Corrigendum: Effects of imidacloprid-induced hormesis on the development and reproduction of the rose-grain aphid Metopolophium dirhodum (Hemiptera: aphididae)
- 1State Key Laboratory for Biology of Plant Disease and Insect Pests, Institute of Plant Protection, Chinese Academy of Agricultural Sciences, Beijing, China
- 2School of Resource and Environmental Sciences, Henan Institute of Science and Technology, Xinxiang, China
- 3Scientific Observing and Experimental Station of Crop Pests in Guilin, Ministry of Agriculture, Guilin, China
Field populations of insect pests are affected by sub-lethal doses of insecticides, leading to hormesis. Imidacloprid is a neonicotinoid insecticide widely used to control various sucking insect pests, including aphids. In this study, the effects of sub-lethal concentrations of imidacloprid on the life table traits of the rose-grain aphid Metopolophium dirhodum (Walker) were evaluated on parental and first filial generations. The results showed that sub-lethal concentrations of imidacloprid significantly reduced the fecundity, adult longevity, and reproductive period of M. dirhodum in parental generation (F0). However, the imidacloprid-induced hormetic effects on development and reproduction were detected in the F1 generation. These hormetic effects were indicated by significantly higher adult longevity, fecundity, survival rate, intrinsic and finite rates of increase, and net reproductive rate of first filial generation (F1) of M. dirhodum. Our finding indicated that the application of sub-lethal concentrations of imidacloprid inhibited parental generation (F0), but it significantly stimulated the population growth of filial generation (F1) in the M. dirhodum. The results support the inclusion of insecticides in integrated pest management programs for managing wheat aphids.
Introduction
The insecticide concentration initially applied for managing insect pests degrades with the passage of time (Desneux and Fauvergue, 2005). The insect pests are eventually exposed to these time driven sub-lethal insecticide concentrations (Desneux et al., 2007). This exposure may affect their current and future generations. These sub-lethal concentrations induce physiological and behavioral changes in individuals that survive the initial pesticide exposure (Desneux et al., 2007). In some insects, sub-lethal pesticide exposure adversely affect survival, longevity, fecundity, developmental time, neurophysiological processes, biochemistry, immune capacity (Guo et al., 2013; Ceuppens et al., 2015; Ma et al., 2022), and even induce multiple behaviours (Samuelson et al., 2016). Sub-lethal insecticidal doses may also increase pest infestation by stimulating the growth and reproduction of arthropods (Cordeiro et al., 2013), including green peach aphid Myzus persicae (Tang et al., 2015; Tang et al., 2019), English grain aphid Sitobion avenae, bird cherry-oat aphid Rhopalosiphum padi (Xin et al., 2019), and cotton aphid Aphis gossypii (Ullah et al., 2020).
The biphasic dose-responsive adaptive response (i.e., hormesis) is characterized by low-dose stimulations and high-dose inhibitions (Guedes and Cutler, 2014). Hormetic responses have been detected in various organisms, including insects exposed to insecticides (Calabrese and Baldwin, 2001). The general overcompensation for a disruption in homeostasis (e.g., toxicity) is one of the mechanisms underlying hormesis (Guedes and Cutler, 2014). Additionally, hormesis is an essential consideration when evaluating the impact of pesticides in insect pest management strategies as it may also lead to pest resurgence (Shi et al., 2011; Chen et al., 2015).
Wheat aphids are one of the most harmful insect pests, which adversely affects grain crop production, and are responsible for considerable economic losses (Chopa and Descamps, 2012). The rose-grain aphid, Metopolophium dirhodum Walker (Hemiptera: Aphididae) is a major wheat aphid species that substantially lowers the productivity of cultivated winter cereals (Cannon, 1986; Ma et al., 2003). It was first detected in the 1970s in crops cultivated in South America. Since then, it has spread to new areas worldwide as an important pest of wheat (Chopa and Descamps, 2012; Abdelaziz et al., 2018; Honek et al., 2018). The nymphs and adults of this aphid, feed on phloem fluids from wheat plants during the wheat seedling and jointing stages. Aphid feeding exacerbates nutrient deficiency in wheat plant, resulting in the low grain production. Additionally, the aphids secrete honeydew, which can cover the leaf surface, and then hinders plant respiration and photosynthesis, ultimately leading to low-quality wheat and yield losses up to 27%–30% (Cannon, 1986). Also, the rose-grain aphid may function as a vector for several plant viruses that can damage cereal crops, especially the barley yellow dwarf virus (Luteovirus) (Chopa and Descamps, 2012).
Synthetic insecticides have a key role in modern pest management. Imidacloprid is the first commercially available systemic neonicotinoid insecticide. Its belongs to IRAC class 4A (nicotinic acetylcholine receptor (nAChR) competitive modulators) and blocks the nicotinic acetylcholine receptors in the central and peripheral nervous systems of insects (Palumbo et al., 2001; Byrne et al., 2003; Fernandez et al., 2009). Because of its long-lasting efficacy against diverse homopterous insect pests and relatively low toxicity to non-target organisms, imidacloprid has been commonly used to control insect pests such as M. persicae, Bemisia tabaci and Nilaparvata lugens (Palumbo et al., 2001; Byrne et al., 2003; Liu and Han, 2006). It has also been used in seed treatments for the long-term control of residual wheat aphids (Ahmed et al., 2001). Imidacloprid-induced pest resurgence, including those due to hormesis, have been reported for many insect pests such as the M. persicae (Yu et al., 2010) and A. gossypii (Ullah et al., 2019). However, the possible effects of sub-lethal doses of imidacloprid on M. dirhodum remain relatively unknown. In the present study, the sub-lethal effects of imidacloprid on M. dirhodum were investigated based on two-sex life tables, with a focus on transgenerational effects.
Materials and methods
Aphid rearing
The M. dirhodum population used in this research was sampled in 2016 from wheat fields located at the Pest Sciences Observation and Testing station in Haidian district, Beijing, China. After field collection, the aphids were maintained on young winter wheat (Triticum aestivum Linnaeus) plants in a growth chamber set at 18°C ± 1°C and 60% ± 10% relative humidity, with a 16-h light/8-h dark photoperiod. The aphids were not exposed to any insecticides prior to this study.
Imidacloprid application
Imidacloprid (Gaucho® 600FS) (97.4%, technical grade) was obtained from Bayer Crop Science (Beijing, China). A stock solution was prepared in acetone (Beijing Chemical Works, China) and diluted with water containing 0.05% (w/v) Triton X-100 (Beijing Solarbio Science and Technology Co., Ltd., China) to concentrations appropriate for generating dose-response curves between 0% and 100% M. dirhodum mortality.
