- Hunan Plant Protection Institute, Hunan Academy of Agricultural Science, Changsha, China
The nuclear receptors HR3 and FTZ-F1 are highly conserved and function to regulate molting and reproduction in both hemimetabolous and holometabolous insects. However, their roles in Nilaparvata lugens are largely unknown. In the present study, we discover that NlHR3 and NlFTZ-F1 are activated in the nymph stages by ecdysone signaling. Transcription disruption of NlHR3 and NlFTZ-F1 expression prevents nymph ecdysis and metamorphosis, which leads to abnormal appearance, malformed ovaries, and lethal phenotypes. In addition, we demonstrate that NlHR3 and NlFTZ-F1 regulate molting and reproduction by interacting with the intrinsic 20E and JH signaling pathways. Our work offers a deep insight into the action mechanisms of HR3 and FTZ-F1 in insects. Moreover, NlHR3 and NlFTZ-F1 could properly be exploited as potential target genes for developing RNAi-based pesticides to control N. lugens.
1 Introduction
The steroid hormone 20-hydroxyecdysone (20E) and the sesquiterpenoid juvenile hormone (JH) play key roles in insect growth and development. In the nucleus, 20E can bind to the heterodimeric complex of the ecdysone receptor (EcR)/ultraspiracle, triggering the expression of 20E-induced cascade genes, such as Broad-Complex (Br-C), hormone receptor 3 (HR3), HR4, ecdysone-induced protein 75B (E75B), and FTZ-F1 (Pierceall et al., 1999; Swevers et al., 2002; Siaussat et al., 2004; Cruz et al., 2008; Liu et al., 2014; Li et al., 2015; Zhang et al., 2022). These ecdysone cascade genes are mostly nuclear receptor genes, having well-conserved DNA-binding domains and ligand-binding domains, and play a critical role in a variety of signaling and metabolic pathways, including the 20E signaling pathway (King-Jones and Thummel, 2005).
Hormone receptor 3 (HR3) belongs to the nuclear receptor superfamily, with a typical domain structure, including variable N-terminal domains (A/B), DNA-binding domains (C), hinge regions (D), and ligand-binding domains (E/F) (Nakagawa and Henrich, 2009). HR3s have been characterized in several species of Lepidoptera (Eystathioy et al., 2001), Diptera (Carney et al., 1997), Blattaria (Cruz et al., 2007), and Coleoptera (Tan and Palli, 2008; Xu et al., 2010; Zhao et al., 2018). DmHR3 plays an important role in enhancing molting and metamorphosis in Drosophila melanogaster (Lam et al., 1997; Lam et al., 1999). RNA interference (RNAi) causes developmental deficiency and mortality, indicating HR3 is a crucial gene for molting and metamorphosis in several insects (Xu et al., 2010; Guo et al., 2015; Zhao et al., 2018).
FTZ-F1 (fushi tarazu factor 1), a nuclear receptor-type transcription factor, was first identified during early embryogenesis as a transcription factor binding to the promoter of the pair-rule segmentation gene known as fushi tarazu (Lala et al., 1992). FTZ-F1 was involved in molting and metamorphosis in Drosophila (Dubrovsky et al., 2011; Sultan et al., 2014), Aedes aegypti (Li et al., 2000), Blattella germanica (Cruz et al., 2008), Spodoptera litura (Tang et al., 2011), Tribolium castaneum (Tan and Palli, 2008), and Leptinotarsa decemlineata (Liu et al., 2014). The knockdown of FTZ-F1 led to developmental arrest and phenotypic defects in these insects. The transcription of ecdysone biosynthetic genes, phantom and disembodied, could also respond to FTZ-F1 to regulate ecdysone biosynthesis (Parvy et al., 2005). The knockdown of LdFTZ-F1 inhibited the expression of ecdysone biosynthetic genes and reduced the titer of 20E, which eventually leads to pupation failure in L. decemlineata (Liu et al., 2014).
The 20E pulse triggers a transcriptional cascade composed of 20E early-response genes, such as hormone receptor 3 (HR3) and HR4 (King-Jones and Thummel, 2005; Ruaud et al., 2010), and then induces a nuclear receptor factor, FTZ-F1, to play a developmental switch role (Dubrovsky, 2005; Zhang et al., 2021). In D. melanogaster, DmHR3 induced the expression of βFTZ-F1 by directly binding to DmHR3-binding sites and transmitted a 20E signal cascade (Parvy et al., 2014). All these results indicated that HR3 and FTZ-F1 play a crucial role in insect growth and development, and there are interactions between them and the 20E signaling pathway.
The brown planthopper, Nilaparvata lugens (Stål), is one of the most destructive pests that feed on rice plants, causing a significant threat to rice production of China and other Asian countries (Cheng et al., 2003; Heong and Hardy, 2009). Although the molecular functions of HR3 and FTZ-F1 at molting and metamorphosis stages in holometabolous insects have been well investigated, their role in N. lugens and the relationship between NlHR3, NlFTZ-F1, and the 20E signaling pathway remain unclear. In the present study, we cloned HR3 and FTZ-F1 from N. lugens and analyzed their developmental and tissue-specific expression profiles. The relationship between NlHR3, NlFTZ-F1, and the 20E signaling pathway was also investigated through RNAi, the gene expression level, and measurements of survival rates. The results will aid the elucidation of insect molting and metamorphosis mediated by nuclear receptor genes and provide potential target genes for the development of RNAi insecticides to control the brown planthopper.
2 Materials and methods
2.1 Insect rearing
Brown planthoppers were reared on a susceptible rice (Oryza sativa) variety, Taichung Native 1 (TN1), cultivated at 27°C ± 0.5°C and 75% ± 5% relative humidity under a 14L: 10D (h) photoperiod, according to a previously described method (Li et al., 2018).
2.2 RNA extraction and cDNA synthesis
Total RNA was extracted with the TRIzol Total RNA Isolation Kit (Invitrogen, Carlsbad, CA, United States). The concentration and purity were measured with the NanoDrop 1000 spectrophotometer (Thermo Fisher Scientific, Rockford, IL, United States) and determined by agarose gel electrophoresis. cDNA was synthesized by using the ReverTra Ace qPCR RT Kit (Toyobo Co., Ltd., Osaka, Japan), following the manufacturer’s manual.
2.3 Molecular cloning and sequence analysis
Based on the published N. lugens genomic and transcriptomic data (Xue et al., 2014; Wan et al., 2015), NlHR3 and NlFTZ-F1 homologies were identified and their sequences were confirmed by the reverse transcription polymerase chain reaction (RT-PCR) using primers, as shown in Supplementary Table S1. The PCR product was gel purified, ligated into the vector TOPO2.1 (Invitrogen, Carlsbad, CA), and transformed into Escherichia coli DH5α competent cells (Novagen, Darmstadt, Germany). A total of 10 recombinant plasmids from several independent subclones were fully sequenced on the Applied Biosystems 3730 automated sequencer (Foster City, CA) from both directions. The newly described transcript variants of NlHR3 and NlFTZ-F1 were submitted to GenBank.
