- 1Underwater research team—ERRSO, Military biomedical research institute-IRBA, Toulon, France
- 2LAMHESS (UPR 6312), Université de Nice, Nice, France
- 3Department of underwater and hyperbaric medicine (SMHEP), Ste Anne military hospital (HIA Ste Anne), Toulon, France
- 4French Navy Diving school, St Mandrier, France
- 5Military Diver Unit, Brest, France
- 6Université Jean Monnet, Ste Etienne, France
Introduction: During military fin swimming, we suspected that oxygen uptake (
Methods: Eighteen male military divers (32.3 ± 4.2 years; 178.0 ± 5.0 cm; 76.4 ± 3.4 kg; 24.1 ± 2.1 kg m-2) participated in this study. They performed two test exercises on two separate days: a maximal incremental cycle test (land condition), and an incremental fin swimming (fin condition) in a motorized swimming flume.
Results: The respective fin and land
Conclusion: Surface immersion did not significantly reduce the
Introduction
During immersed physical exercise, cardiovascular, ventilatory and metabolic capacities of divers are altered by at least three mechanisms: the specific thoraco-pulmonary effects of immersion (Morrison and Butt, 1972; Morrison et al., 1975; Prefaut et al., 1976; Taylor and Morrison, 1999; Taylor et al., 2014; Castagna et al., 2018b; Castagna et al., 2021); the effects related to the breathing apparatus (Moon et al., 2009); and the effects of the depth of immersion which increases the density and thus the viscosity of the gases breathed (Kao, 1963; Lanphier, 1963; Davies et al., 1970; Morrison and Butt, 1972; Morrison et al., 1975; Morrison et al., 1976; Thalmann et al., 1979; Warkander et al., 1992).
Studies of oxygen uptake underwater have been undertaken with subjects counteracting active drag (Di Prampero et al., 1974), or swimming on a swimming flume since this device was first described by Holmér and Åstrand (Holmer and Astrand, 1972). Several groups have described an increase in
Military surface fin diving is an intense activity that can result in accidents. This activity thus deserves thorough investigation. Immersion pulmonary edemas (IPE) can develop during fin swimming exercises (with a snorkel or a diving breathing apparatus). Indeed, IPE are the first cause of hospitalization among military divers, more frequent even than decompression accidents (Coulange et al., 2010; Gempp et al., 2011; Castagna et al., 2017; Castagna et al., 2018a). It is now well established that the increased ventilatory and cardiovascular demands induced by fin swimming contribute to the occurrence of IPE (Fraser et al., 2011; Peacher et al., 2015; Moon et al., 2016; Moon, 2019; Wilmshurst, 2021; Hageman et al., 2022). We must therefore precisely measure these adaptations to ensure that military divers are adequately trained to withstand the constraints associated with their diving in practice.
The first purpose of this study was to check whether fin swimming altered maximal pulmonary ventilation (
Methods
Subjects
Eighteen male divers (32.3 ± 4.2 yrs; 178.0 ± 5.0 cm; 76.4 ± 3.4 kg; 24.1 ± 2.1 kg m-2) participated in this study. Subjects were healthy non-smokers reporting no history of cardiopulmonary disease. All subjects were military divers with at least 5 years’ experience. At the time of the study, they performed a minimum of two training dives per week. All experimental procedures were conducted in line with the declaration of Helsinki. The study protocol was approved by the local ethics committee (Comité de Protection des Personnes-CPP Sud Méditerranée V, ref 160077). Each subject gave written consent before participating in this study.
Experimental overview
All subjects performed two test exercises on two separates days in no specified order (Figure 1). On day 1, subjects performed a maximal incremental cycle test (land condition) in the IRBA Physiology Lab. On day 2, subjects performed a maximal incremental fin swimming test (fin condition) in a motorized swimming flume housed within the IRBA Physiology Lab. A rest period of 48–72 h was interposed between tests.
Spirometry measurements at rest
When taking resting spirometry measurements, in the control condition (land), subjects were standing upright in the air. In immersion condition, subjects were positioned upright on their knees, immersed up to the sternal notch (head out of the water immersion) in the pool.
In line with the guidelines of the American Thoracic and European Respiratory Societies [27], forced vital capacity (FVC), forced expiratory volume exhaled in 1 s (FEV1.0), peak expiratory Flow (PEF), expiratory reserve volume (ERV), inspiratory reserve volume (IRV), and tidal volume (Vt) were measured. A Cosmed® Quark PFT Ergo device was used (Cosmed®, Rome, Italy).
Maximal voluntary ventilation (MVV) was calculated from the FEV1.0 using the formula MVV = FEV1.0 × 39. Breathing Reserve (BR) i.e., the difference between MVV and the maximum ventilation measured during the exercise test, was determined at peak exercise.
Each subject repeated the spirometry maneuver five times in both land and fin conditions. The two extreme values for each variable were discarded, and the mean of the remaining three was retained.
Maximal incremental cycling test (land condition)
Participants performed a 10-min standardized 50-W warm-up on a cycle ergometer (E5, COSMED, Rome, Italy). Exercise intensity was then increased stepwise in 25-W increments every minute until volitional exhaustion. Subjects chose a pedaling frequency between 75 and 90 rpm. The selected frequency was maintained throughout the graded exercise test and replicated in all experimental trials.