Bioassay
A wheat seedling dipping method was used in this study (Gong et al., 2020). Briefly, the imidacloprid stock solution was diluted with distilled water containing 0.05% (w/v) Triton-X to generate nine treatment solutions (i.e., 12.5, 25, 50, 200, 600, 1,200, 1,500, 1,800, and 2,000 mg/L). Wheat seedlings were dipped in the prepared imidacloprid solutions or distilled water containing 0.05% (w/v) Triton-X (control) for 10 s. The seedling roots were wrapped with moistened cotton. The seedlings were then air-dried at room temperature and then placed in 500-mL plastic plates, each containing approximately 10 M. dirhodum adults. The treated and control insects were incubated in a plant growth chamber maintained at 18°C ± 1 °C and 60% ± 10% relative humidity, with a 16-h light/8-h dark photoperiod. Insect mortality was recorded after 24 h. If no more than two legs moved in response to a slight touch with a soft brush, the individual was considered dead. The imidacloprid treatments along with the control treatment were replicated ten times.
Life table analysis
A life table study was completed with 50, 100, and 200 mg/L imidacloprid solutions (prepared in distilled water) and water containing 0.05% (w/v) Triton X-100 as the control. Wheat seedlings were dipped in the imidacloprid or control solutions for 10 s. A cohort of 90 third instar nymphs was treated with imidacloprid as described in bioassay section. After 24 h, the surviving aphids were individually transferred to pots (10 × 10 × 10 cm) containing young winter wheat plants. The short-term (i.e., in the parental generation) sub-lethal effects of imidacloprid on the treated M. dirhodum (F0) fecundity and survival were determined. The number of new born nymphs was recorded and the offspring were removed daily until death. The long-term (i.e., in the filial generation) sub-lethal effects of imidacloprid on M. dirhodum (F1) life table parameters were also examined, including the developmental period, life span, survival rate and fecundity. Accordingly, the life table data of the filial generation were recorded. For each treated female, one or two new born nymphs produced overnight were transferred to a new wheat plant, i.e., 100–120 newborn nymphs (≤24-h old) were observed individually in each group. The number of new-born nymphs was recorded and the offspring were removed daily until death. In each experiment, the aphids were transferred to new plants every 3–4 days.
Data analyses
Corrected aphid mortality was calculated using Abbott’s formula. The LC15, LC25, LC35, were calculated with 95% confidence interval (95% CI), and slope were calculated by a probit analysis using the DPS software (version 7.05). The raw life history data for the M. dirhodum F0 and F1 were evaluated with the TWOSEX-MSChart program (Chi, 2022b), which is based on an age-stage, two-sex life table (Chi, 1988). A bootstrap test with a sample size of 100,000 was completed to detect differences among the means and standard errors of the populations and minimize the variation in the results (Efron and Tibshirani, 1993). To estimate the total population growth, the analysis of the initial M. dirhodum population (50 newborn nymphs) was projected to 60 days based on the above data with the TIMING-MSChart program (Chi, 2022a).
Results
The sub-lethal concentrations of imidacloprid against M. dirhodum
The toxicity of imidacloprid to the M. dirhodum adults was determined, the results show that the estimated LC15, LC25, LC35, and LC50 values were 53.936 mg/L (95% CI: 22.867–94.934 mg/L), 114.734 mg/L (95% CI: 59.716–182.705 mg/L), 209.699 mg/L (95% CI: 124.736–317.737 mg/L), and 380 mg/L (95% CI: 274–852 mg/L), respectively. Finally, 50, 100, and 200 mg/L imidacloprid were used as the sub-lethal concentrations (i.e., LC15, LC25, and LC35, respectively) in subsequent experiments. The effects of these concentrations on third instar nymphs were evaluated. At 24 h after the 50, 100, and 200 mg/L imidacloprid treatments, the aphid mortality rates were 15% ± 0.925%, 24% ± 0.845%, and 35% ± 1.352%, respectively.
The sub-lethal effects of imidacloprid on M. dirhodum parental generation (F0)
The M. dirhodum third instar nymphs were treated with three sub-lethal concentrations of imidacloprid (50, 100, and 200 mg/L). The effects of different sub-lethal doses of imidacloprid on adult longevity, reproductive period and fecundity of M. dirhodum F0 are shown in Figure 1. The results show that the sub-lethal dose of imidacloprid had a significant negative effect on the adult longevity, reproductive period and fecundity of M. dirhodum F0, which showed a trend of significant decrease with the increase of the concentrations of imidacloprid.
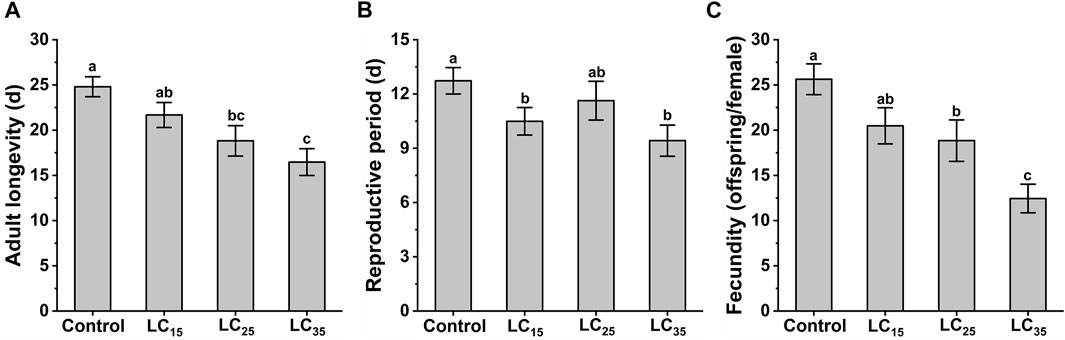
FIGURE 1. The adult longevity (A), reproductive period (B) and fecundity (C) of Metopolophium dirhodum F0 generation under control conditions, treated with 50 mg/L of imidacloprid (LC15), 100 mg/L of imidacloprid (LC25) and 200 mg/L of imidacloprid (LC35).
