- 1School of Life Science, Huaiyin Normal University, Huai’an, China
- 2Key Laboratory of Freshwater Aquatic Genetic Resources Ministry of Agriculture, Shanghai Ocean University, Shanghai, China
Metamifop (MET) is a widely used herbicide. It is likely for it to enter water environment when utilized, thus potential impacts may be produced on aquatic animals. Little information is available about its effects on the endocrine system of fish to date. In the current study, female rice field eels (Monopterus albus) were exposed to different MET concentrations (0, 0.2, 0.4, 0.6, 0.8 mg L −1) for 96 h to examine the effect of MET on the hypothalamic-pituitary-gonadal (HPG) axis and sexual reversal. The results showed that high concentrations of MET exposure increased vitellogenin (VTG) levels in liver and plasma, but plasma sex hormone levels were not affected by MET exposure. MET exposure increased the expression of CYP19A1b and CYP17 that regulate sex hormone production in the brain, but the expression of genes (CYP19A1a, CYP17, FSHR, LHCGR, hsd11b2, 3β-HSD) associated with sex hormone secretion in the ovary and the estrogen receptor genes (esr1, esr2a, esr2b) in the liver were all suppressed. In addition, the expression of sex-related gene (Dmrt1) was suppressed. This study revealed for the first time that MET has estrogen-like effects and has a strong interference with the expression of HPG axis genes. MET did not show the ability to promote the sexual reversal in M. albus, on the contrary, the genes expression showed that the occurrence of male pathway was inhibited.
Introduction
Endocrine-disrupting chemicals (EDCs) can interfere with various aspects of biological endocrine secretion through intake, accumulation and other ways. Exposure to EDCs are thought to have an irreversible effect on animals during critically susceptible phases of sexual differentiation. Synthetic chemicals particularly used in agriculture are thought to cause endocrine disturbances in wildlife, and herbicides are among the most commonly used agricultural chemicals (Mnif et al., 2011; Combarnous, 2017). For a long time, herbicides have been widely concerned because of their behavioral toxicity (Yalsuyi et al., 2021), neurotoxicity (Merola et al., 2022), reproductive toxicity (Soni and Verma, 2020) and many other effects on aquatic organisms.
Aryloxyphenoxypropionate (AOPP) herbicides have been widely used in paddy fields due to their excellent weed control ability. Metamifop (MET) ((R)-2-[4-(6-chloro-1,3-benzoxazol-2-yloxy) phenoxy]-2′-fluoro-N-methyl propionanilide), an AOPP herbicide developed by Dongbu Hannong Chemical Co., Ltd (South Korea), can effectively control annual weeds by inhibiting the activity of acetyl-CoA carboxylase (ACCase), thereby interfering with lipid synthesis (Xia et al., 2016). Due to its high safety to cereal crops, MET has been widely used around the world, including China, Korea, Japan, Philippines (Parker et al., 2015; Cooper et al., 2016). The half-life of MET varies in different environments, for example, 15.83–17.5 days in laboratory water (pH = 7.0) (Saha et al., 2018), 21.5–40.80 days in paddy fields (Wang et al., 2012), and about 70 days in soil (Janaki and Chinnusamy, 2012). Because MET residues persist in water and soil for a longer time, the implications of MET for aquatic organisms should be concerned.
MET was reported to be highly toxic to zebrafish (Danio rerio) embryos (acute 96 h LC50 value of 0.648 mg L−1) and juvenile zebrafish (acute 96 h LC50 value of 0.216 mg L−1) (Zhao et al., 2019). According to the information provided by the International Union of Pure and Applied Chemistry (IUPAC, 2009), MET is moderately toxic to rainbow trout (Oncorhynchus mykiss) (96 h LC50 value of 0.307 mg L−1), invertebrate (Daphnia magna) (48 h EC50 value of 0.288 mg L−1), and algae (72 h EC50 value of 2.03 mg L−1). A study of quizalofop-p-ethyl, also an AOPP herbicide, showed that exposure at sublethal doses increased estrogen axis activity in male zebrafish (Zhu et al., 2017). Recently, Liu et al. (2021) reported that the thyroid hormone levels and related genes expression in tadpoles (Xenopus laevis) were affected by MET, reflecting the endocrine interference effect of MET. To date, the impact of MET on the sex hormone of fish has not been reported, and the underlying mechanism is unclear.
M. albus is a commercially important freshwater species in China and very popular for its delicious taste. Although the prodution of M. albus in China was about 307,200 tons in 2020 (China Fisheries Statistical Yearbook, 2021), the supply still demand exceeds supply. It is difficult to meet the market demand due to the low yield of monoculture. In order to meet the demand and increase the income of farmers, the rice-eel farming model has been gradually promoted in recent years. In this case, use of herbicides in paddy fields is necessary to ensure yield, meanwhile the safety of herbicides for M. albus need to be considered. All M. albus are females prior to maturity and can naturally transform into males through a bisexual stage (Cheng et al., 2021). The low number of male M. albus is an important factor that restricts the artificial reproduction of M. albus. Therefore, normal sex reversal is crucial to the breeding of M. albus. Our preliminary experiment showed that sublethal concentration of MET (0.8 mg L−1) resulted in a significant increase in plasma vitellogenin (VTG) levels in female M. albus after 4 days of exposure. VTG has been shown to be a biomarker for EDCs (Yamamoto et al., 2017; Li et al., 2018; Merola et al., 2021). Therefore, MET may have an effect on endocrine homeostasis in M. albus, acting as an endocrine disruptor to affect sex reversal of M. albus. In the present study, the levels of plasma steroid hormone and the expression of related genes on hypothalamic-pituitary-gonadal (HPG) axis were detected to evaluate influence of MET on the endocrine system of M. albus. The results of this work may help elucidate the mechanism of MET action on the endocrine secretion and sex reversal of M. albus.
Materials and methods
Chemical
Metamifop (CAS No.256412-89-2, purity 99%) was supplied by Hubei JiangMinTaiHua Chemical Co., Ltd. (Hubei, China). MET stock solution of 1,000 mg L−1 was prepared as previously described by Zhao et al. (2019). Acetone containing 1% Tween-80 (v/v) was used to prepare solvent control in all the tests. For other chemicals, Analytical Reagent (AR) grade chemicals were used.
