- 1Central Laboratory, Liaoning Cancer Hospital & Institute, Cancer Hospital of China Medical University, Shenyang, China
- 2Department of Medical Image, Liaoning Cancer Hospital & Institute, Cancer Hospital of China Medical University, Shenyang, China
- 3Department of Neurosurgery, Liaoning Cancer Hospital & Institute, Cancer Hospital of China Medical University, Shenyang, China
Purpose: To analyze and review the progress of glucose metabolism-based molecular imaging in detecting tumors to guide clinicians for new management strategies.
Summary: When metabolic abnormalities occur, termed the Warburg effect, it simultaneously enables excessive cell proliferation and inhibits cell apoptosis. Molecular imaging technology combines molecular biology and cell probe technology to visualize, characterize, and quantify processes at cellular and subcellular levels in vivo. Modern instruments, including molecular biochemistry, data processing, nanotechnology, and image processing, use molecular probes to perform real-time, non-invasive imaging of molecular and cellular events in living organisms.
Conclusion: Molecular imaging is a non-invasive method for live detection, dynamic observation, and quantitative assessment of tumor glucose metabolism. It enables in-depth examination of the connection between the tumor microenvironment and tumor growth, providing a reliable assessment technique for scientific and clinical research. This new technique will facilitate the translation of fundamental research into clinical practice.
Introduction
Metabolic reprogramming is a cancer characteristic in which cells are rewired to enable optimized neoplastic development (Madhavan and Nagarajan, 2020; Lin et al., 2022). Typically, differentiated cells rely on oxidative phosphorylation within mitochondria to produce the energy required for cellular processes. In contrast, most cancer cells rely on aerobic glycolysis, a phenomenon known as the “Warburg effect” (Sieow et al., 2023). Given the dramatic increase in glucose absorption during this process, glycolysis is a promising early target for cancer therapy (Rangel Rivera et al., 2021). Therefore, study of cancer metabolism is important for understanding its basic pathophysiology and clinical oncology (Karlstaedt et al., 2021). This review focuses on the processes behind the non-invasive study of glucose metabolism dysregulation in cancer.
The critical role of aerobic glycolysis in tumor metabolism
A greater understanding of the interplay between systemic and tumor metabolism could uncover new treatment targets (Agbu and Carthew, 2021). Active glycolysis pathways, decreased oxygen consumption, and enhanced glucose absorption are hallmarks of solid malignant tumors. At the cellular level, glucose enters tumor cells by glucose transporters. Subsequently, it is transformed into pyruvate by a series of glycolytic enzymes. Finally, lactate dehydrogenase converts pyruvate into lactate (Guo et al., 2022; Norton et al., 2022) (Figure 1). As a proinflammatory and immunosuppressive mediator, lactic acid contributes to the malignant growth of tumors, and its accumulation in significant amounts alters the pH of the tumor microenvironment (De Jesus et al., 2022). Lactic acid can also activate matrix metalloproteinase (MMP) and directly enhance cell migration ability (Broadfield et al., 2021). In conclusion, the glucose metabolic process is helpful for tumor cell proliferation, invasion, and metastasis, as well as radiotherapy and chemotherapy resistance, which complicates treatment decisions (Zhu and Thompson, 2019; Gao et al., 2023).
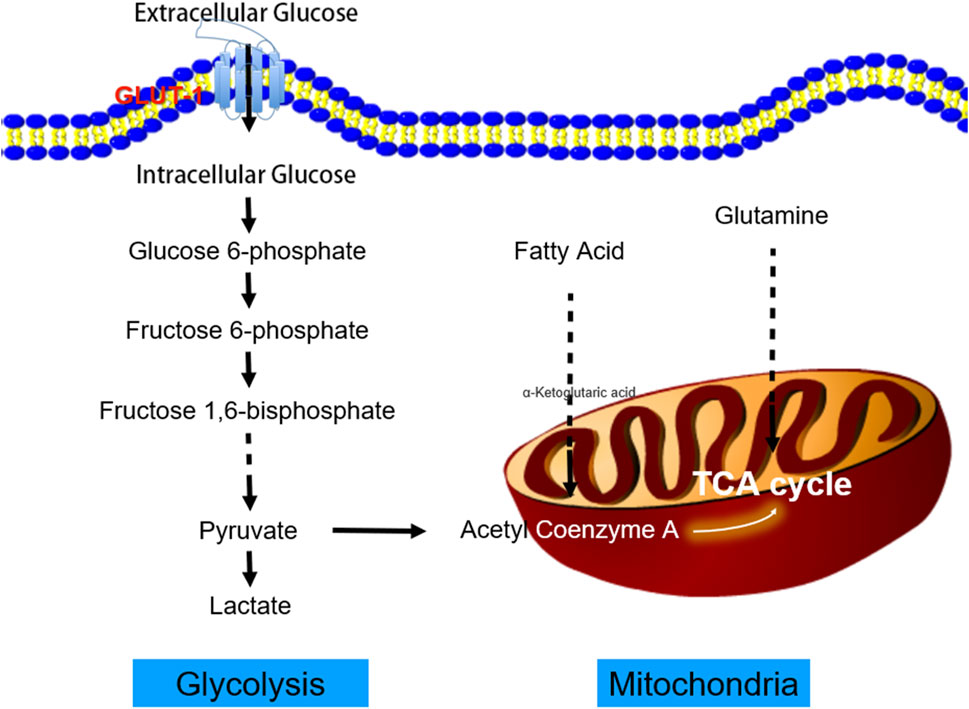
FIGURE 1. The process of tumor aerobic glycolysis. Cancer cells increase the uptake of glucose and the production of lactic acid by enhancing aerobic glycolysis. Glucose provides the primary carbon source for the tricarboxylic acid cycle (TCA). Cancer cells use the glycolytic pathway to rapidly produce adenosine triphosphate (ATP) and its intermediate product as a carbon source to synthesize macromolecular compounds, for example, to provide a sufficient carbon source for the pentose phosphate pathway to generate 5-phosphate ribose backbone. The reduced nicotinamide adenine dinucleotide phosphate (NADPH) produced by the pentose phosphate pathway can maintain the cell’s redox homeostasis. In addition, the lactic acid produced by glycolysis can promote the acidification of the tumor microenvironment, which is conducive to tumor cell invasion and migration.
Molecular imaging enables visualization of glycolytic effects
Medical imaging techniques such as nuclear medicine, magnetic resonance imaging (MRI), and optical imaging, are at the heart of molecular imaging technology. These allow for non-invasive, real-time imaging of the cellular and molecular levels of physiological and pathological processes inside the human body (Tian et al., 2021; Hod et al., 2023). Owing to advancements in artificial intelligence and innovative imaging agents, molecular imaging may soon become an essential part of therapeutic care (Rowe and Pomper, 2022). The fluorine-18-fluorodeoxyglucose (18F-FDG) positron emission tomography (PET) is a functional molecular imaging technique that takes advantage of the enhanced glycolysis of cancer cells to obtain both structural information and metabolic activity (Vaarwerk et al., 2021). PET with innovative radiotracers and MR-based approaches provides exciting new ways to study glycolysis and broadens the metabolic imaging toolkit (Edmonds et al., 2022). To completely define tumor biology and better guide therapy, multiparametric imaging must combine various modalities.
Principal patterns of molecular imaging to detect glycolytic effects
PET imaging of tumor glycolysis using pyruvate kinase M2 (PKM2)
The core of tumor metabolism is the glycolytic pathway, which promotes tumor growth through the production of ATP and synthesis of biosynthetic reaction intermediates. PKM2 catalyzes the final rate-limiting steps in tumor glycolysis and controls the balance between energy production and synthesis of metabolic precursors (Ouyang et al., 2018). Considering the importance of PKM2 in regulating tumor metabolism, scientists have attempted to measure its expression non-invasively by developing specific radioactive preparations of PKM2. Witney et al. (2015) described the manufacture and assessment of [11C]DASA-23, a PET radiotracer that offers a direct, non-invasive measurement of PKM2 expression in preclinical models of glioblastoma multiforme (GBM). This study laid the foundation for the clinical use of [11C]DASA-23, which could be used for imaging primary brain tumors and other tumors that may metastasize to the brain. Beinat et al. (2017); Beinat et al., 2020b) reported that a novel radiotracer, 1-((2-fluoro- 6-[18F]fluorophenyl)sulfonyl)-4-((4-methoxyphenyl)sulfonyl)piperazine ([18F]DASA- 23), can be safely used to evaluate pyruvate kinase M2 levels. Its feasibility and safety were further verified through animal experiments and clinical trials (Saidi et al., 2018; Beinat et al., 2021).