ClustalW2 was used to perform a homologous sequence alignment of HR3 and FTZ-F1 proteins from Nilaparvata lugens, Drosophila melanogaster, Tribolium castaneum, Bombyx mori, Apis mellifera, Aedes aegypti, Pediculus humanus corporis, Blattella germanica, and Acyrthosiphon pisum (Larkin et al., 2007). The conserved domains were predicted by using the Simple Modular Architectural Research Tool (SMART; http://smart.embl-heidelberg.de/) and InterPro: protein sequence analysis and classification (http://www.ebi.ac.uk/interpro/).
2.4 Developmental- and tissue-specific expression profiles of NlHR3 and NlFTZ-F1
For temporal expression analysis of NlHR3 and NlFTZ-F1, total RNA samples were prepared from eggs; first (N1), second (N2), and third (N3) instar nymphs; each day of the fourth and fifth instar nymphs (N4D1, N4D2, N4D3, N5D1, N5D2, N5D3, and N5D4); and newly emerged (New-A) and 2-day-old female adults (AD2). To analyze the tissue-specific expression patterns, the different tissues were obtained by dissecting the fourth and fifth instar nymphs. The first group comprised the head (He), thorax (Th), and abdomen (Ab). The second group comprised the integument (In), wingbud (Wi), midgut (Mg), leg (Lg), and fat body (Fb). Quantitative real-time PCR (qRT-PCR) was conducted to estimate expression levels of NlHR3 and NlFTZ-F1 of various samples using internal control genes (RSP15 and RSP11), according to the published methods (Wang et al., 2018). All the qRT-PCR primers are shown in Supplementary Table S1. There were three independent replications of each biological sample with three technical replicates. Data were analyzed by the 2−ΔΔCT method (Livak and Schmittgen, 2001) and in accordance with the MIQE guidelines (Bustin et al., 2009).
2.5 20E treatment
The response of NlHR3 and NlFTZ-F1 to 20E application was determined by referring to previous methods (Gao et al., 2022). 20E was purchased from Sigma (Sigma-Aldrich, Shanghai, China). The fourth instar nymphs (0–12 h) were collected and treated with 0.2 μL 20E (0.1 μg/μL in acetone). After treatment with 20E, samples were taken at 6, 12, and 24 h after application to extract total RNA, and the expression of NlHR3 and NlFTZ-F1 was examined. Three replicates (10*3 nymphs, a total of 30 nymphs) were used to extract total RNA, and acetone was used as the control.
2.6 dsRNA synthesis and RNAi bioassay
The dsRNA synthesis and RNAi bioassay method were applied, as previously described (Li et al., 2018). The dsDNA fragments were amplified by RT-PCR using specific primers (Supplementary Table S1) and used as templates to synthesize dsRNA using the MEGAscript T7 High-Yield Transcription Kit (Ambion, Austin, TX, United States). The quality and concentration of the dsRNA were detected and kept at −80°C until further use. The dsRNA targeting the gene-encoding green fluorescence protein (dsGFP) served as a negative control.
RNA interference (RNAi) was performed by the FemtoJet microinjector (Eppendorf), as previously reported (Wang et al., 2018). Approximately 200 ng and 400 ng dsRNA were microinjected into each individual of the third and fifth instar nymphs, respectively (Xu et al., 2015). A total of 250 nymphs (10 replicates) were used for each treatment, including three survival evaluation replicates, three phenotypic evaluation replicates, three qRT-PCR verification replicates, and one backup replicate. QRT-PCR verification was conducted 3 days after injection.
2.7 Data analyses
Data were analyzed using Student’s t-test (the difference between two samples), Tukey’s test (the difference among three or more samples), and analysis of variance (ANOVA) by data processing system software (Tang and Zhang, 2013).
3 Results
3.1 Identification of the HR3 and FTZ-F1 transcripts of N. lugens
The cDNA of putative HR3 and FTZ-F1 transcripts in N. lugens was cloned and submitted to GenBank (OP937347, OP937348, KU928171.1, and KU928171.1). We identified two transcript variants of HR3 (NlHR3a and NlHR3b) that encoded 520 and 462 amino acid residues and two transcript variants of FTZ-F1 (NlFTZ-F1a and NlFTZ-F1b) that encoded 609 and 633 amino acid residues. The HR3 and FTZ-F1 family proteins contain two conserved nuclear receptor functional domains, among which the DNA-binding domain (DBD) at the amino terminal and the ligand-binding domain (LBD) at the carboxy terminal are highly conserved (Supplementary Figures S1A, S2A). The phylogenetic tree analysis showed that NlHR3 has a close relationship with that of A. pisum, and NlFTZ-F1 has a close relationship with that of A. pisum and P. humanus (Supplementary Figures S1C, S2C).
3.2 Expression profiles of NlHR3 and NlFTZ-F1
Developmental expression analysis showed that the transcript levels of NlHR3 were the highest in the first instar period (Figure 1A), while NlFTZ-F1 had two expression peaks at the later stages of the fourth and fifth instar periods (Figure1C). The expression profiles of NlHR3 and NlFTZ-F1 in different tissues are similar and relatively higher in the thorax and appendage of the nymphs (Figures 1B,D). The spatiotemporal data are compatible with the common idea that NlHR3 and NlFTZ-F1 play a vital role in the 20E signaling pathway.
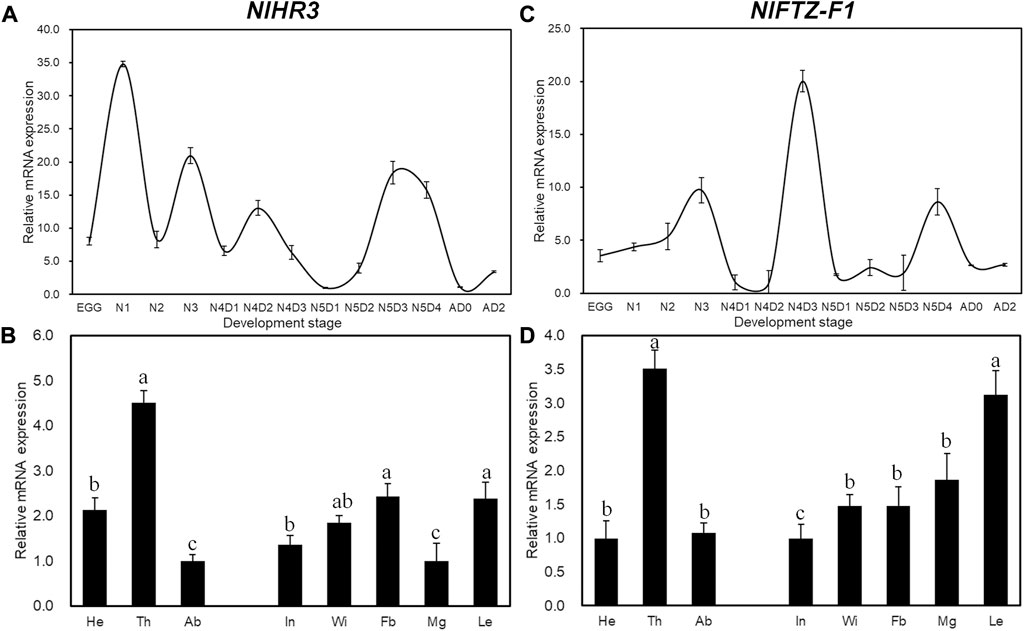
FIGURE 1. Temporal and spatial expression profiles of NlHR3 and NlFTZ-F1. (A, C) Relative expression levels of NlHR3 and NlFTZ-F1 at different developmental stages. N1 (the first instar nymph) to N5D4 (day 4 of the fifth instar nymph), New-A (newly emerged female adults), and AD2 (2-day-old female adults). (B, D): NlHR3 and NlFTZ-F1 relative expression levels of the head (He), thorax (Th), abdomen (Ab), integument (In), wingbud (Wi), midgut (Mg), leg (Le), and fat body (Fb) of nymphs (fourth–fifth instar). The standard error (SE) was determined from three independent biological replicates, each with three technical replications. Different letters indicate a significant difference at a p-value < 0.05 (Tukey’s test).