Exposure experiment
Healthy female M. albus with ovary developing at the third stage (weight 10–15 g, length 24–28 cm) were taken from Luoma Lake, Jiangsu Province, China. M. albus were acclimated in polyethylene tanks (5 m × 0.8 m × 0.4 m, water depth 0.3 m) of the Jiangsu Special Aquatic Breeding Engineering Laboratory for 1 week. During the acclimation period, the eels were fed with earthworm (once a day, 19: 30 pm, approx. 1.5 g fish−1 day−1), with a 14 L: 10 D photoperiod. pH 7.8 ± 0.1; dissolved oxygen 9.0 ± 0.5 mg L−1; temperature 20°C ± 2°C; only exist the aerator working sound. Water was changed every other day. Feeding stopped 1 day before the experiment, and no feeding was given during all the experiment period. According to the results of preliminary experiment, the 96 h LC50 of MET to M. albus was determined to be 0.785 mg L−1. Dechlorinated tap water and 0.08% acetone and 0.0008% Tween 80 dissolved in dechlorinated tap water (v/v) were used as blank and solvent control, respectively. The concentration of MET in the experiment was set at 0.2, 0.4, 0.6, 0.8 mg L−1, respectively, and was determined using high phase liquid chromatography (HPLC). During the experiment, 144 M. albus were selected randomly into six groups, each group with three replications. Then the M. albus were placed in culture tanks (40 cm × 30 cm × 15 cm, water depth: 10 cm). The water tanks used in the experiment were covered with gauze to prevent eels from escaping, and the physicochemical parameters of the water were consistent with those in acclimation period. Tiles were placed as shelter. The test solutions were renewed every 24 h in order to maintain MET concentration constant and dead fish were cleared at the same time.
Sample collection
After 96 h, eels were anesthetized with 3-aminobenzoic acid ethyl ester methanesulfonate (MS-222) (Sigma-Aldrich Co., St. Louis, MO, United States of America). The blood samples were collected by severing the caudal peduncle. The blood was immediately mixed with anticoagulant (0.50‰ EDTA) and centrifuge at 1,500 rpm/min for 30 min to obtain plasma. Then the plasma was extracted and stored at 4°C for analysis. After, the M. albus were decapitated on ice and ovary, brain (complex of brain and pituitary) and liver tissues were collected and preserved at −80°C.
Hormone and vitellogenin measurement
Estradiol (E2), testosterone (T) and vitellogenin (VTG) in anticoagulated plasma and liver were detected by enzyme linked immunosorbent assay (ELISA), ELISA kits from Shanghai QiaoDu Biotechnology Co., Ltd. (ShangHai, China) were used following the instructions of the manufacturer.
Gene expression
Total RNA of ovaries, brain and liver samples was extracted using TRIzol reagent (CWBIO, Beijing, China). The purity, concentration and integrity of RNA samples were detected by Nanodrop, Qubit 2.0, Aglient 2,100, respectively. Single-stranded cDNA was synthesized using HiFiScript cDNA Syntheses Kit (ComWin Biotech Co., Ltd, Beijing, China) following the instructions. The transcripts of genes were measured by LightCycler® 480 II RT–qPCR machine (Roche, Switzerland). The primers were designed by Primer Premier 6.0 and are shown in Table 1. The reaction mixtures were 20 μL, containing 10 μL SYBR Premix Ex Taq (2 ×), primers (0.5 μM) 1 μL each, 2 μL cDNA sample, and 6 μL dH2O. Cycling conditions were 95°C for 5 min, followed by 40 cycles of 95°C for 10 s, 60°C for 30 s and 72°C for 30 s. All samples were run in triplicate, and each assay was repeated three times. The threshold cycle (Ct) values were obtained from each sample. Relative gene expression levels were evaluated using 2−△△CT method (Livak and Schmittgen, 2001).
Statistical analysis
Prior to conducting statistical comparisons, the experimental data which were presented as the mean ± standard deviation were assessed for normality and homogeneity of variances using the Shapiro-Wilk test and Levene’s test, respectively. The experimental data were analyzed using unifactor ANOVA, followed by SNK post hoc test. The critical value for statistical significance was p < 0.05. All statistical analyses were conducted using SPSS 26.0 software (SPSS, Chicago, IL).
Results
Solvent effect and analytical quantification of exposure solutions
Statistical analysis showed that solvent had no effect on all indicators in this study. Therefore, the solvent group could be used as a control. HPLE analysis of the water samples indicated that the exposure solutions ranged approximately 93–120% of all nominal concentrations (Table 2). Since all tested solutions were updated daily and the concentration of solutions were measured before and after the replacement, therefore, the nominal dose can represent the actual content in this study.
Effect of metamifop on sex hormones
In the present study, no significant effect on measured plasma E2 and T levels were observed at all concentrations of metamifop (p > 0.05). However, compared with the control group, E2 and T showed a non-significant decrease at 0.2 mg L−1 and 0.4 mg L−1 of MET and a non-significant increase at 0.6 mg L−1 and 0.8 mg L−1 of MET (Figures 1A,B). In addition, the ratio of estrogen to androgen (E2/T) remained unchanged after exposure to MET (Figure 1C).
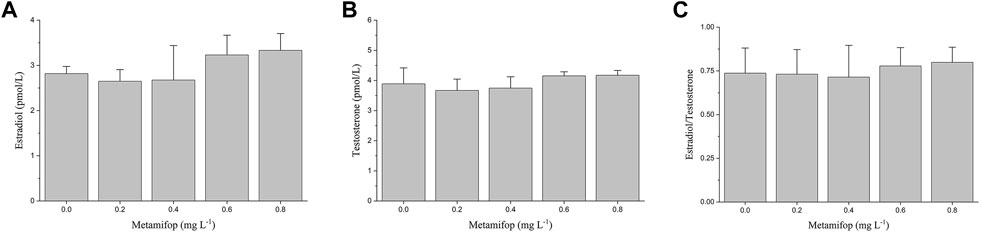
FIGURE 1. Plasma E2 (A), T (B) levels and E2/T ratio (C) in female Monopterus albus exposed to 0, 0.2, 0.4, 0.6, 0.8 mg L−1 metamifop for 96 h. Results are presented as mean ± SD of three replicate samples (n = 8). *p < 0.05 and **p < 0.01 indicate significant differences between exposure and control group.
Effect of metamifop on vitellogenin
The VTG content in plasma of female M. albus was significantly elevated (p < 0.05) in the 0.8 mg L−1 treatment compared with the control (Figure 2A). In liver, VTG levels were significantly increased (p < 0.05) in both 0.6 and 0.8 mg L−1 MET-treated groups than the control group (Figure 2B). However, compared with the control group, at lower MET concentrations (0.2 mg L−1 and 0.4 mg L−1), VTG levels in both plasma and liver did not change significantly.
