- 1School of Agriculture, Ningxia University, Yinchuan, Ningxia, China
- 2Department of Laboratory Medicine, General Hospital of Ningxia Medical University, Ningxia Medical University, Yinchuan, China
Exosomes are a kind of extracellular vesicles that are produced and secreted by different mammalian cells. They serve as cargo proteins and can transfer different kinds of biomolecules, including proteins, lipids, and nucleic acids, which consequently act on target cells to exert different biological effects. Recent years have witnessed a significant increase in the number of studies on exosomes due to the potential effects of exosomes in the diagnosis and treatment of cancers, neurodegenerative diseases, and immune disorders. Previous studies have demonstrated that exosomal contents, especially miRNAs, are implicated in numerous physiological processes such as reproduction, and are crucial regulators of mammalian reproduction and pregnancy-related diseases. Here, we describe the origin, composition, and intercellular communication of exosomes, and discuss their functions in follicular development, early embryonic development, embryonic implantation, male reproduction and development of pregnancy-related diseases in humans and animals. We believe this study will provide a foundation for revealing the mechanism of exosomes in regulating mammalian reproduction, and providing new approaches and ideas for the diagnosis and treatment of pregnancy-related diseases.
1 Introduction
Extracellular vesicles (EVs) that are released by different kinds of cells, mediate cell communication between tissues and organs. EVs can be classified into microvesicles, apoptotic vesicles, and exosomes according to their characteristics and functions. Exosomes, a special subtype of extracellular vesicles with a diameter of approximately 30 nm–150 nm (Jeppesen et al., 2019), function as carriers of information molecules for intercellular communication. In addition, they participate in different physiological and pathological responses (Farooqi et al., 2018).
Exosomes were first identified in sheep reticulocytes, and little was known about their functions (Johnstone et al., 1987). Substantial research in recent years has revealed the functions and significance of exosomes in physiological and pathological processes. For instance, Valadi et al. (2007) found that nucleic acid substances, such as mRNA and miRNA in the exosomes of mouse mast cells, can regulate the biological functions of other cells. Similarly, other studies have demonstrated that exosomes carrying miRNAs, IncRNAs, and circRNAs, can be transported to specific tissues and organs via body fluids and play an important role in regulating physiological functions (Zheng et al., 2020). In addition, exosomes participate in several pathological processes, such as tumorigenesis, the development of neurodegenerative diseases, and immune disorders.
2 Mammalian exosomes: Their origin and composition
Almost all cells, including epithelial cells, macrophages, mast cells, neuronal cells, and mesenchymal cells, can secrete exosomes. Furthermore, immune cells can secrete exosomes, which play an important role in the immune response during tumorigenesis by influencing the proliferation and activity of receptor cells (Homer et al., 2017; Chung et al., 2020). Moreover, exosomes have been identified from body fluids, such as cerebrospinal fluid, saliva, serum, breast milk, and urine (Nguyen et al., 2016).
Exosomes have a complicated biological composition, with several of them containing distinct components and different species possessing different exosomes. Exosomes primarily contain proteins, lipids, and nucleic acids (Figure 1). The majority of exosomal proteins are essential proteins although certain unique proteins produced during exosome formation are also present. Exosomes contain tetra-transmembrane proteins, namely, CD9, CD63, CD81, and CD82, which are now considered to be the marker proteins of exosomes (Pluchino and Smith, 2019). Furthermore, exosomes are enriched with endonuclear somatic sorting complex proteins (ALIX and TSG101), heat shock proteins (HSP70 and HSP90), and certain special proteins responsible for the formation of extracellular vesicles and their release, such as RAB27A, RAB11B, and ARF6 (Kurian and Modi, 2019). Moreover, exosomes are rich in glycoproteins and transmembrane proteins, including EGFR, MHC I, MHC II, and other transmembrane proteins (Zaborowski et al., 2015).
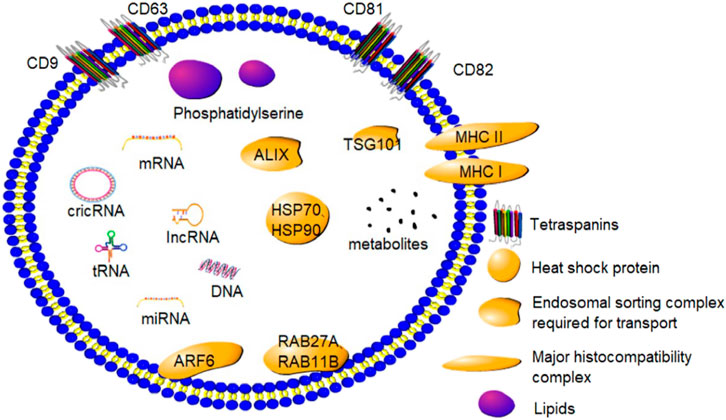
FIGURE 1. Composition and marker proteins of exosomes: Exosomes are small phospholipid bilayer vesicles that contain a variety of proteins, lipids, and nucleic acids. CD9, CD63, CD81, and CD82 are marker proteins of exosomes.
Exosomes are a rich source of different kinds of lipids. The exosome membrane is largely composed of sphingolipids, gangliosides, and unsaturated lipids, with a low content of phosphatidylcholines and diglycerides. In addition, ganglioside-rich GM3 and ceramide derivatives such as phosphatidylserine have been found in the exosomes (Llorente et al., 2013). The composition of exosomes changes in response to changes in the extracellular environment (Kalluri and LeBleu, 2020).
Since the discovery of mRNAs and miRNAs in the exosomes, different kinds of exosomal biomolecules have been identified. Several studies have demonstrated that exosomes contain different kinds of small RNAs, including ribosomal 18 S and 28 S subunit rRNAs and certain tRNAs. In a study, rRNA was found to be a major biomolecule of exosomes secreted by human mammary cell lines (Jenjaroenpun et al., 2013). In addition, lncRNA and circRNA have been located in the exosomes (Abels and Breakefield, 2016). These exosomal biomolecules can bind to target cells to govern several key physiological functions in animals.
3 Exosome formation and intercellular communication
Exosomes are synthesized by the double invagination of the protoplasmic membrane and the inward budding of the luminal membrane of intracellular multivesicular bodies (MVBs) containing intraluminal vesicles (ILVs, Figure 2). The initial invagination of the plasma membrane leads to the formation of early sorting endosomes, including cellular proteins, lipids, metabolites, small molecules, and ions (Urbanelli et al., 2013). Subsequently, the second invagination allows early sorted endosomes to mature into late sorted endosomes, eventually producing MVBs containing ILVs. The formation of exosomes is largely controlled by two mechanisms, namely, the ESCRT-dependent mechanism and the ESCRT-independent mechanism (van Niel et al., 2018). ESCRT-dependent formation of MVBs is a mono-ubiquitination modification of membrane proteins and occurs by the addition of ubiquitin molecules to lysine residues recognized by ESCRT proteins and ESCRT-related proteins, including ALIX, TSG101, Chmp4, and SKD1. These proteins bind to the ubiquitinated vesicles to form MVBs. In contrast, the ESCRT-independent formation of MVBs is facilitated by neutral sphingomyelinase (nSMase), which promotes membrane invagination of ILVs and the formation of MVBs. Finally, MVBs merge with lysosomes and autophagosomes to degrade biomolecules or merge with the plasma membrane to release the exosomes (Abels and Breakefield, 2016; Jeppesen et al., 2019).
