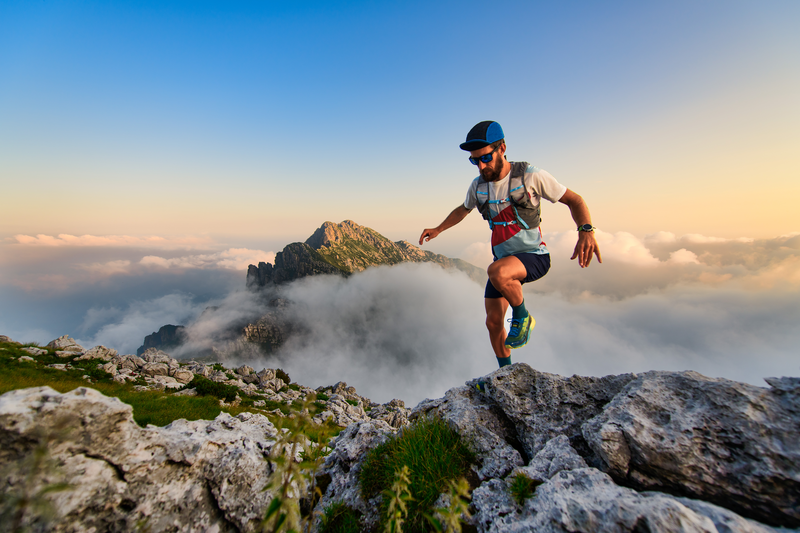
95% of researchers rate our articles as excellent or good
Learn more about the work of our research integrity team to safeguard the quality of each article we publish.
Find out more
ORIGINAL RESEARCH article
Front. Physiol. , 18 April 2023
Sec. Craniofacial Biology and Dental Research
Volume 14 - 2023 | https://doi.org/10.3389/fphys.2023.1111857
Background: This study attempts to detect the potential effects of local bone morphogenetic protein -2 (BMP-2) on orthodontic tooth movement and periodontal tissue remodeling.
Methods: Forty adult SD rats were randomly divided into four groups: blank control group, unilateral injection of BMP-2 on the pressure side or tension side of orthodontic teeth and bilateral injection of BMP-2. Their maxillary first molar was moved by a 30 g constant force closed coil spring. 60 μL of BMP-2 with a concentration of 0.5 μg/mL was injected into each part at a time. In addition, three rats were selected as healthy control rats without any intervention. Fluorescent labeled BMP-2 was used to observe the distribution of exogenous BMP-2 in tissues. Micro-CT was used to measure the microscopic parameters of tooth displacement, trabecular bone and root absorption volume. Three different histological methods were used to observe the changes of tissue remodeling, and then the number of osteoclasts and the content of collagen fibers were calculated.
Results: Compared with the blank control group, BMP-2 injection reduced the movement distance and increased the collagen fiber content and bone mass (p < 0.01). There was no significant difference in tooth movement distance, BV/TV ratio and BMD between injection sites in unilateral injection group (p > 0.05). In the case of bilateral injection of BMP-2, the osteogenesis is enhanced. Unilateral injection of BMP-2 did not promote root resorption, but double injection showed root resorption (p < 0.01).
Conclusion: Our study does show that the osteogenesis of BMP-2 is dose-dependent rather than site-dependent when a certain amount of BMP-2 is applied around orthodontic teeth. Local application of BMP-2 around orthodontic teeth in an appropriate way can enhance bone mass and tooth anchorage without increasing the risk of root absorption volume. However, high levels of BMP-2 may cause aggressive root resorption. These findings are of great significance, that is, BMP-2 is an effective target for regulating orthodontic tooth movement.
By applying spatial analysis and more adjustable movement, orthodontic goals can be achieved more accurately and effectively. Orthodontic tooth movement (OTM) is achieved through the remodeling of periodontal ligament (PDL) and alveolar bone, in response to controlled mechanical load, bone absorption under pressure and bone formation under tension (Melsen, 2001). Bone remodeling is considered to be the decisive factor of orthodontic tooth movement, and osteoblasts and osteoclasts are the main cell effectors (Baloul et al., 2011).
Efficient orthodontic augmentation devices, osteoprotegerin (OPG) and sclerostin as potential targets, physical therapy techniques (such as low laser therapy and vibration), surgical treatment and gene therapy are all effective methods to regulate OTM (Fernández-González et al., 2016; Sugimori et al., 2018; Isola et al., 2019; Lu et al., 2019; Sakamoto et al., 2019; Sivarajan et al., 2019; Yoo-Sung et al., 2022). Bone reconstruction is a key component of most methods. There are several cytokines and signal molecules that affect the biological trend and rate of bone remodeling, which may be related to tooth movement in theory (Lee et al., 2021; Li et al., 2021). Bone morphogenetic protein-2 (BMP-2) is essential for controlling the proliferation, differentiation and secretion of bone matrix of osteoblasts, and regulating osteoclast differentiation and activating bone resorption through RANKL/OPG pathway (Kamiya and Mishina, 2011). The level of BMP-2 is related to the histological changes during OTM, and the expression of BMP-2 can be used as a marker to detect osteogenic activity and evaluate the efficacy of various techniques to regulate bone remodeling (Kamiya and Mishina, 2011). Due to mechanical contraction, BMP-2 protein level and gene expression on the tension side can be significantly enhanced (Wescott et al., 2007).
In the dental application of bone growth, the concentration of BMP-2 ranges from 0.5 μg/mL to 2.5 μg/mL (Stenport et al., 2003; Vercellotti and Podesta, 2007; Razzouk and Sarkis, 2012). It is reported that the combined application of BMP-2 and periodontal accelerated osteogenesis orthodontics (PAOO) can shorten the treatment time and increase bone mineral density compared with traditional cortical resection (Gao et al., 2021). However, this is still an ongoing debate, because some authors have found that the application of BMP-2 on the tension side can not promote OTM acceleration (Iglesias-Linares et al., 2012; Kurohama et al., 2017). The role of exogenous BMP-2 in the regulation of tooth mobility or bone remodeling of orthodontic teeth has not been clarified. There is still uncertainty about whether local application of BMP-2 around orthodontic teeth can enhance the resistance to traction, or on the contrary, whether it can promote the movement rate by accelerating the pace of bone reconstruction. The purpose of this study is to evaluate the overall effect of clinically acceptable dose of BMP-2 on orthodontic tooth mobility and biological tissue remodeling.