Transgenerational sub-lethal effects of imidacloprid on the developmental duration, longevity and fecundity of the first filial generation (F1)
The developmental duration, longevity and fecundity for the M. dirhodum F1 whose parents were treated with three sub-lethal concentrations of imidacloprid are presented in Table 1. The filial M. dirhodum aphids (first to fourth instars) developed rapidly following all treatments. Moreover, except for the third instar nymphs, the development was significantly negatively (first instars nymphs) and positively (second and fourth instar nymphs) affected by the three sub-lethal concentrations of imidacloprid. The pre-adult stage duration of F1 individuals tended to decrease, which was in contrast to the increase in the average adult stage duration following the imidacloprid treatments (relative to the effects of the control solution). The adult and total longevity of the F1 individuals was highest in response to the 50 mg/L imidacloprid treatment (LC15). The adult pre-reproductive period (APRP) and total pre-reproductive period (TPRP) was significantly reduced by the imidacloprid treatments, but the reproductive period was not significantly different between imidacloprid and the control treatments. Also, the fecundity of the F1 individuals was highest in response to the 100 mg/L imidacloprid treatment (LC25).
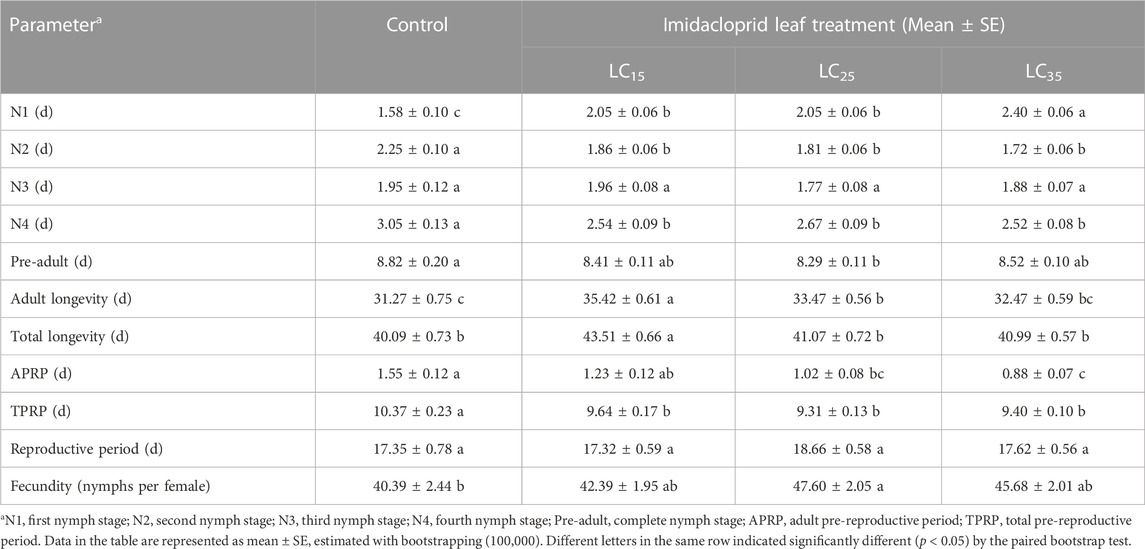
TABLE 1. The sub-lethal effects of imidacloprid on developmental duration and fecundity of the F1 generation of Metopolophium dirhodum.
Transgenerational sub-lethal effects of the imidacloprid on survival rates, life expectancy and reproductive value of the M. dirhodum F1 generation
The curves of age-stage specific survival rates (sxj) reflect the high age-stage-specific survival rates for the females following all treatments, however, the imidacloprid-treated M. dirhodum F1 generation had a higher survival rate than that of the control in later stages (Figure 2). The age-specific survival rate (lx), the age-specific fecundity (mx), and the age-specific maternity (lxmx) reflected the effects of the increasing imidacloprid concentrations on the M. dirhodum population over time, which indicate that these parameters were higher in different doses of imidacloprid treated groups of aphids middle and later stage (Figure 3).
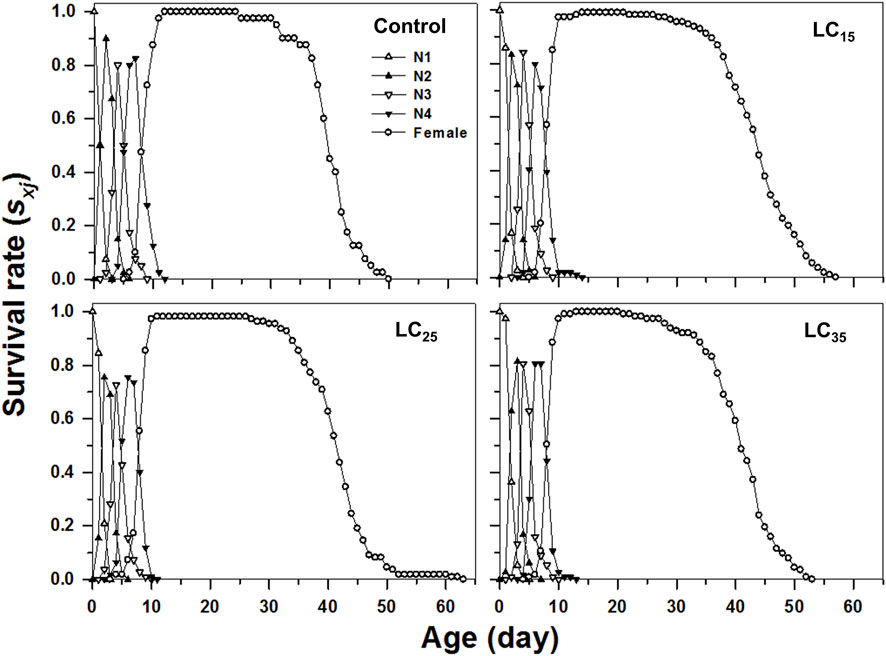
FIGURE 2. Age-stage-specifc survival rates (sxj) of Metopolophium dirhodum individuals of the F1 generation under control conditions, treated with 50 mg/L of imidacloprid (LC15), 100 mg/L of imidacloprid (LC25) and 200 mg/L of imidacloprid (LC35).
