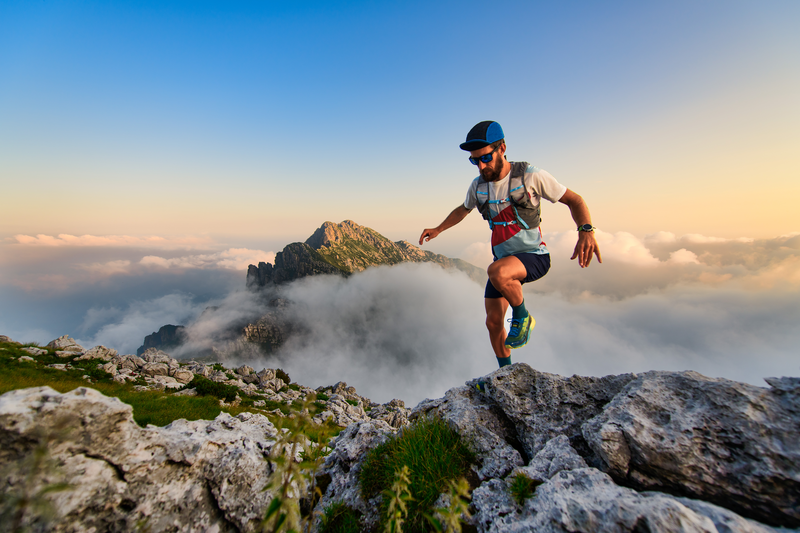
95% of researchers rate our articles as excellent or good
Learn more about the work of our research integrity team to safeguard the quality of each article we publish.
Find out more
ORIGINAL RESEARCH article
Front. Physiol. , 30 January 2023
Sec. Invertebrate Physiology
Volume 14 - 2023 | https://doi.org/10.3389/fphys.2023.1067354
An 8-week feeding trial was conducted to evaluate the effects of L-methionine and methionine hydroxy analogue calcium (MHA-Ca) supplements in low-fishmeal diet on growth performance, hepatopancreas morphology, protein metabolism, anti-oxidative capacity, and immunity of Pacific white shrimp (Litopenaeus vannamei). Four isonitrogenous and isoenergetic diets were designed: PC (203.3 g/kg fishmeal), NC (100 g/kg fishmeal), MET (100 g/kg fishmeal +3 g/kg L-methionine) and MHA-Ca (100 g/kg fishmeal +3 g/kg MHA-Ca). White shrimp (initial body weight 0.23 ± 0.00 g, 50 shrimp per tank) were allocated to 12 tanks and divided among 4 treatments in triplicates. In response to L-methionine and MHA-Ca supplementations, the shrimp exhibited higher weight gain rate (WGR), specific growth rate (SGR), condition factor (CF), and lower hepatosomatic index (HSI) compared to those fed the NC diet (p < 0.05). The WGR and SGR of shrimp fed L-methionine and MHA-Ca showed no difference with those in the PC diet (p > 0.05). Both of L-methionine and MHA-Ca supplementary diets significantly decreased the malondialdehyde (MDA) levels of shrimp when compared with the NC diet (p < 0.05). L-methionine supplementation improved the lysozyme (LZM) activity and total antioxidant capacity (T-AOC) of shrimp, while the MHA-Ca addition elevated the reduced glutathione (GSH) levels in comparison with those fed the NC diet (p < 0.05). Hypertrophied blister cells in hepatocytes were observed in shrimp fed the NC diet, and alleviated with L-methionine and MHA-Ca supplementations. Shrimp fed the MET and MHA-Ca diets had higher mRNA expression levels of target of rapamycin (tor) than those fed the NC diet (p < 0.05). Compared to the NC group, dietary MHA-Ca supplementation upregulated the expression level of cysteine dioxygenase (cdo) (p < 0.05), while L-methionine supplementation had no significant impact (p > 0.05). The expression levels of superoxide dismutase (sod) and glutathione peroxidase (gpx) were significantly upregulated by L-methionine supplemented diet in comparison with those in the NC group (p < 0.05). Overall, the addition of both L-methionine and MHA-Ca elevated the growth performance, facilitated protein synthesis, and ameliorated hepatopancreatic damage induced by plant-protein enriched diet in L. vannamei. L-methionine and MHA-Ca supplements enhanced anti-oxidants differently.
Pacific white shrimp (Litopenaeus vannamei) is preferred by several consumers and aquaculture farmers because of its nutritional value, tenderness, palatability, and high tolerance to salinity and temperature (Zhou et al., 2013; Gao et al., 2017). Fishmeal is an optimal protein source in aquafeeds because of its high level of protein, balanced amino acid composition and taste (Liu et al., 2021). The sustainable development of L. vannamei farming faces challenges due to escalating fishmeal cost and limited amounts of wild fish resources. Thus, reducing the reliance on fishmeal is increasingly inevitable, and plant protein sources are promising alternatives to fishmeal in intensive aquaculture in the view of sustainability, as advocated by the Food and Agriculture Organization of the United Nations (FAO, 2018). Derived from soy, soy protein concentrate has a more reasonable amino acid composition and palatability, as well as fewer anti-nutritional factors, despite the lack of sulfur-containing amino acids (Cruz-Suárez et al., 2009; Bauer et al., 2012; Lu et al., 2021). Among the sulfur-containing amino acids, methionine is considered as the first limiting essential amino acid (EAA) with biological functions in protein synthesis, transmethylation reactions, synthesis of cysteine and cystine, as well as anti-oxidative ability (Mastrototaro et al., 2016; Lu et al., 2021; Zhou et al., 2021). Studies have demonstrated that methionine deficiency may lead to oxidative stress, liver damage and growth retardation in animals (Bin et al., 2017; Lee et al., 2019). Chen et al. (2018) reported that methionine deficiency led to lower weight gain rate, protein retention efficiency and higher feed conversion rate of L. vannamei when 25% fishmeal was replaced with soybean protein concentrate. As such, to cover the methionine requirements of animals, the exploration of methionine supplementation under low-fishmeal aquafeeds is being extensively researched.
Methionine products have been mainly supplemented in the following forms: crystalline DL-methionine, crystalline L-methionine and DL-2-hydroxy-4-methylthiobutanoic acid (methionine hydroxy analogue, MHA). The MHA comes in the form of hydroxy analogue free acid (MHA-FA) or methionine hydroxy analogue salts such as methionine hydroxy analogue calcium (MHA-Ca) (Huyghebaert, 1993; Nunes et al., 2014; Powell et al., 2017; Niu et al., 2018). As products of chemical synthesis, both crystalline DL-methionine and MHA are racemic mixture of two enantiomers (D-isomers and L-isomers) (Sveier et al., 2001). Crystalline amino acids are characterized by a high leaching rate in the aquatic environment, specifically for slow-feeding crustaceans, and have a lower utilization rate due to the rapid absorption and metabolism of free amino acids by animals in comparison with amino acids obtained from digested protein (Chi et al., 2011; Gu et al., 2013). As an organic acid salt rather than an amino acid, MHA exhibits lower water solubility when compared to crystalline methionine, although it cannot be utilized by animals until it is converted into L-methionine after a series of metabolic transformations. In addition, there are distinguished differences between crystalline methionine and MHA in absorption, transport and metabolism in vivo as the result of their different chemical properties (Maenz and Engele-Schaan, 1996; Pham Thi Ha To et al., 2020; To et al., 2021). Recent research has shown that the transport of L-methionine relies on multiple apical sodium-dependent/independent high/low-affinity transporters, while the transport of MHA is mediated by proton-dependent monocarboxylate transporters of the SLC16 family and apical sodium monocarboxylate transporters of the SLC5 family (To et al., 2021). The application effects of crystalline methionine and analogue products are still controversial in aquaculture. Some studies have shown that these methionine products are similar in their ability to satisfy the qualitative requirements of aquatic species without adverse effects (Goff and Gatlin, 2004; Forster and Dominy, 2006; Zhou et al., 2018), while others have indicated that analogue products supplementation was not comparable with crystalline methionine (Keembiyehetty and Gatlin, 1995; Zhao et al., 2017; Chen et al., 2018).