Heart rate (HR), was monitored with a waterproof heart rate monitor (Polar V800, Helsinki, Finland); tidal volume (Vt), breath frequency (f), oxygen uptake (
Incremental fin swimming on a flume (fin condition)
The fin condition was conducted in a motorized swimming flume (Endless Pools, Dilsen-Stokkem, Belgium) housed within the IRBA Physiology Lab. The linearity and the relationship between engine power and water speed were fully calibrated using a water flowmeter.
The same material as for the cycling test was used to measure HR and gas exchanges at mouth-level. To introduce the metabolimeter, the mouth-piece was connected to a snorkel with a low airflow resistance (Dalacqua, Cosmed), as validated by Rodriguez et al. (Rodriguez et al., 2008).
After 10 min of rest, subjects performed a maximal test according to an incremental protocol. After 1 min at a flume speed of 1.0 m s-1, the speed was increased by 0.2 m s-1 until volitional exhaustion. The
Statistical analysis
Statistical analyses were performed using Prism 6 software (GraphPad Software, La Jolla California United States). Each subject served as his own control. Data distribution was assessed using a Kolmogorov-Smirnov test. To compare the maximum physiological responses from the two incremental tests, a paired Student’s t-test was applied when the data were normally distributed. For non-normally distributed data, a Wilcoxon test was used. The same approach was used to assess lung function at rest in both conditions.
For values obtained at repeated points (
Differences between groups were considered statistically significant at p < 0.05. All values are expressed as mean ± SD.
Results
Immersion
Lung function was assessed at rest, in both chest-out-of-water and chest immersed (head-out-of-water) conditions. Data are reported in Table 1.
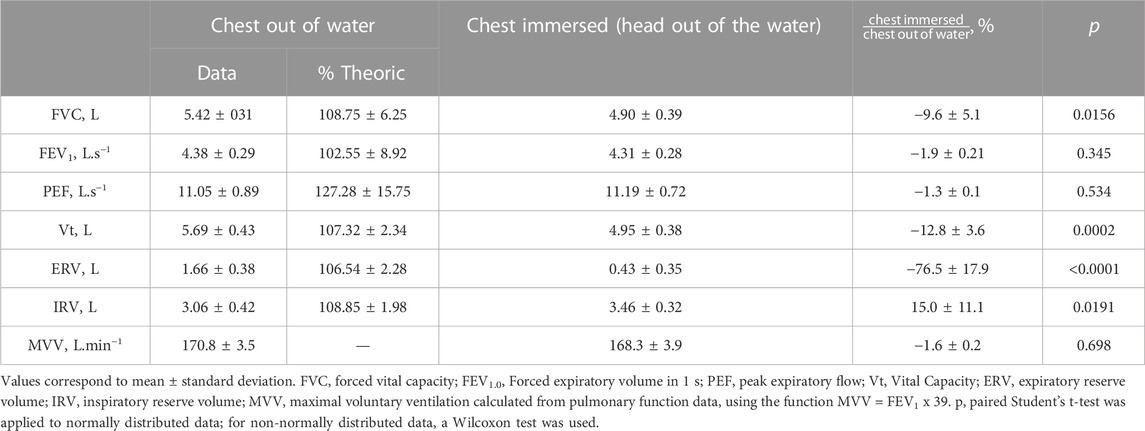
TABLE 1. Lung function assessed at rest, on land and during head-out-of-water immersion (Immersion).
Immersion was associated with a 74% collapse of ERV (p < 0.001), which was not compensated by a 13% increase in IRV (p = 0.02). These two immersion situations resulted in small decreases in FVC and Vt (p < 0.02 and p < 0.001, respectively). Chest immersion induced no further significant alterations to FEV1.0, PEF, and MVV. An example of immersion-induced changes in lung function at rest (total lung capacity) is illustrated in the supplementary files (Supplementary Figure S4).
Incremental tests
To compare the physiological adaptations in response to land and fin conditions, since no significant difference in
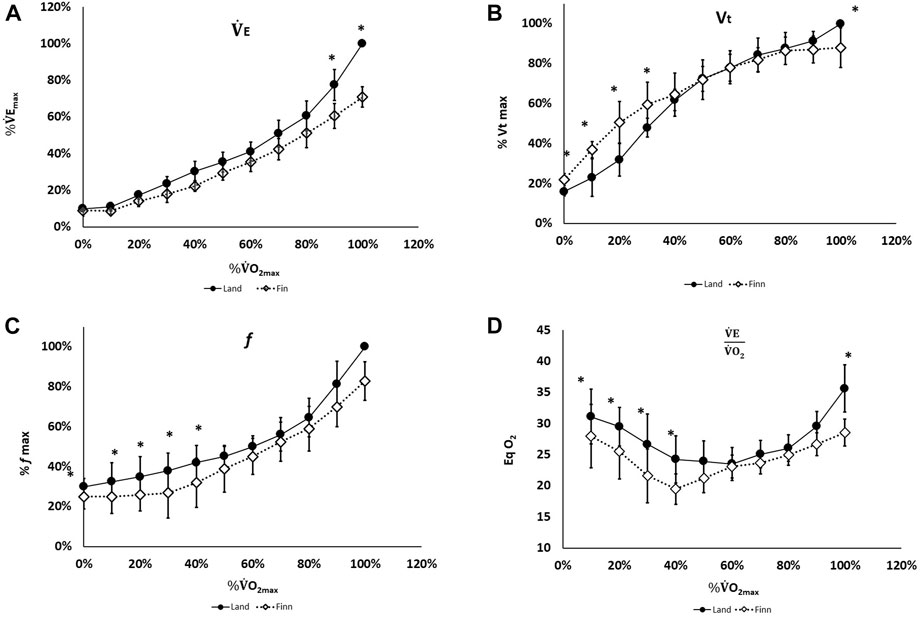
FIGURE 2. Changes to respiratory variables and heart rate (HR) expressed as a percentage relative to maximal oxygen consumption on land. Variables are expressed as a percentage of the maximum value recorded during the test.