18F-FDG-PET/CT was used to visualize the metabolic activity of surviving tumor cells
FDG is a radiolabeled glucose analog that is transported into tumor cells and phosphorylated by hexokinase (Dezhakam et al., 2022). Therefore, FDG-PET can indicate the need for glucose by cells or tumors and provide some information about the tumor’s processing of glucose into usable components (Cossu et al., 2019). Yu and his team (Liang et al., 2020) used 18F-FDG-PET/CT to visualize the metabolic activity of surviving tumor cells. The maximum standardized uptake value (SUVmax) has been used as a prognostic indicator of pancreatic ductal adenocarcinoma (PDAC). PET/CT volume parameters, metabolic tumor volume (MTV), and total lesion glycolysis (TLG) fully reveal tumor metabolic activity and volume. The transition to aerobic glycolysis is a characteristic of advanced cancers and is easily assessed by 18F-FDG-PET (Zhao et al., 2022). A crucial route governing the development and metabolism of these malignancies is the mechanistic target of the rapamycin (mTOR) pathway, which can be efficiently addressed by utilizing selective catalytic mTOR kinase inhibitors (Ferrara and Roz, 2022). This was investigated in mice with lung cancer, where therapeutic response was evaluated using 18F-FDG-PET and computed tomography (CT) imaging before and after the administration of mTOR inhibitor MLN0128 (Momcilovic et al., 2018a; Momcilovic et al., 2018b). After targeted therapy intervention, the results demonstrated that 18F-FDG-PET/CT could evaluate dynamic changes in glucose metabolism in lung cancer within mice.
Lactate concentration was measured by proton magnetic resonance spectroscopy (1H-MRS) and SUV by FDG-PET
PET detection of the radioactive glucose analog 18FDG is the only commonly accessible metabolic imaging technology in current clinical practice. However, 18FDG-PET findings are unclear in tissues with intrinsically high glucose absorption, such as the brain, and provides little information on the metabolic processes that occur after glucose intake (De Feyter et al., 2018; Zhang et al., 2022). 1H-MRS is a non-invasive imaging tool for brain function that can reliably detect and quantitatively evaluate brain metabolites for subjective identification of gliomas. 1H-MRS has good accuracy and specificity in diagnosis and grading, as well as the ability to assess the glioma lesion spectrum (Rodriguez-Nieto et al., 2023). This technology is also suitable for simultaneously analyzing multiple target tissue regions, thereby enabling 1H-MRS imaging (1H-MRSI). 1H-MRSI generates a “voxel” table, which can “map” lactate levels (Corbin, 2019; Lussey-Lepoutre et al., 2020). Esfahani et al. (2022) evaluated the use of hyperpolarized (HP) [1-13C]pyruvate magnetic resonance spectroscopic imaging (HP-13C MRSI) to quantitatively measure early changes in glycolytic metabolism and its ability to predict the response to pan-tyrosine kinase inhibitor (pan-TKI) therapy in gastric cancer (GC). HP-13C MRSI was found to be a more representative biomarker of early metabolic changes in response to pan-TKIs in GC than 18F-FDG-PET, and can be used for the early prediction of response to targeted therapies. The combination of FDG-PET and MRSI may provide a more sensitive clinical indicator of aerobic glycolysis (Casey et al., 2020; Tarumi et al., 2020). Therefore, an imaging technology that can identify aerobic glycolysis in tumors non-invasively would enhance cancer research and monitoring (Figure 2; Table 1 (Witney et al., 2015; Beinat et al., 2017; Momcilovic et al., 2018a; Beinat et al., 2018; Momcilovic et al., 2018b; Choi et al., 2018; Hundshammer et al., 2018; Saidi et al., 2018; Scroggins et al., 2018; Zhao et al., 2018; Beinat et al., 2020a; Beinat et al., 2020b; Yamamoto et al., 2020; Beinat et al., 2021; Esfahani et al., 2022)).
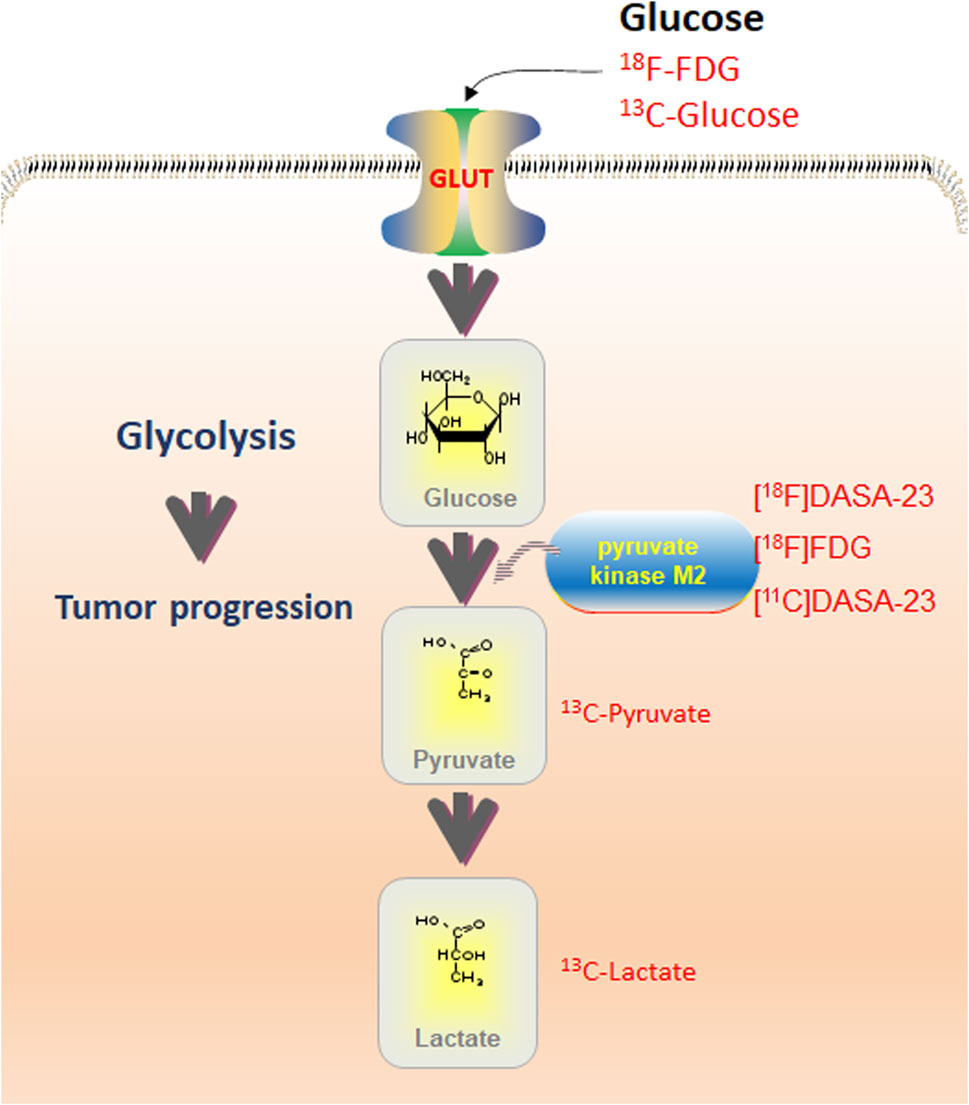
FIGURE 2. Overview of glycolysis routes detectable with various imaging techniques. PET with 18F-FDG may detect glucose uptake; MRS with 13C-Glucose can be used for optical imaging. Pyruvate kinase M2 (PKM2) is detectable with PET utilizing [18F]DASA-23, [18F]FDG, and [11C]DASA-23. Contribution of pyruvate and lactate may be assessed using 13C-Pyruvate and 13C-Lactate utilizing MRS. Combining imaging modalities with quantitative immunohistochemistry allows the assessment of total protein levels and phosphorylation.
Molecular imaging technology combined with glycolysis products
FDG-PET in conjunction with HP MRI
HP MRI provides additional information that cannot be inferred from FDG-PET or hypoxia measures (Zhao et al., 2018). The tissue contrast of 18F-FDG-PET in the prostate is poor and its application is complicated because of its proximity to the excretory system. In addition, the fundamental change in prostate cancer is a change in carbon use, not just glucose uptake. Therefore, after the injection of HP [1-13C] pyruvate, rapid 13C MR was used to measure the metabolic conversion of this aggressive cancer and its response to treatment, with direct biological significance (Fantin et al., 2006; Keshari et al., 2013a; Keshari et al., 2013b; Wilson and Kurhanewicz, 2014). Using this approach, Qi et al. (2022) utilized 18F-FDG-PET and HP [1-13C]pyruvate MRSI with chemical exchange saturation transfer (CEST) MRI measures to investigate the effect of glucose infusion on intracellular pH and its relationship to the tumor.