Currently, supplementation of methionine products in low-fishmeal diet of L. vannamei has been a hot research topic. To the best of our knowledge, the enhanced growth performance and antioxidant enzyme response with methionine products supplementation in low-fishmeal diet of L. vannamei have been demonstrated (Gu et al., 2013; Niu et al., 2018; Xie et al., 2018; Wang et al., 2019), yet the physiological mechanism underlying these characteristics is poorly understood. Likewise, the assessment of these different methionine products on the immunity is a field of great interest. Machado et al. (2015) presented that L-methionine supplementation in the diet of European seabass (Dicentrarchus labrax) improved immune response to inflammation induced with UV-inactivated Photobacterium damselae subsp. Piscicida (Phdp). The addition of methionine in low-fishmeal aquafeeds represents a strategy to boost immune ability and welfare, as well as provide a lower-cost alternative to replace antibiotics.
With the progress of production technology, the properties of artificial methionine products have been improved, and the purity of MHA-Ca in product has been elevated from 95.0% to 98.8%. Hence, it is important to continuously assess the efficacy of different methionine products in vivo. The objectives of this study were to compare the impacts of L-methionine and MHA-Ca products in low-fishmeal diets on the growth performance, anti-oxidative ability, methionine metabolism, protein synthesis, and immunity of L. vannamei.
Based on nutritional requirements of L. vannamei, four isonitrogenous (40.21% crude protein) and isoenergetic (17.89 kJ/g gross energy) diets were designed and formulated as shown in Table 1. The dietary design including the supplemental amount of methionine products in this work referred to previous study in our laboratory (Wang et al., 2019). The positive control (PC) diet was formulated with fishmeal at 203.3 g/kg while the negative control (NC) diet contained a lower proportion of fishmeal (100 g/kg) attained with higher soy protein concentrate. At the same fishmeal level as the NC diet, 3 g/kg crystalline L-methionine and 3 g/kg MHA-Ca with 87.8% MHA (purity of 98.8%) were supplemented in the other two diets and designated as MET and MHA-Ca diets, respectively. Among the four diets, fishmeal, soybean meal, fermented soybean meal, soybean protein concentrate and shrimp meal were the main protein sources. Aspartic acid: glycine (1:1) and α-starch were added to realize isonitrogenous and isoenergetic requirements in the four diets. The proximate composition of these ingredients is presented in supplementary material (Supplementary Table S1). The AA profiles and MHA-Ca content of the four diets are shown in Table 2. All ingredients were ground adequately through a 178 μm mesh before being weighed and mixed thoroughly. A feed machine (Modle HKJ-218; HUARUI) was used to transform the homogenized mixture into pellets with a particle size of 1.2 mm. Subsequently, all pellets were steamed for 10 min and dried for 72 h under an air conditioner. All diets were stored at −20 °C until used.
Healthy L. vannamei were obtained from Zhejiang Mariculture Research Institute (Wenzhou, China). The feeding trial was conducted in Xixuan Fishery Science and Technology Island (Zhoushan, China). Before the feeding experiment, shrimp were fed commercial diet for 4 weeks to acclimate to the culture conditions. Following a 24-h fasting, 600 healthy shrimp (initial body weight: 0.23 ± 0.00 g per shrimp) were randomly selected and transferred into 12 500-L fibreglass tanks (50 shrimp per tank), and each diet was assigned to triplicate tanks. During the 8-week feeding trial, shrimp were reared in a flow-through system, supplied with filtered seawater continuously and fed to apparent satiation three times a day (7:00, 13:00 and 18:00) under natural illumination. Any uneaten feed and feces were removed every day. The water conditions were as follows: temperature, 26–28°C; pH, 8.0–8.3; dissolved oxygen, ≥6.5 mg/L; salinity, 27.2–30.0 g/L; and total ammonia nitrogen, 0.3–0.5 mg/L. These rearing conditions are well within the acceptable levels of L.vannamei.
After the 8-week feeding trial and the 24-h fasting period, all surviving shrimp were weighed and counted. 15 shrimp from each tank were selected randomly for the measurement of whole length, body weight and hepatopancreas weight, and then 10 shrimp from each tank were collected for proximate chemical composition analysis. Hemolymph was extracted from the pericardial sinus located at the base of the first abdominal segment using a 1-mL disposable sterile syringe and blended with an equal volume of pre-cooled anticoagulant (0.34 M sodium chloride, 0.16 M disodium hydrogen phosphate, 0.04 M sodium dihydrogen phosphate, 0.02 M EDTA, pH 7.4). Plasma for biochemical analysis was collected after centrifugation at 3500 g, 4°C for 10 min and frozen in liquid nitrogen immediately. Hepatopancreas for subsequent biochemical and genetic analysis were frozen in liquid nitrogen after dissection. Then, samples collected in the liquid nitrogen were stored at − 80°C. For histological observation, hepatopancreas were taken and fixed in 10% (v/v) formaldehyde solution for 24 h, then transferred to 70% ethanol solution until further use.
According to the standards of Association of Official Analytical Chemists (Kavanagh, 2010), moisture, crude protein, crude lipid and ash contents of the four diets and whole shrimp were determined. Following the description in previous research (Yuan et al., 2011; Chen et al., 2018; Niu et al., 2018), the amino acid composition of diets and whole shrimp were determined by an automatic amino acid analyzer (Hitachi LA8080, Tokyo, Japan) after acid hydrolysis in HCl solution, while methionine measurement was conducted by performic acid oxidation methods. The MHA-Ca measurement followed the protocol in Balschukat et al. (1988).
Hepatopancreas were homogenized with PBS (1:9) and centrifuged immediately at 4000 × g, 4°C for 10 min. The obtained supernatants were used to measure biochemical parameters with relevant kits. Specifically, total protein was measured with BCA Protein Assay Kit (A045-3, Jiancheng Bioengineering Institute, Nanjing, China). Total antioxidant capacity (T-AOC), catalase (CAT), superoxide dismutase (SOD), reduced glutathione (GSH), and malondialdehyde (MDA) in supernatants were analyzed with A015–1, A007-1-1, A001–1, A006-1-1, and A003-1 kits (Jiancheng Bioengineering Institute, Nanjing, China), respectively. In addition, total cholesterol (T-CHO), lysozyme (LZM), aspartate aminotransferase (AST), and alanine aminotransferase (ALT) in plasma were detected using A111-1-1, A050-1-1, C010-1-1, and C009-1-1 kits (Jiancheng Bioengineering Institute, Nanjing, China), respectively. The phenoloxidase (PO) determination was as referenced by Lin et al. (2012) with kits (G0146W, Suzhou Grace Biotechnology Co., Ltd., China).
Prefixed hepatopancreas were put in a series of graded ethanol for dehydration, treated with xylene, and embedded in paraffin wax (Gao et al., 2019). Paraffin blocks containing samples were cut into 5-μm-thick sections, stained with hematoxylin and eosin (H&E), and viewed under an optical microscope (Nikon Ni-U, Japan).
Total RNA of hepatopancreas was extracted with Trizol reagent (Vazyme Biotech Co., Ltd., Nanjing, China) as previously described (Liu et al., 2018). The concentration and quality of RNA were examined with NanoDrop 2000 spectrophotometer (Thermo Fisher Scientific, Delaware, USA) and 1% agarose gel electrophoresis. Only RNA sample with OD260/280 ratio between 1.8 and 2.0, and with OD260/230 ratio ≥2.0 were selected to synthesize cDNA using reverse transcription with a Prime Script™ RT Reagent Kit with gDNA Eraser (Takara, China). The specific primers for qRT-PCR were designed by premier primer 5.0 software, and the nucleotide sequences were referenced from NCBI (https://www.ncbi.nlm.nih.gov/) and previous studies. The stability of all primers were examined using 1% agarose gel electrophoresis, and the verification of primers specificity followed protocols in Chang et al. (2018); Bustin et al. (2010). A detailed description of primers can be found in Table 3, with β-actin as the internal control. The cDNA was diluted with DEPC-treated water (1:5), and mixed with primer and reagents TB Green® Premix Ex Taq™ (Takara, China) for qRT-PCR, which was conducted in the LightCycler 480 apparatus (Roche, Switzerland). The reaction profile for qRT-PCR was set up as follows: initial denaturation at 95°C for 1 min, 45 cycles of 5 s denaturation, 15 s annealing at 60°C and 20 s extension at 72°C, following a melting curve and cooling to 37°C. All RT-qPCR reactions were performed in triplicates and the relative expression levels of selected genes were calculated by 2−ΔΔCT (Livak and Schmittgen, 2001).