The rat OTM model used in previous studies was established (King et al., 1991; Zhou et al., 2019). Rats were anesthetized by intraperitoneal injection of 10% ketamine hydrochloride (0.08 mL per 100 g body weight) and 2% tolthiazine hydrochloride (0.04 mL per 100 g body weight). Using a dental diamond drill, the retention notches were ground on the distal, mesial and labial sides of the left maxillary incisors and the mesial and buccal and lingual sides of the left maxillary first molar (M1). The incisors and the neck of M1 were ligated with 0.2 mm ligature (CrNi, Morelj, Brazil), and the tooth surface was etched with 35% phosphoric acid (Gluma, Etch 35%, Heraeus Kulzer, Germany) for 30 s, washed, dried, insulated, and coated with adhesive (Transbond, 3M Unitek, Monrovia, California). A thin layer of resin (Filtek Flow composite, 3M/ESPE, St. Paul, MN, USA) was covered on the surface of the line and incision to prevent the dislocation of the ligature line. A closed coil NiTi spring (Light, Sentalloy, GAC, Dentsply) was ligated between the left maxillary central incisor and M1 for traction (Figure 1A), and the force was calculated by an dynamometer (YS-31D, YDM). Check this force activation device every day, and if it is damaged or released, immediately reinstall or add rat specimens.
FIGURE 1. Experimental design. Establishment of orthodontic tooth movement (OTM) model (A). A closed coil NiTi spring was ligated between the left maxillary central incisor and the first molar (M1). A constant force of 30 g was ensured. The administration site was defined on the pressure side (P) and tension side (T) side of the orthodontic tooth 0.5 mm close to the periodontal ligament. The whole morphology of the mesial root of M1 was captured in sections taken from the mesial and distal sides (double red bar) along the long axis of M1. Group distribution (B). 40 healthy SD rats were randomly allocated into four groups based on different interventions on the established OTM model: 1-blank control (C) group without intervention on the orthodontic tooth; 2-Pressure BMP-2(PBMP-2) group with administration of BMP-2 on the pressure side of the orthodontic tooth; 3-Tension BMP-2 (TBMP-2) group with administration of BMP-2 on the tension side of the orthodontic tooth; 4-Pressure and Tension BMP-2 (PTBMP-2) group with administration of BMP-2 on both the pressure and tension side of the orthodontic tooth. Three healthy SD rats were selected and housed in the identical condition to the healthy control (H) group without any intervention. The flow of the experiment contains the time point of intervention and intraoral representation of the experimental procedures (C). The first injection was administered immediately after the placement of the orthodontic augmentation devices (Day 0), and then every 3 days (Day 4, Day 8, and Day 12). All samples were sacrificed after 16 days. ◀ Represents the installation of the orthodontic augmentation devices, ● represents the injection of BMP-2, ★ represents the execution of animals for material.
All animal studies were conducted in accordance with the guidelines of the National Institutes of Health (NIH) and approved by the Fourth Medical Center of the General Hospital of the Chinese People’s Liberation Army (approval number ZJU20160455). The animals included in this study adapted to the environment for at least 1 week before the experiment. Forty Sprague-Dawley rats (8 weeks old, 280–300 g) were randomly divided into four groups according to different administration procedures (Figure 1B):1- blank control group (C) without any intervention on orthodontic teeth; In 2- pressure BMP-2 (PBMP-2) group, BMP-2 was applied to the pressure side of orthodontic teeth; In 3- tension BMP-2 (TBMP-2) group, BMP-2 was applied to the tension side of orthodontic teeth; In the 4- pressure and tension BMP-2 (PTBMP-2) group, BMP-2 was applied on the pressure and tension sides of orthodontic teeth. The other three SD rats were placed under the same conditions as the healthy control group (H) without any intervention.
The recombinant protein BMP-2 (molecular weight 13 kDa, R&D Systems, Minneapolis, Minnesota) was recombined according to the manufacturer’s instructions. Freeze-dried from 0.2 micron filtered solution in glycine, sucrose, Tween 80 and glutamic acid, using BSA as carrier protein. * Provide 1 mg package size (01 M) in the form of 0.2 m filtered solution in glycine, sucrose, Tween 80 and glutamic acid, with BSA as carrier protein. Reconstructed in sterile 4 mM HCl containing at least 0.1% human or bovine serum albumin at a concentration of 100–200 μg/mL. And adjust to that concentration of 0.5 μg/mL/60 μL injection to produce BMP-2. BMP-2 was injected with Hamilton micro syringe (microliter syringe) within 0.5 mm near periodontal ligament on the pressure or tension side of orthodontic teeth (Figure 1A). The first injection was made immediately after the orthodontic appliance was installed (day 0), and then the injection was made with ether anesthetic every 3 days (day 4, day 8 and day 12). After 16 days, all the samples were killed due to an overdose of narcotic drugs (Figure 1C). During this period, it has been proved that significant tooth movement has been achieved (Marcantonio et al., 2021; Nogueira et al., 2017). After death, the left maxilla of each sample was taken out, and then reset and randomly assigned for micro-computed tomography (micro-CT) and histological analysis.
Three samples were randomly selected from each experimental BMP-2 injection group and given fluorescently labeled BMP-2 (R&D Systems, Minneapolis, Minnesota). One sample in each group was killed on the first, second, and third day after injection. After decalcification and dehydration, the left maxillary samples were collected and sliced. The distribution of BMP-2 in periodontal tissue was observed by confocal laser scanning microscope (Olympus, Japan). Microscopic CT examination.
After the rats were killed, the left maxilla was taken out, and the repaired bone was immediately placed on the scanning table with a micro-CT machine (Skyscan 1176 Bruker, Kontich, Belgium). The scanning parameters were set to 70 kVp voltage, 114 uA current, 8 W, 38.9 mm viewing angle diameter and 10 μm image resolution. Using nrecon (Bruker version 1.6.1.5), Data Viewer (Bruker version 1.4.3.1) and CTAN (Bruker version 1.10.1.0 by CTan (CT-Analyser software), the 3D image was reconstructed, and the image location and stereological analysis were carried out. The region of interest (ROI) is defined as a cube of 700 μm × 700 μm × 700 μm, which is located in the mesial alveolar bone space between the distal end and the labial root, and is 200 μm away from the root surface (Figure 2). Root and periodontal ligament were excluded from ROI. The microstructure parameters evaluated included bone volume fraction (BV/TV), bone mineral density (BMD) and trabecular number (Tb). N), trabecular separation (Tb. Sp) and trabecular thickness (Tb. Th). The volume of the absorption pit (106 μm3) on the mesial root of M1 cementum surface was measured in Mimics Medical 21.0 software (Materialise, Belgium), and the convex hull algorithm was used for calculation. In order to determine the tooth movement, the horizontal distance between the distal surface of M1 and the highest convex surface of the proximal surface of M2 was measured (Nogueira et al., 2021).
