacturer, is not guaranteed or endorsed by the publisher.
References
Ai, Q., Zhao, J., Mai, K., Xu, W., Tan, B., Ma, H., et al. (2008). Optimal dietary lipid level for large yellow croaker (Pseudosciaena crocea) larvae. Aquac. Nutr. 14, 515–522. doi:10.1111/j.1365-2095.2007.00557.x
Bai, H., Chang, Q., Shi, B., and Shan, A. (2013). Effects of fulvic acid on growth performance and meat quality in growing-finishing pigs. Livest. Sci. 158, 118–123. doi:10.1016/j.livsci.2013.10.013
Cahu, C. L., and Infante, J. L. Z. (1994). Early weaning of sea bass (Dicentrarchus labrax) larvae with a compound diet: Effect on digestive enzymes. Cony. Eiochent. Plty. 109, 213–222. doi:10.1016/0300-9629(94)90123-6
Cai, Z., Xie, F. J., Mai, K., and Ai, Q. (2017). Molecular cloning and genetic ontogeny of some key lipolytic enzymes in large yellow croaker larvae (Larimichthys crocea R.). Aquac. Res. 48, 1183–1193. doi:10.1111/are.12960
Chang, Q., Lu, Z., He, M., Gao, R., Bai, H., Shi, B., et al. (2014). Effects of dietary supplementation of fulvic acid on lipid metabolism of finishing pigs. J. Animal Sci. 92, 4921–4926. doi:10.2527/jas.2014-8137
Comabella, Y., Hernández Franyutti, A., Hurtado, A., Canabal, J., and García-Galano, T. (2013). Ontogenetic development of the digestive tract in Cuban gar (Atractosteus tristoechus) larvae. Rev. Fish. Biol. Fish. 23, 245–260. doi:10.1007/s11160-012-9289-z
Crane, R. K., Boge, G., and Rigal, A. (1979). Isolation of brush border membranes in vesicular form from the intestinal spiral valve of the small dogfish (Scyliorhinus canicula). Biochimica Biophysica Acta 554, 264–267. doi:10.1016/0005-2736(79)90024-5
Dabrowski, K. (1984). The feeding of fish larvae: Present « state of the art » and perspectives. Reprod. Nutr. Dévelop. 24, 807–833. doi:10.1051/rnd:19840701
Dominguez-Meijide, A., Vasili, E., König, A., Cima-Omori, M.-S., Ibáñez de Opakua, A., Leonov, A., et al. (2020). Effects of pharmacological modulators of α-synuclein and tau aggregation and internalization. Sci. Rep. 10, 12827. doi:10.1038/s41598-020-69744-y
Elsabagh, M., Mohamed, R., Moustafa, E. M., Hamza, A., Farrag, F., Decamp, O., et al. (2018). Assessing the impact of Bacillus strains mixture probiotic on water quality, growth performance, blood profile and intestinal morphology of Nile tilapia, Oreochromis niloticus. Aquac. Nutr. 24, 1613–1622. doi:10.1111/anu.12797
Gao, Y., He, J., He, Z., Li, Z., Zhao, B., Mu, Y., et al. (2017). Effects of fulvic acid on growth performance and intestinal health of juvenile loach Paramisgurnus dabryanus (Sauvage). Fish Shellfish Immunol. 62, 47–56. doi:10.1016/j.fsi.2017.01.008
Gao, Y., Zhu, J., Bao, H., Hector, V., Zhao, B., and Chu, Z. (2018). Effect of lignite fulvic acid on growth, antioxidant ability, and HSP70 of Pacific white shrimp, Litopenaeus vannamei. Aquacult Int. 26, 1519–1530. doi:10.1007/s10499-018-0302-y
Geng, X., Dong, X., Tan, B., Yang, Q., Chi, S., Liu, H., et al. (2011). Effects of dietary chitosan and Bacillus subtilis on the growth performance, non-specific immunity and disease resistance of cobia, Rachycentron canadum. Fish Shellfish Immunol. 31, 400–406. doi:10.1016/j.fsi.2011.06.006
Gildea, J. J., Roberts, D. A., and Bush, Z. (2017). Protective effects of lignite extract supplement on intestinal barrier function in glyphosate-mediated tight junction injury. J. Clin. Nutr. Diet. 03, 1. doi:10.4172/2472-1921.100035
Goenadi, D. H. (2021). Fulvic acid – A small but powerful natural substance. MP 89, 73–90. doi:10.22302/iribb.jur.mp.v89i1.424
He, Y., Tang, Y., Xu, N., Yao, C., Gong, Y., Yin, Z., et al. (2022). Effects of supplemental phytosterol on growth performance, body composition, serum biochemical indexes and lipid metabolism of juvenile large yellow croaker (Larimichthys crocea) fed with high lipid diet. Aquaculture 551, 737889. doi:10.1016/j.aquaculture.2022.737889