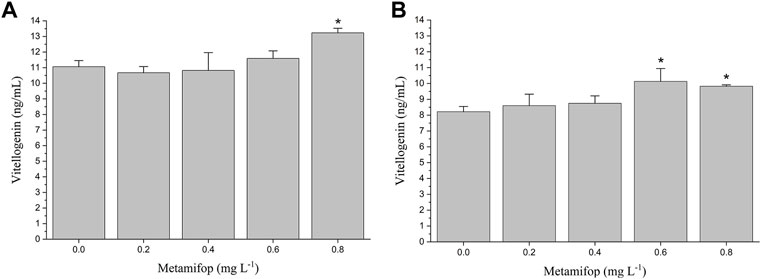
FIGURE 2. Plasma vitellogenin (VTG) (A) and liver VTG (B) levels in female Monopterus albus exposed to 0, 0.2, 0.4, 0.6 and 0.8 mg L−1 metamifop for 96 h. Results are presented as mean ± SD of three replicate samples (n = 8). *p < 0.05 and **p < 0.01 indicate significant differences between exposure and control group.
Effect of metamifop on the expression of endocrine-related genes
Endocrine-related gene expression in the brain
In brain, CYP19A1b was upregulated in MET exposure groups compared with the control (by 1.6- to 2.5-folds) (Figure 3A). CYP17 expression was increased by about 2- to 2.5- folds in all exposure groups (Figure 3A). FSHβ was downregulated in all exposure groups (by 2.5- to 5-folds) (Figure 3A). The expression of LHβ was increased by 5.5-fold, 9.0-fold, 14.0-fold and 23.0-fold after exposure to 0.2, 0.4, 0.6, and 0.8 mg L−1 MET, respectively (Figure 3B).
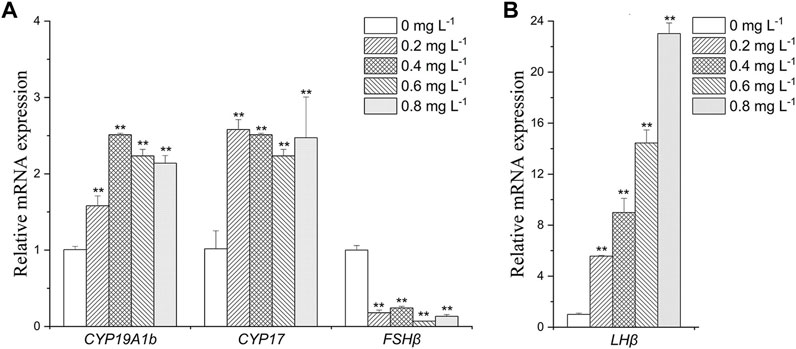
FIGURE 3. Expression profiles of genes (A): CYP19A1b, CYP17, FSHβ; (B) LHβ) in Monopterus albus brain exposed to 0, 0.2, 0.4, 0.6 and 0.8 mg L−1 metamifop for 96 h. Results are presented as mean ± SD of three replicate samples (n = 8). *p < 0.05 and **p < 0.01 indicate significant differences between exposure and control group.
Endocrine-related gene expression in the ovary
As shown in Figure 4. The expressive levels of CYP19A1a and CYP17 were significantly decreased in all the MET exposed groups compared with the control (p < 0.05). Similarly, FSHR and LHCGR mRNA levels were significantly decreased at all MET concentrations compared to the control (all about 5-fold). At low MET concentration (0.2 mg L−1), both the expressive of HSD11b2 and 3β-HSD did not occur significant change. However, after the increase of MET concentration, the expressive levels of HSD11b2 and 3β-HSD were decreased. For example, the expressive level of HSD11b2 was significantly decreased when exposure to 0.4, 0.6, and 0.8 mg L−1 MET (3.3-fold, 2-fold and 3.3-fold, respectively). Transcription level of 3β-HSD was downregulated by 2-fold in the 0.4 mg/L group (p < 0.05), 0.6-fold in the 0.6 mg L−1 group (p < 0.05) and 0.7-fold in the 0.8 mg L−1 group (p < 0.05).
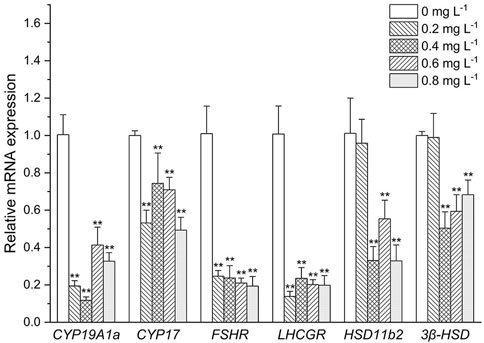
FIGURE 4. Expression profiles of genes associated with the HPG axis in Monopterus albus ovary exposed to 0, 0.2, 0.4, 0.6, and 0.8 mg L−1 metamifop for 96 h. Results are presented as mean ± SD of three replicate samples (n = 8). *p < 0.05 and **p < 0.01 indicate significant differences between exposure and control group.
Endocrine-related gene expression in the liver
As shown in Figure 5, in liver, esr1, esr2a, and esr2b were downregulated in all exposure groups (by 1.3- to 5-folds, 1.1- to 5-folds and 2- to 10-folds, respectively). While under the influence of high concentration of MET (0.8 mg L−1), the expression of the receptor genes esr1, esr2a, and esr2b was recovered compared to other treatment groups.
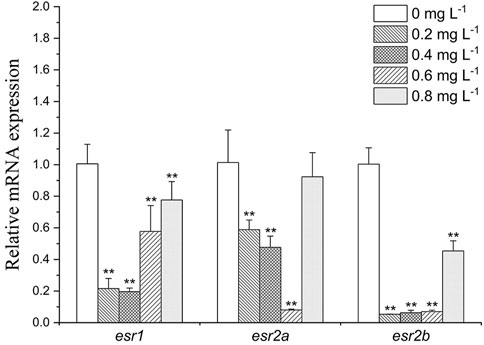
FIGURE 5. Estrogen receptor gene expression profiles in Monopterus albus liver exposed to 0, 0.2, 0.4, 0.6, and 0.8 mg L−1 metamifop for 96 h. Results are presented as mean ± SD of three replicate samples (n = 8). *p < 0.05 and **p < 0.01 indicate significant differences between exposure and control group.
Effect of metamifop on the expression of sex-related genes
As shown in Figure 6, Dmrt1 expression decreased by about 0.7-fold in the 0.2 and 0.4 mg L−1 group (p < 0.05), and 0.6-fold in the 0.6 mg L−1 group (p < 0.05). foxl3 was downregulated in all exposure groups (by about 2.5- to 3.3- folds). Expression level of JNK1 was slightly increased after exposure to MET (by about 1.2- to 1.3- folds).