FIGURE 2. Definition of the region of interest (ROI) on the coronal view (A), axial view (B), sagittal view (C) of micro-CT, and the three-dimensional overall view (D). The region of ROI was defined as a 700 μm × 700 μm × 700 μm cube in the mesial alveolar bone interval in the middle 1/3 of the distal and labial root, 200 μm from the root surface.
The left half of the maxilla of each sample was fixed in 4% formaldehyde with pH7.2 buffered with 0.1 M sodium phosphate for 24 h, and then decalcified in EDTA (EDTA 10%, 0.5 M) at 4°C for 6–8 weeks until the needle tip could pierce the teeth without resistance, and then embedded in paraffin for subsequent tissue sections. A series of 4 μm parasagittal sections were fixed on a glass slide. Cut the slice from proximal to distal along the long axis of the tooth. For each staining method, 10 slices containing the complete morphology of the proximal root of M1 were selected from each sample.
HEmatoxylin-eosin (He) staining was used to observe the histological changes. Masson staining was performed to observe collagen fibers. We also measured the contents of collagen fibers on the pressure side and the tension side of M1 in each standard visual field of the selected slice. The volume fraction (CVF) of collagen was calculated by ImageJ (ImageJ 1.53b, National Institutes of Health). Tartaric acid phosphatase (TRAP) staining was used to observe osteoclasts and cementum-destroying cells. Three standard visual fields, the neck, the middle third of the root and the apical area, were selected on each side and observed under 40 times magnification. The number of red stained nucleated cells in every three standard visual fields was calculated for each slice and averaged. All analyses were performed by calibrated blind reviewers. The same inspector measures each part twice.
Samples of organs (heart, liver, spleen, lung and kidney) of rats were collected after execution. All the collected organ samples were fixed in 10% neutral formaldehyde fixative, dehydrated, embedded and stained with HE, and the possible pathological changes of organs were observed.
SPSS 23.0 software (IBM, Chicago, il) was used for statistical analysis. Shapiro-wilk test and D’Agostino-Pearson test are used to test the normal distribution. Normal variables are expressed as average standard deviation, while non-normal variables are expressed as median and interquartile interval. Using parameter test ANOVA and Tukey back testing to compare normal distribution variables. For the data with non-normal distribution, Kruskal-Wallis test and Dunn back testing are used. Statistical test is used to evaluate whether there are statistically significant differences between groups. The difference is considered significant at the level of p < 0.05.
The sagittal section of the sample injected with fluorescently labeled BMP-2 shows the distribution of exogenous BMP-2 in the periodontal tissue of orthodontic teeth (Figure 3). Under confocal microscope, the fluorescently labeled BMP-2 was green. On the first day, the drug was mainly concentrated in periodontal tissue, less distributed in alveolar bone, and gradually spread to the surrounding alveolar bone on the second day. On the third day, the green fluorescent coloring drugs were mainly distributed in alveolar bone. Regardless of the injection site, BMP-2 was distributed in the proximal root of M1 on the pressure side and the tension side.
FIGURE 3. Distribution of exogenous BMP-2 in periodontal tissues of orthodontic tooth. The fluorescently labeled BMP-2 appeared green in color. Scale bar = 200 μm. AB, alveolar bone; D, dentin; PDL, periodontal ligament; P, dental pulp. Yellow arrows, pressure side of the root. Red arrows, tension side of the root. BMP-2 distribution could be seen in both pressure side and the tension side M1’s mesial root.
By comparing the tooth movement of group C and group H (Figure 4), the OTM model was successfully established. The tooth movement distance of group H without orthodontic appliance was 20 μm. There was no statistical difference in tooth movement distance between PBMP-2 group and TBMP-2 group (p > 0.05), and the movement distance of both groups was significantly shorter than that of group C (p < 0.01). The tooth movement distance of PTBMP-2 group was significantly shorter than that of C group, PBMP-2 group and TBMP-2 group (p < 0.01). There was no statistical difference between PTBMP-2 group and H group (p > 0.05).
FIGURE 4. Mean and standard deviation of tooth movement (μm) measured by the horizontal distance between the highest convexity on the distal surface of first molars and the highest convexity on the mesial surface of second molars (A). Representative three-dimensional constructive micro-CT views images showing tooth moving distance (B), scale bar = 1,000 μm *Significant (p < 0.05) difference between groups, **Significant (p < 0.01) difference between groups.
The CT slice from three-dimensional direction reveals the qualitative change of microstructure parameter values in ROI (Figure 5A). The BV/TV of C, PBMP-2 and TBMP-2 groups was significantly lower than that of healthy control group and PTBMP-2 group (p < 0.01) (Figure 5B). There was no difference between PBMP-2 group and TBMP-2 group (p > 0.05), and the BV/TV ratio of both groups was significantly higher than that of group C (p < 0.05). In BMD (Figure 5C), there was no statistical difference between PBMP-2 group and TBMP-2 group (p > 0.05). Bone mineral density in both groups was significantly higher than that in group C (p < 0.05). PTBMP-2 group was significantly larger than the other four groups (p < 0.01). Trends in tuberculosis. N (Figure 5D) is consistent with BMD. Tuberculosis. Compared with other groups, the Sp in group C (Figure 5E) was significantly larger (p < 0.05). Tuberculosis. Sp in PBMP-2 group was higher than that in TBMP-2 group (p < 0.05). Tuberculosis. Sp in PTBMP-2 group was significantly lower than that in other groups (p < 0.01). Tuberculosis. Th (Figure 5F) in PTBMP-2 group was significantly higher than that in other four groups (p < 0.01).
FIGURE 5. Bone remodeling observation in three dimensions of micro-CT, scale bar = 1000 μm (A). Micro-CT measurement of the microarchitecture parameters in the selected ROI of the maxillary first molars: BV/TV (B), BMD (C), Tb.N (D), Tb.Sp (E), Tb.Th (F). Root resorption volume of the mesial root of M1 cementum surface using Convex Hull algorithm (G). Data are presented as mean and standard deviation. *Significant (p < 0.05) difference between groups, **Significant (p < 0.01) difference between groups.