Huang, W., Yao, C., Liu, Y., Xu, N., Yin, Z., and Xu, W. (2020). Dietary Allicin Improved the Survival and Growth of Large Yellow Croaker (Larimichthys crocea) Larvae via Promoting Intestinal Development, Alleviating Inflammation and Enhancing Appetite. Frontiers in Physiology 11, 587674. doi:10.3389/fphys.2020.587674
Hullár, I., Vucskits, A. V., Berta, E., Andrásofszky, E., Bersényi, A., and Szabó, J. (2018). Effect of fulvic and humic acids on copper and zinc homeostasis in rats. Acta Veterinaria Hung. 66, 40–51. doi:10.1556/004.2018.005
Isam, K. M. S., Gropp, J. M., and Schuhmacher, A. (2005). Humic acid substances in animal agriculture. Pak. J. Nutr. 4, 126–134. doi:10.3923/pjn.2005.126.134
Jayasooriya, R. G. P. T., Dilshara, M. G., Kang, C.-H., Lee, S., Choi, Y. H., Jeong, Y. K., et al. (2016). Fulvic acid promotes extracellular anti-cancer mediators from RAW 264.7 cells, causing to cancer cell death in vitro. Int. Immunopharmacol. 36, 241–248. doi:10.1016/j.intimp.2016.04.029
Jusadi, D., Aprilia, T., Setiawati, M., Agus Suprayudi, M., and Ekasari, J. (2020). Dietary supplementation of fulvic acid for growth improvement and prevention of heavy metal accumulation in Nile tilapia fed with green mussel. Egypt. J. Aquatic Res. 46, 295–301. doi:10.1016/j.ejar.2020.04.002
Kolkovski, S. (2001). Digestive enzymes in fish larvae and juveniles—Implications and applications to formulated diets. Aquaculture 200, 181–201. doi:10.1016/S0044-8486(01)00700-1
Kolkovski, S., Lazo, J., Leclercq, D., and Izquierdo, M. (2009). “11 - fish larvae nutrition and diet: New developments,” in New Technologies in aquaculture woodhead publishing series in food science, technology and nutrition. Editors G. Burnell, and G. Allan (United Kingdom: Woodhead Publishing).
Lallès, J.-P. (2020). Influence of different levels of humic acid and esterified glucomannan on growth performance and intestinal morphology of broiler chickens. Reviews in Aquaculture 12, 555–581. doi:10.1111/raq.12340
Li, W., Xu, B., Wang, L., Sun, Q., Deng, W., Wei, F., et al. (2021). Effects of Clostridium butyricum on growth performance, gut microbiota and intestinal barrier function of broilers. Front. Microbiol. 12, 777456. doi:10.3389/fmicb.2021.777456
Li, X., Chen, Q., Chen, Q., Mai, K., and Ai, Q. (2020). Effects of dietary terrestrial oils supplemented with L-carnitine on growth, antioxidant capacity, lipid metabolism and inflammation in large yellow croaker (Larimichthys crocea). Br. J. Nutr. 125, 732–742. doi:10.1017/S0007114520003244
Liu, J., Xu, W., Liu, Y., Wang, Y., Zhang, J., Wang, Z., et al. (2022). Effects of chitosan-coated microdiet on dietary physical properties, growth performance, digestive enzyme activities, antioxidant capacity, and inflammation response of large yellow croaker (Larimichthys crocea) larvae. Aquac. Nutr. 2022, 4355182. doi:10.1155/2022/4355182
Liu, Y., Miao, Y., Xu, N., Ding, T., Cui, K., Chen, Q., et al. (2020). Effects of dietary Astragalus polysaccharides (APS) on survival, growth performance, activities of digestive enzyme, antioxidant responses and intestinal development of large yellow croaker (Larimichthys crocea) larvae. Aquaculture 517, 734752. doi:10.1016/j.aquaculture.2019.734752
Ma, H., Cahu, C., Zambonino, J., Yu, H., Duan, Q., Le Gall, M.-M., et al. (2005). Activities of selected digestive enzymes during larval development of large yellow croaker (Pseudosciaena crocea). Aquaculture 245, 239–248. doi:10.1016/j.aquaculture.2004.11.032
Magnadottir, B. (2010). Immunological control of fish diseases. Mar. Biotechnol. 12, 361–379. doi:10.1007/s10126-010-9279-x
Mai, K., Yu, H., Ma, H., Duan, Q., Gisbert, E., Infante, J. L. Z., et al. (2005). A histological study on the development of the digestive system of Pseudosciaena crocea larvae and juveniles. J. Fish. Biol. 67, 1094–1106. doi:10.1111/j.0022-1112.2005.00812.x