No significant difference in
At rest and up to an exercise intensity of 40%
The respective maximal values obtained during incremental cycling and fin swimming tests are reported in Table 2. No significant differences between the two conditions were observed in the maximum values of HR [La]b, and R.
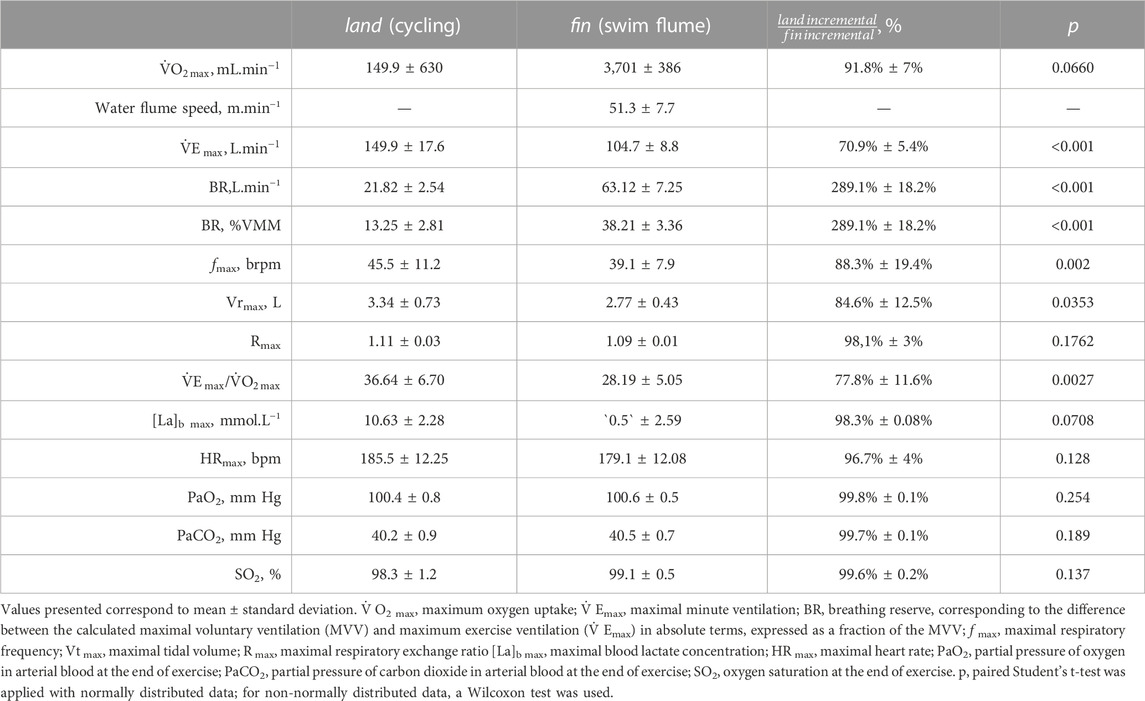
TABLE 2. Maximum physiological responses observed in cycling (land condition) and fin swimming (fin condition) incremental tests.