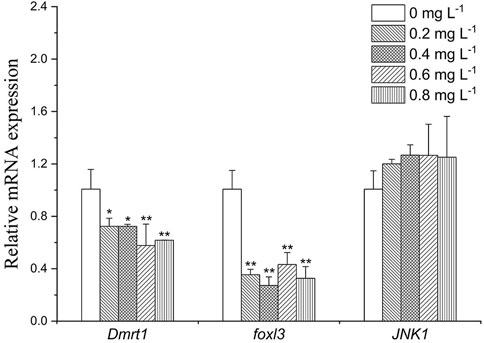
FIGURE 6. Sex-related gene expression profiles in Monopterus albus ovary exposed to 0, 0.2, 0.4, 0.6, and 0.8 mg L−1 metamifop for 96 h. Results are presented as mean ± SD of three replicate samples (n = 8). *p < 0.05 and **p < 0.01 indicate significant differences between exposure and control group.
Discussion
Vitellogenin (VTG), the precursor of yolk proteins, is produced after estrogen combine with specific Estrogen receptors (ESRs) in the liver, while VTG is absent or present at very low concentration in male and juvenile fish (Tyler et al., 1996). It has been found that some endocrine disruptors have estrogen-like effect and can induce the synthesis of VTG (Nikoleris et al., 2016). Therefore, VTG is considered as a biomarker of the presence of environmental estrogen-like compounds. In the present study, the upregulation of VTG concentrations in liver and plasma of female M. albus was observed at high concentration of MET exposure (Figure 2), while no significant change of E2 was found at all MET concentrations. This indicates that MET may have a potential estrogenic effects, mimicking the effect of endogenous estrogen and directly acting on liver estrogen receptors and stimulating VTG synthesis.
Sex steroids exist naturally in vertebrates, it can affect the sex differentiation and reproduction of fish by regulating various parts of the HPG axis (Li et al., 2013). At present, it is found that some exogenous molecules can affect sex steroids by acting on key steroidogenic enzymes or eliminating endogenous hormones (Ankley et al., 2005; Cheshenko et al., 2008). Therefore, the measurement of sex steroid hormones is often suggested as one of the most integrative and functional endpoints to understand the effects of external chemical on sexual development in fish (Ma et al., 2012; Jo et al., 2014). Unlike previous endocrine disruptors that affect the secretion of sex steroids, in the present study, plasma E2 and T levels of M. albus showed no significant change under the influence of MET (Figures 1A,B). This indicates that the interference effect of MET on M. albus is direct, not through affecting endogenous sex hormones, and then regulating the genes expression on HPG axis through the changing sex hormone levels. Since the ratio of E2/T is thought to regulate the direction of sexual differentiation in fish (Rougeot et al., 2007; de Carvalho et al., 2017), the difference in E2/T ratio may determine whether the gonad develops into ovary or testis. Previous studies also showed that sex reversal in M. albus was accompanied by a significant increase in androgens (Chan and Phillips, 1969). However, under the influence of MET, the sex hormone level of M. albus did not change significantly. This indicates that the sexual reversal of M. albus may not be affected by exposure to MET because of the consistency in sex hormone.
However, sex steroid synthesis and homeostasis depend on regulation of the HPG axis (Nagahama, 1994), which involves the actions of several genes. As the last enzyme in the steroidogenic pathway, cytochrome P450 aromatase (CYP19s) is in charge of turning androgens into estrogens, which has a direct impact on the E2/T ratio. There are two genes in teleost, CYP19A1a and CYP19A1b, which encode two proteins with different structures but the same catalytic activities (Kazeto et al., 2004). The CYP19A1a is predominantly expressed in ovary, and CYP19A1b predominantly expressed in brain. As CYP19A1b has estrogen response elements (EREs) (Callard et al., 2001), chemicals with estrogenic effects may induce structural changes of receptor proteins by binding to estrogen receptors. This results in dimerization and binding with EREs sequence, inducing CYP19A1b expression (Zhu et al., 2017). However, EREs are not present in all teleosts’ CYP19A1a promoter sequences (Piferrer and Blazquez, 2005), which result in the response of CYP19A1a expression to estrogen or estrogenic EDCs that vary in different fish (Li et al., 2013). In the present study, the mRNA expression of CYP19A1b was significantly upregulated, but the mRNA expression of CYP19A1a was significantly downregulated. This indicates that MET may be used as a ligand to simulate the direct binding of endogenous estrogen to estrogen receptor and estrogen receptor on CYP19a1b to induce the expression of CYP19a1b. Faria et al. (2021) also found that fenitrothion, a pesticide, could increase the expression of CYP19a1b in zebrafish larvae by acting on sex receptors. CYP17, an enzyme that synthesizes androgens in vertebrates (Govoroun et al., 2001; Chen et al., 2010), is found to be expressed primarily in brain and gonad (Chen et al., 2010). In this study, the CYP17 expression showed synchrony with that of CYP19, and was increased in brain and decreased in ovary under the influence of MET. The contrary respect at down-regulations of CYP19A1b and CYP17 in brain and transcriptional upregulation of CYP19A1a and CYP17 in ovary may explain, the secretion of sex hormones in the brain and ovary may have different response mechanisms after Met stress, which may be the reason why the concentrations of E2 and T in female plasma remained constant. In addition, the difference in the expression in ovary and brain suggests that MET have different interference mechanisms in different tissues.