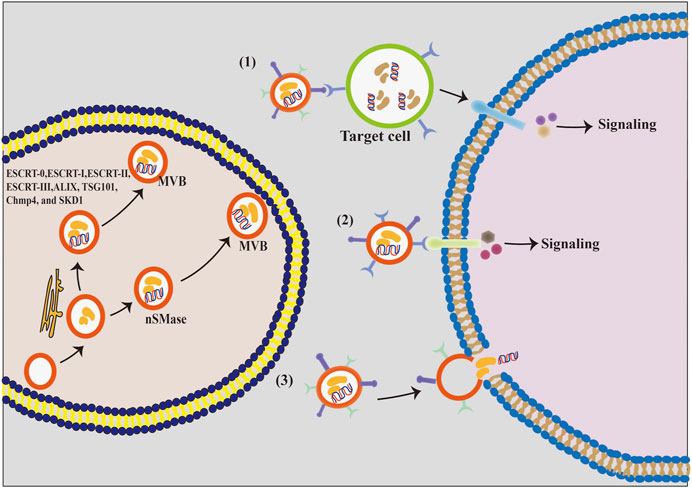
FIGURE 2. The formation process and the intercellular communication mode of exosomes. (1) Exosomal membrane protein first binds to the cellular receptor, and then acts on its target cells and activates the intracellular signaling cascades. (2) Exosomal membrane proteins bind directly to the target cell’s receptors and activate their intracellular signaling pathways. (3) Exosomal membrane directly fuses with the phospholipid bilayer of the target cell, and exosomal components, including proteins, lipids, nucleic acids, and other substances, are released into the cytoplasm of the target cell in a non-selective manner.
Exosomes play a crucial ‘postman’ function in the extracellular environment by efficiently delivering the information molecules to certain target cells, thereby regulating the activities of target cells (Carp et al., 2004; Cui et al., 2012). It is currently believed that exosomes communicate with cells in three ways (Figure 2). First, exosome vesicles adhere to the surface of the recipient cells by interacting with the lipid or ligand–receptor, subsequently activating numerous signaling pathways of the effector cell. Second, exosomes can directly trigger the target cells and activate the related signaling pathways. Third, exosomes release biomolecules into the cytoplasm of target cells after fusing with their plasma membranes, thereby activating these cells (Wei et al., 2021). Therefore, exosomes can regulate several physiological functions in animals through different communication ways.
4 Exosomes regulate mammalian reproduction
Exosomal biomolecules, including non-coding RNAs, hormones, and proteins, have been implicated in regulating human and animal reproduction. Exosomes can transport RNAs, proteins, enzymes, and lipids, thereby affecting multiple physiological and pathological processes in several diseases, including cancer, neurodegenerative diseases, infections, and autoimmune diseases. Exosomal bioinformatic molecules of different origins can regulate their physiological functions by acting on the corresponding target tissues. Exosomes in the follicular fluid carrying miRNA, mRNA, and proteins can communicate bidirectionally with granulosa cells and oocytes to regulate granulosa cell proliferation, steroid production, and oocyte maturation (Collado-Fernandez et al., 2012; Yuan C et al., 2021). Besides, exosomes are released from the uterus, oviduct epithelium, endometrium, and preimplantation embryos and are known to regulate follicle development, embryo development, and implantation (Machtinger et al., 2016; Qamar et al., 2020). Endometrium can regulate the process of embryo attachment by secreting exosomes into the uterine cavity fluid, the embryo itself can also secrete exosomes during embryo implantation. Exosomes secreted by the embryo have been reported to alter the morphology of the endometrium and its gene expression profile, thereby regulating embryo positioning and invasion for embryo implantation (Kusama et al., 2018). Furthermore, exosomal miRNAs and proteins in the placenta are known to regulate the inflammatory response and trophoblast invasion (Gurunathan et al., 2022). Exosomes derived from embryonic and uterine mucosa can mediate immune tolerance through mutual communication with the mother, facilitating embryo implantation and subsequent pregnancy (Wu Y et al., 2022). Recent studies have demonstrated that intrauterine EV-derived bta-miR-26b and miR-98 downregulate the maternal immune system, which contributes to the implantation of the conceptus (Nakamura et al., 2019; Nakamura et al., 2021). Exosomal biomolecules in the reproductive system mediate bidirectional communication between cells and tissues and regulate different physiological events. Of these, exosomes regulating follicular and embryonic development and embryo implantation have attracted the highest attention of researchers. In addition, extensive evidence has revealed the role of exosomes in the male genital tract. Recently, exosomes derived from testicular cells not only regulate normal spermatogenesis and steroidogenesis in the testis but can also be used as a diagnostic marker for male infertility (Ma et al., 2022).
4.1 Exosomes regulate follicles and early embryonic development
Granulosa cells are essential for follicular development. Follicular exosomal biomolecules are known to regulate granulosa cell steroid production, proliferation, and apoptosis. Human umbilical cord MSC-derived EVs promote estrogen production of granulosa cells (Cai et al., 2022). Another study involving patients with polycystic ovarian syndrome (PCOS) reported that the deletion of exosomal circLDLR in receptor cells enhanced the expression of miR-1294 and inhibited that of CYP19A1, which consequently inhibited estradiol secretion and ultimately resulted in aberrant follicular growth (Huang et al., 2020). In contrast, Yao et al. (2010) reported that exosomal miR-224 stimulated estradiol secretion and promoted follicular growth by increasing the expression of CYP19A1 mRNAs. This suggests that different exosomal biomolecules exert varying regulatory effects on the same target tissue. In addition, exosomal miRNAs can affect follicular development by regulating the proliferation and apoptosis of ovarian granulosa cells. Bovine follicular fluid-derived EVs promote the proliferation of granulosa cells (Andrade et al., 2017). However, miR-424 in follicular fluid EVs from patients with PCOS inhibits granule proliferation by targeting CDCA4 to inhibit the Rb/E2F1 signaling (Yuan D et al., 2021). In addition, granulosa apoptosis can cause follicular developmental arrest and atresia. Xu et al. (2017) demonstrated that exosomal miR-145 in follicular fluid protected mouse granulosa cells from oxidative stress-induced apoptosis and suppressed aberrant follicular development by targeting the KLF4 receptor. Another study reported that miR-92a in follicular fluid exosomes targets SMAD7 to inhibit the apoptosis of porcine granulosa cells and maintain follicular development. MiR-23a and miR-27a from follicular fluid exosomes can target SMAD5 to promote the apoptosis of human ovarian granulosa cells via the FasL–Fas signaling pathway, ultimately causing follicular atresia (Nie et al., 2015). This suggests that different exosomal molecules in follicular fluid carrying different communication signals can influence follicular development by regulating granulosa cell functions.
In addition, exosomes have been implicated in the development of oocytes. It has been previously demonstrated that bovine follicular exosomes can enhance oocyte development by promoting the proliferation of cumulus cells (Hung et al., 2015). Yang et al. (2020) claimed that miR-146a-5p or miR-21–5p carried by exosomes isolated from human umbilical cord mesenchymal stem cells promoted oocyte development and improved the number and quality of mouse oocytes via the PI3K/mTOR signaling pathway. Similarly, exosomal miR-17- and miR-92a-derived follicular fluid increased the oocyte diameter and H4K12 acetylation levels (Inoue et al., 2020). On the contrary, miR-145 suppressed oocyte development by reducing the levels of neurotrophic factors (Xu et al., 2017). Subsequently, increased exosomal miRNAs regulating the oocyte development were identified using bioinformatic approaches. Hu et al. (2020a) identified miR-125b, let7d-5p, miR-200b, miR-26a, and miR-92a in porcine follicular fluid exosomes by next-generation sequencing, and reported that they could be involved in the regulation of the maturation of the nucleus and cytoplasm of pig oocytes via the TGF signaling pathway. Thus, during the development of the oocyte, exosomes in the follicle carry several information molecules into different cells to coordinate the communications among cells, thereby contributing to the growth and maturation of the oocyte.
Furthermore, follicular fluid exosomes can promote zygote division and blastocyst development. Exosomes have been found to promote the proliferation of cumulus cells and the development of the embryo as well as reduce the side effects of heat shock on blastocyst cleavage and development (Rodrigues et al., 2019). Furthermore, exosomal miRNA-21 is known to modulate the growth and development of the zygote and suppress embryonic death (Lv et al., 2018). Supplementation of EVs with small follicles (3–6 mm) during the development of embryos in vitro culture altered the levels of embryonic transcripts associated with epigenetic modifications and embryonic development, and altered the overall DNA methylation and hydroxymethylation levels, thereby increasing blastocyst rates (Da et al., 2017). This suggests that follicular fluid-derived EVs contribute to early embryonic development. Although exosomal biomolecules are known to regulate follicular and embryonic development, the exact underlying molecular mechanism is still poorly understood.