The results of micro-CT showed that the teeth receiving orthodontic traction showed different degrees of root resorption compared with group H (Figure 5G). After unilateral injection of bmp2, there was no significant difference in root resorption between the experimental group and the control group. The volume of root resorption pits in PTBMP-2 group was significantly larger than that in other groups (p < 0.01).
Morphological changes of periodontal tissue and quantitative determination of collagen fiber content during OTM.
On HE stained sections, it was noted that the periodontal fiber arrangement and gap width in group H were consistent (Figure 6 a–c). With the traction of orthodontic force, the mesial root of M1 periodontal space on the pressure side is thinner than that on the tension side, showing the performance of pressure. On the pressure side, the alveolar bone surface is irregular and continuous, and shows positive absorption. In addition, periodontal fibers are disordered and broken (discontinuous), and a wide gap can be seen in the section. Compared with the pressure side, the periodontal ligament tissue is wider, the periodontal fibers seem to be stretched, and the fibers are longer and arranged more orderly (Figure 6 d–o). At the same time, new bone deposition and bone formation were found on the tension side of alveolar bone surface (Figure 6 f, i, l, o). On the tension side and pressure side of PTBMP-2 group, there were significant new bone deposits (Figure 6 m, o).
FIGURE 6. Light micrographs of sagittal hematoxylin-eosin (HE) stained sections of the mesial root of left maxillary first molar (M1) in ×10 magnification and ×20 magnification on the pressure and tension sides. Red boxes, periodontal tissue of pressure side; blue boxes, periodontal tissue of tension side. Scale bar = 100 μm. AB, alveolar bone; D, dentin; PDL, periodontal ligament; P, dental pulp. *, new bone deposition.
Masson staining section showed that the collagen fibers in group H were arranged neatly, without breakage, and the staining was even (Figure 7C a–c). In the experimental group receiving orthodontic traction, the collagen fiber bundle on the pressure side of the root became narrow, partially broken into strips and disorganized, and the area percentage of blue collagen fiber was lower than that on the tension side (Figure 7C d–o). In the experimental group injected with BMP-2, compared with group C and group H, the percentage of collagen on the pressure side and tension side is larger.
FIGURE 7. Histological measurement of collagen fiber content on Masson-stained sections. The periodontal ligament was measured on the pressure (A) and tension (B) sides of the mesial root of M1. The collagen volume fraction (CVF) of each side was measured on three standard fields and averaged. Data are presented as mean and standard deviation. *Significant (p < 0.05) difference between groups, **Significant (p < 0.01) difference between groups. Light micrographs of sagittal Masson-stained sections of the mesial root of left maxillary first molar (M1) in ×10 magnification and ×20 magnification on the pressure and tension sides (C). Red boxes, periodontal tissue of pressure side; black boxes, periodontal tissue of tension side. Scale bar = 100 μm. AB, alveolar bone; D, dentin; PDL, periodontal ligament.
Collagen volume fraction (CVF) was calculated after semi-quantitative measurement and analysis of collagen content in the pressure side and tension side of the first molar. The results showed that on the pressure side (Figure 7B), the CVF of BMP-2 injection group increased compared with H and C groups, while that of blank control group decreased compared with H group (p < 0.05). In tension (Figure 7A), compared with group H, the four experimental groups have significantly increased (p < 0.05). There was no difference in CVF between T-BMP and PTBMP-2 groups (p > 0.05), but it was significantly increased compared with other groups (p < 0.05).
On the section stained by TRAP, cementum-destroying cells showed positive multinucleated giant cells on the root surface deep in the dentin absorption trap, while osteoclasts showed red multinucleated giant cells stained by trap in the absorption trap or on the alveolar bone surface (Figure 8C). The results of Trap positive cell count showed that osteoclasts in PBMP2 group were significantly more than those in C group and TBMP-2 group (Figures 8A, C). The number of cemental destruction cells in dental slices with orthodontic augmentation devices is much higher than that in group H (Figures 8B, C). Compared with the other four groups, the number of osteoclasts and cementum-destroying cells in PTBMP-2 group increased significantly.
FIGURE 8. Histological measurement of osteoclasts (A) and cementoclasts (B) on TRAP-stained sections. Data are presented as mean and standard deviation. *Significant (p < 0.05) difference between groups, **Significant (p < 0.01) difference between groups. Light micrographs of sagittal TRAP-stained sections of the mesial root of left maxillary first molar (M1) in ×10 magnification and ×40 magnification (C). →, osteoclast appeared as trap-stained red-positive multinucleated giant cells found in resorption traps or on the surface of the alveolar bone. →, cementoclasts appeared as positive multinucleated giant cells on the root surface deep within the resorption traps of dentin. Scale bar = 100 μm. AB, alveolar bone; D, dentin; PDL, periodontal ligament.
The H&E staining results of the vital organs of rats (including heart, liver, spleen, lung and kidney sections) are shown in Supplementary Files, and no abnormality or pathological morphology is observed in all tissue sections, which indicates that there is no abnormality in the functions of the vital organs of experimental animals. And it is reasonable to expect that injecting a certain dose of BMP-2 into periodontal tissue has good biological safety in vivo.
The regulation of OTM has been popularized in orthodontic research (Yamaguchi et al., 2010). Tooth movement in response to mechanical stress is a complex process (Will, 2016; Shoji-Matsunaga et al., 2017; Odagaki et al., 2018). According to the significant statistical differences in tooth movement distance, microstructure parameters and histological manifestations between BMP-2 injection group and blank control group, local application of BMP-2 around orthodontic teeth can reduce movement distance, increase collagen fiber content and enhance tooth stability. According to the difference between unilateral and bilateral injection groups, this enhancement is dose-dependent, and the distribution of BMP-2 labeled by fluorescence is position-independent. 0.5 μg/mL BMP-2 of 60 μL per tooth may be used as a drug to control orthodontic tooth movement without increasing the risk of root resorption and organ damage.