The growth performance and feed utilization parameters were calculated as follows:
Weight gain rate (WGR, %) = (final body weight–initial body weight)/initial body weight × 100.
Specific growth rate (SGR, %/day) = (ln final body weight–ln initial body weight)/day × 100.
Survival rate (SR, %) = final shrimp number/initial shrimp number × 100.
Condition factor (CF, g/cm3) = body weight/body length3 × 100
Hepatosomatic index (HSI, %) = hepatopancreas weight/whole body weight × 100
Feed conversion rate (FCR) = dry feed intake/(final body weight − initial body weight)
All data were presented as means ± standard deviation (SD) and analyzed for normality and homogeneity in SPSS 19.0 software. The differences among treatments were determined by one-way analysis of variance (ANOVA), followed by Duncan’s multiple-range test at p < 0.05.
The growth and feed utilization parameters of shrimp in response to the four different diets are presented in Table 4. Compared to the NC group, shrimp fed the PC diet exhibited higher WGR, SGR and CF as well as lower HSI (p < 0.05). The supplementation of L-methionine and MHA-Ca (MET and MHA-Ca diets) led to higher WGR, SGR and CF, as well as lower HSI in comparison with those fed the NC diet (p < 0.05), while the HSI of shrimp fed the MHA-Ca diet was higher than those fed the PC and MET diets (p < 0.05). No difference of WGR and SGR was observed among shrimp fed the MET, MHA-Ca and PC diets (p > 0.05).There was no distinction in CF and HSI between the PC and MET groups (p > 0.05). Furthermore, shrimp from the MHA-Ca group showed the highest CF (p < 0.05). The SR and FCR of shrimp did not differ significantly among the four groups (p > 0.05).
The effects of the four different diets on the whole-body composition and amino acid profiles of L. vannamei are shown in Table 5 and Table 6, respectively. No significant difference was found in moisture, crude protein, crude lipid and ash contents of shrimp among the four groups (p > 0.05). Shrimp fed the PC diet presented the highest methionine content among all groups, and those fed the MET and MHA-Ca diets showed significantly higher levels of methionine content than the NC group (p < 0.05). The supplementation of MHA-Ca increased the valine content of shrimp significantly (p < 0.05), with some notable increases in those fed the PC and MET diets as well. The contents of threonine, isoleucine, leucine, phenylalanine, lysine histidine, arginine, and non-essential amino acids (NEAA) of shrimp in different groups were not significantly affected (p > 0.05)
TABLE 6. The amino acid composition in the whole body of L. vannamei fed the experimental diets (g/kg dry matter).
The biochemical parameters in plasma and hepatopancreas of L. vannamei after feeding with the four diets are displayed in Table 7. In the plasma, the highest LZM activity was observed in shrimp fed the MET diet, followed by shrimp fed the PC diet (p < 0.05). No significant difference in T-CHO levels was observed among the four groups (p > 0.05). Shrimp fed the NC diet had the highest AST activity, albeit significantly higher than only the PC group (p < 0.05). No significant difference in ALT of L. vannamei occurred among the four groups (p > 0.05). In the hepatopancreas, the T-AOC level of shrimp fed the MET diet was significantly higher than that of the NC group (p < 0.05). Shrimp from the MHA-Ca group had significantly higher GSH when compared with the NC group (p < 0.05). The highest MDA was observed in shrimp fed the NC diet, and dietary L-methionine and MHA-Ca supplementation reduced the MDA levels significantly (p < 0.05). The results indicated no difference in SOD activity among all groups (p > 0.05).
Histological sections of hepatopancreas tubules from L. vannamei fed the four diets are presented in Figure 1. The blister cells (B-cell), embryonic cells (E cells) and fibrillar cells (F cells) in the hepatocytes were identified in shrimp fed the four diets. Shrimp fed the NC diet had hypertrophied B-cell in hepatopancreas, while L-methionine and MHA-Ca supplementations alleviated the hypertrophy. L. vannamei fed the PC, MET and MHA-Ca diets exhibited normal hepatocyte structures with regular arrangement and no abnormality in tubules.
FIGURE 1. Representative histological photomicrographs of hepatopancreas from L. vannamei fed different diets (H&E staining, magnification × 400). The letters B, E, F in tubules represent blister cells (B cells), embryonic cells (E cells) and fibrillar cells (F cells), respectively.
The expression of genes involved in methionine metabolism, mTOR signaling pathway, anti-oxidative and immune system in hepatopancreas are presented in Figure 2. Shrimp fed the PC diet showed significantly higher expression levels of methionine adenosyltransferase II, alpha (mat2a) and cysteine dioxygenase (cdo) in comparison with those fed the NC diet (p < 0.05). The addition of L-methionine had no significant implication on the expression levels of mat2a and cdo compared to the NC group (p > 0.05). With the supplementation of MHA-Ca, the expression level of cdo was upregulated significantly (p < 0.05). In the mTOR signaling pathway, compared with shrimp fed the NC diet, those fed the PC diet exhibited significantly higher expression levels of target of rapamycin (tor) and Ras-related GTP-binding protein C (rag c) significantly in the mTOR signaling pathway (p < 0.05). Both L-methionine and MHA-Ca supplements significantly upregulated the expression of tor (p < 0.05). The expression of rag c was not influenced by L-methionine or MHA-Ca (p > 0.05). The expression level of eukaryotic translation initiation factor 4 E binding protein 1 (4ebp1) in the MET and MHA-Ca groups showed no differences with another two groups (p > 0.05). In the anti-oxidant system, the expression levels of sod, cat and glutathione peroxidase (gpx) in shrimp fed the PC diet were markedly higher than those in the NC group (p < 0.05). A significant upregulation of sod and gpx was observed in the MET group (p < 0.05). Comparatively, the addition of MHA-Ca had no significant effect on sod and gpx expression (p > 0.05). The expression of cat in the MET and MHA-Ca groups showed no significant difference compared to the NC group (p > 0.05). The expression levels of lzm in shrimp fed the MET and MHA-Ca diets increased slightly, albeit without statistical significance. No significant difference in casp 3 was observed among four groups (p > 0.05).
FIGURE 2. Relative expression of methionine metabolism, mTOR signaling pathway, anti-oxidative and immune system related genes in hepatopancreas of L. vannamei fed with four diets. All gene expression of the NC group was standardized as about 1. All expression values are normalized with β-actin (n=3), with SD denoted by vertical bars. Different letters over columns indicate significantly different (p < 0.05).
In order to reduce the reliance on fishmeal, the feasibility of balancing amino acid composition with synthetic amino acids supplementation in plant-protein diets has been well researched in aquaculture (Hidalgo et al., 1987; Fauconneau, 1988; Ren et al., 2019; Li et al., 2021). Several studies have demonstrated that L-methionine and MHA-Ca can be used as methionine supplements and improve the growth performance of animals (Keembiyehetty and Gatlin, 1995; Goff and Gatlin, 2004; Luo et al., 2005; Powell, et al., 2017), which was also demonstrated in this study. After the 8-week feeding trial, dietary methionine supplementation had a positive effect on the growth performance of L. vannamei, by improving the WGR, SGR and CF, regardless of the source of methionine. Niu et al. (2018) reported that 0.3% DL-methionine (8.2 g/kg methionine content in diet) improved WGR, SGR and SR of L. vannamei significantly. Furthermore, the results of Xie et al. (2018) also indicated that dietary 0.3% DL-methionine supplementation (10.1 g/kg methionine content in diet) could notably improve WGR and SGR of L. vannamei when compared with shrimp fed a low-methionine diet (7.3 g/kg methionine content in diet). The comparison of L-methionine and MHA-Ca was conducted in Goff and Gatlin, (2004). They revealed that both L-methionine and MHA-Ca performed with equivalent efficiency on elevating the growth performance of red drum (Sciaenops ocellatus). In contrast, MHA-Ca showed less efficiency in supporting the growth of channel catfish when compared to L-methionine (Robinson et al., 1978). In this study, L-methionine and MHA-Ca were added in equal amounts rather than as equal amounts of methionine as in most previous studies, and the results indicated similar growth-promoting effects of both on L. vannamei. When comparing the effects of the two additives on growth performance, it is important to consider their characteristics such as solubility, digestion sites and pathways, and purity, as well as the aquatic animal species.