Chemokine receptor-4 targeted PET/CT with 68Ga-Pentixafor
While 18F-FDG-PET/CT glucose absorption measurements provides useful metabolic information for evaluating most malignant tumors it is limited in evaluating multiple myeloma (MM) (Zamagni et al., 2021). Since chemokine receptor four is overexpressed in MM, Pan and his team (Pan et al., 2020) conducted a prospective cohort study to compare the performance of 68Ga-pentixafor and 18F-FDG-PET/CT in newly diagnosed MM. Quantitative analysis revealed that the bone marrow uptake value of 68Ga-Pentixafor (TBmUCXCR4, SUVmax, and SUVmean) was positively correlated with tumor burden-related end-organ damage, and the stage was positively associated with laboratory biomarkers. 68Ga-Pentixafor was more abundant than 18F-FDG in newly diagnosed MM. These results indicated that 68Ga-pentixafor might be a superior biomarker to 18F-FDG for PET quantification assessment of tumor burden in newly diagnosed MM. However, further investigations are needed to determine whether this affects patient prognosis and survival.
Combined molecular imaging of glycolysis with angiogenesis
Clemmensen et al. (2021) used [68Ga] Ga-NODAGA-RGD-PET with vascular imaging and hyperpolarization [1-13C] pyruvate-MRSI to detect energy metabolism. [68Ga] Ga-NODAGA-RGD-PET and [1-13C] pyruvate-MRSI may provide additional information than either technique alone, indicating that non-invasive hyperPET combined with angiogenesis and glycolysis imaging can help to analyze cancer phenotypes. They showed that imaging of angiogenesis and tumor metabolism by [68Ga]Ga-NODAGA-RGD-PET and [1-13C] pyruvate-MRSI, respectively, is feasible in canine cancer patients.
Synergistic construction of aerobic and anaerobic glycolysis zones
As reported, tumor hypoxia and glycolysis, including low-oxygen glycolysis and anaerobic glycolysis, were predicted using 18F-FDG and 18F-fluoromisonidazole (FMISO) PET technologies (Leimgruber et al., 2020). This method has been applied in a prospective clinical trial of 10 patients with GBM who underwent 18F-FDG and 18F-FMISO PET and MRI studies after surgery, before radiotherapy, or early after radiotherapy. Spatial mapping of aerobic and anaerobic glycolysis can provide unique information regarding tumor metabolism and hypoxia through PET. This prospective preliminary study will facilitate the development of a powerful and intuitive software tool for assessing glucose metabolism under hypoxic conditions. In addition, a unique software algorithm, “Glyoxia,” has been developed (Bi et al., 2019; Moscoso et al., 2019), which can detect glycolysis and hypoxia through the automatic processing of 18F-FDG and 18F-FMISO PET images. The algorithm allows serial dual-track molecular imaging comparisons to classify and delineate hypoxic areas at a risk of recurrence. The simplicity of this method can be extended to other hypoxic cancers and possibly to other molecular imaging compounds. By understanding the glucose metabolism in GBM under hypoxic conditions, the effects of aerobic glycolysis in patients and the relationship with treatment resistance can be assessed.
A new hybrid mode—PET/MR
Precision treatment is inseparable from the development of biomedical imaging technology, aiming to identify changes in tumor biology at the cellular and molecular levels (Goutsouliak et al., 2020). The novel PET/MR hybrid imaging modality allows for the simultaneous acquisition of high-resolution anatomical images and metabolic data. The critical step of the Warburg effect, a hallmark of tumors, can be measured non-invasively using this emerging technology. Hundshammer and his team (Hundshammer et al., 2018) utilized the multimodal imaging workflow of the PET/MR system, including proton MRI, to obtain accurate morphological information and diffusion-weighted imaging (DWI) to resolve tumor cellularity. Metabolic data were measured using dynamic 18F-FDG-PET and HP 13C-pyruvate MRSI. This technique was also used to determine how cell density influences variations in glycolytic parameters that occur due to cell expansion. Researchers have found that an increase in LDH activity corresponded with increased glucose absorption by tumor cells. Longitudinal DWI data showed that cell density decreased during tumor growth, affecting the quantification of PET and MRSI data. The workflow included multiparameter and non-invasive tumor characterization, which can be applied to larger animal models. This has the potential for clinical applications, paving the way for tailored and patient-specific treatment methods.
Visualization of glucose metabolism for cancer immunotherapy
Immunotherapy replaces traditional radiotherapies and chemotherapies with immune checkpoint inhibitors, which use the patient’s immune system to identify and target cancer cells (Vernieri et al., 2022). Despite the tremendous clinical success and aggressive research on immunotherapies, there remains a considerable unmet need for a rigorous technique to identify immunotherapy responders (Kraehenbuehl et al., 2022). Early and precise monitoring of immunotherapy response is essential for optimal medication development and tailored treatment (Goncalves et al., 2021). Texture characteristics generated from PET, which are known to suggest glucose absorption distribution, have been shown to be linked with the expression of PD-L1 mRNA, but not with the expression of PD-1, CTLA-4, or TMB. To differentiate between those who will respond to treatment and those who will not, non-invasive in vivo 18F-FDG-PET/CT imaging of glucose metabolism in lymphatic organs might be a valuable preclinical and clinical technique (Schwenck et al., 2020). Vos et al. (2022) discovered 18F-FDG-PET-based DeltaMTV and DeltaTLG reliably identified pathological responses to neoadjuvant immune checkpoint blockade (ICB) in head and neck squamous cell carcinoma (HNSCC) before surgery, surpassing the European Organisation For Research And Treatment Of Cancer (EORTC) criteria. However, neck lymph nodes exhibited pseudoprogression. Upon validation, 18F-FDG-PET might identify HNSCC patients for response-driven therapy adaptation in future clinical studies. PET biomarkers based on 18F-FDG imaging have been successfully used for prognosis and therapeutic response monitoring in patients undergoing anti-PD1 immunotherapy for malignant melanoma, where hematopoietic tissue metabolism correlates adversely with survival. Additionally, compared to CT imaging alone, metabolic imaging with FDG-PET at termination of immune checkpoint inhibitor medication helps identify melanoma patients with a low risk of recurrence and favorable prognosis (Ferdinandus et al., 2022). Yang’s group (Yang et al., 2022) demonstrated the promise of sensitive and comprehensive monitoring using label-free metabolic intravital imaging (LMII) for visualizing the dynamic changes in heterogeneous cell metabolism of cancer cells and immunological infiltrates in response to immunotherapy. The uptake of 18F-FDG and 18F-fluorodeoxythymidine (FLT) on PET may serve as an imaging biomarker for assessing the efficacy of immune checkpoint inhibitor therapy (Oh et al., 2022). Another research group (Wang Y. et al., 2020) looked at how the expression of intra-tumor immunomarkers in non-small cell lung carcinoma (NSCLC) patients correlated with their metabolic status, as measured by 18F-FDG-PET/CT. These findings showed that SUVmax on 18F-FDG-PET/CT may be a valuable predictor for identifying patients for immunotherapy by revealing a link between metabolic variables and immune cell expression in the tumor microenvironment. In oral squamous cell carcinoma, the absorption of 18F-FDG is an independent predictor of cold tumors. Imaging using 18F-FDG-PET may be a viable diagnostic technique for estimating the immunological state of tumors. However, quantitative 18F-FDG-PET imaging of the spleen performed poorly in predicting clinical improvement using ipilimumab (Sachpekidis et al., 2019).
Importance in clinical practice
By leveraging the radiological properties of SUVmean and entropy on 18F-FDG-PET/CT, researchers discovered a potential benefit of 2-year progression-free survival (PFS) (Mayerhoefer et al., 2019). Moreover, when combined with clinical/laboratory and biological parameters, they could obtain more valuable PFS and prognostic information. Ceriani and his team (Ceriani et al., 2021) established a multiparameter prediction model that integrated texture features and conventional PET indicators into incidental thyroid tumors. This model can be combined with three independent PET-derived prediction parameters: TLG, SUVmax, and shape sphericity. This approach was used in a study monitoring 19 patients with malignant pleural mesothelioma (MPM) before and after two cycles (6 weeks) of chemoimmunotherapy (Reynolds et al., 2020). To determine the malignancy of [18F]-fluorodeoxyglucose-avid thyroid incidentalomas, six separate radiological characteristics were used that describe the shape, heterogeneity, and intensity of lesion tracer uptake. A threshold-based Interactive Data Language (IDL) tool was then created to quantify tumor volume in 18F-FDG-PET scans. In this MPM study, there was a causal relationship between tumor volume and TLG. Research also showed that, regardless of whether the tumor size changed or responded to therapy, the overall intake of 18F-FDG is always proportional to the total metabolite volume (TMV) of the tumor. This finding was unexpected because standard teaching indicates that changes in metabolism, measured by FDG uptake, precede changes in volume. MPM may be a condition in which FDG uptake by each cell and its proliferation vary simultaneously. Additional clinical data are listed in Table 2 (Kahraman et al., 2012; Lee et al., 2013; Maharjan et al., 2013; Usmanij et al., 2013; Bhoil et al., 2014; Boers-Sonderen et al., 2014; Choi et al., 2014; Soussan et al., 2014; Elimova et al., 2015; Tateishi et al., 2015; Wong et al., 2016; Xie et al., 2016; Lim et al., 2017; Liu et al., 2017; Park et al., 2017; Salavati et al., 2017; Calandriello et al., 2018; Kaira et al., 2018; Lovinfosse et al., 2018; Wang et al., 2018; Avallone et al., 2019; Carpenter et al., 2019; Dag et al., 2019; Mayerhoefer et al., 2019; van Berkel et al., 2019; Wang L. et al., 2020; Deckers et al., 2020; Fiore et al., 2020; Mathew et al., 2020; Mitchell et al., 2020; Pan et al., 2020; Dunet et al., 2021; Vos et al., 2022).