In the family of bone morphogenetic proteins (BMPs), BMP-2 can activate the formation of osteoblasts and osteoclasts, which may regulate the rate of bone remodeling (Simonet et al., 1997; Kaneko et al., 2000; Kamiya et al., 2008a; Kamiya et al., 2008b). The changes of alveolar bone provide biological basis for the designed tooth movement. The application of orthodontic force initiates the cellular and molecular activities that lead to periodontal remodeling. In the periodontal ligament area of stressed teeth, tension side and pressure side are generated during orthodontic treatment of tooth movement. Tension causes periodontal tissue to stretch, and osteoblasts (OB), alkaline phosphate (ALP), type I collagen and bone morphogenetic protein (BMPs) are gradually induced, which promotes bone formation and tissue remodeling (Wescott et al., 2007). At the same time, on the pressure side, periodontal tissue is compressed, which leads to the activation of osteoclasts and the absorption of alveolar bone. Finally, the dynamic balance is achieved, in which the bone absorption in the traction direction is dominant, which leads to the teeth moving in response to orthodontic force. If we can better understand the complex function of BMP-2 in controlling chondrocytes, osteoblasts and osteoclasts, it will be beneficial to the development of therapeutic methods. Considering the importance of experimental rat model in clarifying biological mechanism and finding new treatment methods in orthodontic field, we designed this in vivo study to study the effects of local application of BMP-2 in different parts of periodontal tissue on tooth movement and periodontal tissue remodeling.
According to the experimental rat model, orthodontic teeth move fastest between 7 and 14 days, and then slow down between 14 and 21 days (Ru et al., 2013). Based on the results of tooth movement distance within 16 days, it was found that the movement of orthodontic teeth with local injection of BMP-2 was limited compared with those without injection. As shown by the changes of microscopic parameters on micro-CT, BV/TV, BMD and Tb in BMP-2 injection group showed an upward trend. The performance of PBMP-2 and TbMP-2 groups is similar, except TB. The Sp index of PBMP-2 group was higher. Trabecular bone is an irregular network three-dimensional structure, which is an inward extension of bone cortex. It is used to store calcium and maintain bone elasticity and strength. The study on the microscopic characteristics of trabecula during orthodontic tooth movement can be used to understand the effects of various intervention measures (Gokce et al., 2008). During orthodontic tooth movement, BV/TV, Tb and other micro-parameters. Th decreased significantly on the pressure side and increased on the tension side (Ru et al., 2013). Compared with group H, this established model is obvious in group C. Applying clinically relevant amount of BMP-2 around mechanically loaded teeth will increase bone growth, thus reducing orthodontic traction resistance.
When comparing PBMP-2 and TBMP-2 groups, the injection site did not affect the clinical manifestations. Drugs injected on the tension side of periodontal ligament can be delivered to the pressure side, because periodontal ligament is a single compartment that can communicate. There are no strict definitions of pressure surface and tension surface, except for the academic term (Iglesias-Linares et al., 2012). Therefore, the injection site has nothing to do with the clinical effect of locally delivered drugs on tooth movement. In this study, the distribution of fluorescently labeled BMP-2 on the slice also confirmed this theory. The application of BMP-2 on the tension side will not stimulate tooth movement, which supports the hypothesis that the osteoclast activity and bone resorption activity on the pressure side determine the OTM speed (Li et al., 2021). At the same time, the fluorescently labeled sections showed that there was fluorescence aggregation in the dentinal tubule adjacent to the pulp, indicating that the drugs injected during periodontal period may spread along the dentinal tubule.
The results of osteoclast count showed that compared with group C, osteoclasts in PBMP-2 and TBMP-2 injection groups were activated, but bone absorption was not dominant. Collagen fibers in periodontal tissue play an important role in the changes of periodontal tissue and alveolar bone during tooth movement. The increase of type I collagen is beneficial to bone synthesis and tissue remodeling (Wescott et al., 2007). The morphological observation and semi-quantitative results of collagen content in this study confirmed the histological changes of collagen during tooth movement, and it was also found that local injection of BMP-2 into periodontal tissue increased the collagen content around orthodontic teeth (Anastasi et al., 2008).
Anchorage is one of the most challenging concepts in orthodontics. In order to achieve the desired orthodontic treatment goal, the anchorage teeth must be in a stable position to resist the back pressure exerted by the target teeth. The displacement of anchorage teeth may prolong the treatment time and reduce the clinical effect. It is reported that there are several ways to enhance anchorage, including intraoral and extraoral devices, micro-screws and drugs (Borsos et al., 2012; Fernández-González et al., 2016). Additional devices may be invasive and increase patient discomfort. Recent studies have focused more on drug therapy to directly or indirectly inhibit bone resorption and avoid unwanted tooth movement (Hudson et al., 2012; Yabumoto et al., 2013). There is no difference in the movement distance between the double dose PTBMP-2 group and the H group, indicating that there is no significant movement under 30 g traction. There were significant differences in tooth displacement and trabecular microscopic parameters between PTBMP-2 group and unilateral injection group. Compared with single injection, double injection of BMP-2 in periodontal tissue showed stronger osteogenic activity and anti-traction ability. The current results show that the osteogenic function of BMP-2 is dose-dependent to some extent. At the same time, Miyaji et al. (2006) found that BMP-2 could lead to a dose-dependent increase of osteoclasts’ dentin absorption at an in vitro dose of 400 mg/mL.
As a common complication of orthodontic tooth movement, root resorption may occur up to 100% of the time and should be avoided as much as possible (Makrygiannakis et al., 2019). Severe root resorption will lead to imbalance of crown-root ratio, tooth loosening or even falling off, and other adverse health effects. Gonzales et al. (2008) found that different orthodontic forces may lead to root resorption during orthodontic tooth movement. The root resorption increases with time and eventually tends to be stable. Excessive orthodontic force causes the root resorption to increase. On the third day after the application of force, active osteoclasts and odontoblasts could be observed. On the seventh day, the absorption of root and alveolar bone was the most obvious, and the most obvious change occurred in the top 1/3 of the pressure side (Verna et al., 1999; Tomizuka et al., 2007). There was no significant difference in root resorption volume between unilateral BMP-2 injection group and group C, indicating that proper injection of BMP-2 into periodontal tissue of orthodontic teeth did not promote root resorption. On the contrary, compared with other experimental groups, bilateral BMP-2 injection leads to high local tissue concentration of drugs, stimulates cementum destruction activity, and leads to significant root absorption. As a part of the current research, we use the mature method developed by Harris et al. (2006) to calculate the volume of root absorption pit by using Micro-CT root model and convex Hell algorithm. Micro-CT can analyze tooth movement, three-dimensional structure of root surface and microstructure parameters of alveolar bone without destroying the integrity of samples, and provide enhanced accuracy, reproducibility and technical sensitivity. Different from the reconstruction model in traditional methods (Chan and Darendeliler, 2004; Chan et al., 2004), the three-dimensional root model obtained by micro-CT is real, not simulated by computer, which leads to more accurate and real measurement.