3.3 Transcriptional responses to 20E
In order to test whether NlHR3 and NlFTZ-F1 are induced by 20E, the fourth instar nymphs were treated with 20E or acetone (control) for 6, 12, and 24 h. The expression of NlHR3 significantly increased by 7.4, 11.4, and 8.3 fold (p-value < 0.01) after 6, 12, and 24 h of 20E application, respectively, compared to the acetone control (Figure 2A), while the expression of NlFTZ-F1 significantly increased 10.7-, 8.9-, and 12.1-fold (p-value < 0.01) (Figure 2B).
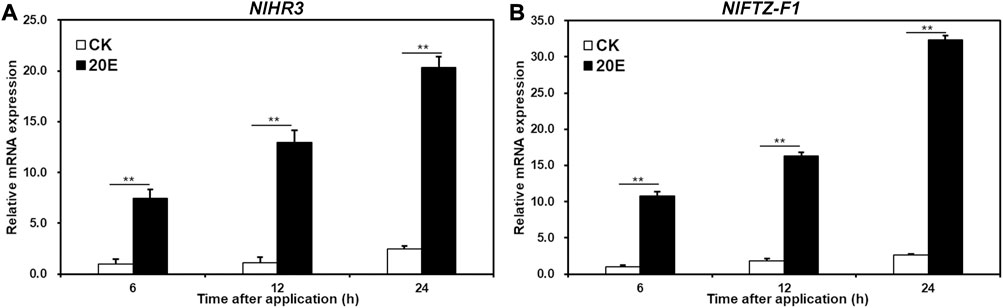
FIGURE 2. Transcriptional responses of NlHR3 and NlFTZ-F1 to the 20E signaling pathway. (A) Effect of 20E on the expression of NlHR3. (B) Effect of ecdysone on the expression of NlHR3. SE was determined from three independent biological replicates, each with three technical replications. ** indicates a significant difference at a p-value < 0.01 (Student’s t-test).
3.4 Regulation network between HR3, FTZ-F1, and the hormone signaling pathway
To explore the regulatory network between HR3, FTZ-F1, and the hormone signaling pathway, dsRNA of NlHR3, NlFTZ-F1, NlCYP314A, and NlKr-h1 (Krüppel homolog 1) were injected into the third instar nymphs. Then, the transcript levels of NlHR3, NlFTZ-F1, NlCYP314A, and NlKr-h1 were measured 3 days later. In the nymphs injected with dsNlHR3, the transcript levels of NlCYP314A were not significantly altered, but NlFTZ-F1 was significantly decreased by 54.8% (p-value < 0.01) and NlKr-h1 was significantly increased by 739.7% (p-value <0.01) compared to the dsGFP control (Figure 3A).
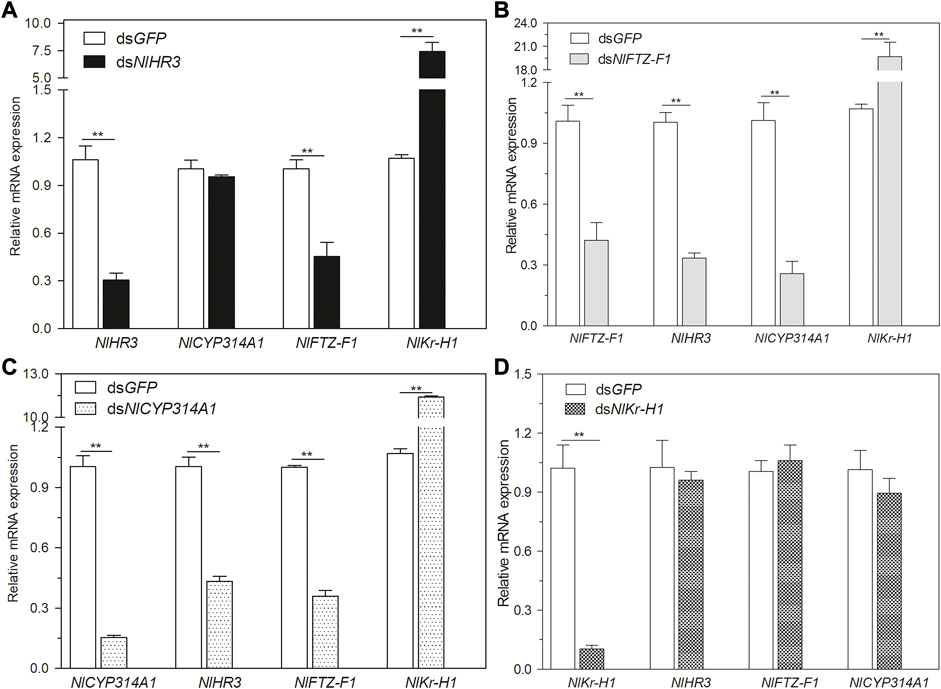
FIGURE 3. Relationship between NlHR3, NlFTZ-F1, and the hormone signaling pathway. (A) Downregulation of NlHR3 affected 20E and JH signaling pathway gene expression. (B) Downregulation of NlFTZ-F1 affected 20E and JH signaling pathway gene expression. (C) Downregulation of NlCYP314A1 affected NlHR3 and NlFTZ-F1 expression. (D) Downregulation of NlKr-H1 did not affect NlHR3 and NlFTZ-F1 expression. The SE was determined from three independent biological replicates, each with three technical replications. ** indicates a significant difference at a p-value < 0.01 (Student’s t-test).
In the nymphs injected with dsNlFTZ-F1, the transcript levels of NlCYP314A and NlHR3 were significantly decreased by 74.2% and 80.3% (p-value <0.01), respectively, and NlKr-h1 was significantly increased by 1771.3% (p-value <0.01) (Figure 3B). In the nymphs injected with dsNlCYP314A (an ecdysone synthesis key gene), the transcript levels of NlHR3 and NlFTZ-F1 were significantly decreased by 56.6% and 64.1% (p-value <0.01), respectively (Figure 3C), while in the nymphs injected with dsNlKr-h1 (a juvenile hormone response key gene), the transcript levels of NlHR3 and NlFTZ-F1 were not significantly different from that of the control (Figure 3D). Ecdysone receptor (EcR) is the starting point of an ecdysone cascade reaction and the upstream of HR3 and FTZ-F1. We analyzed the expression level of NlEcR, but there was no significant difference after the knockdown of NlHR3 and NlFTZ-F1 (Supplementary Figure S3).