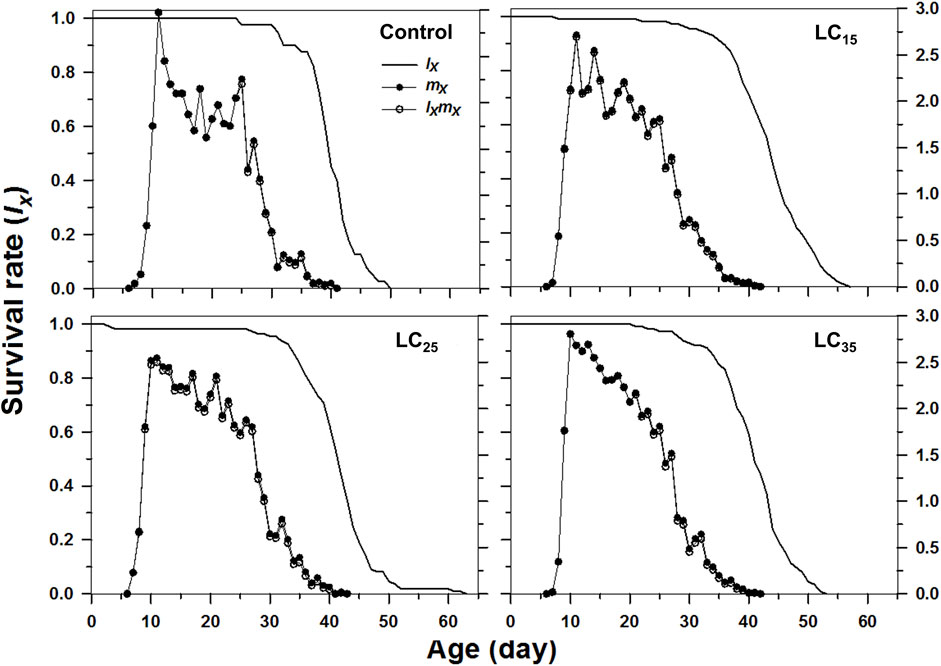
FIGURE 3. Age-specifc survival rates (lx), age-specifc fecundity (mx), and net maternity (lxmx) of Metopolophium dirhodum individuals of the F1 generation under control conditions, treated with 50 mg/L of imidacloprid (LC15), 100 mg/L of imidacloprid (LC25) and 200 mg/L of imidacloprid (LC35).
The age-stage specific life expectancy (exj) refers to the predicted survival time of an individual at age x and stage j. Compared with control, the F1 individuals produced by F0 imidacloprid-treated had a higher life expectancy (Figure 4). Moreover, newborn M. dirhodum nymphs were expected to live for 57, 63, and 53 days following the 50, 100, and 200 mg/L imidacloprid treatments, respectively, for only 50 days in response to the control treatment (Figure 4). An analysis of the M. dirhodum age-stage-specific reproductive rate (vxj) following each imidacloprid treatment revealed that it was highest for LC35 (day 10) than for the control (day 12), as well as for LC15 and LC25 (day 11) (Figure 5).
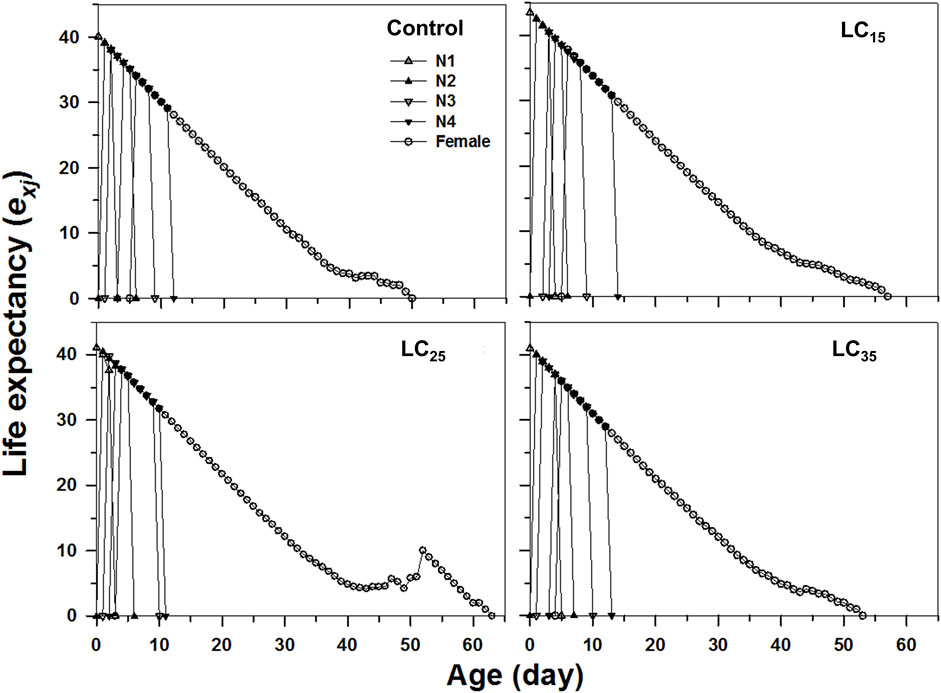
FIGURE 4. Age-stage-specifc life expectancy (exj) of Metopolophium dirhodum individuals of the F1 generation under control conditions, treated with 50 mg/L of imidacloprid (LC15), 100 mg/L of imidacloprid (LC25) and 200 mg/L of imidacloprid (LC35).
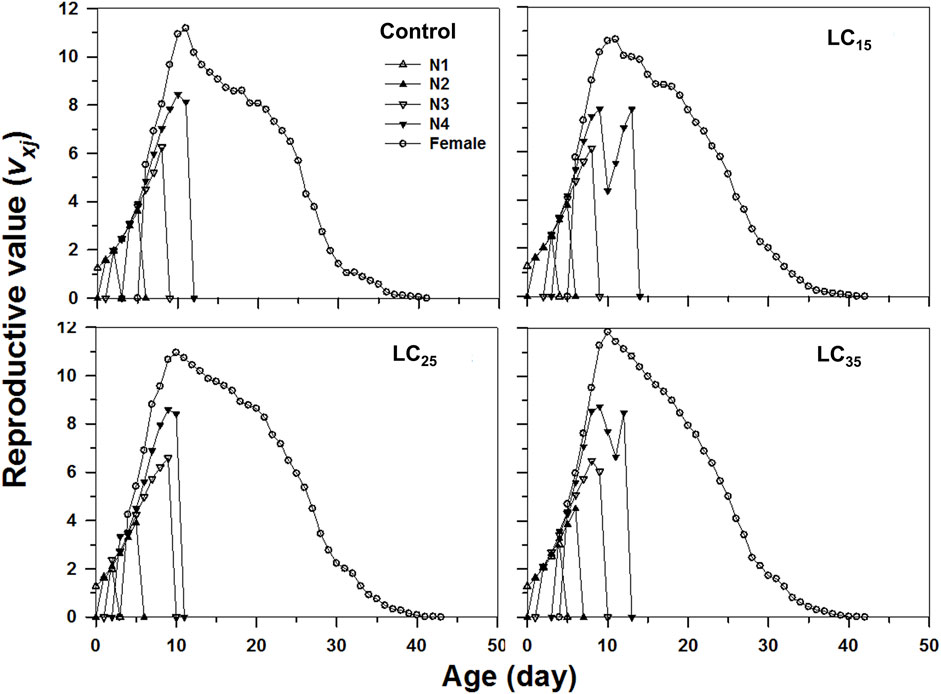
FIGURE 5. Age-stage-specifc reproductive value (vxj) of Metopolophium dirhodum individuals of the F1 generation under control conditions, treated with 50 mg/L of imidacloprid (LC15), 100 mg/L of imidacloprid (LC25) and 200 mg/L of imidacloprid (LC35).