The
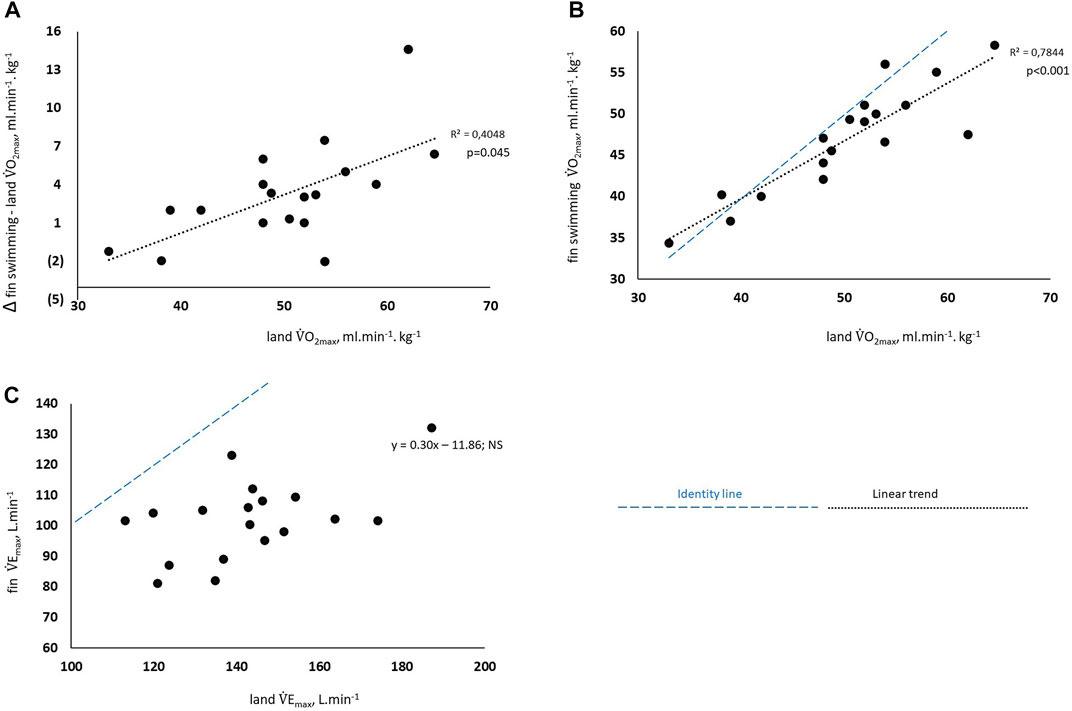
FIGURE 3. Maximum minute ventilation and maximum oxygen uptake in both land cycling and fin swimming conditions. • (A) Changes to differences in maximum oxygen uptake (
Discussion
This mainly descriptive study was designed to investigate an operational military problem: the assessment of metabolic load and of the corresponding variables during a surface fin swimming exercise in well-trained military divers. We first investigated the immersion-specific effects on ventilation to determine whether ventilation would contribute to limiting gas exchanges when performing an incremental surface fin swimming exercise. A second purpose was to determine whether surface fin swimming and land exercise entailed different metabolic loads (
Effects of surface immersion on gas exchanges during fin swimming on water surface in trained divers.
In the fin swimming condition, the higher resting Vt and lower resting f, associated with a lower
Immersion induced a cephalad displacement of the diaphragm
Immersion led to significant changes in ventilation due to the water pressure exerted on tissues (Choukroun et al., 1989; Christie et al., 1990). Indeed, immersion pushes part of the blood content from the limbs back to the right heart, leading to a tendency for accumulation in the pulmonary circulation and abdominal vascular territory. This type of effect is known as a blood shift. From a ventilatory point of view, the blood shift leads to pulmonary vascular congestion (Agostoni et al., 1966). The reduced expiratory reserve volume (ERV) associated with head-out-of-water immersion reflected a decrease in residual functional capacity (Paton and Sand, 1947; Jarrett, 1965). By compressing the abdomen, hydrostatic pressure pushes the diaphragm toward the chest, thus reducing chest wall compliance and reducing the pulmonary gas volume. As a consequence, vital capacity is reduced. We recently confirmed observations from 1978 (Dahlback et al., 1978), showing that the immersion-induced changes in lung spirometry volumes are associated with an overall impaired compliance of the respiratory system (Castagna et al., 2021).
During incremental exercise, differences between land and fin conditions reflect the conflicting effects of immersion and exercise on ventilation. Up to 40%
However, the decreased
The
This enhanced ventilation efficiency during immersion, could mainly be explained by better lung perfusion. Indeed, the blood shift induced by immersion generated a rise in pulmonary perfusion due to the increase in right ventricle preloading and slight pulmonary vascular congestion.
Altogether the data presented suggest that metabolic gas exchanges are more efficient during maximal fin swimming than in land conditions, due to improved ventilation efficiency upon immersion. The improvement was mainly related to enhanced lung perfusion. The
Effects of surface immersion on O2max during fin swimming on water surface in trained divers
The land values recorded for the military divers studied here were higher than those reported for general male subjects in a similar age range (Wilson and Tanaka, 2000; Trappe et al., 2013). This difference confirms the good training status of our subjects. The fin
An important feature of this study was that immersion does not reduce the maximum aerobic capacity of trained subjects. The
The decrease in
These results contrast with the data obtained by Jammes et al. (Jammes et al., 2009) who compared cardiovascular responses during incremental exercise on a cycle ergometer to an underwater swimming exercise. Although these authors also reported no significant difference in
Perspectives and practical interests of the study
Age (over 50 years) and high blood pressure are factors that favor the occurrence of IPE (Gempp et al., 2011; Gempp et al., 2014). However, despite their young age and good health profiles, IPE have become the first cause of hospitalization for military divers - ahead of decompression sickness. The development of IPE in professional male SCUBA divers was previously confirmed by our team to involve a key role of negative pressure breathing induced by hydrostatic imbalance (Castagna et al., 2018b). As hydrostatic imbalance is now taken into account by manufacturers and the military hierarchy, most IPE developing in military divers occur during surface fin swimming exercises. Accidents mainly involve students training to become military divers, and specifically tend to occur during timed surface fin swimming exercises. During these tests, student divers must cover a distance (between 1,000 and 5,000 m) as quickly as possible at the risk of being eliminated. To pass these tests, the students must perform a high-intensity fin swimming exercise lasting several dozen minutes.