In summary, the transcript levels of 20E biosynthetic and cascade regulated genes were decreased significantly by silencing NlFTZ-F1, while only the ecdysone cascade regulated genes were decreased significantly by silencing NlHR3. The transcript level of the JH response key gene was increased significantly by silencing NlHR3 and NlFTZ-F1. The transcript level of NlFTZ-F1 was decreased significantly by silencing NlHR3.
3.5 Effect of expression silencing of NlHR3 and NlFTZ-F1 on nymph–nymph molting
In order to investigate the role of NlHR3 and NlFTZ-F1 in nymph–nymph molting, dsNlHR3 and dsNlFTZ-F1 from the common region (marked with black lines in Supplementary Figure S1) were synthesized in vitro and injected into the third instar nymphs. Compared to the control, the transcript levels of NlHR3 and NlFTZ-F1 were significantly decreased by 69.6% and 57.8% (p-value <0.01), respectively, 48 h after injecting with dsNlHR3 (Figure 4D).
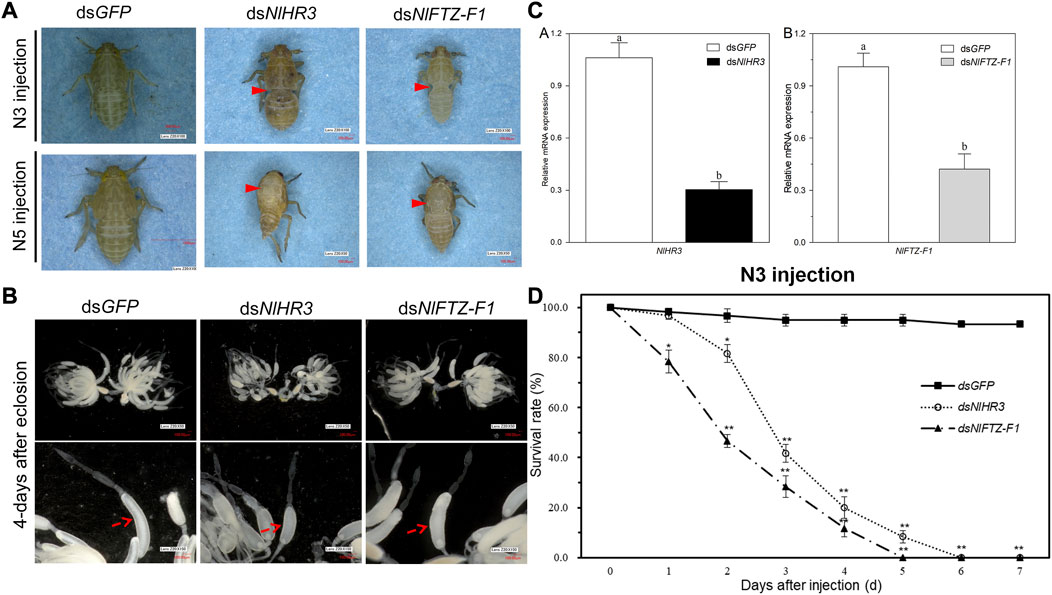
FIGURE 4. Phenotypes and the survival rate of N. lugens subjected to dsNlHR3 and dsNlFTZ-F1 injection. (A) Abnormal appearance of the phenotype of N. lugens subjected to dsNlHR3 and dsNlFTZ-F1 injection. (B) Abnormal appearance of the ovary phenotype of N. lugens subjected to dsNlHR3 and dsNlFTZ-F1 injection. (C) Relative expression level of NlHR3 and NlFTZ-F1 subjected to dsNlHR3 and dsNlFTZ-F1 injection in the third instar nymphs. (D) Survival rate of N. lugens subjected to dsNlHR3 and dsNlFTZ-F1 injection in the third instar nymphs. The SE was determined from three independent biological replicates, each with three technical replications. Different letters indicate a significant difference at a p-value < 0.05 (Student’s t-test). * and ** indicate a significant difference at a p-value < 0.05 or < 0.01 (Student’s t-test).
Upon injecting with dsRNA into the early third instar nymphs, the knockdown of NlHR3 resulted in a significantly lower survival rate (81.7%; p-value < 0.05), which started on the second day after dsRNA injection (Figure 4D). The development of these nymphs was inhibited on the third instar stage, and all individuals (n = 60 out of 60) were unable to molt normally into the fourth instar stage and eventually died on the sixth day after dsRNA injection (Figures 4A,D). The knockdown of NlFTZ-F1 resulted in a significantly lower survival rate (78.3%; p-value < 0.05) which started on the first day after dsRNA injection (Figure 4D). The insects injected with dsRNA, targeting NlFTZ-F1, exhibited lethal phenotypes at approximately 3 days after injection, and the development was inhibited on the third nymph–nymph molting transition; as a result, the insect bodies became slender and extended. The insects then failed to shed their old cuticle and died (Figure 4A). In contrast, the dsGFP-treated nymphs successfully completed all nymph–nymph molting transition, and the survival rate on the seventh day after injection was 93.3% (Figures 4A,D). Furthermore, NlFTZ-F1 silencing caused a lower survival rate than NlHR3 from the second day after injection. The survival rate decreased to 0% on the fifth day after downregulating NlHR3, while all individuals died on the sixth day for downregulating NlFTZ-F1 (Figure 4D).
3.6 Effect of expression silencing of NlHR3 and NlFTZ-F1 on the nymph–adult transition
In order to investigate the role of NlHR3 and NlFTZ-F1 in nymph–adult molting, dsRNAs were injected into the fifth instar nymphs. The transcript levels of NlHR3 and NlFTZ-F1 were significantly decreased from the controls. The knockdown of NlHR3 and NlFTZ-F1 resulted in a significantly higher mortality rate (42.7% and 53.3%, p-value < 0.05) on the fourth day after dsRNA injection, and these individuals were unable to molt normally into adults; the old cuticle of the notum was split open, and the insects then failed to shed their old cuticle and died (Figure 4A). The individuals that molt normally into female adults were dissected to observe the development of ovaries. The ovaries of female individuals injected with dsNlHR3 and dsNlFTZ-F1 were deficient of oocytes and abnormally formed. The oocytes of individuals injected with dsNlHR3 were smaller than those of the dsGFP, and the egg shell of oocytes in individuals injected with dsNlFTZ-F1 had no luster and elasticity (Figure 4B), while the ovaries of those injected with dsGFP were full of oocytes (banana-shaped), and the egg shell of oocytes had luster and elasticity (Figure 4B). No offspring was produced by female individuals injected with dsNlHR3 and dsNlFTZ-F1.