4.2 Exosomes regulate the process of mammalian embryo implantation
The exchange of information between the endometrium and the embryo is indispensable for successful embryo implantation (Salomon et al., 2016). For instance, exosomal proteins are necessary for mutual communication between the embryo and the endometrium during the early stages of pregnancy, facilitating embryo implantation. Exosomes derived from endometrial epithelial cells are taken up by embryonic trophoblast cells during embryo implantation and secrete specific proteins, thereby enhancing the adhesion of trophoblast cells to the endometrium and promoting embryo implantation. Exosomal miRNAs isolated from the supernatant of cultured porcine endothelial cell lines have been reported to regulate the function of trophoblast cells (Bidarimath and Tayade, 2017). Moreover, in pigs, recent studies have demonstrated that miR-92b-3p, an exosome derived from porcine endometrial epithelial cells, is engulfed by porcine trophectoderm cells and regulates the proliferation, migration, and adhesion of trophoblast cells by targeting TSC1 and DKK3 (Hua et al., 2022). Another study reported that Hsa-miR-30d, an exosomal message molecule released from human endometrial cells, is engulfed by mouse embryos to regulate embryo implantation (Vilella et al., 2015). In addition, other miRNAs in the exosomes can increase the adhesion of endometrial epithelial cells and the invasiveness of blastocyst trophectoderm during embryo implantation (Gurung et al., 2020). This suggests that the regulatory function of homologous exosomal informative molecules is conserved between species. Not only are exosomal miRNAs important informative molecules that regulate embryo implantation but exosomal proteins also play an important role in embryo implantation. Osteopontin, also known as secreted phosphoprotein 1 (SPP1), is an extracellular matrix protein that binds to integrin receptors on porcine and sheep trophectoderm cells (Johnson et al., 2014). SPP1 has been reported to mediate trophoblast adhesion in the endometrium by activating the mTOR, PI3K, MAPK3/MAPK1, and MAPK14 signaling pathways. Furthermore, exosomal proteins from trophoblast cells are involved in maternal–fetal recognition during bovine pregnancy. In addition, the variations in exosomal proteins regulate endometrial receptivity (Su et al., 2021). Certain proteins can regulate embryo implantation in the form of both secreted proteins and exosomal informative molecules. Interferon-tau (IFNT) is known to be the main trophoblast factor acts on the endometrium, inhibiting luteolysis and maintaining progesterone secretion. Imakawa et al. (2017) reported that endogenous retroviruses in maternal exosomes promote the growth of trophoblast ectodermal cells and release IFNT via binding to Toll-like receptors, thus inducing the expression of adhesion molecules and interferon-stimulated genes during early sheep gestation. Subsequently; Kusama et al. (2018) showed that IFNT is not only a secretory protein stimulated by exosomes but also an exosomal component in the endometrium of pregnant ruminants; Salomon et al. (2017) reported that trophoblast cell-derived exosomes promote extrachorionic trophoblast infiltration and embryonic implantation by activating the expression of matrix metalloproteinases (MMPs) and protein kinase MAPK signaling pathways. Endometrium-derived exosomes MMP-14 could degrade and remodel the extracellular matrix in the embryo–endometrium and promote embryo implantation (Latifi et al., 2018). Proteins and miRNAs contained in different sources of exosomes play an important role in the interaction between blastocyst and endometrium during embryo implantation (Figure 3). These findings suggest that biomolecules carried by exosomes promote the adhesion and implantation of embryos; however, the exact regulatory mechanisms warrant further investigations.
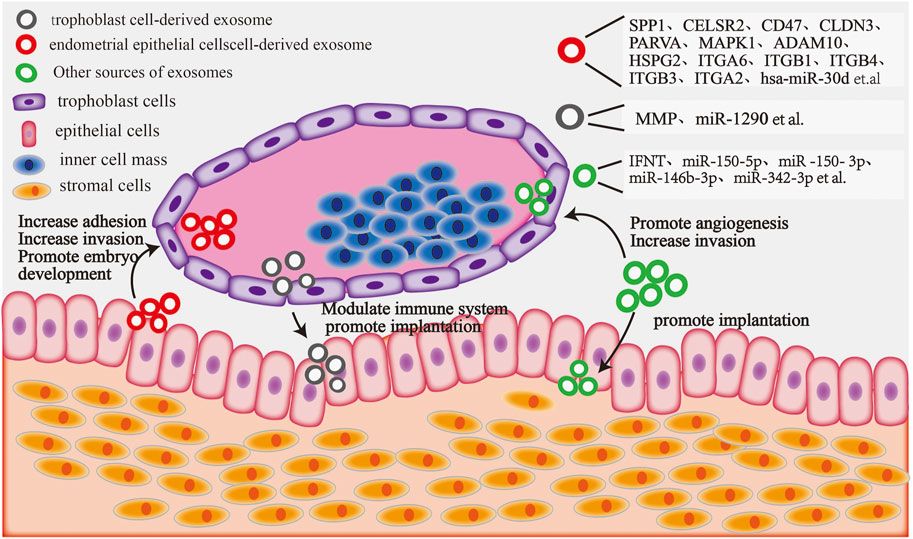
FIGURE 3. The blastocyst and endometrium interact with exosome components: endometrial epithelial cell-derived exosomal proteins and miRNAs can target trophoblast cells, increasing their adhesion and invasion and promoting embryo development. Embryonic trophoblast cell-derived exosomal proteins and miRNAs can regulate the maternal immune system and increase embryo attachment by targeting endometrial epithelial cells. Moreover, exosomal components derived from other sources (including plasma, serum, and follicular fluid) can target trophoblast cells to facilitate invasion and angiogenesis, whereas others can target endometrial epithelial cells to promote embryonic implantation.
In addition, exosome-derived biomolecules can be employed as novel indicators to evaluate endometrial receptivity and embryo implantation in mammals. Bioinformatic analysis revealed that 348 endometrial exosomal miRNAs were identified as possible biomarkers of endometrial receptivity and fertility in mammals (Altmae et al., 2017). Similarly, Zhou et al. (2020) stated that exosome-specific miRNAs in peripheral blood are potential tools for determining embryonic implantation. Furthermore, Zeng et al. (2021) reported that miR-150–5p, miR-150–3p, miR-146b-3p, and miR-342–3p in plasma exosomes are involved in embryo implantation, whereas miR-146b-3p and miR-150–5p serve as diagnosis targets of embryo implantation. Moreover, exosomal miR-17 and miR-20a, belonging to the miR-17/92 family can exchange trophoblast invasion and embryo implantation by targeting TGF-receptor II, Smad2, and Smad4 (Mogilyansky and Rigoutsos, 2013). The trophoblast-derived exosomal miR-1290 can suppress the expression of LHX6 and promote the epithelial–mesenchymal transition, thereby enhancing endometrial receptivity (Shi et al., 2021). In all, the miR-17/92 cluster and miR-1290 are potential novel biomarkers for evaluating endometrial receptivity and embryo implantation. However, further studies are required to assess endometrial tolerance and embryo implantation using exosomal informative molecules.