The results of the present study demonstrate that pulmonary ventilation related to military fin swimming is much higher than expected. The relationship between an increase in pulmonary ventilation demand and increased work of breathing (WOB) has now been clearly established (Coulange et al., 2010; Bates et al., 2011; Carter and Koehle, 2011; Castagna et al., 2017; Castagna et al., 2018b). Furthermore, some studies have demonstrated the major role played by a high WOB, even in the absence of hydrostatic imbalance, in the occurrence of this type of accident (Peacher et al., 2015; Moon, 2019). We therefore hypothesize that the respiratory work induced by high-intensity fin swimming is susceptible to induce IPE.
Manufacturers of breathing apparatus are developing systems for divers with the lowest possible ventilatory constraints. In Europe, they can rely on the WOB limit values prescribed by European standards (EN 250 and EN 14143). These standards define the maximum acceptable values of WOB in a range of ventilatory regimes (
Limitations
It may seem counter-intuitive that, in trained military divers, surface immersion does not lead to a reduction in pulmonary ventilation. Indeed, as immersion reduces lung capacity it should reduce pulmonary ventilation. A study carried out in non-athletic subjects, with no training for swimming or fin swimming, would therefore be necessary to determine whether the results of this study also apply to subjects without specific training in immersion exercise.
Due to this unexpected observation, pulmonary ventilation related to military fin swimming was much higher than predicted, even exceeding the limits set by the standards with which the manufacturers of diving breathing apparatus must comply. We hypothesized that, in military divers, high pulmonary ventilation was associated with a high WOB, which would favor the occurrence of IPE. Unfortunately, we were unable to confirm this hypothesis. The duration usually prescribed for maximal incremental exercise (8–12 min) is too short for clinical signs of IPE to appear. Moreover, no Ultrasound Lung Comets (ULC) were observed on the lung ultrasounds performed at the end of the immersions. It would be necessary to reproduce this study by measuring the WOB required of each subject breath by breath. To perform these measurements, the operational pneumo-baro tachograph, PBO, has been developed in our laboratory (Castagna et al., 2017; Castagna et al., 2018b; Castagna et al., 2021).
The swimming condition for this study was carried out in a laboratory-generated swim flume allowing the precise assessment of metabolic and ventilatory variables. The results obtained will need to be confirmed through measurements on swimmers in the sea.
The results from this study, involving swimming at the surface of the water, cannot be directly transposed to deep underwater diving. Indeed, the density of the gases breathed, increasing with the depth of immersion, the resistance to the flow of gases in the lungs and the breathing apparatus used strongly reduce the ventilatory aptitudes of divers (Thalmann et al., 1979; Held and Pendergast, 2013). Further studies will be necessary to confirm these observations, in particular by carrying out joint measurements of ventilation and WOB in immersion.
In fin condition, the use of the snorkel made breath-by-breath analysis of exhaled gases impossible. Consequently, values were averaged every 10 s. Therefore, we do not have access to the Pet O2 and Pet CO2 values for the fin swimming exercise, making it impossible to calculate/estimate alveolar PO2 during swimming. This would provide valuable data regarding overall pulmonary gas exchange efficiency (e.g., via the AaDO2) to confirm the relative contribution of ventilation-perfusion matching.
We observed no significant differences between the values for arterial oxygen saturation (SO2), arterial partial pressure of oxygen (PaO2), and alveolar partial pressure of oxygen (PACO2) measured at rest and immediately after the end of exercise in either condition (land vs fin). Using the equation proposed by Elliot et al. (Elliott et al., 2014), we estimated the values of alveolar partial pressure of carbon dioxygen (PACO2). Secondarily, we determined values for the alveolar - arterial difference in partial pressure of oxygen (A-aDO2), which is a measure of efficiency for gas exchange. The values of A-a DO2 were similar between the two conditions (land vs fin), and no significant difference in the expansion of A-a DO2, induced by physical exercise, was observed whether exercise was performed on land (6.69 ± 0.15 mmHg) or in water (7.27 ± 0.13 mmHg).
Since the peak values of
Conclusion
Surface immersion was found not to impair the maximal aerobic power (
Data availability statement
The original contributions presented in the study are included in the article/Supplementary Material, further inquiries can be directed to the corresponding author.
Ethics statement
The studies involving human participants were reviewed and approved by The study protocol was approved by the local ethics committee (Comité de Protection des Personnes-CPP Sud Méditerranée V, ref 160077). Each subject gave written consent before participating in this study. The patients/participants provided their written informed consent to participate in this study.
Author contributions
OC: conceptualization and design of the study, analysis and interpretation of the data, writing and revising the manuscript. AD, and JA: interpretation of the data and revising the manuscript. J-EB: revising the manuscript J-RL: analysis and interpretation of the data, writing and revising the manuscript. All authors contributed to the article and approved the submitted version.
Acknowledgments
We thank B. Schmid, Engineer, for his invaluable contribution to this work.
Conflict of interest
The authors declare that the research was conducted in the absence of any commercial or financial relationships that could be construed as a potential conflict of interest.