In fish, the gonadotropins follicle stimulating hormone (FSH) and luteinizing hormone (LH) are secreted by the pituitary and act by binding to specific receptor (FSHR, LHCGR) in gonad, which are the primary mediators of signals to induce steroidogenesis and gametogenesis (Clelland and Peng, 2009). FSH and LH are heterodimeric glycoproteins consisting of a common α-subunit and unique β-subunits, FSHβ and LHβ, respectively (Pierce and Parsons, 1981). The effects of exogenous hormones on FSH and LH mainly act on the mRNA levels of the corresponding β subunits (Li et al., 2013). There exist both positive and negative feedback of steroids for regulation of gonadotropin in fish. Positive feedback of sex steroid is mainly through direct interaction of the ESRs complex with an estrogen regulatory element on FSHβ and LHβ genes. Negative feedback of sex steroid on gonadotropin mainly includes two: one is reducing the content of gonadotropin releasing hormone (GnRH) receptor on the pituitary to reduce the regulation from GnRH (Habibi et al., 1989), the other is to act directly on GnRH neurons in hypothalamus or other neural systems known to affect GnRH system, regulating the release of more or less GnRH from the hypothalamus, to Regulates the release of gonadotropins from the pituitary gland (Montero et al., 1995). In our case, after expose to MET, FSHβ expression was suppressed, while LHβ expression was activated. The results were similar to the effect of E2 on the expression of FSHβ and LHβ in the pituitary cells of M. albus cultured in vitro (Zhang et al., 2014). This indicates that the effect of estrogen on the expression of FSHβ may be mainly through GnRH system, while the effect on the expression of LHβ is direct. The differential expression of FSHβ and LHβ in M. albus indicates that the MET may has the same biological effects as E2. In addition, it has been shown that gonadotropin signaling may be involved in the sex changes in a variety of teleost fish, such as Trimma okinawae (Kobayashi et al., 2009) and Epinephelus merra (Kobayashi et al., 2010). Zhang et al. (2010) also found that the ratio of FSHβ/LHβ was important for the gonadal differentiation of M. albus. However, it is unclear whether the decline in the ratios of FSHβ/LHβ after MET exposure is related to sexual reversal of M. albus, which needs further research. FSH and LH eventually act on gonads through blood circulation to promote the release of gonadal hormones. As gonadotropin receptors, the expression of FSHR and LHCGR should be closely related to the changes of FSH and LH. In this study, the expression levels of FSHR and FSHβ were consistent while the expression levels of LHCGR and LHβ was opposite under the influence of MET. The processes for synthesis and release of LH have been proven to be different, synthesized LH was stored in the pituitary gland rather than released into the plasma (Mateos et al., 2002). Thus, the expression of LHCGR in ovary is not directly affected by LHβ expression, the expression of LHCGR may be more influenced by LH in plasma.
In teleost, the type 2, 11β-hydroxysteroid dehydrogenase (HSD11b2) and 3β-hydroxysteroid dehydrogenase (3β-HSD) are involved in androgen synthesis, which are considered to play an important role in masculinity (Kusakabe et al., 2003; Ma et al., 2012). In the present study, HSD11b2 and 3β-HSD expression decreased significantly after MET exposure. Androgen synthesis genes can be suppressed by estrogen-like chemicals, which has been reported in odontesthes bonariensis (Perez et al., 2012), Oncorhynchus mykiss (Nakamura et al., 2009) and Salmo salar (Kortner et al., 2009). The results of the present study were similar to these previous results, which further demonstrate that MET may have estrogenic potency. However, unlike previous studies, plasma T levels did not decrease due to the decreased expression levels of androgen synthesis genes. One explanation for this phenomenon is that production of steroids is a complex process with multiple sensitive control points, so the genes within the steroidogenesis pathway are not transcribed to the same extent and have no simple linear relationship with hormone production. However, whether this reduction in the expression of genes associated with sex steroid levels inhibits the reversal of female M. albus to males requires further investigation.
ESRs are ligand-activated transcription factor that interacts with the response elements in the target gene promoter to activate or inhibit transcription (Heldring et al., 2007). Both estrogens and estrogen-like environmental chemicals can interact with the ligand binding domain (LBD) of ESRs to induce conformational changes of the receptors, and the ligand-receptor complexes may interact with estrogen-responsive elements (ERE) in the promoter regions of target genes and then alters the expression of target genes (Tee et al., 2004). In vertebrates, the expression levels of ESRs are usually closely related to hormone levels (Ogino et al., 2018). The present study found that after exposure to MET, the expression of esr1, esr2a and esr2b in liver decreased to varying degrees, and the expression of ESRs was inconsistent with hormone levels. Previous study demonstrated that basal ESRs levels might be enough to initiate oogenic protein genes (Mortensen and Arukwe, 2007). When basal ESRs levels are depleted, transcriptional machinery is activated to regulate the synthesis of oogenesis protein genes (Pakdel et al., 1997; Yadetie et al., 1999; Bowman et al., 2002). This suggests that even though the expression levels of ESRs are decreased under the MET exposure, the levels of transcriptionally generated ESRs still meet the requirements for regulating vitellogenin production. In addition, ESRs are also thought to play a role in sex differentiation due to their involvement in sex steroid signaling (Ogino et al., 2018). Currently, ESRs have been shown to mediate sex reversal in zebrafish (Lu et al., 2017). However, the decreased expression of hepatic ESRs after MET exposure in this study did not affect vitellogenin production. Therefore, it can be said that MET does not affect the ovarian development of M. albus by inhibiting this mether of oocytes.
To further understand the possible impact of MET on sex reversal, the expressions of sex-related genes Dmrt1, Foxl3 and JNK1 have been studied. In the present study, the expression of Dmrt1 was inhibited under MET exposure, which is similar to the result of the effect of estrogen compounds on other teleosts (Farmer and Orlando, 2012). Dmrt1 control the formation, development and maintenance of male reproductive organs (Gao et al., 2016; Cui et al., 2017). The results showed that the male pathway is inhibited after exposure to MET. In addition, Foxl3 and JNK1 were considered to play a role in sex reversal in M. albus, Foxl3 is thought to promote oocyte degeneration and spermatogenesis, while JNK1 may be important for oocyte growth and differentiation (Xiao et al., 2010; Gao et al., 2016). In the present study, the significant downregulation of foxl3 and non-significant upregulation of JNK1 at all MET concentrations may mean that spermatogenesis is inhibited and oocyte development is promoted after MET exposure. This indicates that MET acts more like an estrogen, inhibiting testis development but promoting oocyte development.
Conclusion
The present study shows that MET has estrogenic effects with perturbance on female M. albus. MET can directly affect the expression of genes on the HPG axis without affecting the level of sex hormones. The exposure of MET increased the expression of CYP19A1b and CYP17, which regulate sex hormone production in the brain, but all genes related to sex hormone secretion in the ovary were suppressed. Furthermore, sex-associated genes did not show a trend of sex reversal after MET influence.
Data availability statement
The original contributions presented in the study are included in the article/Supplementary Material, further inquiries can be directed to the corresponding author.
Ethics statement
Ethical review and approval was not required for the animal study because M. albus used in this study is now cultured extensively in China. No endangered or protected species is involved in this experiment. The whole experimental process follows national and institutional guidelines, so it does not need specific permission.
Author contributions
HW and CZ conceived and designed the research. YZ, TG, LW and XM performed the experiments. YZ, TG, and LW analyzed the data and interpreted the experimental results. YZ wrote the original draft. YZ and HW edited and revised the manuscript. HW and JL approved the final version of the manuscript.