The effects of the imidacloprid on population parameters and total population size of the M. dirhodum F1 generation
Population life-table parameters of the control and imidacloprid-treated M. dirhodum F1 generation are listed in Table 2. The data presented in this table shows that the imidacloprid treatments decreased the mean generation time (T) of M. dirhodum F1 generation. However, increases in imidacloprid concentrations significantly increased the net reproductive rate (R0), intrinsic rate of increase (r) and finite rate of increase (λ), indicated that all imidacloprid treatments induced a rapid M. dirhodum population growth.
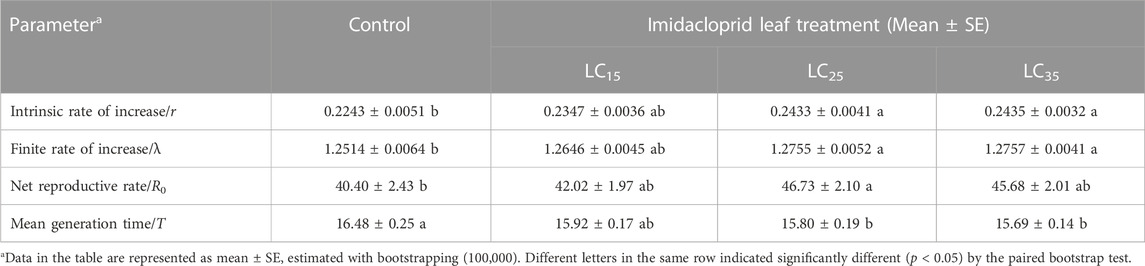
TABLE 2. Sub-lethal effects of imidacloprid on population parameters of the F1 generation of Metopolophium dirhodum.
The projected population was analysed based on the age-stage, two-sex life table and the data for F1 (Figure 6). The control population on day 60 was expected to reach approximately 2.47 million aphids. In contrast, the 50 (LC15), 100 (LC25), and 200 (LC35) mg/L imidacloprid treatments on day 60 were predicted to result in 4.72, 7.81, and 7.80 million aphids, respectively. Forty days later, the M. dirhodum population growth curves calculated on a logarithmic scale were nearly linear, implying that M. dirhodum populations were approaching the stable age-stage distribution. Such linear population increases are indicated by the slopes of the regression lines, which are equal to log(λ) for each cohort. These results suggest that an exposure to sub-lethal concentrations of imidacloprid induced M. dirhodum population growth.
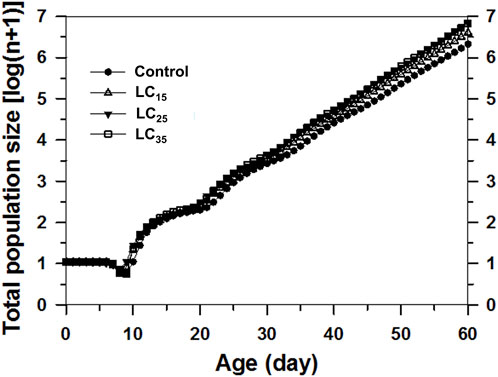
FIGURE 6. Comparison of population projections for Metopolophium dirhodum whose parental females treated with three concentrations of imidacloprid or as the control, based on the age-stage, two-sex life table. The regression formulas describe the linear population growth of each cohort from day 40 onwards as the population approached the stable age-stage distribution.
Discussion
Imidacloprid is a typical neonicotinoid insecticide widely used to control various sucking pests, including aphids (Palumbo et al., 2001; Byrne et al., 2003; Fernandez et al., 2009) Target and non-target arthropods are often exposed to low insecticide concentrations in treated fields. The potential sub-lethal effects of insecticides should be evaluated while developing effective integrated pest management programs (Desneux et al., 2007; Guedes et al., 2016). Assessment of sub-lethal insecticidal effects on target insects is critical for enhancing the pesticide efficiency (Liang et al., 2012; Xiao et al., 2015). The primary objective of the current study was to clarify the sub-lethal effects of imidacloprid on the development of the M. dirhodum population.
Insecticide-induced hormesis may result in pest resurgence and/or secondary pest outbreaks, which may necessitate additional pesticide applications and a steady accumulation of potentially harmful chemicals in the field (Cordeiro et al., 2013; Guedes et al., 2016). Hormesis due to insecticide applications has been observed in other aphid species, including M. persicae treated with low imidacloprid concentrations (Christopher Cutler et al., 2009; Murali-Mohan et al., 2013) and A. gossypii exposed to sulfoxaflor, pirimicarb, and flonicamid (Koo et al., 2015; Xiao et al., 2015; Chen et al., 2016).
Earlier investigations indicated that treatments with sub-lethal doses of thiamethoxam adversely affect Hippodamia variegate and Bradysia odoriphaga population growth (Rahmani and Bandani, 2013; Zhang et al., 2014). In the current study, the sub-lethal dose of imidacloprid had also a significant adverse effect on M. dirhodum F0 generation, indicating that the stimulatory (hormetic) effects were not observed in F0 individuals treated with low concentrations of imidacloprid. This is consistent with the reported lack of hormetic effects in the F0 generations of A. gossypii exposed to a sub-lethal concentration of afidopyropen and sulfoxaflor (Chen et al., 2016; Ma et al., 2022) and an imidacloprid-resistant A. gossypii strain treated with a low lethal concentration of imidacloprid (Shi et al., 2011). Similar effects were also observed in M. persicae, B. brassicae, B. tabaci, and Apolygus lucorum (Meyer-Dür) treated with sub-lethal doses of imidacloprid (Lashkari et al., 2007; Wang et al., 2008; Tan et al., 2012; He et al., 2013).