Publisher’s note
All claims expressed in this article are solely those of the authors and do not necessarily represent those of their affiliated organizations, or those of the publisher, the editors and the reviewers. Any product that may be evaluated in this article, or claim that may be made by its manufacturer, is not guaranteed or endorsed by the publisher.
Supplementary Material
The Supplementary Material for this article can be found online at: https://www.frontiersin.org/articles/10.3389/fphys.2023.1145204/full#supplementary-material
References
Agostoni, E., Gurtner, G., Torri, G., and Rahn, H. (1966). Respiratory mechanics during submersion and negative-pressure breathing. J. Appl. Physiol. 21 (1), 251–258. doi:10.1152/jappl.1966.21.1.251
Bates, M. L., Farrell, E. T., and Eldridge, M. W. (2011). The curious question of exercise-induced pulmonary edema. Pulm. Med. 2011, 361931. doi:10.1155/2011/361931
Begin, R., Epstein, M., Sackner, M. A., Levinson, R., Dougherty, R., and Duncan, D. (1976). Effects of water immersion to the neck on pulmonary circulation and tissue volume in man. J. Appl. Physiol. 40 (3), 293–299. doi:10.1152/jappl.1976.40.3.293
Carter, E. A., and Koehle, M. S. (2011). Immersion pulmonary edema in female triathletes. Pulm. Med. 2011, 261404. doi:10.1155/2011/261404
Castagna, O., de Maistre, S., Schmid, B., Caudal, D., and Regnard, J. (2018a). Immersion pulmonary oedema in a healthy diver not exposed to cold or strenuous exercise. Diving Hyperb. Med. 48 (1), 40–44. doi:10.28920/dhm48.1.40-44
Castagna, O., Gempp, E., Poyet, R., Schmid, B., Desruelle, A. V., Crunel, V., et al. (2017). Cardiovascular mechanisms of extravascular lung water accumulation in divers. Am. J. Cardiol. 119 (6), 929–932. doi:10.1016/j.amjcard.2016.11.050
Castagna, O., Michoud, G., Prevautel, T., Delafargue, A., Schmid, B., Similowski, T., et al. (2021). Broad individual immersion-scattering of respiratory compliance likely substantiates dissimilar breathing mechanics. Sci. Rep. 11 (1), 9434. doi:10.1038/s41598-021-88925-x
Castagna, O., Regnard, J., Gempp, E., Louge, P., Brocq, F. X., Schmid, B., et al. (2018b). The key roles of negative pressure breathing and exercise in the development of interstitial pulmonary edema in professional male SCUBA divers. Sports Med. Open 4 (1), 1. doi:10.1186/s40798-017-0116-x
Choukroun, M. L., Kays, C., and Varene, P. (1989). Effects of water temperature on pulmonary volumes in immersed human subjects. Respir. Physiol. 75 (3), 255–265. doi:10.1016/0034-5687(89)90036-4
Christie, J. L., Sheldahl, L. M., Tristani, F. E., Wann, L. S., Sagar, K. B., Levandoski, S. G., et al. (1990). Cardiovascular regulation during head-out water immersion exercise. J. Appl. Physiol. 69 (2), 657–664. doi:10.1152/jappl.1990.69.2.657
Coulange, M., Rossi, P., Gargne, O., Gole, Y., Bessereau, J., Regnard, J., et al. (2010). Pulmonary oedema in healthy SCUBA divers: New physiopathological pathways. Clin. Physiol. Funct. Imaging 30 (3), 181–186. doi:10.1111/j.1475-097X.2010.00922.x
Craig, A. B., and Dvorak, M. (1975). Expiratory reserve volume and vital capacity of the lungs during immersion in water. J. Appl. Physiol. 38 (1), 5–9. doi:10.1152/jappl.1975.38.1.5
Dahlback, G. O., Jonsson, E., and Liner, M. H. (1978). Influence of hydrostatic compression of the chest and intrathoracic blood pooling on static lung mechanics during head-out immersion. Undersea Biomed. Res. 5 (1), 71–85.