Hepatopancreas, analogous to liver and pancreas in mammals, plays a key role in nutrient metabolism and health for crustaceans (Verri et al., 2001). Our results found higher HSI levels in shrimp fed the NC diet because of methionine deficiency. High HSI is one of the consequences of liver swelling (Li et al., 2016). Gu et al. (2013) demonstrated that the deprivation of methionine in plant-protein diets caused liver swelling from fat accumulation or disorder in sulphur metabolism. The current study detected a decrease in HSI with the use of L-methionine and MHA-Ca supplements. Dietary methionine supplementation is reported to improve amino acid ratio, and it is well known that nutrients balance is crucial to liver size (Hansen et al., 2007). Furthermore, the effective supplementation of methionine in feed has been reported to alleviate fat accumulation in liver (Chiji et al., 1990). In this study, partial fishmeal replacement in the diet NC led to hypertrophied B-cell, which is consistent with results in Burri et al. (2020). Dietary methionine deficiency has been reported to bring about a range of physiological disorders, such as liver damage, and suppression of intestinal epithelial growth (Oz et al., 2007). In previous researches, the histomorphological effects of methionine supplementations on the hepatopancreas have rarely been reported. Normal structure of hepatocytes and regular arrangement of hepatopancreas tubules in the MET and MHA-Ca groups demonstrated the restoration effect of methionine supplementation in low-fishmeal diet on hepatopancreas of L. vannamei.
In the present study, dietary supplementations elevated shrimp methionine content to different extents depending on the king of supplements. The divergence in methionine levels in vivo appeared to be associated with the slow release and absorption of MHA-Ca, while crystalline methionine has high leaching and rapid absorption rate (Drew et al., 2003; Niu et al., 2018). The addition of crystal methionine and MHA-Ca significantly increased growth performance of white shrimp without significant changes in crude protein content. This phenomenon has also been observed in previous studies (Chen et al., 2018; Wang et al., 2019). The fact that protein deposition was not found in crude nutrients might be related to the organization in which protein synthesis occurred. The utilization of different methionine sources was compared using the relative expression levels of associated key genes. Free methionine is absorbed and used for protein synthesis or is converted to S-adenosylmethionine (SAM), a methyl group donor for the methylation, transsulfuration, and aminopropylation (Anstee and Day, 2012; Machado et al., 2021). In the methionine cycle, the synthesis of SAM requires a key molecule methionine adenosyltransferase (MAT) (Froese et al., 2019). As one of MAT isomerases, mat2a is essential to modulate catalytic activity of MAT. In the current study, compared to the higher mat2a level in shrimp fed the PC diet, methionine deficiency signals in the NC group caused the reduction of methionine consumption in methylation cycle (Wan et al., 2017). Most marine animals cannot synthesize enough taurine by themselves to fulfil the requirement for healthy growth and development (Tong et al., 2019). In the process of taurine synthesis, the cdo is sensitive to flux of nutrient availability and considered as key enzyme that catalyzes cysteine sulfur to derive taurine (Salze and Davis, 2015). In the present study, the mat2a, whose expression was unaffected by supplementation of MHA-Ca in diets, and the cdo gene, whose expression was elevated, suggested that the absorbed free amino acids might prefer protein synthesis to the methionine cycle. However, the addition of crystalline L-methionine did not promote the expression of cdo. Zhou et al. (2021) reported that both DL-methionine and MHA-Ca elevated the expression of cdo in common carp (Cyprinus carpio). It was generally believed that the differences in gene expression may be caused by methionine levels, metabolic differences, and species.
With the deepening of research, the mTOR signaling pathway is not only considered as a key node in terms of the immune system, but also an amino acid-sensing pathway to regulate cell growth and autophagy (Powell et al., 2012; Tang et al., 2015). When amino acids are sufficient, the pyruvate dehydrogenase system activates the mTOR pathway, further promotes protein synthesis, and suppresses autophagy (Chotechuang et al., 2009; Jewell and Guan, 2013). In contrast, amino acid starvation triggers amino acid response (AAR) pathway and suppresses the nutrient sensing mTOR pathway to arrest cellular proliferation (Tang et al., 2015). In contrast to the results of Zhou et al. (2021), whose work found that the addition of methionine products did not affect the gene expression level of mTOR in common carp (Cyprinus carpio), the present study showed that both L-methionine and MHA-Ca supplements led to higher levels of tor in the mTOR signaling pathway, albeit still lower than the level of shrimp fed the PC diet. 4ebp1 is a significant translational regulator of mTOR signaling pathway (Fingar and Blenis, 2004). The activation of tor by methionine directly phosphorylates 4ebp1, which enhances protein synthesis and promotes cell growth and proliferation (Hsieh et al., 2010). When amino acid supply is sufficient, Rag family GTPases will facilitate the localization of mTORC1 to the lysosome, thus inducing an increase in mTORC1 activity (Zhu and Thompson, 2019). Although our results indicated no significant changes of rag c even with methionine supplementation, it might be able to activate the expression of other Rag proteins. The stimulatory effect of supplementary methionine on mTOR signaling pathway has been demonstrated in previous studies (Su et al., 2018; Farinha et al., 2021; Lu et al., 2021).
In evaluating the difference of methionine products, it is important to ascertain the impact on nutrients parameters and health-related indices to have a more comprehensive assessment. When about 50% fishmeal was replaced, the T-CHO in plasma remained constant in the current study, and methionine supplements did not alter the T-CHO levels. Lu et al. (2021) suggested that the T-CHO levels appeared to be more closely related to fishmeal levels than methionine in diets. The reduction of T-CHO content in plasma in response to methionine deficiency was observed in L. vannamei when 65% fishmeal was replaced (Ji et al., 2021), while the T-CHO levels of L. vannamei exhibited no alterations when 60% fishmeal was replaced (Wang et al., 2019). As key transaminases in amino acid metabolism, AST and ALT are employed as sensitive hepatic damage biomarkers (Ma et al., 2018; Hu et al., 2021). In this work, higher plasma AST level indicated hepatopancreatic injury in response to low fishmeal. Dietary L-methionine and MHA-Ca supplementations slightly decreased the AST levels. The results of this work suggested the adverse effect of methionine deficiency on the health status of L. vannamei by decreasing anti-oxidants including CAT and GSH, as well as by increasing MDA content, which is an indicator of cell damage as the product of lipid peroxidation (Dawood et al., 2017). Our results corroborated the enhancement of antioxidant system due to methionine supplements. Specifically, the addition of 3 g/kg L-methionine boosted the T-AOC level and the gene expression levels of gpx and sod, while dietary 3 g/kg MHA-Ca supplementation upped GSH of L. vannamei. Meanwhile, both additions reduced MDA levels. T-AOC represents the total antioxidant capacity of animal (Hu et al., 2019). CAT, SOD, GSH and GPx are major anti-oxidants that participate in scavenging reactive oxygen species (ROS) (Chen et al., 2020). Methionine takes the forms of cysteine precursor during transsulfuration pathway in GSH synthesis (Machado et al., 2018). It has been reported that methionine supplementation in feed can reduce ROS-induced tissue damage by increasing GSH activity (Del Vesco et al., 2014). Furthermore, methionine supplementation in diets also exerts a positive effect on other anti-oxidants, such as T-AOC, CAT and SOD (Kuang et al., 2012; Zhou et al., 2018; Ji et al., 2021; Song et al., 2021). In this work, it was found that supplementing with L-methionine markedly increased LZM activity of L. vannamei. By attacking peptidoglycan in the bacterial wall, lysozyme works as part of the innate immunity of invertebrates against bacterial and viral infections (Paulsen et al., 2001; Li et al., 2015).