In this study, assessing the transgenerational effects of imidacloprid treatments on M. dirhodum, we observed that an exposure to sub-lethal concentrations of imidacloprid in the F0 generation (i.e., parent generation) significantly stimulated the population growth of the F1 generation, the analysed life table parameters (r, λ, and R0), fecundity, longevity, and survival of the F1 generation were positively affected by the sub-lethal concentrations of imidacloprid treatments. Additionally, the population prediction results show that an exposure to sub-lethal concentrations of imidacloprid induced M. dirhodum population growth. Similar effects on population growth were observed in an earlier study on sweetpotato whitefly B. tabaci, which proved that imidacloprid increases the gross reproduction rate, but does not significantly affect the mean generation time (Esmaeily et al., 2014). Another study involving field experiments in Australian revealed that the egg production and population development of the Australian predatory mite Amblyseius victoriensis (Womersley) significantly increase in response to systemic spray treatments of imidacloprid (James, 1997). Other investigations confirmed that a low dose of imidacloprid shortens the mean generation time of cabbage aphid Brevicoryne brassicae (Lashkari et al., 2007), whereas a sub-lethal imidacloprid concentration significantly extends the mean generation time of B. tabaci, while also increasing fecundity and egg production (Sohrabi et al., 2011). Furthermore, the systemic application of imidacloprid to control psyllids on pear trees reportedly increase the fecundity of mite populations (James and Price, 2002).
The stimulated reproduction of insects exposed to low lethal concentration of insecticides is due to hormesis (Guedes and Cutler, 2014). The imidacloprid or one of its metabolites might have altered aphid physiology, with potential consequences for reproduction and population growth. There are reports describing the increased fecundity in Tetranychus urticae, A. victoriensis, and Tryporyza incertulas due to low imidacloprid concentrations (James and Price, 2002; Wang et al., 2005). Additionally, the exposure of M. persicae to sub-lethal doses of imidacloprid was observed to result in a hormetic effect on fecundity in the F2 generation (Christopher Cutler et al., 2009). In this study, the hormetic effects of imidacloprid on the life table parameters of M. dirhodum were detected in F1 generation, and fecundity was highest for LC25 and the adult longevity was longest for LC15. When the insecticide concentration is too low, the overcorrection is not triggered or it is not discernible (Stebbing, 2003). These results imply that the development of hormetic effects may be influenced by time and the imidacloprid concentration, and the complex mechanism underlying the imidacloprid dose effect on the occurrence of hormesis.
In conclusion, our findings indicated that the application of sub-lethal concentrations of imidacloprid has inhibitory effects on the parental generation (F0), but has stimulatory effects on the first filial generation (F1). Considering all of the arthropod biological processes affected by pesticides, it is possible that hormetic responses are induced in arthropods exposed to these chemicals (Kendig et al., 2010; Liang et al., 2012). Future research should examine the effects of various low lethal and sub-lethal insecticide concentrations to comprehensively characterize the putative hormetic responses of wheat aphid pests to neonicotinoid insecticides. The results of this research may be relevant for optimizing integrated pest management strategies involving neonicotinoid insecticides.
Data availability statement
The original contributions presented in the study are included in the article/supplementary material, further inquiries can be directed to the corresponding author.
Author contributions
YZ and DC conceived and designed the research. XnL and YL conducted the experiments. XZ analysed the data. XnL and XZ wrote the manuscript. XiL and DC revised the manuscript. All authors have read and approved the manuscript.
Funding
This study was supported by the China Agriculture Research System of MOF and MARA (CARS-03).
Acknowledgments
We thank Liwen Bianji, Edanz Editing China (www.liwenbianji.cn/ac) for editing the English text of a draft of this manuscript.
Conflict of interest
The authors declare that the research was conducted in the absence of any commercial or financial relationships that could be construed as a potential conflict of interest.
Publisher’s note
All claims expressed in this article are solely those of the authors and do not necessarily represent those of their affiliated organizations, or those of the publisher, the editors and the reviewers. Any product that may be evaluated in this article, or claim that may be made by its manufacturer, is not guaranteed or endorsed by the publisher.
References
Abdelaziz, O., Senoussi, M. M., Oufroukh, A., Birgücü, A. K., Karaca, İ., Kouadri, F., et al. (2018). Pathogenicity of three entomopathogenic fungi, to the aphid species, Metopolophium dirhodum (Walker) (Hemiptera: Aphididae), and their Alkaline protease activities. Egypt. J. Biol. Pest Co. 28, 24. doi:10.1186/s41938-018-0030-7
Ahmed, N. E., Kanan, H. O., Inanaga, S., Ma, Y. Q., and Sugimoto, Y. (2001). Impact of pesticide seed treatments on aphid control and yield of wheat in the Sudan. Crop Prot. 20, 929–934. doi:10.1016/S0261-2194(01)00047-3
Byrne, F. J., Castle, S., Prabhaker, N., and Toscano, N. C. (2003). Biochemical study of resistance to imidacloprid in B biotype Bemisia tabaci from Guatemala. Pest Manag. Sci. 59, 347–352. doi:10.1002/ps.649
Calabrese, E. J., and Baldwin, L. A. (2001). Hormesis: A generalizable and unifying hypothesis. Crit. Rev. Toxicol. 31, 353–424. doi:10.1080/20014091111730
Cannon, R. J. C. (1986). Summer populations of the cereal aphid Metopolophium dirhodum (walker) on winter wheat: Three contrasting years. J. Appl. Ecol. 23, 101–114. doi:10.2307/2403084
Ceuppens, B., Eeraerts, M., Vleugels, T., Cnops, G., Roldan-Ruiz, I., and Smagghe, G. (2015). Effects of dietary lambda-cyhalothrin exposure on bumblebee survival, reproduction, and foraging behavior in laboratory and greenhouse. J. Pest Sci. 88, 777–783. doi:10.1007/s10340-015-0676-9
Chen, X., Ma, K., Li, F., Liang, P., Liu, Y., Guo, T., et al. (2016). Sublethal and transgenerational effects of sulfoxaflor on the biological traits of the cotton aphid, Aphis gossypii Glover (Hemiptera: Aphididae). Ecotoxicology 25, 1841–1848. doi:10.1007/s10646-016-1732-9
Chen, Z., Qu, Y., Xiao, D., Song, L., Zhang, S., Gao, X., et al. (2015). Lethal and social-mediated effects of ten insecticides on the subterranean termite Reticulitermes speratus. J. Pest Sci. 88, 741–751. doi:10.1007/s10340-015-0656-0
Chi, H. (1988). Life-table analysis incorporating both sexes and variable development rates among individuals. Environ. Entomol. 17, 26–34. doi:10.1093/ee/17.1.26
Chi, H. (2022a). TIMING-MSChart-exe.rar. Available at: http://140.120.197.173/Ecology/prod102.htm.
Chi, H. (2022b). TWOSEX-MSChart-exe-B100000. Available at: http://140.120.197.173/Ecology/prod102. htm.