Davies, C. T., Tuxworth, W., and Young, J. M. (1970). Physiological effects of repeated exercise. Clin. Sci. 39 (2), 247–258. doi:10.1042/cs0390247
Di Prampero, P. E., Pendergast, D. R., Wilson, D. W., and Rennie, D. W. (1974). Energetics of swimming in man. J. Appl. Physiol. 37 (1), 1–5. doi:10.1152/jappl.1974.37.1.1
Donald, K. W., and Davidson, W. M. (1954). Oxygen uptake of booted and fin swimming divers. J. Appl. Physiol. 7 (1), 31–37. doi:10.1152/jappl.1954.7.1.31
Edvardsen, E., Hem, E., and Anderssen, S. A. (2014). End criteria for reaching maximal oxygen uptake must be strict and adjusted to sex and age: A cross-sectional study. PLoS One 9 (1), e85276. doi:10.1371/journal.pone.0085276
Elliott, J. E., Duke, J. W., Hawn, J. A., Halliwill, J. R., and Lovering, A. T. (2014). Increased cardiac output, not pulmonary artery systolic pressure, increases intrapulmonary shunt in healthy humans breathing room air and 40% O2. J. Physiol. 592 (20), 4537–4553. doi:10.1113/jphysiol.2014.274829
Fraser, J. A., Peacher, D. F., Freiberger, J. J., Natoli, M. J., Schinazi, E. A., Beck, I. V., et al. (2011). Risk factors for immersion pulmonary edema: Hyperoxia does not attenuate pulmonary hypertension associated with cold water-immersed prone exercise at 4.7 ATA. J. Appl. Physiol. (1985) 110 (3), 610–618. doi:10.1152/japplphysiol.01088.2010
Gempp, E., Demaistre, S., and Louge, P. (2014). Hypertension is predictive of recurrent immersion pulmonary edema in scuba divers. Int. J. Cardiol. 172 (2), 528–529. doi:10.1016/j.ijcard.2014.01.021
Gempp, E., Louge, P., Blatteau, J. E., and Hugon, M. (2011). Descriptive epidemiology of 153 diving injuries with rebreathers among French military divers from 1979 to 2009. Mil. Med. 176 (4), 446–450. doi:10.7205/milmed-d-10-00420
Goff, L. G., Brubach, H. F., and Specht, H. (1957). Measurements of respiratory responses and work efficiency of underwater swimmers utilizing improved instrumentation. J. Appl. Physiol. 10 (2), 197–202. doi:10.1152/jappl.1957.10.2.197
Hageman, S. M., Chakraborty, R. K., and Murphy-Lavoie, H. M. (2022). "Immersion pulmonary edema," in StatPearls. (Treasure island (FL)).
Hampson, N. B., and Dunford, R. G. (1997). Pulmonary edema of scuba divers. Undersea Hyperb. Med. 24 (1), 29–33.
Held, H. E., and Pendergast, D. R. (2013). Relative effects of submersion and increased pressure on respiratory mechanics, work, and energy cost of breathing. J. Appl. Physiol. (1985) 114 (5), 578–591. doi:10.1152/japplphysiol.00584.2012
Holmer, I., and Astrand, P. O. (1972). Swimming training and maximal oxygen uptake. J. Appl. Physiol. 33 (4), 510–513. doi:10.1152/jappl.1972.33.4.510
Hong, S. K., Cerretelli, P., Cruz, J. C., and Rahn, H. (1969). Mechanics of respiration during submersion in water. J. Appl. Physiol. 27 (4), 535–538. doi:10.1152/jappl.1969.27.4.535
Jammes, Y., Coulange, M., Delliaux, S., Jammes, C., Gole, Y., Boussuges, A., et al. (2009). Fin swimming improves respiratory gas exchange. Int. J. Sports Med. 30 (3), 173–181. doi:10.1055/s-0028-1105939
Jarrett, A. S. (1965). Effect of immersion on intrapulmonary pressure. J. Appl. Physiology 20 (6), 1261–1266. doi:10.1152/jappl.1965.20.6.1261
Kao, F. (1963). An experimental study of the pathways involved in exercice hypernea employing cross circulation techniques. régulation Hum. Respir., 461–502.
Lanphier, E. H. (1963). Influence of increased ambient pressure upon alveolar ventilation. In Proc. 2nd Symp. Underwater Physiology. Ed. C. J. Lambertsen and L. J. Greenbaum, Jr. pp. 124–133. Washington DC • Natl Acad. Sci.-Natl Res. Council (Publ. 1181).
Mier, C. M., Alexander, R. P., and Mageean, A. L. (2012). Achievement of VO2max criteria during a continuous graded exercise test and a verification stage performed by college athletes. J. Strength Cond. Res. 26 (10), 2648–2654. doi:10.1519/JSC.0b013e31823f8de9
Moon, R. E. (2019). Immersion pulmonary edema: Drowning from the inside. Undersea Hyperb. Med. 46 (5), 577–579.
Moon, R. E., Cherry, A. D., Stolp, B. W., and Camporesi, E. M. (2009). Pulmonary gas exchange in diving. J. Appl. Physiol. 106 (2), 668–677. doi:10.1152/japplphysiol.91104.2008
Moon, R. E., Martina, S. D., Peacher, D. F., Potter, J. F., Wester, T. E., Cherry, A. D., et al. (2016). Swimming-induced pulmonary edema: Pathophysiology and risk reduction with sildenafil. Circulation 133 (10), 988–996. doi:10.1161/CIRCULATIONAHA.115.019464
Morrison, J. B., and Butt, W. S. (1972). Effect of underwater breathing apparatus and absolute air pressure on divers' ventilatory capacity. Aerosp. Med. 43 (8), 881–886.
Morrison, J. B., Butt, W. S., Florio, J. T., and Mayo, I. C. (1976). Effects of increased O2-N2 pressure and breathing apparatus on respiratory function. Undersea Biomed. Res. 3 (3), 217–234.
Morrison, J. B., Florio, J., Butt, W. S., and Mayo, I. C. (1975). The effects of underwater breathing apparatus and absolute pressure on the respiratory response to exercise. R. Nav. Physiol. Lab. Rep.