Mao, Y. (2019). Modulation of the growth performance, meat composition, oxidative status, and immunity of broilers by dietary fulvic acids. Poult. Sci. 98, 4509–4513. doi:10.3382/ps/pez281
Maroux, S., Louvard, D., and Barath, J. (1973). The aminopeptidase from hog intestinal brush border. Biochimica Biophysica Acta (BBA) - Enzym. 321, 282–295. doi:10.1016/0005-2744(73)90083-1
Neamatallah, W. A., Sadek, K. M., El-Sayed, Y. S., Saleh, E. A., and Khafaga, A. F. (2022). 2, 3-Dimethylsuccinic acid and fulvic acid attenuate lead-induced oxidative misbalance in brain tissues of Nile tilapia Oreochromis niloticus. Environ. Sci. Pollut. Res. 29, 21998–22011. doi:10.1007/s11356-021-16359-z
Pederzoli, A., and Mola, L. (2016). The early stress responses in fish larvae. Acta Histochem. 118, 443–449. doi:10.1016/j.acthis.2016.03.001
Pérez-Sirkin, D. I., Solovyev, M., Delgadin, T. H., Herdman, J. E., Miranda, L. A., Somoza, G. M., et al. (2020). Digestive enzyme activities during pejerrey (Odontesthes bonariensis) ontogeny. Aquaculture 524, 735151. doi:10.1016/j.aquaculture.2020.735151
Plaza, C., García-Gil, J. C., Polo, A., Senesi, N., and Brunetti, G. (2005). Proton binding by humic and fulvic acids from pig slurry and amended soils. J. Environ. Qual. 34, 1131–1137. doi:10.2134/jeq2004.0378
Rojo-Cebreros, A. H., Ibarra-Castro, L., and Martínez-Brown, J. M. (2018). Immunostimulation and trained immunity in marine fish larvae. Fish Shellfish Immunol. 80, 15–21. doi:10.1016/j.fsi.2018.05.044
Saurabh, S., and Sahoo, P. K. (2008). Lysozyme: An important defence molecule of fish innate immune system. Aquac. Res. 39, 223–239. doi:10.1111/j.1365-2109.2007.01883.x
Segner, H., Rösch, R., Schmidt, H., and von Poeppinghausen, K. J. (1989). Digestive enzymes in larval Coregonus lavaretus L. J. Fish Biol. 35, 249–263. doi:10.1111/j.1095-8649.1989.tb02974.x
Sherry, L., Millhouse, E., Lappin, D. F., Murray, C., Culshaw, S., and Nile, C. J. (2013). Investigating the biological properties of carbohydrate derived fulvic acid (CHD-FA) as a potential novel therapy for the management of oral biofilm infections. BMC Oral Health 13, 47. doi:10.1186/1472-6831-13-47
Taklimi, S. M. S. M., Ghahri, H., and Isakan, M. A. (2012). Influence of different levels of humic acid and esterified glucomannan on growth performance and intestinal morphology of broiler chickens. Agricultural Sciences 3, 663–668. doi:10.4236/as.2012.35080
Turner, J. R. (2006). Molecular basis of epithelial barrier regulation: From basic mechanisms to clinical application. Am. J. Pathology 169, 1901–1909. doi:10.2353/ajpath.2006.060681
Van Rensburg, C. E. J. (2015). The anti-inflammatory properties of humic substances: A mini review. Phytother. Res. 29, 791–795. doi:10.1002/ptr.5319
Van Rensburg, C. E. J., Malfeld, S. C. K., and Dekker, J. (2001). Topical application of oxifulvic acid suppresses the cutaneous immune response in mice. Drug Dev. Res. 53, 29–32. doi:10.1002/ddr.1166
Wang, Y., Xu, W., Zhang, J., Liu, J., Wang, Z., Liu, Y., et al. (2022). Effects of glycyrrhizin (GL) supplementation on survival, growth performance, expression of feeding-related genes, activities of digestive enzymes, antioxidant capacity, and expression of inflammatory factors in large yellow croaker (Larimichthys crocea) larvae. Aquac. Nutr. 2022, 5508120. doi:10.1155/2022/5508120
Winkler, J., and Ghosh, S. (2018). Therapeutic potential of fulvic acid in chronic inflammatory diseases and diabetes. J. Diabetes Res. 2018, 5391014. doi:10.1155/2018/5391014
Wu, L., Qi, J., Luo, X., and An, X. (2016). “Effect of dietary fulvic acid on growth and digestive enzyme activities of crucian crap (Carassius auratus),”. China Feed 32–34.