4.3 Exosomes regulate male reproduction
In addition to regulating female reproduction, the role of exosomes in male reproduction has recently received increasing attention. Some studies have demonstrated that exosomes derived from male genital tract including Sertoli cell, epididymis and prostate are involved in germ cell development, sperm maturation and fertilization outcomes (Baskaran et al., 2020). Recent study showed that exosomal miR-486–5p derived from Sertoli cell acts as a communication molecule between Sertoli cells and spermatogonial stem cell (SSCs), modulating differentiation of SSCs through binding PTEN in mice (Li et al., 2021). Further study also demonstrated that exosomes released Sertoli cells may cross the blood-testis barrier and promote the survival of Leydig cells (Ma et al., 2022). It is well known that seminal plasma is essential for the maturation of spermatozoa. Meanwhile, exosomal biomolecules containing protein and miRNA in seminal plasma regulate a range of biological processes involving maturation of spermatozoa in the epididymis. A study has indicated that seminal exosomes could promote sperm capacitation through inducing acrosome reaction (Murdica et al., 2019a). Yang et al. (2017) identified 1,474 proteins in male seminal plasma exosomes, and found that these proteins were mainly involved in biologic processes, including metabolism, energy pathways, protein metabolism, cell growth and maintenance. Another study also found a total of 2,138 proteins isolated from seminal plasma of normozoospermic (NSP) and severe asthenozoospermic (SA) men by comprehensive proteomic analysis, of which 37 miRNAs were increased in the NSP group and 52 miRNAs were increased in the SA group, supporting the evidence that the network of seminal exosomes proteins may be connected to the molecular mechanisms involved in sperm maturation and motility (Murdica et al., 2019b). In animals, boar seminal plasma exosomes were essential in maintaining sperm motility, sperm membrane integrity, antioxidant capacities and inhibition of premature capacitation (Du J et al., 2016). In addition to various exosomal proteins, non-coding RNAs, including microRNA, Y RNA and tRNA derived from seminal plasma are able to modulate sperm function. Sperm cytoplasmic droplets (CDs) are remnants of cytoplasm, and their migration is a morphological characteristic of epididymal maturation. Sun et al.(2021) identified total of 348 known and 206 new miRNAs in boar seminal plasma exosomes isolated from semen containing spermatozoa with CDs, of which 13 miRNA were significantly upregulated, whereas three miRNAs were significantly downregulated, suggesting that seminal plasma exosomes might play a key role in the regulation of sperm CDs. Currently, semen exosomes are also able to play an important role in male mammalian reproduction as biomarker candidates for the identification of patients with azoospermia, hypospermia and teratozoospermia or other male infertility (Candenas and Chinease, 2020). Furthermore, extracellular adenosine triphosphate (exATP) produced by boar seminal plasma exosomes may control sperm motility by regulating mitochondrial metabolism (Guo et al., 2019). Further study indicated that fresh boar sperm incubated with exATP significantly increased sperm motility and reduced apoptotic rate (Guo et al., 2019). Most recently, using seminal plasma exosomes isolated from the seminal plasma of a bull of proven fertility could also improve the fertilizing capacity of male gametes of low-fertility bulls (Lange-Consiglio et al., 2022). These suggest that exosomes have some potential improving male reproduction. However, isolation and purification of exosome derived from male genital tract still presents a substantial hurdle (Goss et al., 2023).
5 Exosomes role in the diagnosis and treatment of pregnancy-related diseases
5.1 Exosomes in diagnosing pregnancy-related diseases
Although exosomes are required for mammalian pregnancy, variations in exosome contents can induce several pregnancy-related diseases. It has been recently reported that the abnormal expression of exosomal proteins and non-coding RNAs is related to pregnancy-related diseases. For instance, patients with preeclampsia have considerably higher levels of miR-136, miR-494, and miR-495 in their peripheral blood exosomes compared to those in 20 weeks of pregnancy peripheral blood, indicating that these miRNAs could serve as biomarkers for early diagnosis of preeclampsia (Motawi et al., 2018). Two clinical studies demonstrated that miR-21 and miR-200 families (miR-200a, miR-200b, and miR-200c) in female serum exosomes can serve as human ovarian cancer biomarkers (Mahmoud et al., 2018). Zhang et al. (2020a) reported that the expression of exosomal miR-22–3p and miR-320a significantly increased in patients with endometriosis, and speculated that these miRNAs can be used as potential biomarkers for the diagnosis of endometriosis. Another study reported that exosomal proteins extracted from the peritoneal fluid of endometriosis patients, such as PRDX1, H2A type 2-C, ANXA2, and ITIH4 and tubulin α-chain, which are absent in the peritoneal fluid of healthy women, could be used as biomarkers to identify endometriosis (Nazri et al., 2020). Furthermore, differential expression analysis of serum exosomes revealed relatively higher miR-27a-5p levels in PCOS patients than in normal women (Che et al., 2020). Therefore, miR-27a-5p levels of circulating exosomes could serve as promising biomarkers for PCOS. Another frequent pregnancy problem is pregnancy loss. A comparison of the differences in blood exosomes between women with normal pregnancy and those with recurrent pregnancy loss revealed that the expression of exosome marker CD9 significantly increased in women with recurrent pregnancy loss at the sixth week of pregnancy (Rajaratnam et al., 2021). Similar to studies on humans, certain exosomal miRNAs can be employed as markers to identify early pregnancy loss in cows. For instance, the expression of miR-25, miR-16b, and miR-3596 carried serum exosomes was higher in early aborting cows, indicating that exosomal miRNAs could trigger luteum regression and cause pregnancy loss (Pohler et al., 2017). Similarly, De Bem et al. (2017) reported reduced levels of 27 miRNAs in peripheral blood from cattle with early embryonic loss. Therefore, these exosomal miRNAs could be used as diagnostic markers for early pregnancy loss in humans and animals. In addition, other exosomal components could be valuable biological targets for the diagnosis of pregnancy-associated abnormalities in humans and animals (Table 1).
5.2 Exosomes in the treatment of pregnancy-related diseases
An increasing number of studies have demonstrated the potential of exosomes in treating pregnancy-related diseases. The exosome-delivered miR-323–3p (exosomes of adipose mesenchymal stem cells from PCOS patients) promoted the proliferation and inhibited apoptosis in CCs by targeting PDCD4, indicating its potential role in the treatment of PCOS (Vojtech et al., 2014; Zhao et al., 2019). Moreover, circLDLR in the follicular fluid exosomes reduced the formation of aberrant follicles in PCOS by targeting miR-1294, suggesting circLDLR is a novel target for future PCOS treatment (Huang et al., 2020). Another study demonstrated that exosomal miR-424–5p exerted a therapeutic impact on PCOS patients via inhibiting granulosa cell proliferation and inducing PCOS cell senescence (Yuan C et al., 2021). Subsequently, different exosomal miRNAs have been demonstrated to be effective in preventing and treating PCOS. Adipose mesenchymal stem cell-derived exosomal miRNA-21–5p increased the number of corpus luteum and reduced the number of abnormal cystic follicles by targeting Btg2 and activating the IRS1 and AKT signaling pathways in PCOS rats (Cao et al., 2022). In addition, Zhou et al. (2022) demonstrated that the overexpression of miR-18b-5p derived from follicular fluid reduced the apoptosis of granulosa cells and attenuated the pathological effects in ovaries by targeting PTEN and activating the PI3K/Akt/mTOR signaling pathway in PCOS rats.
Endometriosis is a common disease in human and animal pregnancy. Exosomal components are involved in regulating the development of endometriosis. For example, Liu et al. (2021) found that the expression of lncRNA CHL1-AS1 promoted the proliferation, migration, and invasion of endometrial stromal cells and inhibited cell apoptosis, thereby causing endometriosis in the mouse. Another study reported the involvement of several exosomal miRNAs in the development of endometriosis by reducing the expression of HOXA10 and LIF in women (Zhou and Dmitridis, 2020). Moreover, endometriosis usually causes tissue fibrosis. Exosomal miR-214–3p inhibited the development of endometriosis fibrosis by directly targeting connective tissue growth factors (Zhang et al., 2021; Li et al., 2022). Furthermore, exosomal miR-30c from endometrial epithelial cells inhibited the invasion and migration of ectodermal endometrial epithelial cells by counter-regulating the expression of BCL9, implying miR-30c to be a potential therapeutic target in endometriosis (Zhang et al., 2022). These findings suggest that exosomal biomolecules regulate endometriosis, and these could be used to design a novel treatment method for endometriosis.
Pregnancy loss can occur due to a disturbance in between the anti-inflammatory and pro-inflammatory states at the maternal–fetal interface. The placenta-derived exosome bta-miR-499 inhibited LPS-induced inflammation in cultured bovine endometrial epithelial cells, and lower levels of mmu-miR-499 have been shown to increase the risk of bovine pregnancy loss in vivo (Zhao et al., 2018). Therefore, miR-499 can serve as a potential therapeutic target for pregnancy loss caused by uterine inflammation. Another study reported that peritoneal injection of exosomal granulocyte–colony-stimulating factor derived from trophoblast cells significantly reduced the rate of miscarriage in mice, suggesting it could be a novel therapeutic regimen for pregnancy loss (Gao et al., 2022). Furthermore, injecting mesenchymal stem cells-derived exosomes into the uterine horn of mice downregulated the levels of IFN-γ and TNF-α, and upregulated the levels of IL-10 and IL-4, suggesting its potential role in reducing embryonic resorption and maintaining normal pregnancy (Xiang et al., 2020). Therefore, specific tissue-derived exosomes can be used for the management of pregnancy loss. However, we need to identify more exosomal molecules and further investigate their potential mechanisms of action.