Through different molecular imaging technologies, the movement of cells can be tracked to determine the dynamic process of aerobic glycolysis. Molecular imaging enables researchers to observe cellular processes in their natural environment in real time, thereby significantly increasing the value and authenticity of the observations (Yamamoto et al., 2020; Chen et al., 2021; Goncalves et al., 2021). Cancer cells divide rapidly as they expand and require enormous amounts of glucose for their high metabolic rates. As a result, tumor cells in vitro will incorporate a large amount of 18F-FDG. Positrons emitted by 18F can be captured and visualized by the PET/CT detector, showing the location, shape, size, number, and distribution of glucose metabolism in the tumor (Nakamoto et al., 2021). This method efficiently evaluates alterations in the tumor’s energy metabolism region, judgement of benign/malignant status, staging of the lesion, and survival status of tumor cells after radiation or chemotherapy (Gomes et al., 2017; Ayubcha et al., 2021). PET/CT hypoxia imaging can detect the hypoxia status in tumor tissues in vivo using molecular probes. Hypoxia imaging agents such as 18F- FMISO, 18F-flortanidazole (HX)4, 18F-Fluoroazomycin Arabinoside (FAZA), and 64Cu-ATSM, have been exploited to effectively demonstrate the hypoxic status inside tumors (Ayati et al., 2021).
18F-FDG-PET/CT was shown to have 100% sensitivity and 78% specificity in monitoring mediastinal lymph node metastasis of NSCLC, according to a study published by Jimenez-Bonilla et al. (2016). Recently, Chinese researchers (Kang et al., 2016) performed 68Ga-labeled RGD peptide-alpha peptide II (68Ga-alpha II) and 18F-FDG-PET/CT imaging in patients with NSCLC and tuberculosis (TB), respectively, and 68Ga-alpha II imaging SUVmax and SUVmean. In detecting NSCLC metastatic lymph nodes, 68Ga-Alfatide II was more specific but less sensitive than 18F-FDG; the false positive rate of 68Ga-Alfatide for TB lymph node identification was much lower than that of 18F-FDG. These findings also indicate that FDG-PET may be used with other standard diagnostic procedures, such as breast ultrasonography and mammography, to further improve diagnostic accuracy. More clinical data are required to support the use of FDG-PET for evaluating lymph node metastases in the context of diagnosis (Stewart et al., 2018; Fowler and Strigel, 2022).
Conclusion
Aerobic glycolysis is a crucial characteristic of tumors and is intimately connected to the incidence and aggressive growth of tumors (Xia et al., 2021). Monitoring aerobic glycolysis in tumor tissue might lead to improved methods for diagnosing and treating cancer (Ma et al., 2022; Raskov et al., 2022). Molecular imaging is an emerging interdisciplinary subject in imaging, medicine, and molecular biology (Jan De Beur et al., 2022). Early detection, tailored treatment, and real-time tumor monitoring will benefit from non-invasive molecular imaging of glucose metabolism in cancer. However, current technology for molecular imaging has deficiencies in terms of resolution, detection limit, accessibility, and energy extension (Ambrosini et al., 2021). Thus, combining many imaging methods may broaden the possibilities of molecular imaging (Lewis et al., 2021). Future multimodal imaging must focus on safe, effective, dual-functional molecular detection and therapy of glucose metabolism to enable the widespread uptake of molecular imaging technologies.
Author contributions
GZ and JS prepared figures and tables. YM designed and wrote the manuscript. HP and TY contributed to the critical revision of the English language. YM was responsible for financial support. All authors approved the final manuscript.
Funding
This research work supported by “the Fundamental Research Funds for the Central Universities”, Natural Science Foundation (20180550488 and 2020-ZLLH-38 to YM) of Liaoning Province, Young and middle-aged technological innovation talents in Shenyang of YM (RC200491), and Excellent Talent Fund of Liaoning Province Cancer Hospital of YM.
Acknowledgments
Thanks to Liaoning Cancer Hospital for supporting scientific research.
Conflict of interest
The authors declare that the research was conducted in the absence of any commercial or financial relationships that could be construed as a potential conflict of interest.
Publisher’s note
All claims expressed in this article are solely those of the authors and do not necessarily represent those of their affiliated organizations, or those of the publisher, the editors and the reviewers. Any product that may be evaluated in this article, or claim that may be made by its manufacturer, is not guaranteed or endorsed by the publisher.
References
Agbu P., Carthew R. W. (2021). MicroRNA-mediated regulation of glucose and lipid metabolism. Nat. Rev. Mol. Cell Biol. 22, 425–438. doi:10.1038/s41580-021-00354-w
Ambrosini V., Kunikowska J., Baudin E., Bodei L., Bouvier C., Capdevila J., et al. (2021). Consensus on molecular imaging and theranostics in neuroendocrine neoplasms. Eur. J. Cancer 146, 56–73. doi:10.1016/j.ejca.2021.01.008
Avallone A., Aloj L., Pecori B., Caraco C., De Stefano A., Tatangelo F., et al. (2019). (18 F-FDG-PET/CT is an early predictor of pathologic tumor response and survival after preoperative radiochemotherapy with bevacizumab in high-risk locally advanced rectal cancer. J. Nucl. Med. 60, 1560–1568. doi:10.2967/jnumed.118.222604
Ayati N., Sadeghi R., Kiamanesh Z., Lee S. T., Zakavi S. R., Scott A. M. (2021). The value of (18)F-FDG-PET/CT for predicting or monitoring immunotherapy response in patients with metastatic melanoma: A systematic review and meta-analysis. Eur. J. Nucl. Med. Mol. Imaging 48, 428–448. doi:10.1007/s00259-020-04967-9
Ayubcha C., Revheim M. E., Newberg A., Moghbel M., Rojulpote C., Werner T. J., et al. (2021). A critical review of radiotracers in the positron emission tomography imaging of traumatic brain injury: FDG, tau, and amyloid imaging in mild traumatic brain injury and chronic traumatic encephalopathy. Eur. J. Nucl. Med. Mol. Imaging 48, 623–641. doi:10.1007/s00259-020-04926-4
Beinat C., Alam I. S., James M. L., Srinivasan A., Gambhir S. S. (2017). Development of [(18)F]DASA-23 for imaging tumor glycolysis through noninvasive measurement of pyruvate kinase M2. Mol. Imaging Biol. 19, 665–672. doi:10.1007/s11307-017-1068-8
Beinat C., Haywood T., Chen Y. S., Patel C. B., Alam I. S., Murty S., et al. (2018). The utility of [(18)F]DASA-23 for molecular imaging of prostate cancer with positron emission tomography. Mol. Imaging Biol. 20, 1015–1024. doi:10.1007/s11307-018-1194-y
Beinat C., Patel C. B., Haywood T., Murty S., Naya L., Castillo J. B., et al. (2021). A clinical PET imaging tracer ([(18)F]DASA-23) to monitor pyruvate kinase M2-induced glycolytic reprogramming in glioblastoma. Clin. Cancer Res. 27, 6467–6478. doi:10.1158/1078-0432.CCR-21-0544
Beinat C., Patel C. B., Haywood T., Shen B., Naya L., Gandhi H., et al. (2020a). Human biodistribution and radiation dosimetry of [(18)F]DASA-23, a PET probe targeting pyruvate kinase M2. Eur. J. Nucl. Med. Mol. Imaging 47, 2123–2130. doi:10.1007/s00259-020-04687-0