It is a promising clinical application strategy to combine bone homeostasis regulator with innovative drug delivery system or program. According to the research results, periodontal application of BMP-2 seems to be a potential treatment method, which can accurately correct tooth movement without increasing root resorption. However, too high local concentration of BMP-2 may lead to root resorption on the pressure side of the root and should be avoided. Care must be taken to prevent drug accumulation. However, this study has potential limitations. It needs a site-specific drug delivery method with long-term effect to determine the best regulator with maximum effectiveness and minimum side effects.
This in vivo study shows that local application of BMP-2 around orthodontic teeth can enhance bone mass and tooth anchorage and help reduce tooth movement. Injecting BMP-2 into periodontal tissue restricts the movement of teeth, rather than accelerating the movement of orthodontic teeth. When administered around orthodontic teeth in a clinical amount, osteogenesis effects of BMP-2 were dose-dependent and site-independent. High levels of BMP-2 in the periodontal tissue of orthodontic teeth may activate osteoclasts and cementoclasts, causing aggressive root resorption. Therefore, we believe local application of BMP-2 has the potential to precisely regulate orthodontic tooth movement and periodontal tissue remodeling with in vivo biosafety.
The original contributions presented in the study are included in the article/Supplementary Material, further inquiries can be directed to the corresponding authors.
The studies involving human participants were reviewed and approved by the Fourth Medical Centre, Chinese PLA General Hospital. The patients/participants provided their written informed consent to participate in this study.
YZ, LL, XH, and YL contributed to conception, design, and critically revised the manuscript; MW contributed to data acquisition and drafted the manuscript; JF, AW, and XJ contributed to data acquisition and interpretation, and performed all statistical analyses; ZZ and XH contribute to software use and made figures; All authors gave their final approval and agree to be accountable for all aspects of the work.
This study was funded by Clinical Research Support Fund (No. 2018FC-304M-TSYS-05), Capital Clinical characteristic application research and achievement promotion project (No. Z171100001017154), PLA General Hospital transformation project (No. 2017TM-030), Capital’s Funds for Health Improvement and Research (No. CFH 2020-2-5021), Exploration project to improve the quality of standardized training for resident Doctors in 2022, Healthcare Project in 2022 (No. 22JSZ13), Haidian Frontier Project of Beijing Natural Science Foundation (No. L212067), Key Military Medical Projects (No. BLB20J001), National Natural Science Foundation of China (No. 82151312), Capital clinical diagnosis and treatment technology research and transformation application project (No. Z201100005520060), and Open Project of state key laboratory of military stomatology (No. 2019KA01).
The authors declare that the research was conducted in the absence of any commercial or financial relationships that could be construed as a potential conflict of interest.
All claims expressed in this article are solely those of the authors and do not necessarily represent those of their affiliated organizations, or those of the publisher, the editors and the reviewers. Any product that may be evaluated in this article, or claim that may be made by its manufacturer, is not guaranteed or endorsed by the publisher.
The Supplementary Material for this article can be found online at: https://www.frontiersin.org/articles/10.3389/fphys.2023.1111857/full#supplementary-material
ALP, alkaline phosphate; BMP-2, bone morphogenetic protein-2; BMD, bone mineral density; BMPs, bone morphogenetic proteins; CT, computed tomography; CVF, collagen volume fraction; HE, Hematoxylin-eosin; OTM, Orthodontic tooth movement; OPG, osteoprotegerin; OC, osteoclasts; PDL, periodontal ligament; PAOO, periodontally accelerated osteogenic orthodontics; PBMP-2, 2-Pressure BMP-2; PTBMP-2, 4-Pressure and Tension BMP-2; ROI, region of interest; TBMP-2, 3-Tension BMP-2; TRAP, Tartrate-resistant acid phosphatase.
Anastasi, G., Cordasco, G., Matarese, G., Rizzo, G., Nucera, R., Mazza, M., et al. (2008). An immunohistochemical, histological, and electron-microscopic study of the human periodontal ligament during orthodontic treatment. Int. J. Mol. Med. 21, 545–554. doi:10.3892/ijmm.21.5.545
Baloul, S. S., Gerstenfeld, L. C., Morgan, E. F., Carvalho, R. S., Van Dyke, T. E., and Kantarci, A. (2011). Mechanism of action and morphologic changes in the alveolar bone in response to selective alveolar decortication-facilitated tooth movement. Am. J. Orthod. Dentofac. Orthop. 139, S83–S101. doi:10.1016/j.ajodo.2010.09.026
Borsos, G., Vokó, Z., Gredes, T., Kunert-Keil, C., and Vegh, A. (2012). Tooth movement using palatal implant supported anchorage compared to conventional dental anchorage. Ann. Anat. 194, 556–560. doi:10.1016/j.aanat.2012.08.003
Chan, E. K., and Darendeliler, M. A. (2004). Exploring the third dimension in root resorption. Orthod. Craniofac Res. 7, 64–70. doi:10.1111/j.1601-6343.2004.00280.x
Chan, E. K., Darendeliler, M. A., Jones, A. S., and Kaplin, I. J. (2004). A calibration method used for volumetric measurement of orthodontically induced root resorption craters. Ann. Biomed. Eng. 32, 880–888. doi:10.1023/b:abme.0000030263.68615.82
Fernández-González, F. J., Cañigral, A., López-Caballo, J. L., Brizuela, A., Cobo, T., de Carlos, F., et al. (2016). Recombinant osteoprotegerin effects during orthodontic movement in a rat model. Eur. J. Orthod. 38, 379–385. doi:10.1093/ejo/cjv056