Chopa, C. S., and Descamps, L. R. (2012). Composition and biological activity of essential oils against Metopolophium dirhodum (Hemiptera: Aphididae) cereal crop pest. Pest Manag. Sci. 68, 1492–1500. doi:10.1002/ps.3334
Christopher Cutler, G., Ramanaidu, K., Astatkie, T., and Isman, M. B. (2009). Green peach aphid, Myzus persicae (Hemiptera: Aphididae), reproduction during exposure to sublethal concentrations of imidacloprid and azadirachtin. Pest Manag. Sci. 65, 205–209. doi:10.1002/ps.1669
Cordeiro, E. M., de Moura, I. L., Fadini, M. A., and Guedes, R. N. (2013). Beyond selectivity: Are behavioral avoidance and hormesis likely causes of pyrethroid-induced outbreaks of the southern red mite Oligonychus ilicis? Chemosphere 93, 1111–1116. doi:10.1016/j.chemosphere.2013.06.030
Desneux, N., Decourtye, A., and Delpuech, J. M. (2007). The sublethal effects of pesticides on beneficial arthropods. Annu. Rev. Entomol. 52, 81–106. doi:10.1146/annurev.ento.52.110405.091440
Desneux, N., Fauvergue, X., Dechaume-Moncharmont, F. X., Kerhoas, L., Ballanger, Y., and Kaiser, L. (2005). Diaeretiella rapae limits Myzus persicae populations after applications of deltamethrin in oilseed rape. J. Econ. Entomol. 98, 9–17. doi:10.1093/jee/98.1.9
Efron, B., and Tibshirani, R. J. (1993). Monographs on Statistics and Applied Probability. London: Chapman & Hall, 436. No. 57.An introduction to the bootstrap
Esmaeily, S., Samih, M. A., Zarabi, M., and Jafarbeigi, F. (2014). Sublethal effects of some synthetic and botanical insecticides on Bemisia tabaci (Hemiptera: Aleyrodidae). J. Plant Prot. Res. 54, 171–178. doi:10.2478/jppr-2014-0027
Fernandez, E., Gravalos, C., Haro, P. J., Cifuentes, D., and Bielza, P. (2009). Insecticide resistance status of Bemisia tabaci Q-biotype in south-eastern Spain. Pest Manag. Sci. 65, 885–891. doi:10.1002/ps.1769
Gong, P., Chen, D., Wang, C., Li, M., Li, X., Zhang, Y., et al. (2020). Susceptibility of four species of aphids in wheat to seven insecticides and its relationship to detoxifying enzymes. Front. Physiol. 11, 623612. doi:10.3389/fphys.2020.623612
Guedes, R. N., and Cutler, G. C. (2014). Insecticide-induced hormesis and arthropod pest management. Pest Manag. Sci. 70, 690–697. doi:10.1002/ps.3669
Guedes, R. N., Smagghe, G., Stark, J. D., and Desneux, N. (2016). Pesticide-induced stress in arthropod pests for optimized integrated pest management programs. Annu. Rev. Entomol. 61, 43–62. doi:10.1146/annurev-ento-010715-023646
Guo, L., Desneux, N., Sonoda, S., Liang, P., Han, P., and Gao, X.-W. (2013). Sublethal and transgenerational effects of chlorantraniliprole on biological traits of the diamondback moth, Plutella xylostella L. Plutella xylostella L. Crop Prot. 48, 29–34. doi:10.1016/j.cropro.2013.02.009
He, Y., Zhao, J., Zheng, Y., Weng, Q., Biondi, A., Desneux, N., et al. (2013). Assessment of potential sublethal effects of various insecticides on key biological traits of the tobacco whitefly, Bemisia tabaci. Int. J. Biol. Sci. 9, 246–255. doi:10.7150/ijbs.5762
Honek, A., Martinkova, Z., Saska, P., and Dixon, A. F. G. (2018). Aphids (Homoptera: Aphididae) on winter wheat: Predicting maximum abundance of Metopolophium dirhodum. J. Econ. Entomol. 111, 1751–1759. doi:10.1093/jee/toy157
James, D. G. (1997). Imidacloprid increases egg production in Amblyseius victoriensis (Acari: Phytoseiidae). Exp. Appl. Acarol. 21, 75–82. doi:10.1023/A:1018493409832
James, D. G., and Price, T. S. (2002). Fecundity in two spotted spider mite (Acari: Tetranychidae) is increased by direct and systemic exposure to imidacloprid. J. Econ. Entomol. 95, 729–732. doi:10.1603/0022-0493-95.4.729
Kendig, E. L., Le, H. H., and Belcher, S. M. (2010). Defining hormesis: Evaluation of a complex concentration response phenomenon. Int. J. Toxicol. 29, 235–246. doi:10.1177/1091581810363012
Koo, H. N., Lee, S. W., Yun, S. w., Kim, H. K., and Kim, G. H. (2015). Feeding response of the cotton aphid, Aphis gossypii, to sublethal rates of flonicamid and imidacloprid. Entomol. Exp. Appl. 154, 110–119. doi:10.1111/eea.12260
Lashkari, M. R., Sahragard, A., and Ghadamyari, M. (2007). Sublethal effects of imidacloprid and pymetrozine on population growth parameters of cabbage aphid, Brevicoryne brassicae on rapeseed, Brassica napus L. Insect Sci. 14, 207–212. doi:10.1111/j.1744-7917.2007.00145.x
Liang, P., Tian, Y.-A., Biondi, A., Desneux, N., and Gao, X.-W. (2012). Short-term and transgenerational effects of the neonicotinoid nitenpyram on susceptibility to insecticides in two whitefly species. Ecotoxicology 21, 1889–1898. doi:10.1007/s10646-012-0922-3
Liu, Z., and Han, Z. (2006). Fitness costs of laboratory-selected imidacloprid resistance in the Brown planthopper, Nilaparvata lugens Stål. Nil. lugens Stål. Pest Manag. Sci. 62, 279–282. doi:10.1002/ps.1169
Ma, C., Hau, B., and Poehling, H. (2003). Effects of pattern and timing of high temperature exposure on reproduction of the rose grain aphid, Metopolophium dirhodum. Metopolophium Dirhodum. Entomol. Exp. Appl. 110, 65–71. doi:10.1111/j.0013-8703.2004.00123.x
Ma, K., Tang, Q., Liang, P., Li, J., and Gao, X. (2022). A sublethal concentration of afidopyropen suppresses the population growth of the cotton aphid, Aphis gossypii Glover (Hemiptera: Aphididae). J. Integr. Agr. 21, 2055–2064. doi:10.1016/s2095-3119(21)63714-0