Beinat C., Patel C. B., Xie Y., Gambhir S. S. (2020b). Evaluation of glycolytic response to multiple classes of anti-glioblastoma drugs by noninvasive measurement of pyruvate kinase M2 using [(18)F]DASA-23. Mol. Imaging Biol. 22, 124–133. doi:10.1007/s11307-019-01353-2
Bhoil A., Singh B., Singh N., Kashyap R., Watts A., Sarika S., et al. (2014). Can 3'-deoxy-3'-(18)F-fluorothymidine or 2'-deoxy-2'-(18)F-fluoro-d-glucose PET/CT better assess response after 3-weeks treatment by epidermal growth factor receptor kinase inhibitor, in non-small lung cancer patients? Preliminary results. Hell. J. Nucl. Med. 17, 90–96. doi:10.1967/s002449910136
Bi W. L., Hosny A., Schabath M. B., Giger M. L., Birkbak N. J., Mehrtash A., et al. (2019). Artificial intelligence in cancer imaging: Clinical challenges and applications. CA Cancer J. Clin. 69, 127–157. doi:10.3322/caac.21552
Boers-Sonderen M. J., De Geus-Oei L. F., Desar I. M., Van Der Graaf W. T., Oyen W. J., Ottevanger P. B., et al. (2014). Temsirolimus and pegylated liposomal doxorubicin (PLD) combination therapy in breast, endometrial, and ovarian cancer: Phase ib results and prediction of clinical outcome with FDG-PET/CT. Target Oncol. 9, 339–347. doi:10.1007/s11523-014-0309-x
Broadfield L. A., Duarte J. a. G., Schmieder R., Broekaert D., Veys K., Planque M., et al. (2021). Fat induces glucose metabolism in nontransformed liver cells and promotes liver tumorigenesis. Cancer Res. 81, 1988–2001. doi:10.1158/0008-5472.CAN-20-1954
Calandriello L., Larici A. R., Leccisotti L., Del Ciello A., Sica G., Infante A., et al. (2018). Multifunctional assessment of non-small cell lung cancer: Perfusion-metabolic correlation. Clin. Nucl. Med. 43, e18–e24. doi:10.1097/RLU.0000000000001888
Carpenter D. J., Jacobs C. D., Wong T. Z., Craciunescu O., Chino J. P. (2019). Changes on midchemoradiation therapy fluorodeoxyglucose positron emission tomography for cervical cancer are associated with prognosis. Int. J. Radiat. Oncol. Biol. Phys. 105, 356–366. doi:10.1016/j.ijrobp.2019.06.2506
Casey R. T., Mclean M. A., Challis B. G., Mcveigh T. P., Warren A. Y., Mendil L., et al. (2020). Fumarate metabolic signature for the detection of reed syndrome in humans. Clin. Cancer Res. 26, 391–396. doi:10.1158/1078-0432.CCR-19-1729
Ceriani L., Milan L., Virili C., Cascione L., Paone G., Trimboli P., et al. (2021). Radiomics analysis of [(18)F]-Fluorodeoxyglucose-Avid thyroid incidentalomas improves risk stratification and selection for clinical assessment. Thyroid 31, 88–95. doi:10.1089/thy.2020.0224
Chen S. H., Miles K., Taylor S. A., Ganeshan B., Rodriquez M., Fraioli F., et al. (2021). FDG-PET/CT in colorectal cancer: Potential for vascular-metabolic imaging to provide markers of prognosis. Eur. J. Nucl. Med. Mol. Imaging 49, 371–384. doi:10.1007/s00259-021-05318-y
Choi M. K., Choi J. Y., Lee J., Heo J. S., Choi S. H., Choi D. W., et al. (2014). Prognostic and predictive value of metabolic tumor volume on (18)F-FDG-PET/CT in advanced biliary tract cancer treated with gemcitabine/oxaliplatin with or without erlotinib. Med. Oncol. 31, 23. doi:10.1007/s12032-014-0023-7
Choi Y. S., Kang S., Ko S. Y., Lee S., Kim J. Y., Lee H., et al. (2018). Hyperpolarized [1-13C] pyruvate MR spectroscopy detect altered glycolysis in the brain of a cognitively impaired mouse model fed high-fat diet. Mol. Brain 11, 74. doi:10.1186/s13041-018-0415-2
Clemmensen A., Hansen A. E., Holst P., Schoier C., Bisgaard S., Johannesen H. H., et al. (2021). [(68)Ga]Ga-NODAGA-E[(cRGDyK)]2 PET and hyperpolarized [1-(13)C] pyruvate MRSI (hyperPET) in canine cancer patients: Simultaneous imaging of angiogenesis and the Warburg effect. Eur. J. Nucl. Med. Mol. Imaging 48, 395–405. doi:10.1007/s00259-020-04881-0
Corbin Z. A. (2019). New metabolic imaging tools in neuro-oncology. Curr. Opin. Neurol. 32, 872–877. doi:10.1097/WCO.0000000000000758
Cossu V., Marini C., Piccioli P., Rocchi A., Bruno S., Orengo A. M., et al. (2019). Obligatory role of endoplasmic reticulum in brain FDG uptake. Eur. J. Nucl. Med. Mol. Imaging 46, 1184–1196. doi:10.1007/s00259-018-4254-2
Dag Z., Yilmaz B., Dogan A. K., Aksan D. U., Ozkurt H., Kizilkaya H. O., et al. (2019). Comparison of the prognostic value of F-18 FDG-PET/CT metabolic parameters of primary tumors and MRI findings in patients with locally advanced cervical cancer treated with concurrent chemoradiotherapy. Brachytherapy 18, 154–162. doi:10.1016/j.brachy.2018.11.005
De Jesus A., Keyhani-Nejad F., Pusec C. M., Goodman L., Geier J. A., Stoolman J. S., et al. (2022). Hexokinase 1 cellular localization regulates the metabolic fate of glucose. Mol. Cell 82, 1261–1277.e9. doi:10.1016/j.molcel.2022.02.028
Deckers E. A., Kruijff S., Brouwers A. H., Van Der Steen K., Hoekstra H. J., Thompson J. F., et al. (2020). The association between active tumor volume, total lesion glycolysis and levels of S-100B and LDH in stage IV melanoma patients. Eur. J. Surg. Oncol. 46, 2147–2153. doi:10.1016/j.ejso.2020.07.011
Dezhakam E., Khalilzadeh B., Mahdipour M., Isildak I., Yousefi H., Ahmadi M., et al. (2022). Electrochemical biosensors in exosome analysis; a short journey to the present and future trends in early-stage evaluation of cancers. Biosens. Bioelectron. 222, 114980. doi:10.1016/j.bios.2022.114980
Dunet V., Halkic N., Sempoux C., Demartines N., Montemurro M., Prior J. O., et al. (2021). Prediction of tumour grade and survival outcome using pre-treatment PET- and MRI-derived imaging features in patients with resectable pancreatic ductal adenocarcinoma. Eur. Radiol. 31, 992–1001. doi:10.1007/s00330-020-07191-z
Edmonds C. E., O'brien S. R., Mankoff D. A., Pantel A. R. (2022). Novel applications of molecular imaging to guide breast cancer therapy. Cancer Imaging 22, 31. doi:10.1186/s40644-022-00468-0
Elimova E., Wang X., Etchebehere E., Shiozaki H., Shimodaira Y., Wadhwa R., et al. (2015). 18-fluorodeoxy-glucose positron emission computed tomography as predictive of response after chemoradiation in oesophageal cancer patients. Eur. J. Cancer 51, 2545–2552. doi:10.1016/j.ejca.2015.07.044
Esfahani S. A., Callahan C., Rotile N. J., Heidari P., Mahmood U., Caravan P. D., et al. (2022). Hyperpolarized [1-(13)C]pyruvate magnetic resonance spectroscopic imaging for evaluation of early response to tyrosine kinase inhibition therapy in gastric cancer. Mol. Imaging Biol. 24, 769–779. doi:10.1007/s11307-022-01727-z
Fantin V. R., St-Pierre J., Leder P. (2006). Attenuation of LDH-A expression uncovers a link between glycolysis, mitochondrial physiology, and tumor maintenance. Cancer Cell 9, 425–434. doi:10.1016/j.ccr.2006.04.023
Ferdinandus J., Zaremba A., Zimmer L., Umutlu L., Seifert R., Barbato F., et al. (2022). Metabolic imaging with FDG-PET and time to progression in patients discontinuing immune-checkpoint inhibition for metastatic melanoma. Cancer Imaging 22, 11. doi:10.1186/s40644-022-00449-3
Ferrara R., Roz L. (2022). Eating away T cell responses in lung cancer. J. Exp. Med. 219, e20221449. doi:10.1084/jem.20221449