Gao, J., Nguyen, T., Oberoi, S., Oh, H., Kapila, S., Kao, R. T., et al. (2021). The significance of utilizing A corticotomy on periodontal and orthodontic outcomes: A systematic review and meta-analysis. Biology 10, 803. doi:10.3390/biology10080803
Gokce, S., Bengi, A. O., Akin, E., Karacay, S., Sagdic, D., Kurkcu, M., et al. (2008). Effects of hyperbaric oxygen during experimental tooth movement. Angle Orthod. 78, 304–308. doi:10.2319/022507-96.1
Gonzales, C., Hotokezaka, H., Yoshimatsu, M., Yozgatian, J. H., Darendeliler, M. A., and Yoshida, N. (2008). Force magnitude and duration effects on amount of tooth movement and root resorption in the rat molar. Angle Orthod. 78, 502–509. doi:10.2319/052007-240.1
Harris, D. A., Jones, A. S., and Darendeliler, M. A. (2006). Physical properties of root cementum: Part 8. Volumetric analysis of root resorption craters after application of controlled intrusive light and heavy orthodontic forces: A microcomputed tomography scan study. Am. J. Orthod. Dentofac. Orthop. 130, 639–647. doi:10.1016/j.ajodo.2005.01.029
Hudson, J. B., Hatch, N., Hayami, T., Shin, J. M., Stolina, M., Kostenuik, P. J., et al. (2012). Local delivery of recombinant osteoprotegerin enhances postorthodontic tooth stability. Calcif. Tissue Int. 90, 330–342. doi:10.1007/s00223-012-9579-4
Iglesias-Linares, A., Yañez-Vico, R. M., Moreno-Fernandez, A. M., Mendoza-Mendoza, A., and Solano-Reina, E. (2012). Corticotomy-assisted orthodontic enhancement by bone morphogenetic protein-2 administration. J. Oral Maxillofac. Surg. 70, e124–e132. doi:10.1016/j.joms.2011.10.020
Isola, G., Ferlito, S., and Rapisarda, E. (2019). Low-level laser therapy increases interleukin-1β in gingival crevicular fluid and enhances the rate of orthodontic tooth movement. Am. J. Orthod. Dentofac. Orthop. 155, 456–457. doi:10.1016/j.ajodo.2019.01.004
Kamiya, N., and Mishina, Y. (2011). New insights on the roles of BMP signaling in bone-A review of recent mouse genetic studies. Biofactors 37, 75–82. doi:10.1002/biof.139
Kamiya, N., Ye, L., Kobayashi, T., Lucas, D. J., Mochida, Y., Yamauchi, M., et al. (2008). Disruption of BMP signaling in osteoblasts through type IA receptor (BMPRIA) increases bone mass. J. Bone Min. Res. 23, 2007–2017. doi:10.1359/jbmr.080809
Kamiya, N., Ye, L., Kobayashi, T., Mochida, Y., Yamauchi, M., Kronenberg, H. M., et al. (2008). BMP signaling negatively regulates bone mass through sclerostin by inhibiting the canonical Wnt pathway. Development 135, 3801–3811. doi:10.1242/dev.025825
Kaneko, H., Arakawa, T., Mano, H., Kaneda, T., Ogasawara, A., Nakagawa, M., et al. (2000). Direct stimulation of osteoclastic bone resorption by bone morphogenetic protein (BMP)-2 and expression of BMP receptors in mature osteoclasts. Bone 27, 479–486. doi:10.1016/s8756-3282(00)00358-6
King, G. J., Keeling, S. D., McCoy, E. A., and Ward, T. H. (1991). Measuring dental drift and orthodontic tooth movement in response to various initial forces in adult rats. Am. J. Orthod. Dentofac. Orthop. 99, 456–465. doi:10.1016/s0889-5406(05)81579-3
Kurohama, T., Hotokezaka, H., Hashimoto, M., Tajima, T., Arita, K., Kondo, T., et al. (2017). Increasing the amount of corticotomy does not affect orthodontic tooth movement or root resorption, but accelerates alveolar bone resorption in rats. Eur. J. Orthod. 39, 277–286. doi:10.1093/ejo/cjw038
Lee, S-Y., Moon, J-S., Yang, D-W., Yoo, H-I., Jung, J-Y., Kim, O-S., et al. (2021). SLPI in periodontal Ligament is not sleepy during biophysical force-induced tooth movement. J. Clin. Periodontol. 48 (4), 528–540. doi:10.1111/jcpe.13416
Li, Y., Zhan, Q., Bao, M., Yi, J., and Li, Y. (2021). Biomechanical and biological responses of periodontium in orthodontic tooth movement: Up-date in a new decade. Int. J. Oral Sci. 13, 20. doi:10.1038/s41368-021-00125-5
Lu, W., Zhang, X., Firth, F., Mei, L., Yi, J., Gong, C., et al. (2019). Sclerostin injection enhances orthodontic tooth movement in rats. Arch. Oral Biol. 99, 43–50. doi:10.1016/j.archoralbio.2018.12.011
Makrygiannakis, M. A., Kaklamanos, E. G., and Athanasiou, A. E. (2019). Effects of systemic medication on root resorption associated with orthodontic tooth movement: A systematic review of animal studies. Eur. J. Orthod. 41, 346–359. doi:10.1093/ejo/cjy048
Marcantonio, C. C., Nogueira, A. V. B., Leguizamón, N. D. P., de Molon, R. S., Lopes, M. E. S., Silva, R. C. L., et al. (2021). Effects of obesity on periodontal tissue remodeling during orthodontic movement. Am. J. Orthod. Dentofac. Orthop. 159, 480–490. doi:10.1016/j.ajodo.2019.12.025
Melsen, B. (2001). Tissue reaction to orthodontic tooth movement-a new paradigm. Eur. J. Orthod. 23, 671–681. doi:10.1093/ejo/23.6.671
Miyaji, H., Sugaya, T., Kato, K., Kawamura, N., Tsuji, H., and Kawanami, M. (2006). Dentin resorption and cementum-like tissue formation by bone morphogenetic protein application. J. Periodontal Res. 41, 311–315. doi:10.1111/j.1600-0765.2006.00878.x
Nogueira, A. V., de Molon, R. S., Nokhbehsaim, M., Deschner, J., and Cirelli, J. A. (2017). Contribution of biomechanical forces to inflammation-induced bone resorption. J. Clin. Periodontol. 44, 31–41. doi:10.1111/jcpe.12636
Nogueira, A. V. B., Marcantonio, C. C., de Molon, R. S., Leguizamón, N. D. P., Silva, R. C. L., Deschner, J., et al. (2021). Experimental models of orthodontic tooth movement and their effects on periodontal tissues remodelling. Arch. Oral Biol. 130, 105216. doi:10.1016/j.archoralbio.2021.105216
Odagaki, N., Ishihara, Y., Wang, Z., Ei Hsu Hlaing, E., Nakamura, M., Hoshijima, M., et al. (2018). Role of osteocyte-PDL crosstalk in tooth movement via SOST/sclerostin. J. Dent. Res. 97, 1374–1382. doi:10.1177/0022034518771331
Razzouk, S., and Sarkis, R. (2012). BMP-2: Biological challenges to its clinical use. N. Y. State Dent. J. 78, 37–39.