Methionine supplementation has been proven to elevate the LZM activity of aquatic animals, such as Jian carp (cyprinus carpio var. Jian) and L. vannamei (Tang et al., 2009; Kuang et al., 2012; Lu et al., 2021). The onset of apoptosis is commonly associated with caspase expression (Bratton et al., 2000; Reed, 2000). Caspase 3 causes a protease cascade reaction that ultimately leads to apoptosis (Hastak et al., 2003; Sun et al., 2004). Non-differential casp 3 in L. vannamei fed four diets indicated that no severe apoptosis occurred due to fishmeal replacement with plant protein.
Both 3 g/kg L-methionine and 3 g/kg MHA-Ca supplemented in diets improved the growth performance and methionine content in L. vannamei, and ameliorated morphology of hepatopancreas blighted by the low-fishmeal inclusion. Moreover, the growth performance of shrimp fed L-methionine and MHA-Ca supplements was almost no different from that of shrimp fed the high-fishmeal diet. As a result of dietary L-methionine and MHA-Ca supplementations, different anti-oxidants were enhanced in L. vannamei. Protein synthesis was facilitated by activating the mTOR signaling pathway in response to the addition of L-methionine and MHA-Ca in low-fishmeal diets, although the expression levels of the relevant genes were still lower compared to those of high-fishmeal diet. Moreover, dietary supplementation with MHA-Ca had a beneficial effect on the taurine synthesis pathway.
The original contributions presented in the study are included in the article/Supplementary Material, further inquiries can be directed to the corresponding author.
The animal study was reviewed and approved by laboratory animal welfare and ethics committee of Zhejiang University (Ethical approval code: ZJU20210256).
LZ: conceptualization; methodology; sample collection; formal analysis; writing–original draft. YL: software; data curation; sample collection; formal analysis. YZ: formal analysis; writing–review & editing. BX: sample collection; formal analysis. GS: sample collection; writing–review & editing. ZW: resources; formal analysis. CC: resources; formal analysis. XL: resources; formal analysis. JZ: resources; formal analysis. QS: resources; conceptualization; methodology; writing–review & editing; supervision; project administration.
This work was supported by the Ministry of Science and Technology, China (Project No.: 2020YFD0900801) and Shandong NHU Amino Acid Co., Ltd., China.
We are very grateful to the allocation of rearing system and logistic support from the China-Norwegian Joint Laboratory of Nutrition and Feed for Marine Fish (Xixuan Island, Zhoushan City, Zhejiang Province) and the Key Lab of Mari culture and Breeding of Zhejiang Province. Also, thanks to Yuxiao Sun, Fabrice Arnaud Tegomo in our lab, and Tan Peng of Zhejiang Ocean University for their assistance.
Authors ZW, CC and XL were employed by Shandong NHU Amino Acid Co., Ltd. JZ was employed by Fujian Tianma Science and Technology Co., Ltd.
The remaining authors declare that the research was conducted in the absence of any commercial or financial relationships that could be construed as a potential conflict of interest.
All claims expressed in this article are solely those of the authors and do not necessarily represent those of their affiliated organizations, or those of the publisher, the editors and the reviewers. Any product that may be evaluated in this article, or claim that may be made by its manufacturer, is not guaranteed or endorsed by the publisher.
The Supplementary Material for this article can be found online at: https://www.frontiersin.org/articles/10.3389/fphys.2023.1067354/full#supplementary-material
Anstee Q. M., Day C. P. (2012). S-Adenosylmethionine (SAMe) therapy in liver disease: A review of current evidence and clinical utility. J. Hepatol. 57, 1097–1109. doi:10.1016/j.jhep.2012.04.041
Balschukat D., Kresse E., Tanner H. (1988). Quantitative determination of methionine and its hydroxy-analogues in compound feed. Landwirtsch. Forsch. 41 (1/2), 120–130.
Bauer W., Prentice-Hernandez C., Tesser M. B., Wasielesky W., Poersch L. H. S. (2012). Substitution of fishmeal with microbial floc meal and soy protein concentrate in diets for the Pacific white shrimp Litopenaeus vannamei. Aquaculture 342-343, 112–116. doi:10.1016/j.aquaculture.2012.02.023
Bin P., Huang R., Zhou X. (2017). Oxidation resistance of the sulfur amino acids: Methionine and cysteine. Biomed. Res. Int. 2017, 9584932. doi:10.1155/2017/9584932
Bratton S. B., MacFarlane M., Cain K., Cohen G. M. (2000). Protein complexes activate distinct caspase cascades in death receptor and stress-induced apoptosis. Exp. Cell Res. 256 (1), 27–33. doi:10.1006/excr.2000.4835
Burri L., Berge K., Chumkam S., Jintasataporn O. (2020). Effects of different feed inclusion levels of krill meal on growth and hepatopancreas morphology of Litopenaeus vannamei. Aquac. Res. 51, 4819–4823. doi:10.1111/are.14809
Bustin S. A., Beaulieu J. F., Huggett J., Jaggi R., Kibenge F. S. B., Olsvik P. A., et al. (2010). MIQE precis: Practical implementation of minimum standard guidelines for fluorescence-based quantitative real-time PCR experiments. BMC Mol. Biol. 11, 74. doi:10.1186/1471-2199-11-74
Cardona E., Saulnier D., Lorgeoux B., Chim L., Gueguen Y. (2015). Rearing effect of biofloc on antioxidant and antimicrobial transcriptional response in Litopenaeus stylirostris shrimp facing an experimental sub-lethal hydrogen peroxide stress. Fish. Shellfish Immunol. 45, 933–939. doi:10.1016/j.fsi.2015.05.041
Chang H. Y., Lin T. H., Anraku K., Shao Y. T. (2018). The effects of continuous acoustic stress on ROS levels and antioxidant-related gene expression in the black porgy (Acanthopagrus schlegelii). Zool. Stud. 57, e59. doi:10.6620/ZS.2018.57-59
Chen J., Li X., Huan D., Yao W., Leng X. (2018). Comparative study on the utilization of crystalline methionine and methionine hydroxy analogue calcium by Pacific white shrimp (Litopenaeus vannamei Boone). Aquac. Res. 49, 3088–3096. doi:10.1111/are.13771
Chi S.-Y., Tan B.-P., Lin H.-Z., Mai K.-S., Ai Q.-H., Wang X.-J., et al. (2011). Effects of supplementation of crystalline or coated methionine on growth performance and feed utilization of the Pacific white shrimp, Litopenaeus vannamei. Aquac. Nutr. 17, e1–e9. doi:10.1111/j.1365-2095.2009.00710.x
Chiji H., Harayama K., Kiriyama S. (1990). Effects of feeding rats low protein diets containing casein or soy protein isolate supplemented with methionine or oligo-L-methionine. J. Nutr. 120, 166–171. doi:10.1093/jn/120.2.166
Chipponi J. X., Bleier J. C., Santi M. T., Rudman D. (1982). Deficiencies of essential and conditionally essential nutrients. Am. J. Clin. Nutr. 35, 1112–1116. doi:10.1093/ajcn/35.5.1112
Chotechuang N., Azzout-Marniche D., Bos C., Chaumontet C., Gausseres N., Steiler T., et al. (2009). mTOR, AMPK, and GCN2 coordinate the adaptation of hepatic energy metabolic pathways in response to protein intake in the rat. Am. J. Physiol.-Endocrinol. Metab. 297, 1313–1323. doi:10.1152/ajpendo.91000.2008
Cruz-Suárez L. E., Tapia-Salazar M., Villarreal-Cavazos D., Beltran-Rocha J., Nieto-López M. G., Lemme A., et al. (2009). Apparent dry matter, energy, protein and amino acid digestibility of four soybean ingredients in white shrimp Litopenaeus vannamei juveniles. Aquaculture 292, 87–94. doi:10.1016/j.aquaculture.2009.03.026
Dawood M. A. O., Koshio S., El-Sabagh M., Billah M. M., Zaineldin A. I., Zayed M. M., et al. (2017). Changes in the growth, humoral and mucosal immune responses following β-glucan and vitamin C administration in red sea bream, Pagrus major. Aquaculture 470, 214–222. doi:10.1016/j.aquaculture.2016.12.036
Del Vesco A. P., Gasparino E., Grieser D. O., Zancanela V., Gasparin F. R. S., Constantin J., et al. (2014). Effects of methionine supplementation on the redox state of acute heat stress-exposed quails. J. Anim. Sci. 92, 806–815. doi:10.2527/jas.2013-6829
Dibner J. J., Knight C. D. (1984). Conversion of 2-hydroxy-4-(methylthio)butanoic acid to L-methionine in the chick: A stereospecific pathway. J. Nutr. 114, 1716–1723. doi:10.1093/jn/114.9.1716
Drew M. D., Van Kessel A. G., Maenz D. D. (2003). Absorption of methionine and 2-hydroxy-4-methylthiobutoanic acid in conventional and germ-free chickens. Poult. Sci. 82, 1149–1153. doi:10.1093/ps/82.7.1149
FAO (2018). The state of world fisheries and aquaculture 2018: Meeting the sustainable development goals. Rome: FAO.