4 Discussion
In the present study, we cloned and characterized HR3 and FTZ-F1 genes in N. lugens, and their temporal and spatial expression profiles were analyzed. This is the first report of splice variants of FTZ-F1 in N. lugens. The HR3 and FTZ-F1 genes have splice variants that are widely distributed in insects, including D. melanogaster (Dubrovsky et al., 2011), A. aegypti (Li et al., 2000), Manduca sexta (Weller et al., 2001), T. castaneum (Tan and Palli, 2008), B. germanica (Cruz et al., 2008), and L. decemlineata (Liu et al., 2014). Many nuclear receptor factors are induced and temporally expressed in response to 20E (Liu et al., 2014; Sultan et al., 2014; Li et al., 2015; Zhang et al., 2022). Among them, FTZ-F1 was expressed after a pulse of 20E during the late nymph stage in almost all organs and is essential for molting and metamorphosis. In N. lugens, NlFTZ-F1 was remarkably induced before or right after each molt in the fourth and fifth instar nymph stages and was induced by 20E. This temporally specific expression was in concert with the cyclical fluctuation of the 20E titer detected in a previous study (Liu et al., 2014; Zhao et al., 2019; Zhang et al., 2022).
Our results showed that the knockdown of NlHR3 and NlFTZ-F1 in nymphs severely impaired nymph molting and metamorphosis, and RNAi nymph resulted in lethal phenotypes and abnormally formed ovaries. Ecdysones regulate molting and reproduction of N. lugens (Zhou et al., 2020). In this study, the expression of NlHR3 and NlFTZ-F1 was significantly increased after 20E treatment (Figure 2), suggesting HR3 and FTZ-F1 were induced by 20E (Liu et al., 2014; Guo et al., 2015). NlCYP314A1 is a key gene of the 20E biosynthetic pathway and played an important role in the nymphal development (Li et al., 2017). By knocking down NlCYP314A1 in N. lugens, NlHR3 and NlFTZ-F1 mRNA levels were decreased (Figure 3C). These data demonstrated that NlHR3 and NlFTZ-F1 can participate in the growth and development regulated by 20E. Similar results have been reported in other insects. The expression pattern of DmHR3 is related to the titer of 20E in D. melanogaster, and 20E promotes the expression of DmHR3 (Carney et al., 1997). In the larvae of B. germanica, 20E directly regulates the expression of BgHR3 and ecdysis was inhibited after the knockdown of BgHR3 (Cruz et al., 2008). In L. decemlineata, LdHR3 regulated the pupation process by responding to 20E (Guo et al., 2015). D. melanogaster larvae died due to severe ecdysis defects, following silencing DmFTZ-F1 (Yamada et al., 2000). In B. germanica, the knockdown of BgFTZ-F1 led to growth retardation and molting failure (Cruz et al., 2008). Recently, the knockdown of HaFTZ-F1 severely damaged larval ecdysis in Helicoverpa armigera (Zhang et al., 2021), and the knockdown of HvFTZ-F1 impaired molting, pupation, and reproduction in Henosepilachna vigintioctopunctata (Liu et al., 2022). The result from this study also suggested that NlHR3 and NlFTZ-F1 could be a potential target for the RNAi-mediated pest control against N. lugens because of the high mortality rate after RNAi. However, the unintended effects of dsNlHR3 and dsNlFTZ-F1 on target and non-target organisms should be investigated before the commercialization of RNAi-based pest control (Pan et al., 2020; He et al., 2022).
Furthermore, the knockdown of NlFTZ-F1 resulted in varying degrees of downregulations of NlCYP314A1 (20E-induced biosynthetic genes) and NlHR3 (20E-induced cascade genes) (Figure 3B). In D. melanogaster, the expression levels of two steroidogenic enzymes, phantom (Phm) and disembodied (Dib), were reduced in DmFTZ-F1 mutant ring gland cells (Parvy et al., 2005). BgFTZ-F1 regulates the onset of production of ecdysteroids at mid-nymphal stages in B. germanica (Cruz et al., 2008). RNAi-mediated knockdown of LdFTZ-F1 significantly repressed the transcription of LdPHM, LdDIB, and LdSHD, lowered the 20E titer in L. decemlineata (Liu et al., 2014). In H. armigera, the knockdown of HaFTZ-F1 lowered the intrinsic 20E titer, and reduced the expression of ecdysone receptors and 20E cascade regulated genes HaBrC and HaE75A (Zhang et al., 2021). These results indicated that the knockdown of FTZ-F1 resulted in irreversible changes to 20E biosynthetic and downstream cascade regulated genes, thereby impairing molting and metamorphosis. However, how FTZ-F1 regulates 20E biosynthetic and cascade regulated genes requires further study.
Kr-h1 is one of the crucial effectors that mediate the interactions between JH and 20E signaling pathways (He and Zhang, 2022). The expression level of NlKr-H1 was significantly increased after the knockdown of NlHR3 and NlFTZ-F1, suggesting that NlKr-H1 was negatively regulated by NlHR3 and NlFTZ-F1 (Figures 3A,B). The knockdown of NlHR3 and NlFTZ-F1 resulted in the reduction of 20E biosynthetic gene expression. These results led us to hypothesize that NlHR3 and NlFTZ-F1 could negatively regulate the JH signaling pathway by affecting 20E biosynthetic genes and the 20E titer. On the other hand, the mutual inhibition of the 20E- and JH-response pathways plays an important role in both embryonic and postembryonic phases (Uyehara et al., 2017; Liu et al., 2018; Truman, 2019; He and Zhang, 2022). Therefore, the interference of these 20E cascade regulated genes would lead to the weakening of the 20E cascade and the enhancement of the JH cascade, disrupting the normal synergy and accurate action time of the two hormone pathways and ultimately affecting the molting, metamorphosis, and reproduction processes of the brown planthopper.
5 Conclusion
Taken together, this is the first detailed report which shows that the nuclear transcription factors NlHR3 and NlFTZ-F1 are involved in the regulation of molting and reproduction in N. lugens. NlHR3 and NlFTZ-F1 regulate molting and reproduction by mediating the intrinsic 20E and JH signaling pathways. These results will deepen our understanding of the action mechanisms of HR3 and FTZ-F1 in insects. In addition, NlHR3 and NlFTZ-F1 could properly be exploited as potential target genes for developing RNAi-based pesticides to control N. lugens.
Data availability statement
The original contributions presented in the study are included in the article/Supplementary Materials; further inquiries can be directed to the corresponding authors.
Author contributions
Conception and design of the experiments: KLi, ZP, and DZ. Execution of the experiments: KLi, MM, WC, XL, and AC. Data analysis: KLi and XW. Manuscript writing and revision: KLi, KLiu, and DZ.
Funding
This research was supported by the National Natural Science Foundation of China (32102210), the National Key R&D Program of China (2021YFD1401100), the Hunan Agricultural Science and Technology Innovation Fund Project (2022CX06), and the Open Project Program (20210302) of State Key Laboratory of Rice Biology.
Conflict of interest
The authors declare that the research was conducted in the absence of any commercial or financial relationships that could be construed as a potential conflict of interest.