Conflict of interest
The authors declare that the research was conducted in the absence of any commercial or financial relationships that could be construed as a potential conflict of interest.
Publisher’s note
All claims expressed in this article are solely those of the authors and do not necessarily represent those of their affiliated organizations, or those of the publisher, the editors and the reviewers. Any product that may be evaluated in this article, or claim that may be made by its manufacturer, is not guaranteed or endorsed by the publisher.
References
Ankley G. T., Jensen K. M., Durhan E. J., Makynen E. A., Butterworth B. C., Kahl M. D., et al. (2005). Effects of two fungicides with multiple modes of action on reproductive endocrine function in the fathead minnow (Pimephales promelas). Toxicol. Sci. 86, 300–308. doi:10.1093/toxsci/kfi202
Bowman C. J., Kroll K. J., Gross T. G., Denslow N. D. (2002). Estradiol-induced gene expression in largemouth bass (Micropterus salmoides). Mol. Cell. Endocrinol. 196, 67–77. doi:10.1016/S0303-7207(02)00224-1
Callard G. V., Tchoudakova A. V., Kishida M., Wood E. (2001). Differential tissue distribution, developmental programming, estrogen regulation and promoter characteristics of cyp19 genes in teleost fish. J. Steroid. Biochem. Mol. Biol. 79, 305–314. doi:10.1016/S0960-0760(01)00147−9
Chan S., Phillips J. G. (1969). The biosynthesis of steroids by the gonads of the ricefield eel Monopterus albus at various phases during natural sex reversal. Gen. Comp. Endocr. 12, 619–636. doi:10.1016/0016-6480(69)90183-X
Chen C. F., Wen H. S., Wang Z. P., He F., Zhang J. R., Chen X. Y., et al. (2010). Cloning and expression of P450c17-I (17alpha-hydroxylase/17, 20-lyase) in brain and ovary during gonad development in Cynoglossus semilaevis. Fish. Physiol. Biochem. 36, 1001–1012. doi:10.1007/s10695-009-9378-7
Cheng H., He Y., Zhou R. (2021). Swamp eel (Monopterus albus). Trends Genet. 37, 1137–1138. doi:10.1016/j.tig.2021.09.007
Cheshenko K., Pakdel F., Segner H., Kah O., Eggen R. I. (2008). Interference of endocrine disrupting chemicals with aromatase CYP19 expression or activity, and consequences for reproduction of teleost fish. Gen. Comp. Endocrinol. 155, 31–62. doi:10.1016/j.ygcen.2007.03.005
China Fisheries Statistical Yearbook (2020). China Fisheries Statistical Yearbook. Beijing, China: China Agriculture Press, 32–33.
Clelland E., Peng C. (2009). Endocrine/paracrine control of zebrafish ovarian development. Mol. Cell. Endocrinol. 312, 42–52. doi:10.1016/j.mce.2009.04.009
Combarnous Y. (2017). Endocrine Disruptor Compounds (EDCs) and agriculture: The case of pesticides. C. R. Biol. 340, 406–409. doi:10.1016/j.crvi.2017.07.009
Cooper T., Beck L. L., Straw C. M., Henry G. M. (2016). Efficacy of metamifop for the control of common bermudagrass. Horttechnology 26, 394–398. doi:10.21273/HORTTECH.26.4.394
Cui Z., Liu Y., Wang W., Wang Q., Zhang N., Lin F., et al. (2017). Genome editing reveals dmrt1 as an essential male sex-determining gene in Chinese tongue sole (Cynoglossus semilaevis). Sci. Rep. 7, 42213. doi:10.1038/srep42213
de Carvalho A. F. S., Assis I. d. L., Paiva I. M., Mansur V. F. R., Castro T. F. D., Felizardo V. d. O., et al. (2017). Identification of sex in Astronotus ocellatus through the evaluation of aggressive behavior and sexual steroid plasma level. Aquaculture 481, 239–244. doi:10.1016/j.aquaculture.2017.09.010
Faria M., Prats E., Ramírez J. R. R., Bellot M., Pagano M., Valls A., et al. (2021). Androgenic activation, impairment of the monoaminergic system and altered behavior in zebrafish larvae exposed to environmental concentrations of fenitrothion. Sci. Total Environ. 775, 145671. doi:10.1016/j.scitotenv.2021.145671
Farmer J. L., Orlando E. F. (2012). Creating females? Developmental effects of 17alpha-ethynylestradiol on the mangrove rivulus' ovotestis. Integr. Comp. Biol. 52, 769–780. doi:10.1093/icb/ics110
Gao Y., Jia D., Hu Q., Li D. (2016). Foxl3, a target of miR-9, stimulates spermatogenesis in spermatogonia during natural sex change in Monopterus albus. Endocrinology 157, 4388–4399. doi:10.1210/en.2016−1256
Govoroun M., Mcmeel O. M., D'Cotta H., Ricordel M. J., Guiguen Y., Fostier A., et al. (2001). Steroid enzyme gene expressions during natural and androgen-induced gonadal differentiation in the rainbow trout, Oncorhynchus mykiss. J. Exp. Zool. 290, 558–566. doi:10.1002/jez.1106
Habibi H. R., Leeuw R. D., Nahorniak C. S., Goos H., Peter R. E. (1989). Pituitary gonadotropin-releasing hormone (GnRH) receptor activity in goldfish and catfish: Seasonal and gonadal effects. Fish. Physiol. Biochem. 7, 109–118. doi:10.1007/BF00004696
Heldring N., Pike A., Andersson S., Matthews J., Cheng G., Hartman J., et al. (2007). Estrogen receptors: How do they signal and what are their targets. Physiol. Rev. 87, 905–931. doi:10.1152/physrev.00026.2006
IUPAC (2009). Agrochemical information. Available at: http://sitem.herts.ac.uk/aeru/iupac/Reports/1498.htm.