Fiore M., Taralli S., Trecca P., Scolozzi V., Marinelli L., Triumbari E. K. A., et al. (2020). A bio-imaging signature as a predictor of clinical outcomes in locally advanced pancreatic cancer. Cancers (Basel) 12, 2016. doi:10.3390/cancers12082016
Fowler A. M., Strigel R. M. (2022). Clinical advances in PET-MRI for breast cancer. Lancet Oncol. 23, e32–e43. doi:10.1016/S1470-2045(21)00577-5
Gao L., Yang F., Tang D., Xu Z., Tang Y., Yang D., et al. (2023). Mediation of PKM2-dependent glycolytic and non-glycolytic pathways by ENO2 in head and neck cancer development. J. Exp. Clin. Cancer Res. 42, 1. doi:10.1186/s13046-022-02574-0
Gomes A., Glaudemans A., Touw D. J., Van Melle J. P., Willems T. P., Maass A. H., et al. (2017). Diagnostic value of imaging in infective endocarditis: A systematic review. Lancet Infect. Dis. 17, e1–e14. doi:10.1016/S1473-3099(16)30141-4
Goncalves A. C., Richiardone E., Jorge J., Polonia B., Xavier C. P. R., Salaroglio I. C., et al. (2021). Impact of cancer metabolism on therapy resistance - clinical implications. Drug Resist Updat 59, 100797. doi:10.1016/j.drup.2021.100797
Goutsouliak K., Veeraraghavan J., Sethunath V., De Angelis C., Osborne C. K., Rimawi M. F., et al. (2020). Towards personalized treatment for early stage HER2-positive breast cancer. Nat. Rev. Clin. Oncol. 17, 233–250. doi:10.1038/s41571-019-0299-9
Guo D., Tong Y., Jiang X., Meng Y., Jiang H., Du L., et al. (2022). Aerobic glycolysis promotes tumor immune evasion by hexokinase2-mediated phosphorylation of IκBα. Cell Metab. 34, 1312–1324.e6. doi:10.1016/j.cmet.2022.08.002
Hod N., Nalbandyan K., Porges T., Lantsberg S. (2023). Progressive transformation of germinal centers simulating malignant axillary lymphadenopathy in a breast cancer patient on FDG-PET/CT. Clin. Nucl. Med. 48, e89–e91. doi:10.1097/RLU.0000000000004493
Hundshammer C., Braeuer M., Muller C. A., Hansen A. E., Schillmaier M., Duwel S., et al. (2018). Simultaneous characterization of tumor cellularity and the Warburg effect with PET, MRI and hyperpolarized (13)C-MRSI. Theranostics 8, 4765–4780. doi:10.7150/thno.25162
Jan De Beur S. M., Minisola S., Xia W. B., Abrahamsen B., Body J. J., Brandi M. L., et al. (2022). Global guidance for the recognition, diagnosis, and management of tumor-induced osteomalacia. J. Intern Med. doi:10.1111/joim.13593
Jimenez-Bonilla J. F., Quirce R., Martinez-Rodriguez I., De Arcocha-Torres M., Carril J. M., Banzo I. (2016). The role of PET/CT molecular imaging in the diagnosis of recurrence and surveillance of patients treated for non-small cell lung cancer. Diagn. (Basel) 6, 36. doi:10.3390/diagnostics6040036
Kahraman D., Holstein A., Scheffler M., Zander T., Nogova L., Lammertsma A. A., et al. (2012). Tumor lesion glycolysis and tumor lesion proliferation for response prediction and prognostic differentiation in patients with advanced non-small cell lung cancer treated with erlotinib. Clin. Nucl. Med. 37, 1058–1064. doi:10.1097/RLU.0b013e3182639747
Kaira K., Higuchi T., Naruse I., Arisaka Y., Tokue A., Altan B., et al. (2018). Metabolic activity by (18)F-FDG-PET/CT is predictive of early response after nivolumab in previously treated NSCLC. Eur. J. Nucl. Med. Mol. Imaging 45, 56–66. doi:10.1007/s00259-017-3806-1
Kang F., Wang S., Tian F., Zhao M., Zhang M., Wang Z., et al. (2016). Comparing the diagnostic potential of 68Ga-alfatide II and 18F-FDG in differentiating between non-small cell lung cancer and tuberculosis. J. Nucl. Med. 57, 672–677. doi:10.2967/jnumed.115.167924
Karlstaedt A., Barrett M., Hu R., Gammons S. T., Ky B. (2021). Cardio-Oncology: Understanding the intersections between cardiac metabolism and cancer biology. JACC Basic Transl. Sci. 6, 705–718. doi:10.1016/j.jacbts.2021.05.008
Keshari K. R., Sriram R., Koelsch B. L., Van Criekinge M., Wilson D. M., Kurhanewicz J., et al. (2013a). Hyperpolarized 13C-pyruvate magnetic resonance reveals rapid lactate export in metastatic renal cell carcinomas. Cancer Res. 73, 529–538. doi:10.1158/0008-5472.CAN-12-3461
Keshari K. R., Sriram R., Van Criekinge M., Wilson D. M., Wang Z. J., Vigneron D. B., et al. (2013b). Metabolic reprogramming and validation of hyperpolarized 13C lactate as a prostate cancer biomarker using a human prostate tissue slice culture bioreactor. Prostate 73, 1171–1181. doi:10.1002/pros.22665
Kraehenbuehl L., Weng C. H., Eghbali S., Wolchok J. D., Merghoub T. (2022). Enhancing immunotherapy in cancer by targeting emerging immunomodulatory pathways. Nat. Rev. Clin. Oncol. 19, 37–50. doi:10.1038/s41571-021-00552-7
Lee S. J., Kim J. G., Lee S. W., Chae Y. S., Kang B. W., Lee Y. J., et al. (2013). Clinical implications of initial FDG-PET/CT in locally advanced rectal cancer treated with neoadjuvant chemoradiotherapy. Cancer Chemother. Pharmacol. 71, 1201–1207. doi:10.1007/s00280-013-2114-0
Leimgruber A., Hickson K., Lee S. T., Gan H. K., Cher L. M., Sachinidis J. I., et al. (2020). Spatial and quantitative mapping of glycolysis and hypoxia in glioblastoma as a predictor of radiotherapy response and sites of relapse. Eur. J. Nucl. Med. Mol. Imaging 47, 1476–1485. doi:10.1007/s00259-020-04706-0
Lewis S. M., Asselin-Labat M. L., Nguyen Q., Berthelet J., Tan X., Wimmer V. C., et al. (2021). Spatial omics and multiplexed imaging to explore cancer biology. Nat. Methods 18, 997–1012. doi:10.1038/s41592-021-01203-6
Liang C., Shi S., Qin Y., Meng Q., Hua J., Hu Q., et al. (2020). Localisation of PGK1 determines metabolic phenotype to balance metastasis and proliferation in patients with SMAD4-negative pancreatic cancer. Gut 69, 888–900. doi:10.1136/gutjnl-2018-317163
Lim Y., Bang J. I., Han S. W., Paeng J. C., Lee K. H., Kim J. H., et al. (2017). Total lesion glycolysis (TLG) as an imaging biomarker in metastatic colorectal cancer patients treated with regorafenib. Eur. J. Nucl. Med. Mol. Imaging 44, 757–764. doi:10.1007/s00259-016-3577-0
Lin J., Liu G., Chen L., Kwok H. F., Lin Y. (2022). Targeting lactate-related cell cycle activities for cancer therapy. Semin. Cancer Biol. 86, 1231–1243. doi:10.1016/j.semcancer.2022.10.009
Liu C. J., Lu M. Y., Liu Y. L., Ko C. L., Ko K. Y., Tzen K. Y., et al. (2017). Risk stratification of pediatric patients with neuroblastoma using volumetric parameters of 18F-FDG and 18F-dopa PET/CT. Clin. Nucl. Med. 42, e142–e148. doi:10.1097/RLU.0000000000001529
Lovinfosse P., Polus M., Van Daele D., Martinive P., Daenen F., Hatt M., et al. (2018). FDG-PET/CT radiomics for predicting the outcome of locally advanced rectal cancer. Eur. J. Nucl. Med. Mol. Imaging 45, 365–375. doi:10.1007/s00259-017-3855-5
Lussey-Lepoutre C., Bellucci A., Burnichon N., Amar L., Buffet A., Drossart T., et al. (2020). Succinate detection using in vivo (1)H-MR spectroscopy identifies germline and somatic SDHx mutations in paragangliomas. Eur. J. Nucl. Med. Mol. Imaging 47, 1510–1517. doi:10.1007/s00259-019-04633-9
Ma Z., Han H., Zhao Y. (2022). Mitochondrial dysfunction-targeted nanosystems for precise tumor therapeutics. Biomaterials 293, 121947. doi:10.1016/j.biomaterials.2022.121947