Publisher’s note
All claims expressed in this article are solely those of the authors and do not necessarily represent those of their affiliated organizations, or those of the publisher, the editors, and the reviewers. Any product that may be evaluated in this article, or claim that may be made by its manufacturer, is not guaranteed or endorsed by the publisher.
Supplementary material
The Supplementary Material for this article can be found online at: https://www.frontiersin.org/articles/10.3389/fphys.2023.1123583/full#supplementary-material
SUPPLEMENTARY FIGURE S1 | Splice variant structure comparison. Sequence alignment and phylogenetic tree of HR3. (A) Prediction of conservative domains and comparison of different transcript structures of NlHR3. (B) HR3s from NlHR3A and NlHR3B (Nilaparvata lugens), AaHR3 (Aedes aegypti, AAF36970), AgHR3 (Anopheles gambiae, EAA14853), AmHR3 (Apis mellifera, XP_006570914), ApHR3X1 (Acyrthosiphon pisum, XP_003240875), ApHR3X2 (Acyrthosiphon pisum, XP_008189753), ApHR3X3 (Acyrthosiphon pisum, XP_008189754), ApHR3X4 (Acyrthosiphon pisum, XP_008189756), BmHR3 (Bombyx mori, NP_001037012), BmHR3X1 (Bombyx mori, XP_012551821), NvHR3 (Nasonia vitripennis, XP_008202385), TcHR3X1 (Tribolium castaneum, XP_974561), TcHR3X2 (Tribolium castaneum, XP_008195824), and TcHR3X3 (Tribolium castaneum, XP_008195825). Amino acids with 100, > 80, and > 60% conservations are shaded in black, dark gray, and light gray, respectively. Gaps have been introduced to permit alignments. (C) Phylogenetic tree of HR3s of N. lugens and other insects constructed by the neighbor-joining method based on the amino acid sequence. The test of phylogeny was carried out using a bootstrap analysis of 1000 replications; bootstrap values > 50% are shown on each node of the tree.
SUPPLEMENTARY FIGURE S2 | Splice variant structure comparison. Sequence alignment and phylogenetic tree of FTZ-F1. (A) Prediction of conservative domains and comparison of different transcript structures of NlFTZ-F1. (B) FTZ-F1s from NlFTZ-F1a and NlFTZ-F1b (Nilaparvata lugens), PhFTZ-F1 (Pediculus humanus corporis, XP_002430379), TcFTZ-F1 (Tribolium castaneum, EFA01263), TcFTZ-F1X1 (Tribolium castaneum, XP_008191373), TcFTZ-F1X2 (Tribolium castaneum, XP_008191374), TcFTZ-F1X3 (Tribolium castaneum, XP_008191375), ApFTZ-F1X1 (Acyrthosiphon pisum, XP_008183294), ApFTZ-F1X2 (Acyrthosiphon pisum, XP_008183295), BgFTZ-F1 (Blattella germanica, CAQ57670), AaFTZ-F1a (Aedes aegypti, XP_001654601), AaFTZ-F1b (Aedes aegypti, CAO79104), AgFTZ-F1a (Anopheles gambiae, EAA11812), AgFTZ-F1b (Anopheles gambiae, EAL39882), BmFTZ-F1 (Bombyx mori, NP_001037528), DmFTZ-F1 (Drosophila melanogaster, AAA28542), DmFTZ-F1b (Drosophila melanogaster, AAA28915), LdFTZ-F1a (Leptinotarsa decemlineata, AJF93908), and LdFTZ-F1b (Leptinotarsa decemlineata, AJF93909). Amino acids with 100, > 80, and > 60% conservations are shaded in black, dark gray, and light gray, respectively. Gaps have been introduced to permit alignments. (C) Phylogenetic tree of FTZ-F1s of N. lugens and other insects constructed by the neighbor-joining method based on the amino acid sequence. The test of phylogeny was carried out using a bootstrap analysis of 1000 replications; bootstrap values > 50% are shown on each node of the tree.
SUPPLEMENTARY FIGURE S3 | Effect of the knockdown of NlHR3 and NlFTZ-F1 on the expression of NlEcR. (A) Effect of knockdown of NlHR3 on the expression of NlEcR. (B) Effect of knockdown of NlFTZ-F1 on the expression of NlEcR. SE was determined from three independent biological replicates, each with three technical replications.
SUPPLEMENTARY TABLE S1 | Primers used for RT-PCR, dsRNA synthesis, and qRT-PCR.
References
Bustin, S. A., Benes, V., Garson, J. A., Hellemans, J., Huggett, J., Kubista, M., et al. (2009). The MIQE guidelines: Minimum information for publication of quantitative real-time PCR experiments. Clin. Chem. 55 (4), 611–622. doi:10.1373/clinchem.2008.112797
Carney, G. E., Wade, A. A., Sapra, R., Goldstein, E. S., and Bender, M. (1997). DHR3, an ecdysone-inducible early-late gene encoding a Drosophila nuclear receptor, is required for embryogenesis. Proc. Natl. Acad. Sci. 94 (22), 12024–12029. doi:10.1073/pnas.94.22.12024
Cheng, X., Wu, J., and Ma, J. (2003). Brown planthopper: Occurrence and control in Chinese. Beijing: China Agricultural Press.
Cruz, J., Martín, D., and Bellés, X. (2007). Redundant ecdysis regulatory functions of three nuclear receptor HR3 isoforms in the direct-developing insect Blattella germanica. Mech. Dev. 124 (3), 180–189. doi:10.1016/j.mod.2006.12.003
Cruz, J., Nieva, C., Mané-Padrós, D., Martín, D., and Bellés, X. (2008). Nuclear receptor BgFTZ-F1 regulates molting and the timing of ecdysteroid production during nymphal development in the hemimetabolous insect Blattella germanica. Dev. Dyn. 237 (11), 3179–3191. doi:10.1002/dvdy.21728
Dubrovsky, E. B., Dubrovskaya, V. A., Bernardo, T., Otte, V., Difilippo, R., and Bryan, H. (2011). The Drosophila FTZ-F1 nuclear receptor mediates juvenile hormone activation of E75A gene expression through an intracellular pathway. Am. Soc. Biochem. Mol. Biol. 286 (38), 33689–33700. doi:10.1074/jbc.M111.273458
Dubrovsky, E. B. (2005). Hormonal cross talk in insect development. Trends Endocrinol. Metabolism 16 (1), 6–11. doi:10.1016/j.tem.2004.11.003
Eystathioy, T., Swevers, L., and Iatrou, K. (2001). The orphan nuclear receptor BmHR3A of Bombyx mori: Hormonal control, ovarian expression and functional properties. Mech. Dev. 103 (1), 107–115. doi:10.1016/S0925-4773(01)00335-5
Gao, H., Jiang, X., Zheng, S., Li, Y., and Lin, X. (2022). Role of groucho and groucho1-like in regulating metamorphosis and ovary development in Nilaparvata lugens (stål). Int. J. Mol. Sci. 23 (3), 1197. doi:10.3390/ijms23031197
Guo, W.-C., Liu, X.-P., Fu, K.-Y., Shi, J.-F., Lü, F.-G., and Li, G.-Q. (2015). Functions of nuclear receptor HR3 during larval-pupal molting in Leptinotarsa decemlineata (Say) revealed by in vivo RNA interference. Insect Biochem. Mol. Biol. 63, 23–33. doi:10.1016/j.ibmb.2015.05.010
He, L., Huang, Y., and Tang, X. (2022). RNAi-based pest control: Production, application and the fate of dsRNA. Front. Bioeng. Biotechnol. 10, 1080576. doi:10.3389/fbioe.2022.1080576
He, Q., and Zhang, Y. (2022). Kr-h1, a cornerstone gene in insect life history. Front. Physiology 13, 905441. doi:10.3389/fphys.2022.905441
Heong, K. L., and Hardy, B. (2009). Planthopphers: New threats to the sustainability of intensive rice production systems in asia. Los Baños, Philippines: International Rice Research Institute IRRI.