Janaki P., Chinnusamy C. (2012). Determination of metamifop residues in soil under direct-seeded rice. Toxicol. Environ. Chem. 94, 1043–1052. doi:10.1080/02772248.2012.691502
Jo A., Ji K., Choi K. (2014). Endocrine disruption effects of long-term exposure to perfluorodecanoic acid (PFDA) and perfluorotridecanoic acid (PFTrDA) in zebrafish (Danio rerio) and related mechanisms. Chemosphere 108, 360–366. doi:10.1016/j.chemosphere.2014.01.080
Kazeto Y., Place A. R., Trant J. M. (2004). Effects of endocrine disrupting chemicals on the expression of CYP19 genes in zebrafish (Danio rerio) juveniles. Aquat. Toxicol. 69, 25–34. doi:10.1016/j.aquatox.2004.04.008
Kobayashi Y., Alam M. A., Horiguchi R., Shimizu A., Nakamura M. (2010). Sexually dimorphic expression of gonadotropin subunits in the pituitary of protogynous honeycomb grouper (Epinephelus merra): Evidence that follicle-stimulating hormone (FSH) induces gonadal sex change. Biol. Reprod. 82, 1030–1036. doi:10.1095/biolreprod.109.080986
Kobayashi Y., Nakamura M., Sunobe T., Usami T., Kobayashi T., Manabe H., et al. (2009). Sex change in the Gobiid fish is mediated through rapid switching of gonadotropin receptors from ovarian to testicular portion or vice versa. Endocrinology 150, 1503–1511. doi:10.1210/en.2008-0569
Kortner T. M., Vang S. H., Arukwe A. (2009). Modulation of salmon ovarian steroidogenesis and growth factor responses by the xenoestrogen, 4-nonylphenol. Chemosphere 77, 989–998. doi:10.1016/j.chemosphere.2009.07.075
Kusakabe M., Nakamura I., Young G. (2003). 11beta-hydroxysteroid dehydrogenase complementary deoxyribonucleic acid in rainbow trout: Cloning, sites of expression, and seasonal changes in gonads. Endocrinology 144, 2534–2545. doi:10.1210/en.2002-220446
Li F., Li Z., Wang Q., Zhai Y. (2013). Effects of BPA and E2 on expression profiles of genes related to hypothalamic-pituitary-gonadal axis of half-smooth tongue sole Cynoglossus semilaevis. Chin. J. Oceanol. Limn. 31, 598–608. doi:10.1007/s00343-013-2232-x
Li Y., Wang J., Zheng M., Zhang Y., Ru S. (2018). Development of ELISAs for the detection of vitellogenin in three marine fish from coastal areas of China. Mar. Pollut. Bull. 133, 415–422. doi:10.1016/j.marpolbul.2018.06.001
Liu R., Qin Y., Diao J., Zhang H. (2021). Xenopus laevis tadpoles exposed to metamifop: Changes in growth, behavioral endpoints, neurotransmitters, antioxidant system and thyroid development. Ecotoxicol. Environ. Saf. 220, 112417. doi:10.1016/j.ecoenv.2021.112417
Livak K. J., Schmittgen T. D. (2001). Schmittgen, Analysis of relative gene expression data using real-time quantitative PCR and the 2−△△CT method. Methods 25 (4), 402–408. doi:10.1006/meth.2001.1262
Lu H., Cui Y., Jiang L., Ge W. (2017). Functional analysis of nuclear estrogen receptors in zebrafish reproduction by genome editing approach. Endocrinology 158, 2292–2308. doi:10.1210/en.2017-00215
Ma Y., Han J., Guo Y., Lam P. K., Wu R. S., Giesy J. P., et al. (2012). Disruption of endocrine function in in vitro H295R cell-based and in in vivo assay in zebrafish by 2, 4-dichlorophenol. Aquat. Toxicol. 106, 173–181. doi:10.1016/j.aquatox.2011.11.006
Mateos J., ManAnos E., Carrillo M., Zanuy S. (2002). Regulation of follicle-stimulating hormone (FSH) and luteinizing hormone (LH) gene expression by gonadotropin-releasing hormone (GnRH) and sexual steroids in the Mediterranean Sea bass. Comp. Biochem. Phys. B 132, 75–86. doi:10.1016/S1096-4959(01)00535-8
Merola C., Bisegna A., Angelozzi G., Conte A., Abete M. C., Satela C., et al. (2021). Study of heavy metals pollution and vitellogenin levels in Brown trout (Salmo trutta trutta) wild fish populations. Appl. Sci. 11, 4965–4968. doi:10.3390/app11114965
Merola C., Fabrello J., Matozzo V., Faggio C., Iannetta A., Tinelli A., et al. (2022). Dinitroaniline herbicide pendimethalin affects development and induces biochemical and histological alterations in zebrafish early-life stages. Sci. Total Environ. 828, 154414. doi:10.1016/j.scitotenv.2022.154414
Mnif W., Hassine A. I., Bouaziz A., Bartegi A., Thomas O., Roig B. (2011). Effect of endocrine disruptor pesticides: A review. Int. J. Env. Res. Pub. He. 8, 2265–2303. doi:10.3390/ijerph8062265
Montero M., Le Belle N., King J. A., Millar R. P., Dufour S. (1995). Differential regulation of the two forms of gonadotropin-releasing hormone (mGnRH and cGnRH-II) by sex steroids in the European female silver eel (Anguilla anguilla). Neuroendocrinology 61, 525–535. doi:10.1159/000126876
Mortensen A. S., Arukwe A. (2007). Effects of 17 alpha-ethynylestradiol on hormonal responses and xenobiotic biotransformation system of Atlantic salmon (Salmo salar). Aquat. Toxicol. 85, 113–123. doi:10.1016/j.aquatox.2007.08.004
Nakamura I., Kusakabe M., Young G. (2009). Differential suppressive effects of low physiological doses of estradiol-17beta in vivo on levels of mRNAs encoding steroidogenic acute regulatory protein and three steroidogenic enzymes in previtellogenic ovarian follicles of rainbow trout. Gen. Comp. Endocrinol. 163, 318–323. doi:10.1016/j.ygcen.2009.04.031
Nikoleris L., Hultin C. L., Hallgren P., Hansson M. C. (2016). 17alpha-Ethinylestradiol (EE2) treatment of wild roach (Rutilus rutilus) during early life development disrupts expression of genes directly involved in the feedback cycle of estrogen. Comp. Biochem. Physiol. C Toxicol. Pharmacol. 180, 56–64. doi:10.1016/j.cbpc.2015.12.002
Ogino Y., Tohyama S., Kohno S., Toyota K., Yamada G., Yatsu R., et al. (2018). Functional distinctions associated with the diversity of sex steroid hormone receptors ESR and AR. J. Steroid. Biochem. Mol. Biol. 184, 38–46. doi:10.1016/j.jsbmb.2018.06.002