In addition, exosomes derived from different tissues can be used to treat other pregnancy-related diseases. A study on patients with endometrial cancer reported that exosome efficiently transported miR-148b to endometrial cancer cells via fibroblasts, where the miRNA inhibited endometrial cancer metastasis by directly binding to its target gene DNMT1 (Li Q et al., 2019). Wang et al. (2021) reported that down-regulating exosomal miR-218 expression in endometrial epithelial cells of cows with endometritis impaired embryonic development and reduced trophoblast migration by inhibiting the WNT signaling pathway. Consequently, an injection of exosomal miR-218 reduced endometritis-induced infertility in cows. Other exosomes have been reported to be involved in the development and treatment of pregnancy-related diseases in both humans and animals as shown in Table 2. Although exosomes regulate the occurrence of mammalian diseases and have shown potential value in treating pregnancy-related diseases, further studies are required to completely understand the underlying mechanisms.
6 Conclusions and perspectives
Although exosomes can be used as potential markers for the early diagnosis of diseases, early pregnancy, and miscarriage detection, their extraction is highly time-consuming and expensive. Therefore, a simple, rapid, and inexpensive method is required to efficiently extract exosomes in the future. In addition, exosomal components are complex, and current research is focused on miRNAs and proteins. However, research on other exosomal components, such as mRNAs and lipids, is very limited and warrants further investigation. Exosomes can cross biological barriers and transport numerous proteins and gene-based drugs into target cells. Certain aspects that limit their use as drug carriers are as follows: First, exosomes cannot be stored indefinitely, and the present protective measures could alter exosome morphology and impair their carrier function. Thus, future studies should concentrate on developing more effective preservation approaches to preserve exosome’s biological activity. Second, the targeting ability of natural exosomes is poor and they are easily cleared in vivo. This problem can be overcome using genetic engineering techniques or chemical linking of targeting peptides. Third, the majority of studies on exosomes are in vitro due to the lack of suitable animal models. Therefore, establishing suitable animal models of specific diseases will help conduct further studies on exosomes. Fourthly, immature techniques for the isolation and purification of exosome have severely limited its clinical use. Yet, it is encouraging that microfluidic technology has the potential to simplify and improve exosome isolation and detection.
Altogether, this review highlights the biological functions of exosomes in different physiological events associated with reproduction and pregnancy-related diseases. The composition, origin, and communication of exosomes have been described in detail. Numerous studies have demonstrated that exosomes carrying functional molecules can regulate different physiological events such as follicle development, oocyte maturation, granulosa cell function, and embryo implantation. In addition, exosomal informative molecules are involved in regulating the development of pregnancy-related diseases, some of which can be used as new biological targets for the diagnosis of pregnancy-related diseases. Exosomes delivering specific target drugs have demonstrated potential clinical applications in the treatment of pregnancy-related diseases. Although certain shortcomings in exosome research cannot be effectively solved at present, it is believed that the research value of exosomes in regulating reproduction and the diagnosis and treatment of mammalian pregnancy-related diseases will increase tremendously with the development of research technologies.
Author contributions
X-RG reviewed the literature and wrote the initial draft. Y-KC and X-GD critically reviewed, revised the manuscript and contributed to the writing of the manuscript. YM, Z-MM, T-SD, and S-HW proposed a modification. All authors have approved the manuscript and agreed to the published version of the manuscript.
Funding
This work was supported by Ningxia Provincial Key Science and Technology Project (2021BBF02037, 2022BBF03023) and Ningxia Natural Science Foundation (2022AAC03057).
Conflict of interest
The authors declare that the research was conducted in the absence of any commercial or financial relationships that could be construed as a potential conflict of interest.
Publisher’s note
All claims expressed in this article are solely those of the authors and do not necessarily represent those of their affiliated organizations, or those of the publisher, the editors and the reviewers. Any product that may be evaluated in this article, or claim that may be made by its manufacturer, is not guaranteed or endorsed by the publisher.
References
Abels, E. R., and Breakefield, X. O. (2016). Introduction to extracellular vesicles: Biogenesis, RNA cargo selection, content, release, and uptake. Cell. Mol. Neurobiol. 36(3), 301–312. doi:10.1007/s10571-016-0366-z
Altmae, S., Koel, M., Vosa, U., Adler, P., Suhorutsenko, M., Laisk-Podar, T., et al. (2017). Meta-signature of human endometrial receptivity: A meta-analysis and validation study of transcriptomic biomarkers. Sci. Rep. 7(1), 10077. doi:10.1038/s41598-017-10098-3
Andrade, G. M., Meirelles, F. V., Perecin, F., and Da, S. J. (2017). Cellular and extracellular vesicular origins of miRNAs within the bovine ovarian follicle. Reprod. Domest. Anim. 52(6), 1036–1045. doi:10.1111/rda.13021
Baskaran, S., Panner, S. M., and Agarwal, A. (2020). Exosomes of male reproduction. Adv. Clin. Chem. 95, 149–163. doi:10.1016/bs.acc.2019.08.004
Bidarimath, M., and Tayade, C. (2017). Pregnancy and spontaneous fetal loss: A pig perspective. Mol. Reprod. Dev. 84(9), 856–869. doi:10.1002/mrd.22847
Cai, J. H., Sun, Y. T., and Bao, S. (2022). HucMSCs-exosomes containing miR-21 promoted estrogen production in ovarian granulosa cells via LATS1-mediated phosphorylation of LOXL2 and YAP. Gen. Comp. Endocrinol. 321-322, 114015. doi:10.1016/j.ygcen.2022.114015
Candenas, L., and Chianese, R. (2020). Exosome composition and seminal plasma proteome: A promising source of biomarkers of male infertility. Int. J. Mol. Sci. 21, 7022(19). doi:10.3390/ijms21197022
Cao, M., Zhao, Y., Chen, T., Zhao, Z., Zhang, B., Yuan, C., et al. (2022). Adipose mesenchymal stem cell-derived exosomal microRNAs ameliorate polycystic ovary syndrome by protecting against metabolic disturbances. Biomaterials, 288, 121739. doi:10.1016/j.biomaterials.2022.121739
Carp, H., Dardik, R., Lubetsky, A., Salomon, O., Eskaraev, R., Rosenthal, E., et al. (2004). Prevalence of circulating procoagulant microparticles in women with recurrent miscarriage: A case-controlled study. Hum. Reprod. 19(1), 191–195. doi:10.1093/humrep/deg512