Farinha A. P., Schrama D., Silva T., Conceição L. E. C., Colen R., Engrola S., et al. (2021). Evaluating the impact of methionine-enriched diets in the liver of European seabass through label-free shotgun proteomics. J. Proteomics. 232, 104047. doi:10.1016/j.jprot.2020.104047
Fauconneau B. (1988). Partial substitution of protein by a single amino acid or an organic acid in rainbow trout diets. Aquaculture 70, 97–106. doi:10.1016/0044-8486(88)90009-9
Fingar D. C., Blenis J. (2004). Target of rapamycin (TOR): An integrator of nutrient and growth factor signals and coordinator of cell growth and cell cycle progression. Oncogene 23, 3151–3171. doi:10.1038/sj.onc.1207542
Forster I. P., Dominy W. G. (2006). Efficacy of three methionine sources in diets for Pacific white shrimp, Litopenaeus vannamei. J. World Aquacult. Soc. 37, 474–480. doi:10.1111/j.1749-7345.2006.00060.x
Friedman M., Gumbmann M. R. (1984). The utilization and safety of isomeric sulfur-containing amino acids in mice. J. Nutr. 114, 2301–2310. doi:10.1093/jn/114.12.2301
Froese D. S., Fowler B., Baumgartner M. R. (2019). Vitamin B12, folate, and the methionine remethylation cycle—Biochemistry, pathways, and regulation. J. Inherit. Metab. Dis. 42, 673–685. doi:10.1002/jimd.12009
Gao F., Kataoka M., Liu N., Liang T., Huang Z.-P., Gu F., et al. (2019). Therapeutic role of miR-19a/19b in cardiac regeneration and protection from myocardial infarction. Nat. Commun. 10, 1802. doi:10.1038/s41467-019-09530-1
Gao J., Zuo H., Yang L., He J.-H., Niu S., Weng S., et al. (2017). Long-term influence of cyanobacterial bloom on the immune system of Litopenaeus vannamei. Fish. Shellfish Immunol. 61, 79–85. doi:10.1016/j.fsi.2016.12.015
Goff J. B., Gatlin D. M. (2004). Evaluation of different sulfur amino acid compounds in the diet of red drum, Sciaenops ocellatus, and sparing value of cystine for methionine. Aquaculture 241, 465–477. doi:10.1016/j.aquaculture.2004.08.002
Gu M., Zhang W. B., Bai N., Mai K. S., Xu W. (2013). Effects of dietary crystalline methionine or oligo-methionine on growth performance and feed utilization of white shrimp (Litopenaeus vannamei) fed plant protein-enriched diets. Aquac. Nutr. 19, 39–46. doi:10.1111/anu.12089
Hansen A.-C., Rosenlund G., Karlsen Ø., Koppe W., Hemre G.-I. (2007). Total replacement of fish meal with plant proteins in diets for Atlantic cod (Gadus morhua L.) I — effects on growth and protein retention. Aquaculture 272, 599–611. doi:10.1016/j.aquaculture.2007.08.034
Hastak K., Gupta S., Ahmad N., Agarwal M. K., Agarwal M. L., Mukhtar H. (2003). Role of p53 and NF-kappaB in epigallocatechin-3-gallate-induced apoptosis of LNCaP cells. Oncogene 22, 4851–4859. doi:10.1038/sj.onc.1206708
Hidalgo F., Alliot E., Thebault H. (1987). Methionine- and cystine-supplemented diets for juvenile sea bass (Dicentrarchus labrax). Aquaculture 64, 209–217. doi:10.1016/0044-8486(87)90326-7
Hsieh A. C., Costa M., Zollo O., Davis C., Feldman M. E., Testa J. R., et al. (2010). Genetic dissection of the oncogenic mTOR pathway reveals druggable addiction to translational control via 4EBP-eIF4E. Cancer Cell 17, 249–261. doi:10.1016/j.ccr.2010.01.021
Hu B., Song L.-p., Mao S.-q., Xu P. (2019). Effects of four Chinese herbal preparations on growth performance and antioxidant activity in juvenile Micropterus salmoides. Acta Geophys. Sin. 39, 101.
Hu Y., Cai M., Zhong H., Chu W., Hu Y. (2021). A study on how methionine restriction decreases the body's hepatic and lipid deposition in rice field eel (Monopterus albus). Int. J. Mol. Sci. 22, 13379. doi:10.3390/ijms222413379
Huyghebaert G. (1993). Comparison of DL-methionine and methionine hydroxy analogue-free acid in broilers by using multi-exponential regression models. Br. Poult. Sci. 34, 351–359. doi:10.1080/00071669308417590
Jewell J. L., Guan K. L. (2013). Nutrient signaling to mTOR and cell growth. Trends Biochem. Sci. 38, 233–242. doi:10.1016/j.tibs.2013.01.004
Ji R., Wang Z., He J., Masagounder K., Xu W., Mai K., et al. (2021). Effects of DL-methionyl-DL-methionine supplementation on growth performance, immune and antioxidative responses of white leg shrimp (Litopenaeus vannamei) fed low fishmeal diet. Aquacult. Rep. 21, 100785. doi:10.1016/j.aqrep.2021.100785
Kavanagh F. (2010). “Official methods of analysis of the AOAC,” in Journal of pharmaceutical Sciences. Editor W. Horwitz 13th Edn (Arlington, VA: The Association of Official Analytical Chemists).