Pakdel F., Delaunay F., Ducouret B., Flouriot G., Kern L., Lazennec G., et al. (1997). Regulation of gene expression and biological activity of rainbow trout estrogen receptor. Fish. Physiol. 17, 123–133. doi:10.1023/A:1007706207857
Parker E. T., Mcelroy J. S., Flessner M. L. (2015). Smooth crabgrass and goosegrass control with metamifop in creeping bentgrass. HortTechnology 25, 757–761. doi:10.21273/HORTTECH.25.6.757
Perez M. R., Fernandino J. I., Carriquiriborde P., Somoza G. M. (2012). Feminization and altered gonadal gene expression profile by ethinylestradiol exposure to pejerrey, Odontesthes bonariensis, a South American teleost fish. Toxicol. Chem. 31, 941–946. doi:10.1002/etc.1789
Pierce J. G., Parsons T. (1981). Glycoprotein hormones: Structure and function. Annu. Rev. Biochem. 50, 465–495. doi:10.1146/annurev.bi.50.070181.002341
Piferrer F., Blazquez M. (2005). Aromatase distribution and regulation in fish. Fish. Physiol. Biochem. 31, 215–226. doi:10.1007/s10695-006-0027-0
Rougeot C., Krim A., Mandiki S. N., Kestemont P., Melard C. (2007). Sex steroid dynamics during embryogenesis and sexual differentiation in Eurasian perch, Perca fluviatilis. Theriogenology 67, 1046–1052. doi:10.1016/j.theriogenology.2006.12.006
Saha S., Majumder S., Das S., Das T. K., Bhattacharyya A., Roy S. (2018). Effect of pH on the transformation of a new readymix formulation of the herbicides bispyribac sodium and metamifop in water. Bull. Environ. Contam. Toxicol. 100, 548–552. doi:10.1007/s00128-018-2269-9
Soni R., Verma S. K. (2020). Impact of herbicide pretilachlor on reproductive physiology of walking catfish, Clarias batrachus (Linnaeus). Fish. Physiol. Biochem. 46 (6), 2065–2072. doi:10.1007/s10695-020-00853-1
Tee M. K., Rogatsky I., Tzagarakis-Foster C., Cvoro A., An J., Christy R. J., et al. (2004). Estradiol and selective estrogen receptor modulators differentially regulate target genes with estrogen receptors alpha and beta. Mol. Biol. Cell. 15, 1262–1272. doi:10.1091/mbc.e03-06-0360
Tyler C. R., Eerden B. V. D., Jobling S., Panter G., Sumpter J. P. (1996). Measurement of vitellogenin, a biomarker for exposure to oestrogenic chemicals, in a wide variety of cyprinid fish. J. Comp. Physiol. B 166, 418–426. doi:10.1007/BF02337886
Wang D. D., Chen Y., Song N. H., Wen-Zhu W. U., Shi L. L. (2012). Residue dynamics of metamifop 10% WP in rice. Agrochemicals 51 (11), 818–821. doi:10.16820/j.cnki.1006-0413.2012.11.013
Xia X., Tang W., He S., Kang J., Ma H., Li J. (2016). Mechanism of metamifop inhibition of the carboxyltransferase domain of acetyl-coenzyme A carboxylase in Echinochloa crus-galli. Sci. Rep. 6, 34066. doi:10.1038/srep34066
Xiao Y. M., Chen L., Liu J., Liu W. B., Chen H. G., Zou L. J., et al. (2010). Contrast expression patterns of JNK1 during sex reversal of the rice-field eel. J. Exp. Zool. B Mol. Dev. Evol. 314, 242–256. doi:10.1002/jez.b.21332
Yadetie F., Arukwe A., Goksoyr A., Male R. (1999). Induction of hepatic estrogen receptor in juvenile Atlantic salmon in vivo by the environmental estrogen, 4-nonylphenol. Sci. Total Environ. 233, 201–210. doi:10.1016/S0048-9697(99)00226-0
Yalsuyi A. M., Forouhar vajargah M., Haijmoradloo A., Galangash M. M., Prokić M. D., Faggio C. (2021). Evaluation of behavioral changes and tissue damages of common carp (Cyprinus carpio) after exposure to herbicide-glyphosate. Veterinary Sci. 8, 218. doi:10.3390/vetsci8100218
Yamamoto F. Y., Garcia J. R. E., Kupsco A., Oliveira Ribeiro C. A. (2017). Vitellogenin levels and others biomarkers show evidences of endocrine disruption in fish species from Iguacu River - southern Brazil. Chemosphere 186, 88–99. doi:10.1016/j.chemosphere.2017.07.111
Zhang L. H., Yang Y. M., Shou J. F., Zang Y., Zhang W. M. (2010). Gonadotropins and their receptors in the hermaphroditic ricefield eel Monopterus albus: cDNA cloning and mRNA expression. Biol. Reprod. 82, 173. doi:10.1093/biolreprod/83.s1.571
Zhang S., Zhang Y., Chen W., Wu Y., Ge W., Zhang L., et al. (2014). Aromatase (Cyp19a1b) in the pituitary is dynamically involved in the upregulation of lhb but not fshb in the vitellogenic female ricefield eel Monopterus albus. Endocrinology 155, 4531–4541. doi:10.1210/en.2014-1069
Zhao F., Li H., Cao F., Chen X., Liang Y., Qiu L. (2019). Short-term developmental toxicity and potential mechanisms of the herbicide metamifop to zebrafish (Danio rerio) embryos. Chemosphere 236, 124590. doi:10.1016/j.chemosphere.2019.124590
Keywords: Monopterus albus, metamifop, endocrine disruption, hypothalamic-pituitary-gonadal axis, sexual reversal
Citation: Zhang Y, Guan T, Wang L, Ma X, Zhu C, Wang H and Li J (2023) Metamifop as an estrogen-like chemical affects the pituitary-hypothalamic-gonadal (HPG) axis of female rice field eels (Monopterus albus). Front. Physiol. 14:1088880. doi: 10.3389/fphys.2023.1088880
Received: 03 November 2022; Accepted: 09 January 2023;
Published: 19 January 2023.
Edited by:
Monia Perugini, University of Teramo, ItalyReviewed by:
Lin Liu, South China Normal University, ChinaFrancesca Falco, National Research Council (CNR), Italy
Copyright © 2023 Zhang, Guan, Wang, Ma, Zhu, Wang and Li. This is an open-access article distributed under the terms of the Creative Commons Attribution License (CC BY). The use, distribution or reproduction in other forums is permitted, provided the original author(s) and the copyright owner(s) are credited and that the original publication in this journal is cited, in accordance with accepted academic practice. No use, distribution or reproduction is permitted which does not comply with these terms.
*Correspondence: Hui Wang, aHl0Y3doaEBoeXRjLmVkdS5jbg==
†These authors have contributed equally to this work