King-Jones, K., and Thummel, C. S. (2005). Nuclear receptors — A perspective from Drosophila. Nat. Rev. Genet. 6 (4), 311–323. doi:10.1038/nrg1581
Lala, D. S., Rice, D. A., and Parker, K. L. (1992). Steroidogenic factor I, a key regulator of steroidogenic enzyme expression, is the mouse homolog of fushi tarazu-factor I. Mol. Endocrinol. 6 (8), 1249–1258. doi:10.1210/mend.6.8.1406703
Lam, G., Hall, B. L., Bender, M., and Thummel, C. S. (1999). DHR3 is required for the prepupal–pupal transition and differentiation of adult structures during Drosophila metamorphosis. Dev. Biol. 212 (1), 204–216. doi:10.1006/dbio.1999.9343
Lam, G. T., Jiang, C., and Thummel, C. S. (1997). Coordination of larval and prepupal gene expression by the DHR3 orphan receptor during Drosophila metamorphosis. Development 124 (9), 1757–1769. doi:10.1242/dev.124.9.1757
Larkin, M. A., Blackshields, G., Brown, N. P., Chenna, R., McGettigan, P. A., McWilliam, H., et al. (2007). Clustal W and clustal X version 2.0. Bioinformatics 23 (21), 2947–2948. doi:10.1093/bioinformatics/btm404
Li, C., Kapitskaya, M. Z., Zhu, J., Miura, K., Segraves, W., and Raikhel, A. S. (2000). Conserved molecular mechanism for the stage specificity of the mosquito vitellogenic response to ecdysone. Dev. Biol. 224 (1), 96–110. doi:10.1006/dbio.2000.9792
Li, K., Guo, E. E., Hossain, M. S., Li, Q. R., Cao, Y., Tian, L., et al. (2015). Bombyx E75 isoforms display stage- and tissue-specific responses to 20-hydroxyecdysone. Sci. Rep. 5 (1), 12114. doi:10.1038/srep12114
Li, K. L., Fu, Q., Wang, W. X., Lai, F. X., and Wan, P. J. (2017). Molecular cloning and functional characterization of Halloween genes involved in ecdysteroid biosynthetic in Nilaparvata lugens. Acta Entomologica Sinica China 60 (10), 1129–1140. doi:10.16380/j.kcxb.2017.10.004
Li, K. L., Yuan, S. Y., Nanda, S., Wang, W. X., Lai, F. X., Fu, Q., et al. (2018). The roles of E93 and Kr-h1 in metamorphosis of Nilaparvata lugens. Front. Physiology 9, 1677. doi:10.3389/fphys.2018.01677
Liu, S. N., Li, K., Gao, Y., Liu, X., Chen, W. T., Ge, W., et al. (2018). Antagonistic actions of juvenile hormone and 20-hydroxyecdysone within the ring gland determine developmental transitions in Drosophila. Proc. Natl. Acad. Sci. U. S. A. 115 (1), 139–144. doi:10.1073/pnas.1716897115
Liu, X. P., Fu, K. Y., Lü, F.-G., Meng, Q. W., Guo, W. C., and Li, G. Q. (2014). Involvement of FTZ-F1 in the regulation of pupation in Leptinotarsa decemlineata (Say). Insect Biochem. Mol. Biol. 55, 51–60. doi:10.1016/j.ibmb.2014.10.008
Liu, Z. Q., Nanda, S., Yang, C. X., Chen, S. M., Guo, M. J., Khan, M. M., et al. (2022). RNAi suppression of the nuclear receptor FTZ-F1 impaired ecdysis, pupation, and reproduction in the 28-spotted potato ladybeetle, Henosepilachna vigintioctopunctata. Pesticide Biochem. Physiology 182, 105029. doi:10.1016/j.pestbp.2021.105029
Livak, K. J., and Schmittgen, T. D. (2001). Analysis of relative gene expression data using real-time quantitative PCR and the 2-ΔΔCT method. Methods 25 (4), 402–408. doi:10.1006/meth.2001.1262
Nakagawa, Y., and Henrich, V. C. (2009). Arthropod nuclear receptors and their role in molting. FEBS J. 276 (21), 6128–6157. doi:10.1111/j.1742-4658.2009.07347.x
Pan, H. P., Yang, X. W., Romeis, J., Siegfried, B. D., and Zhou, X. G. (2020). Dietary RNAi toxicity assay exhibits differential responses to ingested dsRNAs among lady beetles. Pest Manag. Sci. 76 (11), 3606–3614. doi:10.1002/ps.5894
Parvy, J. P., Blais, C., Bernard, F., Warren, J. T., Petryk, A., Gilbert, L. I., et al. (2005). A role for βFTZ-F1 in regulating ecdysteroid titers during post-embryonic development in Drosophila melanogaster. Dev. Biol. 282 (1), 84–94. doi:10.1016/j.ydbio.2005.02.028
Parvy, J. P., Wang, P., Garrido, D., Maria, A., Blais, C., Poidevin, M., et al. (2014). Forward and feedback regulation of cyclic steroid production in Drosophila melanogaster. Development 141 (20), 3955–3965. doi:10.1242/dev.102020
Pierceall, W. E., Li, C., Biran, A., Miura, K., Raikhel, A. S., and Segraves, W. A. (1999). E75 expression in mosquito ovary and fat body suggests reiterative use of ecdysone-regulated hierarchies in development and reproduction. Mol. Cell. Endocrinol. 150 (1), 73–89. doi:10.1016/S0303-7207(99)00022-2
Ruaud, A.-F., Lam, G., and Thummel, C. S. (2010). The Drosophila nuclear receptors DHR3 and βFTZ-F1 control overlapping developmental responses in late embryos. Development 137 (1), 123–131. doi:10.1242/dev.042036
Siaussat, D., Bozzolan, F., Queguiner, I., Porcheron, P., and Debernard, S. (2004). Effects of juvenile hormone on 20-hydroxyecdysone-inducible EcR, HR3, E75 gene expression in imaginal wing cells of Plodia interpunctella lepidoptera. Eur. J. Biochem. 271 (14), 3017–3027. doi:10.1111/j.1432-1033.2004.04233.x
Sultan, A. R. S., Oish, Y., and Ueda, H. (2014). Function of the nuclear receptor FTZ-F1 during the pupal stage in Drosophila melanogaster. Dev. Growth and Differ. 56 (3), 245–253. doi:10.1111/dgd.12125
Swevers, L., Eystathioy, T., and Iatrou, K. (2002). The orphan nuclear receptors BmE75A and BmE75C of the silkmoth Bombyx mori: Hornmonal control and ovarian expression. Insect Biochem. Mol. Biol. 32 (12), 1643–1652. doi:10.1016/S0965-1748(02)00104-2