Keembiyehetty C. N., Gatlin D. M. (1995). Evaluation of different sulfur compounds in the diet of juvenile sunshine bass (Morone chrysops ♀ × M. Saxatilis ♂). Comp. Biochem. Physiol. 112, 155–159. doi:10.1016/0300-9629(95)00075-I
Koehnle T. J., Russell M. C., Gietzen D. W. (2003). Rats rapidly reject diets deficient in essential amino acids. J. Nutr. 133, 2331–2335. doi:10.1093/jn/133.7.2331
Kuang S. Y., Xiao W. W., Feng L., Liu Y., Jiang J., Jiang W. D., et al. (2012). Effects of graded levels of dietary methionine hydroxy analogue on immune response and antioxidant status of immune organs in juvenile Jian carp (Cyprinus carpio var. Jian). Fish. Shellfish Immunol. 32, 629–636. doi:10.1016/j.fsi.2011.12.012
Lee S., Kwak J.-H., Kim S. H., Jeong T. B., Son S. W., Kim J.-H., et al. (2019). Comparative study of liver injury induced by high-fat methionine- and choline-deficient diet in ICR mice originating from three different sources. Lab. Anim. Res. 35, 15. doi:10.1186/s42826-019-0016-y
Li J., Xu Y., Jin L., Li X. (2015). Effects of a probiotic mixture (Bacillus subtilis YB-1 and Bacillus cereus YB-2) on disease resistance and non-specific immunity of sea cucumber, Apostichopus japonicus (Selenka). Aquac. Res. 46, 3008–3019. doi:10.1111/are.12453
Li M., Lai H., Li Q., Gong S., Wang R. (2016). Effects of dietary taurine on growth, immunity and hyperammonemia in juvenile yellow catfish Pelteobagrus fulvidraco fed all-plant protein diets. Aquaculture 450, 349–355. doi:10.1016/j.aquaculture.2015.08.013
Li S., Dai M., Qiu H., Chen N. (2021). Effects of fishmeal replacement with composite mixture of shrimp hydrolysate and plant proteins on growth performance, feed utilization, and target of rapamycin pathway in largemouth bass, Micropterus salmoides. Micropterus Salmoides. Aquac. 533, 736185. doi:10.1016/j.aquaculture.2020.736185
Li Z., Wang F., Liang B., Su Y., Sun S., Xia S., et al. (2020). Methionine metabolism in chronic liver diseases: An update on molecular mechanism and therapeutic implication. Signal Transduct. Target. Ther. 5, 280. doi:10.1038/s41392-020-00349-7
Lin Y. C., Chen J. C., Sn C. M., Wz W. M., As N. A. S., Cheng S. Y., et al. (2012). Modulation of innate immunity and gene expressions in white shrimp Litopenaeus vannamei following long-term starvation and re-feeding. Results Immunol. 2, 148–156. doi:10.1016/j.rinim.2012.07.001
Liu Y., Lu Q., Xi L., Gong Y., Su J., Han D., et al. (2021). Effects of replacement of dietary fishmeal by cottonseed protein concentrate on growth performance, liver health, and intestinal histology of largemouth bass (Micropterus salmoides). Front. Physiol. 12, 764987. doi:10.3389/fphys.2021.764987
Liu Z. P., Gu W. B., Tu D. D., Zhu Q. H., Zhou Y. L., Wang C., et al. (2018). Effects of both cold and heat stress on the liver of the giant spiny frog (Quasipaa spinosa): Stress response and histological changes. J. Exp. Biol. 221, jeb186379. doi:10.1242/jeb.186379
Livak K. J., Schmittgen T. D. (2001). Analysis of relative gene expression data using real-time quantitative PCR and the 2−ΔΔCT method. Methods 25, 402–408. doi:10.1006/meth.2001.1262
Lu J., Zhang X., Zhou Q., Cheng Y., Luo J., Masagounder K., et al. (2021). Dietary DL-methionyl-DL-methionine supplementation could improve growth performance under low fishmeal strategies by modulating TOR signalling pathway of Litopenaeus vannamei. Aquac. Nutr. 27, 1921–1933. doi:10.1111/anu.13329
Luo Z., Liu Y.-j., Mai K.-s., Tian L.-x., Yang H.-j., Tan X.-y., et al. (2005). Dietary L-methionine requirement of juvenile grouper Epinephelus coioides at a constant dietary cystine level. Aquaculture 249, 409–418. doi:10.1016/j.aquaculture.2005.04.030
Ma S., Huang Q., Yada K., Liu C., Suzuki K. (2018). An 8-week ketogenic low carbohydrate, high fat diet enhanced exhaustive exercise capacity in mice. Nutrients 10, 673. doi:10.3390/nu10060673
Machado M., Azeredo R., Diaz-Rosales P., Afonso A., Peres H., Oliva-Teles A., et al. (2015). Dietary tryptophan and methionine as modulators of European seabass (Dicentrarchus labrax) immune status and inflammatory response. Fish. Shellfish Immunol. 42, 353–362. doi:10.1016/j.fsi.2014.11.024
Machado M., Azeredo R., Fontinha F., Fernandez-Boo S., Conceicao L. E. C., Dias J., et al. (2018). Dietary methionine improves the European seabass (Dicentrarchus labrax) immune status, inflammatory response, and disease resistance. Front. Immunol. 9, 2672. doi:10.3389/fimmu.2018.02672
Machado M., Serra C. R., Oliva-Teles A., Costas B. (2021). Methionine and tryptophan play different modulatory roles in the European seabass (Dicentrarchus labrax) innate immune response and apoptosis signaling — an in vitro study. Front. Immunol. 12, 660448. doi:10.3389/fimmu.2021.660448
Maenz D. D., Engele-Schaan C. M. (1996). Methionine and 2-hydroxy-4-methylthiobutanoic acid are transported by distinct Na+-dependent and H+-dependent systems in the brush border membrane of the chick intestinal epithelium. J. Nutr. 126, 529–536. doi:10.1093/jn/126.2.529
Mastrototaro L., Sponder G., Saremi B., Aschenbach J. R. (2016). Gastrointestinal methionine shuttle: Priority handling of precious goods. IUBMB Life 68, 924–934. doi:10.1002/iub.1571
Mente E., Deguara S., Santos M. B., Houlihan D. (2003). White muscle free amino acid concentrations following feeding a maize gluten dietary protein in Atlantic salmon (Salmo salar L.). Aquaculture 225, 133–147. doi:10.1016/S0044-8486(03)00285-0
Niu J., Lemme A., He J.-Y., Li H.-Y., Xie S.-W., Liu Y.-J., et al. (2018). Assessing the bioavailability of the Novel Met-Met product (AQUAVI® Met-Met) compared to DL-methionine (DL-Met) in white shrimp (Litopenaeus vannamei). Aquaculture 484, 322–332. doi:10.1016/j.aquaculture.2017.08.021
Nunes A. J. P., Sá M. V. C., Browdy C. L., Vazquez-Anon M. (2014). Practical supplementation of shrimp and fish feeds with crystalline amino acids. Aquaculture 431, 20–27. doi:10.1016/j.aquaculture.2014.04.003
Oz H. S., Chen T. S., Neuman M. (2007). Methionine deficiency and hepatic injury in a dietary steatohepatitis model. Dig. Dis. Sci. 53, 767–776. doi:10.1007/s10620-007-9900-7
Paulsen S. M., Engstad R. E., Robertsen B. (2001). Enhanced lysozyme production in Atlantic salmon (Salmo salar L.) macrophages treated with yeast β-glucan and bacterial lipopolysaccharide. Fish. Shellfish Immunol. 11, 23–37. doi:10.1006/fsim.2000.0291
Pham Thi Ha To V., Subramaniam M., Masagounder K., Loewen M. E. (2020). Characterization of the segmental transport mechanisms of DL-methionine hydroxy analogue along the intestinal tract of rainbow trout with an additional comparison to DL-methionine. Comp. Biochem. Physiol. A-Mol. Integr. Physiol. 249, 110776. doi:10.1016/j.cbpa.2020.110776
Powell C. D., Chowdhury M. A. K., Bureau D. P. (2017). Assessing the bioavailability of L-methionine and a methionine hydroxy analogue (MHA-Ca) compared to DL-methionine in rainbow trout (Oncorhynchus mykiss). Aquac. Res. 48, 332–346. doi:10.1111/are.12885
Powell J. D., Pollizzi K. N., Heikamp E. B., Horton M. R. (2012). Regulation of immune responses by mTOR. Annu. Rev. Immunol. 30, 39–68. doi:10.1146/annurev-immunol-020711-075024
Reed J. C. (2000). Mechanisms of apoptosis. Am. J. Pathol. 157 (5), 1415–1430. doi:10.1016/S0002-9440(10)64779-7
Ren M., He J., Liang H., Ji K., Ge X., Sun A., et al. (2019). Use of supplemental amino acids on the success of reducing dietary protein levels for Jian carp (Cyprinus carpio var. Jian). Aquac. Nutr. 25, 567–576. doi:10.1111/anu.12879
Robinson E. H., Allen O. W., Poe W. E., Wilson R. P. (1978). Utilization of dietary sulfur compounds by fingerling channel catfish: L-methionine, DL-methionine, methionine hydroxy analogue, taurine and inorganic sulfate. J. Nutr. 108 (12), 1932–1936. doi:10.1093/jn/108.12.1932