Madhavan S., Nagarajan S. (2020). GRP78 and next generation cancer hallmarks: An underexplored molecular target in cancer chemoprevention research. Biochimie 175, 69–76. doi:10.1016/j.biochi.2020.05.005
Maharjan S., Sharma P., Patel C. D., Sharma D. N., Dhull V. S., Jain S. K., et al. (2013). Prospective evaluation of qualitative and quantitative ¹⁸F-FDG PET-CT parameters for predicting survival in recurrent carcinoma of the cervix. Nucl. Med. Commun. 34, 741–748. doi:10.1097/MNM.0b013e3283622f0d
Mathew B., Vijayasekharan K., Shah S., Purandare N. C., Agrawal A., Puranik A., et al. (2020). Prognostic value of 18F-FDG-PET/CT-Metabolic parameters at baseline and interim assessment in pediatric anaplastic large cell lymphoma. Clin. Nucl. Med. 45, 182–186. doi:10.1097/RLU.0000000000002927
Mayerhoefer M. E., Riedl C. C., Kumar A., Gibbs P., Weber M., Tal I., et al. (2019). Radiomic features of glucose metabolism enable prediction of outcome in mantle cell lymphoma. Eur. J. Nucl. Med. Mol. Imaging 46, 2760–2769. doi:10.1007/s00259-019-04420-6
Mitchell K. G., Amini B., Wang Y., Carter B. W., Godoy M. C. B., Parra E. R., et al. (2020). (18 F-fluorodeoxyglucose positron emission tomography correlates with tumor immunometabolic phenotypes in resected lung cancer. Cancer Immunol. Immunother. 69, 1519–1534. doi:10.1007/s00262-020-02560-5
Momcilovic M., Bailey S. T., Lee J. T., Fishbein M. C., Braas D., Go J., et al. (2018a). The GSK3 signaling Axis regulates adaptive glutamine metabolism in lung squamous cell carcinoma. Cancer Cell 33, 905–921. doi:10.1016/j.ccell.2018.04.002
Momcilovic M., Bailey S. T., Lee J. T., Zamilpa C., Jones A., Abdelhady G., et al. (2018b). Utilizing 18F-FDG-PET/CT imaging and quantitative histology to measure dynamic changes in the glucose metabolism in mouse models of lung cancer. J. Vis. Exp., 57167. doi:10.3791/57167
Moscoso A., Silva-Rodriguez J., Aldrey J. M., Cortes J., Fernandez-Ferreiro A., Gomez-Lado N., et al. (2019). Staging the cognitive continuum in prodromal Alzheimer's disease with episodic memory. Neurobiol. Aging 84, 1–8. doi:10.1016/j.neurobiolaging.2019.07.014
Nakamoto R., Zaba L. C., Liang T., Reddy S. A., Davidzon G., Aparici C. M., et al. (2021). Prognostic value of bone marrow metabolism on pretreatment (18)F-FDG-PET/CT in patients with metastatic melanoma treated with anti-PD-1 therapy. J. Nucl. Med. 62, 1380–1383. doi:10.2967/jnumed.120.254482
Norton L., Shannon C., Gastaldelli A., Defronzo R. A. (2022). Insulin: The master regulator of glucose metabolism. Metabolism 129, 155142. doi:10.1016/j.metabol.2022.155142
Oh S., Youn H., Paeng J. C., Kim Y. H., Lee C. H., Choi H., et al. (2022). Glucose-thymidine ratio as a metabolism index using (18)F-FDG and (18)F-FLT PET uptake as a potential imaging biomarker for evaluating immune checkpoint inhibitor therapy. Int. J. Mol. Sci. 23, 9273. doi:10.3390/ijms23169273
Ouyang X., Han S. N., Zhang J. Y., Dioletis E., Nemeth B. T., Pacher P., et al. (2018). Digoxin suppresses pyruvate kinase M2-promoted HIF-1α transactivation in steatohepatitis. Cell Metab. 27, 1156. doi:10.1016/j.cmet.2018.04.007
Pan Q., Cao X., Luo Y., Li J., Feng J., Li F. (2020). Chemokine receptor-4 targeted PET/CT with (68)Ga-pentixafor in assessment of newly diagnosed multiple myeloma: Comparison to (18)F-FDG-PET/CT. Eur. J. Nucl. Med. Mol. Imaging 47, 537–546. doi:10.1007/s00259-019-04605-z
Park S., Ha S., Kwon H. W., Kim W. H., Kim T. Y., Oh D. Y., et al. (2017). Prospective evaluation of changes in tumor size and tumor metabolism in patients with advanced gastric cancer undergoing chemotherapy: Association and clinical implication. J. Nucl. Med. 58, 899–904. doi:10.2967/jnumed.116.182675
Qi Q., Fox M. S., Lim H., Sullivan R., Li A., Bellyou M., et al. (2022). Glucose infusion induced change in intracellular pH and its relationship with tumor glycolysis in a C6 rat model of glioblastoma. Mol. Imaging Biol. doi:10.1007/s11307-022-01726-0
Rangel Rivera G. O., Knochelmann H. M., Dwyer C. J., Smith A. S., Wyatt M. M., Rivera-Reyes A. M., et al. (2021). Fundamentals of T Cell metabolism and strategies to enhance cancer immunotherapy. Front. Immunol. 12, 645242. doi:10.3389/fimmu.2021.645242
Raskov H., Gaggar S., Tajik A., Orhan A., Gogenur I. (2022). Metabolic switch in cancer - survival of the fittest. Eur. J. Cancer 180, 30–51. doi:10.1016/j.ejca.2022.11.025
Reynolds J. C., Maass-Moreno R., Thomas A., Ling A., Padiernos E. B., Steinberg S. M., et al. (2020). (18 F-FDG-PET assessment of malignant pleural mesothelioma: Total lesion volume and total lesion glycolysis-the central role of volume. J. Nucl. Med. 61, 1570–1575. doi:10.2967/jnumed.119.238733
Rodriguez-Nieto G., Levin O., Hermans L., Weerasekera A., Sava A. C., Haghebaert A., et al. (2023). Organization of neurochemical interactions in young and older brains as revealed with a network approach: Evidence from proton magnetic resonance spectroscopy ((1)H-MRS). Neuroimage 266, 119830. doi:10.1016/j.neuroimage.2022.119830
Rowe S. P., Pomper M. G. (2022). Molecular imaging in oncology: Current impact and future directions. CA Cancer J. Clin. 72, 333–352. doi:10.3322/caac.21713
Sachpekidis C., Larribere L., Kopp-Schneider A., Hassel J. C., Dimitrakopoulou-Strauss A. (2019). Can benign lymphoid tissue changes in (18)F-FDG-PET/CT predict response to immunotherapy in metastatic melanoma? Cancer Immunol. Immunother. 68, 297–303. doi:10.1007/s00262-018-2279-9
Saidi D., Cheray M., Osman A. M., Stratoulias V., Lindberg O. R., Shen X., et al. (2018). Glioma-induced SIRT1-dependent activation of hMOF histone H4 lysine 16 acetyltransferase in microglia promotes a tumor supporting phenotype. Oncoimmunology 7, e1382790. doi:10.1080/2162402X.2017.1382790
Salavati A., Duan F., Snyder B. S., Wei B., Houshmand S., Khiewvan B., et al. (2017). Optimal FDG-PET/CT volumetric parameters for risk stratification in patients with locally advanced non-small cell lung cancer: Results from the ACRIN 6668/RTOG 0235 trial. Eur. J. Nucl. Med. Mol. Imaging 44, 1969–1983. doi:10.1007/s00259-017-3753-x
Schwenck J., Schorg B., Fiz F., Sonanini D., Forschner A., Eigentler T., et al. (2020). Cancer immunotherapy is accompanied by distinct metabolic patterns in primary and secondary lymphoid organs observed by non-invasive in vivo(18)F-FDG-PET. Theranostics 10, 925–937. doi:10.7150/thno.35989
Scroggins B. T., Matsuo M., White A. O., Saito K., Munasinghe J. P., Sourbier C., et al. (2018). Hyperpolarized [1-(13)C]-Pyruvate magnetic resonance spectroscopic imaging of prostate cancer in vivo predicts efficacy of targeting the Warburg effect. Clin. Cancer Res. 24, 3137–3148. doi:10.1158/1078-0432.CCR-17-1957
Sieow J. L., Penny H. L., Gun S. Y., Tan L. Q., Duan K., Yeong J. P. S., et al. (2023). Conditional knockout of hypoxia-inducible factor 1-alpha in tumor-infiltrating neutrophils protects against pancreatic ductal adenocarcinoma. Int. J. Mol. Sci. 24, 753. doi:10.3390/ijms24010753
Soussan M., Cyrta J., Pouliquen C., Chouahnia K., Orlhac F., Martinod E., et al. (2014). Fluorine 18 fluorodeoxyglucose PET/CT volume-based indices in locally advanced non-small cell lung cancer: Prediction of residual viable tumor after induction chemotherapy. Radiology 272, 875–884. doi:10.1148/radiol.14132191