Tan, A. J., and Palli, S. R. (2008). Identification and characterization of nuclear receptors from the red flour beetle, Tribolium castaneum. Insect Biochem. Mol. Biol. 38 (4), 430–439. doi:10.1016/j.ibmb.2007.09.012
Tang, L., Zhang, C., Liu, L., Feng, Q. L., and Zheng, S. C. (2011). Cloning and expression of the nuclear transcription factor SlβFTZ-F1 cDNA from Spodoptera litura. Insect Sci. 18 (6), 635–644. doi:10.1111/j.1744-7917.2011.01412.x
Tang, Q. Y., and Zhang, C. X. (2013). Data Processing System (DPS) software with experimental design, statistical analysis and data mining developed for use in entomological research. Insect Sci. 20 (2), 254–260. doi:10.1111/j.1744-7917.2012.01519.x
Truman, J. W. (2019). The evolution of insect metamorphosis. Curr. Biol. 29 (23), R1252–R1268. doi:10.1016/j.cub.2019.10.009
Uyehara, C., Nystrom, S., Niederhuber, M., Leatham-Jensen, M., Ma, Y., Buttitta, L., et al. (2017). Hormone-dependent control of developmental timing through regulation of chromatin accessibility. Genes and Dev. 31, 862–875. doi:10.1101/gad.298182.117
Wan, P. J., Yuan, S. Y., Tang, Y. H., Li, K. L., Yang, L., Fu, Q., et al. (2015). Pathways of amino acid degradation in Nilaparvata lugens (stål) with special reference to lysine-ketoglutarate reductase/saccharopine dehydrogenase (LKR/SDH). PLoS One 10 (5), e0127789. doi:10.1371/journal.pone.0127789
Wang, W. X., Wan, P. J., Lai, F. X., Zhu, T. H., and Fu, Q. (2018). Double-stranded RNA targeting calmodulin reveals a potential target for pest management of Nilaparvata lugens. Pest Manag. Sci. 74 (7), 1711–1719. doi:10.1002/ps.4865
Weller, J., Sun, G. C., Zhou, B., Lan, Q., Hiruma, K., and Riddiford, L. M. (2001). Isolation and developmental expression of two nuclear receptors, MHR4 and βFTZ-F1, in the tobacco hornworm, Manduca sexta. Insect Biochem. Mol. Biol. 31 (8), 827–837. doi:10.1016/S0965-1748(00)00188-0
Xu, H. J., Xue, J., Lu, B., Zhang, X. C., Zhuo, J. C., He, S. F., et al. (2015). Two insulin receptors determine alternative wing morphs in planthoppers. Nature 519 (7544), 464–467. doi:10.1038/nature14286
Xu, J. J., Tan, A. J., and Palli, S. R. (2010). The function of nuclear receptors in regulation of female reproduction and embryogenesis in the red flour beetle, Tribolium castaneum. J. Insect Physiology 56 (10), 1471–1480. doi:10.1016/j.jinsphys.2010.04.004
Xue, J., Zhou, X., Zhang, C. X., Yu, L. L., Fan, H. W., Wang, Z., et al. (2014). Genomes of the rice pest Brown planthopper and its endosymbionts reveal complex complementary contributions for host adaptation. Genome Biol. 15 (12), 521. doi:10.1186/s13059-014-0521-0
Yamada, M., Thirose, S., Gsuzuki, E. H., LavorGna, G., and Ueda, H. (2000). Temporally restricted expression of transcription factor betaFTZ-F1: Significance for embryogenesis, molting and metamorphosis in Drosophila melanogaster. Development 127 (23), 5083–5092. doi:10.1242/dev.127.23.5083
Zhang, W. N., Ma, L., Liu, X. Y., Peng, Y. C., Liang, G. M., and Xiao, H. J. (2021). Dissecting the roles of FTZ-F1 in larval molting and pupation, and the sublethal effects of methoxyfenozide on Helicoverpa armigera. Pest Manag. Sci. 77 (3), 1328–1338. doi:10.1002/ps.6146
Zhang, Y. W., Zheng, S. W., Li, Y., Jiang, X. J., Gao, H., and Lin, X. D. (2022). The function of Nilaparvata lugens (Hemiptera: Delphacidae) E74 and its interaction with βFtz-F1. J. Insect Sci. 22 (3), 15. doi:10.1093/jisesa/ieac041
Zhao, X. M., Qin, Z. Y., Liu, W. M., Liu, X. J., Moussian, B., Ma, E. B., et al. (2018). Nuclear receptor HR3 controls locust molt by regulating chitin synthesis and degradation genes of Locusta migratoria. Insect Biochem. Mol. Biol. 92, 1–11. doi:10.1016/j.ibmb.2017.11.001
Zhao, X. M., Qin, Z. Y., Zhang, J., Yang, Y., Jia, P., Yang, Q., et al. (2019). Nuclear receptor hormone receptor 39 is required for locust moulting by regulating the chitinase and carboxypeptidase genes. Insect Mol. Biol. 28, 537–549. doi:10.1111/imb.12569
Keywords: HR3, FTZ-F1, Nilaparvata lugens, molting, reproduction
Citation: Li K, Liu K, Wang X, Ma M, Luo X, Chen W, Chen A, Peng Z and Zhang D (2023) Role of nuclear receptors NlHR3 and NlFTZ-F1 in regulating molting and reproduction in Nilaparvata lugens (stål). Front. Physiol. 14:1123583. doi: 10.3389/fphys.2023.1123583
Received: 14 December 2022; Accepted: 23 February 2023;
Published: 15 March 2023.
Edited by:
Bin Tang, Hangzhou Normal University, ChinaReviewed by:
Yuichiro Suzuki, Wellesley College, United StatesRobert Farkas, Slovak Academy of Sciences (SAS), Slovakia
Copyright © 2023 Li, Liu, Wang, Ma, Luo, Chen, Chen, Peng and Zhang. This is an open-access article distributed under the terms of the Creative Commons Attribution License (CC BY). The use, distribution or reproduction in other forums is permitted, provided the original author(s) and the copyright owner(s) are credited and that the original publication in this journal is cited, in accordance with accepted academic practice. No use, distribution or reproduction is permitted which does not comply with these terms.
*Correspondence: Kailong Li, bGFubnVvbGtsQGZveG1haWwuY29t; Deyong Zhang, ZGV5b25nemhhbmcyMDE1QDE2My5jb20=
†ORCID ID: Kailong Li, orcid.org/0000-0003-2386-6897