Salze G. P., Davis D. A. (2015). Taurine: A critical nutrient for future fish feeds. Aquaculture 437, 215–229. doi:10.1016/j.aquaculture.2014.12.006
Song B., Zeng Q., Liu Y., Wu B. (2021). Effect of methionine deficiency on the apoptosis and cell cycle of kidney in broilers. Res. Vet. Sci. 135, 228–236. doi:10.1016/j.rvsc.2019.09.013
Su Y. N., Wu P., Feng L., Jiang W. D., Jiang J., Zhang Y. A., et al. (2018). The improved growth performance and enhanced immune function by DL-methionyl-DL-methionine are associated with NF-kappaB and TOR signalling in intestine of juvenile grass carp (Ctenopharyngodon idella). Fish. Shellfish Immunol. 74, 101–118. doi:10.1016/j.fsi.2017.12.051
Sun X.-M., Butterworth M., MacFarlane M., Dubiel W., Ciechanover A., Cohen G. M. (2004). Caspase activation inhibits proteasome function during apoptosis. Mol. Cell 14, 81–93. doi:10.1016/S1097-2765(04)00156-X
Sveier Nordås, Berge Lied (2001). Dietary inclusion of crystalline D- and L-methionine: Effects on growth, feed and protein utilization, and digestibility in small and large atlantic salmon (Salmon salar L.). Aquac. Nutr. 7, 169–181. doi:10.1046/j.1365-2095.2001.00169.x
Tang L., Wang G. X., Jiang J., Feng L., Yang L., Li S. H., et al. (2009). Effect of methionine on intestinal enzymes activities, microflora and humoral immune of juvenile Jian carp (Cyprinus carpio var. Jian). Aquac. Nutr. 15, 477–483. doi:10.1111/j.1365-2095.2008.00613.x
Tang X., Keenan M. M., Wu J., Lin C. A., Dubois L., Thompson J. W., et al. (2015). Comprehensive profiling of amino acid response uncovers unique methionine-deprived response dependent on intact creatine biosynthesis. PLoS Genet. 11, e1005158. doi:10.1371/journal.pgen.1005158
To V. P. T. H., Masagounder K., Loewen M. E. (2021). Critical transporters of methionine and methionine hydroxyl analogue supplements across the intestine: What we know so far and what can be learned to advance animal nutrition. Comp. Biochem. Physiol. A-Mol. Integr. Physiol. 255, 110908. doi:10.1016/j.cbpa.2021.110908
Tong S., Wang L., Kalhoro H., Volatiana J. A., Shao Q. (2019). Effects of supplementing taurine in all-plant protein diets on growth performance, serum parameters, and cholesterol 7α-hydroxylase gene expression in black sea bream,Acanthopagrus schlegelii. Acanthopagrus Schlegelii. J. World Aquacult. Soc. 51, 990–1001. doi:10.1111/jwas.12611
Vázquez-Añón M., Bertin G., Mercier Y., Reznik G., Roberton J. L. (2017). Review of the chemistry, metabolism, and dose response of two supplemental methionine sources and the implications in their relative bioefficacy. Worlds Poult. Sci. J. 73, 725–736. doi:10.1017/S0043933917000551
Velázquez-Lizárraga A. E., Juárez-Morales J. L., Racotta I. S., Villarreal-Colmenares H., Valdes-Lopez O., Luna-González A., et al. (2019). Transcriptomic analysis of Pacific white shrimp (Litopenaeus vannamei, Boone 1931) in response to acute hepatopancreatic necrosis disease caused by Vibrio parahaemolyticus. PLoS One 14, e0220993. doi:10.1371/journal.pone.0220993
Verri T., Mandal A., Zilli L., Bossa D., Mandal P. K., Ingrosso L., et al. (2001). D-Glucose transport in decapod crustacean hepatopancreas. Comp. Biochem. Physiol. A-Mol. Integr. Physiol. 130, 585–606. doi:10.1016/S1095-6433(01)00434-2
Wan J., Ding X., Wang J., Bai S., Peng H., Luo Y., et al. (2017). Dietary methionine source and level affect hepatic sulfur amino acid metabolism of broiler breeder hens. Anim. Sci. J. 88, 2016–2024. doi:10.1111/asj.12882
Wang L., Ye L., Hua Y., Zhang G., Li Y., Zhang J., et al. (2019). Effects of dietary dl-methionyl-dl-methionine (met-met) on growth performance, body composition and haematological parameters of white shrimp (Litopenaeus vannamei) fed with plant protein-based diets. Aquac. Res. 50, 1718–1730. doi:10.1111/are.14064
Won S., Hamidoghli A., Choi W., Bae J., Jang W. J., Lee S., et al. (2020). Evaluation of potential probiotics Bacillus subtilis WB60, Pediococcus pentosaceus, and Lactococcus lactis on growth performance, immune response, gut histology and immune-related genes in whiteleg shrimp, Litopenaeus vannamei. Microorganisms 8, 281. doi:10.3390/microorganisms8020281
Xie J. J., Lemme A., He J. Y., Yin P., Figueiredo-Silva C., Liu Y. J., et al. (2018). Fishmeal levels can be successfully reduced in white shrimp (Litopenaeus vannamei) if supplemented with DL-Methionine (DL-Met) or DL-Methionyl-DL-Methionine (Met-Met). Aquac. Nutr. 24, 1144–1152. doi:10.1111/anu.12653
Yuan Y.-c., Gong S.-y., Yang H.-j., Lin Y.-c., Yu D.-h., Luo Z. (2011). Effects of supplementation of crystalline or coated lysine and/or methionine on growth performance and feed utilization of the Chinese sucker, Myxocyprinus asiaticus. Myxocyprinus Asiat. Aquac. 316, 31–36. doi:10.1016/j.aquaculture.2011.03.015
Zhao J.-X., Li X.-Q., Leng X.-J., Peng S., Hu J., Zhao X.-X. (2017). Comparative study on the utilization of different methionine sources by channel catfish, Ictalurus punctatus (Rafinesque, 1818). Aquac. Res. 48, 3618–3630. doi:10.1111/are.13187
Zhou F., Wang Y.-Q., Bei Y.-J., Ng W.-K., Wang D.-N., Li S.-Y., et al. (2018). Assessing the efficacy of three methionine sources in low protein and low fish meal diet for Chinese soft-shelled turtle, Pelodiscus sinensis. Aquac. Int. 26, 15–26. doi:10.1007/s10499-017-0193-3
Zhou Q.-C., Wang Y.-L., Wang H.-L., Tan B.-P. (2013). Dietary threonine requirements of juvenile Pacific white shrimp, Litopenaeus vannamei. Aquaculture 392-395, 142–147. doi:10.1016/j.aquaculture.2013.01.026
Zhou Y., He J., Su N., Masagounder K., Xu M., Chen L., et al. (2021). Effects of DL-methionine and a methionine hydroxy analogue (MHA-Ca) on growth, amino acid profiles and the expression of genes related to taurine and protein synthesis in common carp (Cyprinus carpio). Aquaculture 532, 735962. doi:10.1016/j.aquaculture.2020.735962
Keywords: growth performance, anti-oxidative capacity, protein synthesis, immunity, methionine, Litopenaeus vannamei
Citation: Zheng L, Liu Y, Zhang Y, Xu B, Sagada G, Wang Z, Chen C, Lang X, Zhang J and Shao Q (2023) Comparative study on the effects of crystalline L-methionine and methionine hydroxy analogue calcium supplementations in the diet of juvenile Pacific white shrimp (Litopenaeus vannamei). Front. Physiol. 14:1067354. doi: 10.3389/fphys.2023.1067354
Received: 11 October 2022; Accepted: 17 January 2023;
Published: 30 January 2023.
Edited by:
Sylvia Anton, Institut National de recherche pour l’agriculture, l’alimentation et l’environnement (INRAE), FranceReviewed by:
Xianyong Bu, Ocean University of China, ChinaCopyright © 2023 Zheng, Liu, Zhang, Xu, Sagada, Wang, Chen, Lang, Zhang and Shao. This is an open-access article distributed under the terms of the Creative Commons Attribution License (CC BY). The use, distribution or reproduction in other forums is permitted, provided the original author(s) and the copyright owner(s) are credited and that the original publication in this journal is cited, in accordance with accepted academic practice. No use, distribution or reproduction is permitted which does not comply with these terms.
*Correspondence: Qingjun Shao, cWpzaGFvQHpqdS5lZHUuY24=
Disclaimer: All claims expressed in this article are solely those of the authors and do not necessarily represent those of their affiliated organizations, or those of the publisher, the editors and the reviewers. Any product that may be evaluated in this article or claim that may be made by its manufacturer is not guaranteed or endorsed by the publisher.
Research integrity at Frontiers
Learn more about the work of our research integrity team to safeguard the quality of each article we publish.