Che, X., Jian, F., Chen, C., Liu, C., Liu, G., and Feng, W. (2020). PCOS serum-derived exosomal miR-27a-5p stimulates endometrial cancer cells migration and invasion. J. Mol. Endocrinol. 64(1), 1–12. doi:10.1530/JME-19-0159
Chung, I. M., Rajakumar, G., Venkidasamy, B., Subramanian, U., and Thiruvengadam, M. (2020). Exosomes: Current use and future applications. Clin. Chim. Acta. 500, 226–232. doi:10.1016/j.cca.2019.10.022
Collado-Fernandez, E., Picton, H. M., and Dumollard, R. (2012). Metabolism throughout follicle and oocyte development in mammals. Int. J. Dev. Biol. 56(10-12), 799–808. doi:10.1387/ijdb.120140ec
Cui, Y., Wang, W., Dong, N., Lou, J., Srinivasan, D. K., Cheng, W., et al. (2012). Role of corin in trophoblast invasion and uterine spiral artery remodelling in pregnancy. Nature. 484(7393), 246–250. doi:10.1038/nature10897
Da, S. J., Andrade, G. M., Del, C. M., Sampaio, R. V., Sangalli, J. R., Silva, L. A., et al. (2017). Supplementation with small-extracellular vesicles from ovarian follicular fluid during in vitro production modulates bovine embryo development. PLoS One. 12(6), e0179451. doi:10.1371/journal.pone.0179451
De Bem, T., Da, S. J., Sampaio, R. V., Sangalli, J. R., Oliveira, M., Ferreira, R. M., et al. (2017). Low levels of exosomal-miRNAs in maternal blood are associated with early pregnancy loss in cloned cattle. Sci. Rep. 7(1), 14319. doi:10.1038/s41598-017-14616-1
Du, J., Shen, J., Wang, Y., Pan, C., Pang, W., Diao, H., et al. (2016). Boar seminal plasma exosomes maintain sperm function by infiltrating into the sperm membrane. Oncotarget. 7(37), 58832–58847. doi:10.18632/oncotarget.11315
Farooqi, A. A., Desai, N. N., Qureshi, M. Z., Librelotto, D., Gasparri, M. L., Bishayee, A., et al. (2018). Exosome biogenesis, bioactivities and functions as new delivery systems of natural compounds. Biotechnol. Adv. 36(1), 328–334. doi:10.1016/j.biotechadv.2017.12.010
Gao, P., Zha, Y., Wei, L., Zhou, X., Zhu, S., Zhang, H., et al. (2022). G-CSF: A vehicle for communication between trophoblasts and macrophages which may cause problems in recurrent spontaneous abortion. Placenta. 121, 164–172. doi:10.1016/j.placenta.2022.03.125
Gong, R. Q., Nuh, A. M., Cao, H. S., and Ma, M. (2021). Roles of exosomes-derived lncRNAs in preeclampsia. Eur. J. Obstet. Gynecol. Reprod. Biol. 263, 132–138. doi:10.1016/j.ejogrb.2021.06.015
Goss, D. M., Vasilescu, S. A., Sacks, G., Gardner, D. K., and Warkiani, M. E. (2023). Microfluidics facilitating the use of small extracellular vesicles in innovative approaches to male infertility. Rev. Urol. 20(2), 66–95. doi:10.1038/s41585-022-00660-8
Guo, H., Chang, Z., Zhang, Z., Zhao, Y., Jiang, X., Yu, H., et al. (2019). Extracellular ATPs produced in seminal plasma exosomes regulate boar sperm motility and mitochondrial metabolism. Theriogenology. 139, 113–120. doi:10.1016/j.theriogenology.2019.08.003
Gurunathan, S., Kang, M. H., Song, H., Kim, N. H., and Kim, J. H. (2022). The role of extracellular vesicles in animal reproduction and diseases. J. Anim. Sci. Biotechnol. 13(1), 62. doi:10.1186/s40104-022-00715-1
Gurung, S., Greening, D. W., Catt, S., Salamonsen, L., and Evans, J. (2020). Exosomes and soluble secretome from hormone-treated endometrial epithelial cells direct embryo implantation. Mol. Hum. Reprod. 26(7), 510–520. doi:10.1093/molehr/gaaa034
Herrera-Van, O. A., Toro-Ortiz, J. C., Lopez, J. A., Noyola, D. E., Garcia-Lopez, D. A., Duran-Figueroa, N. V., et al. (2020). Placental exosomes isolated from urine of patients with gestational diabetes exhibit a differential profile expression of microRNAs across gestation. Int. J. Mol. Med. 46(2), 546–560. doi:10.3892/ijmm.2020.4626
Homer, H., Rice, G. E., and Salomon, C. (2017). Review: Embryo- and endometrium-derived exosomes and their potential role in assisted reproductive treatments-liquid biopsies for endometrial receptivity. Placenta. 54, 89–94. doi:10.1016/j.placenta.2016.12.011
Hu, J., Dong, J., Zeng, Z., Wu, J., Tan, X., Tang, T., et al. (2020a). Using exosomal miRNAs extracted from porcine follicular fluid to investigate their role in oocyte development. BMC Vet. Res. 16(1), 485. doi:10.1186/s12917-020-02711-x
Hu, J., Tang, T., Zeng, Z., Wu, J., Tan, X., and Yan, J. (2020b). The expression of small RNAs in exosomes of follicular fluid altered in human polycystic ovarian syndrome. PeerJ. 8, e8640. doi:10.7717/peerj.8640
Hua, R., Liu, Q., Lian, W., Kang, T. T., Gao, D., Huang, C., et al. (2022). Extracellular vesicles derived from endometrial epithelial cells deliver exogenous miR-92b-3p to affect the function of embryonic trophoblast cells via targeting TSC1 and DKK3. Reprod. Biol. Endocrinol. 20(1), 152. doi:10.1186/s12958-022-01023-z
Huang, Q., Gong, M., Tan, T., Lin, Y., Bao, Y., and Fan, C. (2021). Human umbilical cord mesenchymal stem Cells-Derived exosomal MicroRNA-18b-3p inhibits the occurrence of preeclampsia by targeting LEP. Nanoscale Res. Lett. 16(1), 27. doi:10.1186/s11671-021-03475-5
Huang, X., Wu, B., Chen, M., Hong, L., Kong, P., Wei, Z., et al. (2020). Depletion of exosomal circLDLR in follicle fluid derepresses miR-1294 function and inhibits estradiol production via CYP19A1 in polycystic ovary syndrome. Aging (Albany NY). 12(15), 15414–15435. doi:10.18632/aging.103602
Huang, Y., Liang, B., and Chen, X. (2021). Exosomal circular RNA circ_0074673 regulates the proliferation, migration, and angiogenesis of human umbilical vein endothelial cells via the microRNA-1200/MEOX2 axis. Bioengineered. 12(1), 6782–6792. doi:10.1080/21655979.2021.1967077
Hung, W. T., Hong, X., Christenson, L. K., and McGinnis, L. K. (2015). Extracellular vesicles from bovine follicular fluid support cumulus expansion. Biol. Reprod. 93(5), 117. doi:10.1095/biolreprod.115.132977
Imakawa, K., Bai, R., Nakamura, K., and Kusama, K. (2017). Thirty years of interferon-tau research; Past, present and future perspective. Anim. Sci. J. 88(7), 927–936. doi:10.1111/asj.12807
Inoue, Y., Munakata, Y., Shinozawa, A., Kawahara-Miki, R., Shirasuna, K., and Iwata, H. (2020). Prediction of major microRNAs in follicular fluid regulating porcine oocyte development. J. Assist. Reprod. Genet. 37(10), 2569–2579. doi:10.1007/s10815-020-01909-0
Jenjaroenpun, P., Kremenska, Y., Nair, V. M., Kremenskoy, M., Joseph, B., and Kurochkin, I. V. (2013). Characterization of RNA in exosomes secreted by human breast cancer cell lines using next-generation sequencing. PeerJ. 1, e201. doi:10.7717/peerj.201
Jeppesen, D. K., Fenix, A. M., Franklin, J. L., Higginbotham, J. N., Zhang, Q., Zimmerman, L. J., et al. (2019). Reassessment of exosome composition. Cell. 177(2), 428–445. doi:10.1016/j.cell.2019.02.029
Johnson, G. A., Burghardt, R. C., and Bazer, F. W. (2014). Osteopontin: A leading candidate adhesion molecule for implantation in pigs and sheep. J. Anim. Sci. Biotechnol. 5(1), 56. doi:10.1186/2049-1891-5-56