Murali-Mohan, A., Christopher, C. G., Scott-Dupree, C. D., Sibley, P. K., and Guy, S. (2013). Transgenerational shifts in reproduction hormesis in green peach aphid exposed to low concentrations of imidacloprid. PLoS ONE 8, e74532. doi:10.1371/journal.pone.0074532
Palumbo, J. C., Horowitz, A. R., and Prabhaker, N. (2001). Insecticidal control and resistance management for Bemisia tabaci. Crop Prot. 20, 739–765. doi:10.1016/S0261-2194(01)00117-X
Rahmani, S., and Bandani, A. R. (2013). Sublethal concentrations of thiamethoxam adversely affect life table parameters of the aphid predator, Hippodamia variegata (Goeze) (Coleoptera: Coccinellidae). Crop Prot. 54, 168–175. doi:10.1016/j.cropro.2013.08.002
Samuelson, E. E., Chen-Wishart, Z. P., Gill, R. J., and Leadbeater, E. (2016). Effect of acute pesticide exposure on bee spatial working memory using an analogue of the radial-arm maze. Sci. Rep. 6, 38957. doi:10.1038/srep38957
Shi, X., Jiang, L., Wang, H., Qiao, K., Wang, D., and Wang, K. (2011). Toxicities and sublethal effects of seven neonicotinoid insecticides on survival, growth and reproduction of imidacloprid-resistant cotton aphid, Aphis gossypii. Pest Manag. Sci. 67, 1528–1533. doi:10.1002/ps.2207
Sohrabi, F., Shishehbor, P., Saber, M., and Mosaddegh, M. S. (2011). Lethal and sublethal effects of buprofezin and imidacloprid on Bemisia tabaci (Hemiptera: Aleyrodidae). Crop Prot. 30, 1190–1195. doi:10.1016/j.cropro.2011.05.004
Stebbing, A. R. D. (2003). A mechanism for hormesis-a problem in the wrong discipline. Crit. Rev. Toxicol. 33, 463–467. doi:10.1080/713611038
Tan, Y., Biondi, A., Desneux, N., and Gao, X.-W. (2012). Assessment of physiological sublethal effects of imidacloprid on the mirid bug Apolygus lucorum (Meyer-Dür). Ecotoxicology 21, 1989–1997. doi:10.1007/s10646-012-0933-0
Tang, Q., Ma, K., Chi, H., Hou, Y., and Gao, X. (2019). Transgenerational hormetic effects of sublethal dose of flupyradifurone on the green peach aphid, Myzus persicae (Sulzer) (Hemiptera: Aphididae). PLoS ONE 14, e0208058. doi:10.1371/journal.pone.0208058
Tang, Q., Xiang, M., Hu, H., An, C., and Gao, X. (2015). Evaluation of sublethal effects of sulfoxaflor on the green peach aphid (Hemiptera: Aphididae) using life table parameters. J. Econ. Entomol. 108, 2720–2728. doi:10.1093/jee/tov221
Ullah, F., Gul, H., Desneux, N., Gao, X., and Song, D. (2019). Imidacloprid-induced hormesis effects on demographic traits of the melon aphid, Aphis gossypii. Entomol. Gen. 39, 325–337. doi:10.1127/entomologia/2019/0892
Ullah, F., Gul, H., Tariq, K., Desneux, N., Gao, X., and Song, D. (2020). Thiamethoxam induces transgenerational hormesis effects and alteration of genes expression in Aphis gossypii. Pestic. Biochem. Physiol. 165, 104557. doi:10.1016/j.pestbp.2020.104557
Wang, A., Wu, J., Yu, Y., Liu, J., Yue, J., and Wang, M. (2005). Selective insecticide-induced stimulation on fecundity and biochemical changes in Tryporyza incertulas (Lepidoptera: Pyralidae). J. Econ. Entomol. 98, 1144–1149. doi:10.1603/0022-0493-98.4.1144
Wang, X. Y., Yang, Z. Q., Shen, Z. R., Lu, J., and Xu, W. B. (2008). Sublethal effects of selected insecticides on fecundity and wing dimorphism of green peach aphid (Hom., Aphididae). J. Appl. Entomol. 132, 135–142. doi:10.1111/j.1439-0418.2007.01225.x
Xiao, D., Yang, T., Desneux, N., Han, P., and Gao, X. (2015). Assessment of sublethal and transgenerational effects of pirimicarb on the wheat aphids Rhopalosiphum padi and Sitobion avenae. PLoS ONE 10, e0128936. doi:10.1371/journal.pone.0128936
Xin, J. J., Yu, W. X., Yi, X. Q., Gao, J. P., Gao, X. W., and Zeng, X. P. (2019). Sublethal effects of sulfoxaflor on the fitness of two species of wheat aphids, Sitobion avenae (F.) and Rhopalosiphum padi (L.). J. Integr. Agr. 18, 1613–1623. doi:10.1016/s2095-3119(18)62094-5
Yu, Y., Shen, G., Zhu, H., and Lu, Y. (2010). Imidacloprid-induced hormesis on the fecundity and juvenile hormone levels of the green peach aphid Myzus persicae (Sulzer). Pestic. Biochem. Physiol. 98, 238–242. doi:10.1016/j.pestbp.2010.06.013
Zhang, J., Yuan, F., Liu, J., Chen, H., and Zhang, R. (2010). Sublethal effects of nitenpyram on life-table parameters and wing formation of Nilaparvata lugens (stål) (Homoptera: Delphacidae). Appl. Entomol. Zool. 45, 569–574. doi:10.1303/aez.2010.569
Keywords: hormesis, imidacloprid, Metopolophium dirhodum, longevity, fecundity
Citation: Li X, Li Y, Zhu X, Li X, Cheng D and Zhang Y (2023) Effects of imidacloprid-induced hormesis on the development and reproduction of the rose-grain aphid Metopolophium dirhodum (Hemiptera: Aphididae). Front. Physiol. 14:1113464. doi: 10.3389/fphys.2023.1113464
Received: 01 December 2022; Accepted: 25 January 2023;
Published: 03 February 2023.
Edited by:
Ting Li, Alabama State University, United StatesReviewed by:
Jianhong Li, Huazhong Agricultural University, ChinaXinzheng Huang, China Agricultural University, China
Copyright © 2023 Li, Li, Zhu, Li, Cheng and Zhang. This is an open-access article distributed under the terms of the Creative Commons Attribution License (CC BY). The use, distribution or reproduction in other forums is permitted, provided the original author(s) and the copyright owner(s) are credited and that the original publication in this journal is cited, in accordance with accepted academic practice. No use, distribution or reproduction is permitted which does not comply with these terms.
*Correspondence: Yunhui Zhang, yhzhang@ippcaas.cn
†These authors have contributed equally to this work