Paton, W. D., and Sand, A. (1947). The optimum intrapulmonary pressure in underwater respiration. J. Physiol. 106 (2), 119–138. doi:10.1113/jphysiol.1947.sp004198
Peacher, D. F., Martina, S. D., Otteni, C. E., Wester, T. E., Potter, J. F., and Moon, R. E. (2015). Immersion pulmonary edema and comorbidities: Case series and updated review. Med. Sci. Sports Exerc 47 (6), 1128–1134. doi:10.1249/MSS.0000000000000524
Pendergast, D. R., and Lundgren, C. E. (2009). The underwater environment: Cardiopulmonary, thermal, and energetic demands. J. Appl. Physiol. 106 (1), 276–283. doi:10.1152/japplphysiol.90984.2008
Pendergast, D. R., Mollendorf, J., Logue, C., and Samimy, S. (2003). Evaluation of fins used in underwater swimming. Undersea Hyperb. Med. 30 (1), 57–73.
Prefaut, C., Lupi-h, E., and Anthonisen, N. R. (1976). Human lung mechanics during water immersion. J. Appl. Physiol. 40 (3), 320–323. doi:10.1152/jappl.1976.40.3.320
Rodriguez, F. A., Keskinen, K. L., Kusch, M., and Hoffmann, U. (2008). Validity of a swimming snorkel for metabolic testing. Int. J. Sports Med. 29 (2), 120–128. doi:10.1055/s-2007-964973
Smirmaul, B. P., Bertucci, D. R., and Teixeira, I. P. (2013). Is the VO2max that we measure really maximal? Front. Physiol. 4, 203. doi:10.3389/fphys.2013.00203
Taylor, B. J., Carlson, A. R., Miller, A. D., and Johnson, B. D. (2014). Exercise-induced interstitial pulmonary edema at sea-level in young and old healthy humans. Respir. Physiol. Neurobiol. 191, 17–25. doi:10.1016/j.resp.2013.10.012
Taylor, N. A., and Morrison, J. B. (1989). Lung centroid pressure in immersed man. Undersea Biomed. Res. 16 (1), 3–19.
Taylor, N. A., and Morrison, J. B. (1999). Static respiratory muscle work during immersion with positive and negative respiratory loading. J. Appl. Physiol. (1985) 87 (4), 1397–1403. doi:10.1152/jappl.1999.87.4.1397
Thalmann, E. D., Sponholtz, D. K., and Lundgren, C. E. (1979). Effects of immersion and static lung loading on submerged exercise at depth. Undersea Biomed. Res. 6 (3), 259–290.
Trappe, S., Hayes, E., Galpin, A., Kaminsky, L., Jemiolo, B., Fink, W., et al. (2013). New records in aerobic power among octogenarian lifelong endurance athletes. J. Appl. Physiol. (1985) 114 (1), 3–10. doi:10.1152/japplphysiol.01107.2012
Warkander, D. E., Norfleet, W. T., Nagasawa, G. K., and Lundgren, C. E. (1992). Physiologically and subjectively acceptable breathing resistance in divers' breathing gear. Undersea Biomed. Res. 19 (6), 427–445.
Wilmshurst, P. (2021). Immersion pulmonary edema. Chest 159 (5), 1711–1712. doi:10.1016/j.chest.2020.12.017
Wilson, T. M., and Tanaka, H. (2000). Meta-analysis of the age-associated decline in maximal aerobic capacity in men: Relation to training status. Am. J. Physiol. Heart Circ. Physiol. 278 (3), H829–H834. doi:10.1152/ajpheart.2000.278.3.H829
Wylegala, J. A., Pendergast, D. R., Gosselin, L. E., Warkander, D. E., and Lundgren, C. E. (2007b). Respiratory muscle training improves swimming endurance in divers. Eur. J. Appl. Physiol. 99 (4), 393–404. doi:10.1007/s00421-006-0359-6
Wylegala, J., Schafer-Owczarzak, M., and Pendergast, D. R. (2007a). Optimization of fin-swim training for SCUBA divers. Undersea Hyperb. Med. 34 (6), 431–438.
Yamaguchi, H., Shidara, F., Naraki, N., and Mohri, M. (1995). Maximum sustained fin-kick thrust in underwater swimming. Undersea Hyperb. Med. 22 (3), 241–248.
Keywords: fin swimming, oxygen uptake, pulmonary ventilation, heart rate, lactate, swim flume
Citation: Castagna O, Blatteau J-E, Druelle A, Amara J and Lacour J-R (2023) Oxygen uptake (
Received: 15 January 2023; Accepted: 21 February 2023;
Published: 06 March 2023.
Edited by:
Karen R. Kelly, Naval Health Research Center, United StatesReviewed by:
Alessandro Marroni, DAN Europe Foundation, MaltaJonathan E. Elliott, Oregon Health and Science University, United States
Copyright © 2023 Castagna, Blatteau, Druelle, Amara and Lacour. This is an open-access article distributed under the terms of the Creative Commons Attribution License (CC BY). The use, distribution or reproduction in other forums is permitted, provided the original author(s) and the copyright owner(s) are credited and that the original publication in this journal is cited, in accordance with accepted academic practice. No use, distribution or reproduction is permitted which does not comply with these terms.
*Correspondence: Jean-Eric Blatteau, YmxhdHRlYXVqZUBnbWFpbC5jb20=