Johnstone, R. M., Adam, M., Hammond, J. R., Orr, L., and Turbide, C. (1987). Vesicle formation during reticulocyte maturation. Association of plasma membrane activities with released vesicles (exosomes). J. Biol. Chem. 262(19), 9412–9420. doi:10.1016/s0021-9258(18)48095-7
Kalluri, R., and LeBleu, V. S. (2020). The biology, function, and biomedical applications of exosomes. Science. 367, eaau6977(6478). doi:10.1126/science.aau6977
Kurian, N. K., and Modi, D. (2019). Extracellular vesicle mediated embryo-endometrial cross talk during implantation and in pregnancy. J. Assist. Reprod. Genet. 36(2), 189–198. doi:10.1007/s10815-018-1343-x
Kusama, K., Nakamura, K., Bai, R., Nagaoka, K., Sakurai, T., and Imakawa, K. (2018). Intrauterine exosomes are required for bovine conceptus implantation. Biochem. Bioph. Res. Co. 495(1), 1370–1375. doi:10.1016/j.bbrc.2017.11.176
Lange-Consiglio, A., Capra, E., Monferrini, N., Canesi, S., Bosi, G., Cretich, M., et al. (2022). Extracellular vesicles from seminal plasma to improve fertilizing capacity of bulls. Reprod. Fertil. 3(4), 313–327. doi:10.1530/RAF-22-0037
Latifi, Z., Fattahi, A., Ranjbaran, A., Nejabati, H. R., and Imakawa, K. (2018). Potential roles of metalloproteinases of endometrium-derived exosomes in embryo-maternal crosstalk during implantation. J. Cell. Physiol. 233(6), 4530–4545. doi:10.1002/jcp.26259
Li, B. L., Lu, W., Qu, J. J., Ye, L., Du, G. Q., and Wan, X. P. (2019). Loss of exosomal miR-148b from cancer-associated fibroblasts promotes endometrial cancer cell invasion and cancer metastasis. J. Cell. Physiol. 234(3), 2943–2953. doi:10.1002/jcp.27111
Li, H., Li, X. H., Jiang, J., Shi, P. X., Zhang, X. G., and Tian, M. (2020a). Effect of miR-26b on gestational diabetes mellitus in rats via PI3K/Akt signaling pathway. Eur. Rev. Med. Pharmacol. Sci. 24(4), 1609–1615. doi:10.26355/eurrev_202002_20335
Li, H., Ouyang, Y., Sadovsky, E., Parks, W. T., Chu, T., and Sadovsky, Y. (2020b). Unique microRNA signals in plasma exosomes from pregnancies complicated by preeclampsia. Hypertension. 75(3), 762–771. doi:10.1161/HYPERTENSIONAHA.119.14081
Li, Q., Li, H., Liang, J., Mei, J., Cao, Z., Zhang, L., et al. (2021). Sertoli cell-derived exosomal MicroRNA-486-5p regulates differentiation of spermatogonial stem cell through PTEN in mice. J. Cell. Mol. Med. 25(8), 3950–3962. doi:10.1111/jcmm.16347
Li, Q., Zhang, J., Su, D. M., Guan, L. N., Mu, W. H., Yu, M., et al. (2019). LncRNA TUG1 modulates proliferation, apoptosis, invasion, and angiogenesis via targeting miR-29b in trophoblast cells. Hum. Genomics. 13(1), 50. doi:10.1186/s40246-019-0237-z
Li, Y., Cui, S., Xu, Z., Zhang, Y., Wu, T., Zhang, J., et al. (2022). Exosomal tRF-Leu-AAG-001 derived from mast cell as a potential non-invasive diagnostic biomarker for endometriosis. BMC Womens Health. 22(1), 253. doi:10.1186/s12905-022-01827-6
Liu, T., Liu, M., Zheng, C., Zhang, D., Li, M., and Zhang, L. (2021). Exosomal lncRNA CHL1-AS1 derived from peritoneal macrophages promotes the progression of endometriosis via the miR-610/MDM2 Axis. Int. J. Nanomedicine. 16, 5451–5464. doi:10.2147/IJN.S323671
Llorente, A., Skotland, T., Sylvanne, T., Kauhanen, D., Rog, T., Orlowski, A., et al. (2013). Molecular lipidomics of exosomes released by PC-3 prostate cancer cells. Biochim. Biophys. Acta. 1831(7), 1302–1309. doi:10.1016/j.bbalip.2013.04.011
Lu, Z., and Gao, Y. (2021). Screening differentially expressed genes between endometriosis and ovarian cancer to find new biomarkers for endometriosis. Ann. Med. 53(1), 1377–1389. doi:10.1080/07853890.2021.1966087
Lv, C., Yu, W. X., Wang, Y., Yi, D. J., Zeng, M. H., and Xiao, H. M. (2018). MiR-21 in extracellular vesicles contributes to the growth of fertilized eggs and embryo development in mice. Biosci. Rep. 38(4). doi:10.1042/BSR20180036
Ma, Y., Zhou, Y., Zou, S. S., Sun, Y., and Chen, X. F. (2022). Exosomes released from Sertoli cells contribute to the survival of Leydig cells through CCL20 in rats. Mol. Hum. Reprod. 28, gaac002(2). doi:10.1093/molehr/gaac002
Machtinger, R., Laurent, L. C., and Baccarelli, A. A. (2016). Extracellular vesicles: Roles in gamete maturation, fertilization and embryo implantation. Hum. Reprod. Update. 22(2), 182–193. doi:10.1093/humupd/dmv055
Mahmoud, E. H., Fawzy, A., and Reham, A. E. (2018). Serum MicroRNA-21 negatively relates to expression of programmed cell death-4 in patients with epithelial ovarian cancer. Asian Pac J. Cancer Prev. 19(1), 33–38. doi:10.22034/APJCP.2018.19.1.33
Mogilyansky, E., and Rigoutsos, I. (2013). The miR-17/92 cluster: A comprehensive update on its genomics, genetics, functions and increasingly important and numerous roles in health and disease. Cell. Death Differ. 20(12), 1603–1614. doi:10.1038/cdd.2013.125
Motawi, T., Sabry, D., Maurice, N. W., and Rizk, S. M. (2018). Role of mesenchymal stem cells exosomes derived microRNAs; MiR-136, miR-494 and miR-495 in pre-eclampsia diagnosis and evaluation. Arch. Biochem. Biophys. 659, 13–21. doi:10.1016/j.abb.2018.09.023
Murdica, V., Cermisoni, G. C., Zarovni, N., Salonia, A., Vigano, P., and Vago, R. (2019b). Proteomic analysis reveals the negative modulator of sperm function glycodelin as over-represented in semen exosomes isolated from asthenozoospermic patients. Hum. Reprod. 34(8), 1416–1427. doi:10.1093/humrep/dez114
Murdica, V., Giacomini, E., Alteri, A., Bartolacci, A., Cermisoni, G. C., Zarovni, N., et al. (2019a). Seminal plasma of men with severe asthenozoospermia contain exosomes that affect spermatozoa motility and capacitation. Fertil. Steril. 111(5), 897–908. doi:10.1016/j.fertnstert.2019.01.030
Nakamura, K., Kusama, K., Hori, M., and Imakawa, K. (2021). The effect of bta-miR-26b in intrauterine extracellular vesicles on maternal immune system during the implantation period. Biochem. Biophys. Res. Commun. 573, 100–106. doi:10.1016/j.bbrc.2021.08.019
Nakamura, K., Kusama, K., Ideta, A., Kimura, K., Hori, M., and Imakawa, K. (2019). Effects of miR-98 in intrauterine extracellular vesicles on maternal immune regulation during the peri-implantation period in cattle. Sci. Rep. 9(1), 20330. doi:10.1038/s41598-019-56879-w
Nazri, H. M., Imran, M., Fischer, R., Heilig, R., Manek, S., Dragovic, R. A., et al. (2020). Characterization of exosomes in peritoneal fluid of endometriosis patients. Fertil. Steril. 113(2), 364–373. doi:10.1016/j.fertnstert.2019.09.032
Nguyen, H. P. T., Simpson, R. J., Salamonsen, L. A., and Greening, D. W. (2016). Extracellular vesicles in the intrauterine environment: Challenges and potential functions. Biol. Reprod. 95(5), 109. doi:10.1095/biolreprod.116.143503
Nie, M., Yu, S., Peng, S., Fang, Y., Wang, H., and Yang, X. (2015). MiR-23a and miR-27a promote human granulosa cell apoptosis by targeting SMAD5. Biol. Reprod. 93(4), 98. doi:10.1095/biolreprod.115.130690
Pluchino, S., and Smith, J. A. (2019). Explicating exosomes: Reclassifying the rising stars of intercellular communication. Cell. 177(2), 225–227. doi:10.1016/j.cell.2019.03.020