Ru, N., Liu, S. S., Zhuang, L., Li, S., and Bai, Y. (2013). In vivo microcomputed tomography evaluation of rat alveolar bone and root resorption during orthodontic tooth movement. Angle Orthod. 83, 402–409. doi:10.2319/031312-219.1
Sakamoto, M., Fukunaga, T., Sasaki, K., Seiryu, M., Yoshizawa, M., Takeshita, N., et al. (2019). Vibration enhances osteoclastogenesis by inducing RANKL expression via NF-κB signaling in osteocytes. Bone 123, 56–66. doi:10.1016/j.bone.2019.03.024
Shoji-Matsunaga, A., Ono, T., Hayashi, M., Takayanagi, H., Moriyama, K., and Nakashima, T. (2017). Osteocyte regulation of orthodontic force-mediated tooth movement via RANKL expression. Sci. Rep. 7, 8753. doi:10.1038/s41598-017-09326-7
Simonet, W. S., Lacey, D. L., Dunstan, C. R., Kelley, M., Chang, M. S., Lüthy, R., et al. (1997). Osteoprotegerin: A novel secreted protein involved in the regulation of bone density. Cell 89, 309–319. doi:10.1016/s0092-8674(00)80209-3
Sivarajan, S., Doss, J. G., Papageorgiou, S. N., Cobourne, M. T., and Wey, M. C. (2019). Mini-implant supported canine retraction with micro-osteoperforation: A split-mouth randomized clinical trial. Angle Orthod. 89, 183–189. doi:10.2319/011518-47.1
Stenport, V. F., Roos-Jansåker, A. M., Renvert, S., Kuboki, Y., Irwin, C., Albrektsson, T., et al. (2003). Failure to induce supracrestal bone growth between and around partially inserted titanium implants using bone morphogenetic protein (BMP): An experimental study in dogs. Clin. Oral Implants Res. 14, 219–225. doi:10.1034/j.1600-0501.2003.00861.x
Sugimori, T., Yamaguchi, M., Shimizu, M., Kikuta, J., Hikida, T., Hikida, M., et al. (2018). Micro-osteoperforations accelerate orthodontic tooth movement by stimulating periodontal ligament cell cycles. Am. J. Orthod. Dentofac. Orthop. 154, 788–796. doi:10.1016/j.ajodo.2018.01.023
Tomizuka, R., Shimizu, Y., Kanetaka, H., Suzuki, A., Urayama, S., Kikuchi, M., et al. (2007). Histological evaluation of the effects of initially light and gradually increasing force on orthodontic tooth movement. Angle Orthod. 77, 410–416. 0410:heoteo]2.0.co;2. doi:10.2319/0003-3219(2007)077[0410:HEOTEO]2.0.CO;2
Vercellotti, T., and Podesta, A. (2007). Orthodontic microsurgery: A new surgically guided technique for dental movement. Int. J. Periodontics Restor. Dent. 27, 325–331.
Verna, C., Zaffe, D., and Siciliani, G. (1999). Histomorphometric study of bone reactions during orthodontic tooth movement in rats. Bone 24, 371–379. doi:10.1016/s8756-3282(99)00009-5
Wescott, D. C., Pinkerton, M. N., Gaffey, B. J., Beggs, K. T., Milne, T. J., and Meikle, M. C. (2007). Osteogenic gene expression by human periodontal ligament cells under cyclic tension. J. Dent. Res. 86, 1212–1216. doi:10.1177/154405910708601214
Will, L. A. (2016). Orthodontic tooth movement: A historic prospective. Front. Oral Biol. 18, 46–55. doi:10.1159/000351899
Yabumoto, T., Miyazawa, K., Tabuchi, M., Shoji, S., Tanaka, M., Kadota, M., et al. (2013). Stabilization of tooth movement by administration of reveromycin A to osteoprotegerin-deficient knockout mice. Am. J. Orthod. Dentofac. Orthop. 144, 368–380. doi:10.1016/j.ajodo.2013.04.016
Yamaguchi, M., Hayashi, M., Fujita, S., Yoshida, T., Utsunomiya, T., Yamamoto, H., et al. (2010). Low-energy laser irradiation facilitates the velocity of tooth movement and the expressions of matrix metalloproteinase-9, cathepsin K, and alpha(v) beta(3) integrin in rats. Eur. J. Orthod. 32, 131–139. doi:10.1093/ejo/cjp078
Yoo-Sung, N., Dong-Wook, Y., Jung-Sun, M., Kang, J. H., Cho, J. H., et al. Sclerostin in periodontal ligament: Homeostatic regulator in biophysical force-induced tooth movement. J. Clin. Periodontol. 2022:49:932–944. doi:10.1111/jcpe.13624
Keywords: orthodontics, bone remodeling, osteoclastogenesis, osteogenesis, anchorage, local delivery
Citation: Wang M, Fan J, Wang A, Jin X, Zhang Z, Hu X, Liu L, Zhao Y and Li Y (2023) Effect of local application of bone morphogenetic protein -2 on experimental tooth movement and biological remodeling in rats. Front. Physiol. 14:1111857. doi: 10.3389/fphys.2023.1111857
Received: 05 December 2022; Accepted: 27 March 2023;
Published: 18 April 2023.
Edited by:
Dimitrios Kloukos, University of Bern, SwitzerlandReviewed by:
Eleftherios Kaklamanos, Aristotle University of Thessaloniki, GreeceCopyright © 2023 Wang, Fan, Wang, Jin, Zhang, Hu, Liu, Zhao and Li. This is an open-access article distributed under the terms of the Creative Commons Attribution License (CC BY). The use, distribution or reproduction in other forums is permitted, provided the original author(s) and the copyright owner(s) are credited and that the original publication in this journal is cited, in accordance with accepted academic practice. No use, distribution or reproduction is permitted which does not comply with these terms.
*Correspondence: Le Liu, bGl1bGU1NTU1QDEyNi5jb20=; Yantao Zhao, dXNlcnp5dEBxcS5jb20=; Yanfeng Li, OTQ5NDI3Nzc5QHFxLmNvbQ==
†These authors have contributed equally to this work
‡Lead contact
Disclaimer: All claims expressed in this article are solely those of the authors and do not necessarily represent those of their affiliated organizations, or those of the publisher, the editors and the reviewers. Any product that may be evaluated in this article or claim that may be made by its manufacturer is not guaranteed or endorsed by the publisher.
Research integrity at Frontiers
Learn more about the work of our research integrity team to safeguard the quality of each article we publish.