Stewart C. L., Warner S., Ito K., Raoof M., Wu G. X., Kessler J., et al. (2018). Cytoreduction for colorectal metastases: Liver, lung, peritoneum, lymph nodes, bone, brain. When does it palliate, prolong survival, and potentially cure? Curr. Probl. Surg. 55, 330–379. doi:10.1067/j.cpsurg.2018.08.004
Tarumi R., Tsugawa S., Noda Y., Plitman E., Honda S., Matsushita K., et al. (2020). Levels of glutamatergic neurometabolites in patients with severe treatment-resistant schizophrenia: A proton magnetic resonance spectroscopy study. Neuropsychopharmacology 45, 632–640. doi:10.1038/s41386-019-0589-z
Tateishi U., Tatsumi M., Terauchi T., Ando K., Niitsu N., Kim W. S., et al. (2015). Prognostic significance of metabolic tumor burden by positron emission tomography/computed tomography in patients with relapsed/refractory diffuse large B-cell lymphoma. Cancer Sci. 106, 186–193. doi:10.1111/cas.12588
Tian M., He X., Jin C., He X., Wu S., Zhou R., et al. (2021). Transpathology: Molecular imaging-based pathology. Eur. J. Nucl. Med. Mol. Imaging 48, 2338–2350. doi:10.1007/s00259-021-05234-1
Usmanij E. A., De Geus-Oei L. F., Troost E. G., Peters-Bax L., Van Der Heijden E. H., Kaanders J. H., et al. (2013). 18F-FDG-PET early response evaluation of locally advanced non-small cell lung cancer treated with concomitant chemoradiotherapy. J. Nucl. Med. 54, 1528–1534. doi:10.2967/jnumed.112.116921
Vaarwerk B., Breunis W. B., Haveman L. M., De Keizer B., Jehanno N., Borgwardt L., et al. (2021). Fluorine-18-fluorodeoxyglucose (FDG) positron emission tomography (PET) computed tomography (CT) for the detection of bone, lung, and lymph node metastases in rhabdomyosarcoma. Cochrane Database Syst. Rev. 11, CD012325. doi:10.1002/14651858.CD012325.pub2
Van Berkel A., Vriens D., Visser E. P., Janssen M. J. R., Gotthardt M., Hermus A., et al. (2019). Metabolic subtyping of pheochromocytoma and paraganglioma by (18)F-FDG pharmacokinetics using dynamic PET/CT scanning. J. Nucl. Med. 60, 745–751. doi:10.2967/jnumed.118.216796
Vernieri C., Fuca G., Ligorio F., Huber V., Vingiani A., Iannelli F., et al. (2022). Fasting-mimicking diet is safe and reshapes metabolism and antitumor immunity in patients with cancer. Cancer Discov. 12, 90–107. doi:10.1158/2159-8290.CD-21-0030
Vos J. L., Zuur C. L., Smit L. A., De Boer J. P., Al-Mamgani A., Van Den Brekel M. W. M., et al. (2022). [(18)F]FDG-PET accurately identifies pathological response early upon neoadjuvant immune checkpoint blockade in head and neck squamous cell carcinoma. Eur. J. Nucl. Med. Mol. Imaging 49, 2010–2022. doi:10.1007/s00259-021-05610-x
Wang C., Zhang X., Yang X., Li H., Cui R., Guan W., et al. (2018). PET response assessment in apatinib-treated radioactive iodine-refractory thyroid cancer. Endocr. Relat. Cancer 25, 653–663. doi:10.1530/ERC-18-0007
Wang L., Ruan M., Lei B., Yan H., Sun X., Chang C., et al. (2020a). The potential of (18)F-FDG-PET/CT in predicting PDL1 expression status in pulmonary lesions of untreated stage IIIB-IV non-small-cell lung cancer. Lung Cancer 150, 44–52. doi:10.1016/j.lungcan.2020.10.004
Wang Y., Zhao N., Wu Z., Pan N., Shen X., Liu T., et al. (2020b). New insight on the correlation of metabolic status on (18)F-FDG-PET/CT with immune marker expression in patients with non-small cell lung cancer. Eur. J. Nucl. Med. Mol. Imaging 47, 1127–1136. doi:10.1007/s00259-019-04500-7
Wilson D. M., Kurhanewicz J. (2014). Hyperpolarized 13C MR for molecular imaging of prostate cancer. J. Nucl. Med. 55, 1567–1572. doi:10.2967/jnumed.114.141705
Witney T. H., James M. L., Shen B., Chang E., Pohling C., Arksey N., et al. (2015). PET imaging of tumor glycolysis downstream of hexokinase through noninvasive measurement of pyruvate kinase M2. Sci. Transl. Med. 7, 310ra169. doi:10.1126/scitranslmed.aac6117
Wong K. H., Panek R., Welsh L., Mcquaid D., Dunlop A., Riddell A., et al. (2016). The predictive value of early assessment after 1 cycle of induction chemotherapy with 18F-FDG-PET/CT and diffusion-weighted MRI for response to radical chemoradiotherapy in head and neck squamous cell carcinoma. J. Nucl. Med. 57, 1843–1850. doi:10.2967/jnumed.116.174433
Xia L., Oyang L., Lin J., Tan S., Han Y., Wu N., et al. (2021). The cancer metabolic reprogramming and immune response. Mol. Cancer 20, 28. doi:10.1186/s12943-021-01316-8
Xie M., Zhai W., Cheng S., Zhang H., Xie Y., He W. (2016). Predictive value of F-18 FDG-PET/CT quantization parameters for progression-free survival in patients with diffuse large B-cell lymphoma. Hematology 21, 99–105. doi:10.1179/1607845415Y.0000000033
Yamamoto K., Brender J. R., Seki T., Kishimoto S., Oshima N., Choudhuri R., et al. (2020). Molecular imaging of the tumor microenvironment reveals the relationship between tumor oxygenation, glucose uptake, and glycolysis in pancreatic ductal adenocarcinoma. Cancer Res. 80, 2087–2093. doi:10.1158/0008-5472.CAN-19-0928
Yang M., Mahanty A., Jin C., Wong A. N. N., Yoo J. S. (2022). Label-free metabolic imaging for sensitive and robust monitoring of anti-CD47 immunotherapy response in triple-negative breast cancer. J. Immunother. Cancer 10, e005199. doi:10.1136/jitc-2022-005199
Zamagni E., Nanni C., Dozza L., Carlier T., Bailly C., Tacchetti P., et al. (2021). Standardization of (18)F-FDG-PET/CT according to deauville criteria for metabolic complete response definition in newly diagnosed multiple myeloma. J. Clin. Oncol. 39, 116–125. doi:10.1200/JCO.20.00386
Zhao G., Yuan H., Li Q., Zhang J., Guo Y., Feng T., et al. (2022). DDX39B drives colorectal cancer progression by promoting the stability and nuclear translocation of PKM2. Signal Transduct. Target Ther. 7, 275. doi:10.1038/s41392-022-01096-7
Zhao Y., Liu C., Zhang Y., Gong C., Li Y., Xie Y., et al. (2018). Prognostic value of tumor heterogeneity on 18F-FDG-PET/CT in HR+HER2- metastatic breast cancer patients receiving 500 mg fulvestrant: A retrospective study. Sci. Rep. 8, 14458. doi:10.1038/s41598-018-32745-z
Keywords: molecular imaging, tumor metabolism, PET, biological characteristic, aerobic glycolysis
Citation: Meng Y, Sun J, Zhang G, Yu T and Piao H (2023) Imaging glucose metabolism to reveal tumor progression. Front. Physiol. 14:1103354. doi: 10.3389/fphys.2023.1103354
Received: 20 November 2022; Accepted: 20 January 2023;
Published: 02 February 2023.
Edited by:
Zhen Cheng, Shanghai Institute of Materia Medica (CAS), ChinaReviewed by:
Xilin Sun, The Fourth Hospital of Harbin Medical University, ChinaCopyright © 2023 Meng, Sun, Zhang, Yu and Piao. This is an open-access article distributed under the terms of the Creative Commons Attribution License (CC BY). The use, distribution or reproduction in other forums is permitted, provided the original author(s) and the copyright owner(s) are credited and that the original publication in this journal is cited, in accordance with accepted academic practice. No use, distribution or reproduction is permitted which does not comply with these terms.
*Correspondence: Tao Yu, dGFveXVsbnN6bHl5QDE2My5jb20=; Haozhe Piao, aGFvemhlcGlhb2xuemx5eUBzaW5hLmNvbQ==