Xu, W., Liu, Y., Huang, W., Yao, C., Yin, Z., Mai, K., et al. (2022). Effects of dietary supplementation of astaxanthin (Ast) on growth performance, activities of digestive enzymes, antioxidant capacity and lipid metabolism of large yellow croaker (Larimichthys crocea) larvae. Aquac. Res. 53, 4605–4615. doi:10.1111/are.15933
Yao, C., Huang, W., Liu, Y., Yin, Z., Xu, N., He, Y., et al. (2020). Effects of dietary silymarin (SM) supplementation on growth performance, digestive enzyme activities, antioxidant capacity and lipid metabolism gene expression in large yellow croaker (Larimichthys crocea) larvae. Aquac. Nutr. 26, 2225–2234. doi:10.1111/anu.13159
Yin, Z., Gong, Y., Liu, Y., He, Y., Yao, C., Huang, W., et al. (2022). Fucoidan improves growth, digestive tract maturation, and gut microbiota in large yellow croaker (Larimichthys crocea) larvae. Nutrients 14, 4504. doi:10.3390/nu14214504
Zhang, J. (2018). Modulation of growth performance and nonspecific immunity of red swamp crayfish Procambarus clarkia upon dietary fulvic acid supplementation. Fish Shellfish Immunol. 83, 158–161. doi:10.1016/j.fsi.2018.09.012
Zhang, J., Wang, Y., Liu, J., Xu, W., Yin, Z., Liu, Y., et al. (2023). Effects of fecal bacteria on growth, digestive capacity, antioxidant capacity, intestinal health of large yellow croaker (Larimichthys crocea) larvae. Aquaculture 562, 738796. doi:10.1016/j.aquaculture.2022.738796
Keywords: fulvic acid, large yellow croaker, larval nutrition, intestinal morphology, immune response
Citation: Zhang C, Liu Y, Yao C, Zhang J, Wang Y, Liu J, Hong Y, Mai K and Ai Q (2023) Effects of supplemental fulvic acid on survival, growth performance, digestive ability and immunity of large yellow croaker (Larimichthys crocea) larvae. Front. Physiol. 14:1159320. doi: 10.3389/fphys.2023.1159320
Received: 05 February 2023; Accepted: 09 March 2023;
Published: 23 March 2023.
Edited by:
Gang Yang, Nanchang University, ChinaReviewed by:
Yan Liu, Huzhou University, ChinaMd. Sakhawat Hossain, Sylhet Agricultural University, Bangladesh
Copyright © 2023 Zhang, Liu, Yao, Zhang, Wang, Liu, Hong, Mai and Ai. This is an open-access article distributed under the terms of the Creative Commons Attribution License (CC BY). The use, distribution or reproduction in other forums is permitted, provided the original author(s) and the copyright owner(s) are credited and that the original publication in this journal is cited, in accordance with accepted academic practice. No use, distribution or reproduction is permitted which does not comply with these terms.
*Correspondence: Qinghui Ai, cWhhaUBvdWMuZWR1LmNu