Pohler, K. G., Green, J. A., Moley, L. A., Gunewardena, S., Hung, W. T., Payton, R. R., et al. (2017). Circulating microRNA as candidates for early embryonic viability in cattle. Mol. Reprod. Dev. 84(8), 731–743. doi:10.1002/mrd.22856
Qamar, A. Y., Mahiddine, F. Y., Bang, S., Fang, X., Shin, S. T., Kim, M. J., et al. (2020). Extracellular vesicle mediated crosstalk between the gametes, conceptus, and female reproductive tract. Front. Vet. Sci. 7, 589117. doi:10.3389/fvets.2020.589117
Qiu, J. J., Lin, X. J., Zheng, T. T., Tang, X. Y., Zhang, Y., and Hua, K. Q. (2019). The exosomal long noncoding RNA aHIF is upregulated in serum from patients with endometriosis and promotes angiogenesis in endometriosis. Reprod. Sci. 26(12), 1590–1602. doi:10.1177/1933719119831775
Rajaratnam, N., Ditlevsen, N. E., Sloth, J. K., Baek, R., Jorgensen, M. M., and Christiansen, O. B. (2021). Extracellular vesicles: An important biomarker in recurrent pregnancy loss? J. Clin. Med. 10, 2549(12). doi:10.3390/jcm10122549
Rodrigues, T. A., Tuna, K. M., Alli, A. A., Tribulo, P., Hansen, P. J., Koh, J., et al. (2019). Follicular fluid exosomes act on the bovine oocyte to improve oocyte competence to support development and survival to heat shock. Reprod. Fertil. Dev. 31(5), 888–897. doi:10.1071/RD18450
Salomon, C., Guanzon, D., Scholz-Romero, K., Longo, S., Correa, P., Illanes, S. E., et al. (2017). Placental exosomes as early biomarker of preeclampsia: Potential role of exosomal MicroRNAs across gestation. J. Clin. Endocrinol. Metab. 102(9), 3182–3194. doi:10.1210/jc.2017-00672
Salomon, C., Scholz-Romero, K., Sarker, S., Sweeney, E., Kobayashi, M., Correa, P., et al. (2016). Gestational diabetes mellitus is associated with changes in the concentration and bioactivity of Placenta-Derived exosomes in maternal circulation across gestation. Diabetes. 65(3), 598–609. doi:10.2337/db15-0966
Shao, X., Qin, J., Wan, C., Cheng, J., Wang, L., Ai, G., et al. (2021). ADSC exosomes mediate lncRNA-MIAT alleviation of endometrial fibrosis by regulating miR-150-5p. Front. Genet. 12. 679643, doi:10.3389/fgene.2021.679643
Shi, S., Tan, Q., Liang, J., Cao, D., Wang, S., Liang, J., et al. (2021). Placental trophoblast cell-derived exosomal microRNA-1290 promotes the interaction between endometrium and embryo by targeting LHX6. Mol. Ther. - Nucleic Acids. 26, 760–772. doi:10.1016/j.omtn.2021.09.009
Song, Y., Wang, M., Tong, H., Tan, Y., Hu, X., Wang, K., et al. (2021). Plasma exosomes from endometrial cancer patients contain LGALS3BP to promote endometrial cancer progression. Oncogene. 40(3), 633–646. doi:10.1038/s41388-020-01555-x
Su, Y., Li, Q., Zhang, Q., Li, Z., Yao, X., Guo, Y., et al. (2021). Exosomes derived from placental trophoblast cells regulate endometrial epithelial receptivity in dairy cows during pregnancy. J. Reprod. Dev. 68, 21, 29. doi:10.1262/jrd.2021-077
Sun, J., Zhao, Y., He, J., Zhou, Q., El-Ashram, S., Yuan, S., et al. (2021). Small RNA expression patterns in seminal plasma exosomes isolated from semen containing spermatozoa with cytoplasmic droplets versus regular exosomes in boar semen. Theriogenology. 176, 233–243. doi:10.1016/j.theriogenology.2021.09.031
Urbanelli, L., Magini, A., Buratta, S., Brozzi, A., Sagini, K., Polchi, A., et al. (2013). Signaling pathways in exosomes biogenesis, secretion and fate. Genes (Basel). 4(2), 152–170. doi:10.3390/genes4020152
Valadi, H., Ekstrom, K., Bossios, A., Sjostrand, M., Lee, J. J., and Lotvall, J. O. (2007). Exosome-mediated transfer of mRNAs and microRNAs is a novel mechanism of genetic exchange between cells. Nat. Cell. Biol. 9(6), 654–659. doi:10.1038/ncb1596
van Niel, G., D'Angelo, G., and Raposo, G. (2018). Shedding light on the cell biology of extracellular vesicles. Nat. Rev. Mol. Cell. Biol. 19(4), 213–228. doi:10.1038/nrm.2017.125
Vargas, A., Zhou, S., Ethier-Chiasson, M., Flipo, D., Lafond, J., Gilbert, C., et al. (2014). Syncytin proteins incorporated in placenta exosomes are important for cell uptake and show variation in abundance in serum exosomes from patients with preeclampsia. FASEB J. 28(8), 3703–3719. doi:10.1096/fj.13-239053
Vilella, F., Moreno-Moya, J. M., Balaguer, N., Grasso, A., Herrero, M., Martínez, S., et al. (2015). Hsa-miR-30d, secreted by the human endometrium, is taken up by the pre-implantation embryo and might modify its transcriptome. Development. 142(18), 3210–3221. doi:10.1242/dev.124289
Vojtech, L., Woo, S., Hughes, S., Levy, C., Ballweber, L., Sauteraud, R. P., et al. (2014). Exosomes in human semen carry a distinctive repertoire of small non-coding RNAs with potential regulatory functions. Nucleic Acids Res. 42(11), 7290–7304. doi:10.1093/nar/gku347
Wang, X., Li, Q., Xie, T., Yuan, M., Sheng, X., Qi, X., et al. (2021). Exosomes from bovine endometrial epithelial cells ensure trophoblast cell development by miR-218 targeting secreted frizzled related protein 2. J. Cell. Physiol. 236(6), 4565–4579. doi:10.1002/jcp.30180
Wang, X., Tian, F., Chen, C., Feng, Y., Sheng, X., Guo, Y., et al. (2019). Exosome-derived uterine microRNAs isolated from cows with endometritis impede blastocyst development. Reprod. Biol. 19(2), 204–209. doi:10.1016/j.repbio.2019.06.003
Wei, H., Chen, Q., Lin, L., Sha, C., Li, T., Liu, Y., et al. (2021). Regulation of exosome production and cargo sorting. Int. J. Biol. Sci. 17(1), 163–177. doi:10.7150/ijbs.53671
Wen, J., and Bai, X. (2021). MiR-520h Inhibits cell survival by targeting mTOR in gestational diabetes mellitus. Acta Biochim. Pol. 68(1), 65–70. doi:10.18388/abp.2020_5389
Wu, H. M., Chen, L. H., Hsu, L. T., and Lai, C. H. (2022). Immune tolerance of embryo implantation and pregnancy: The role of human decidual stromal cell- and Embryonic-Derived extracellular vesicles. Int. J. Mol. Sci. 23, 13382(21). doi:10.3390/ijms232113382
Wu, J., Fang, X., Huang, H., Huang, W., Wang, L., and Xia, X. (2021). Construction and topological analysis of an endometriosis-related exosomal circRNA-miRNA-mRNA regulatory network. Aging (Albany NY). 13(9), 12607–12630. doi:10.18632/aging.202937
Wu, Y., Yuan, W., Ding, H., and Wu, X. (2022). Serum exosomal miRNA from endometriosis patients correlates with disease severity. Arch. Gynecol. Obstet. 305(1), 117–127. doi:10.1007/s00404-021-06227-z
Xiang, Y. J., Hou, Y. Y., Yan, H. L., Liu, H., Ge, Y. X., Chen, N., et al. (2020). Mesenchymal stem cells-derived exosomes improve pregnancy outcome through inducing maternal tolerance to the allogeneic fetus in abortion-prone mating mouse. Kaohsiung J. Med. Sci. 36(5), 363–370. doi:10.1002/kjm2.12178
Xiao, L., He, Y., Peng, F., Yang, J., and Yuan, C. (2020). Endometrial cancer cells promote M2-Like macrophage polarization by delivering exosomal miRNA-21 under hypoxia condition. J. Immunol. Res. 2020, 9731049. doi:10.1155/2020/9731049
Xu, L., Sun, H., Zhang, M., Jiang, Y., Zhang, C., Zhou, J., et al. (2017). MicroRNA-145 protects follicular granulosa cells against oxidative stress-induced apoptosis by targeting Kruppel-like factor 4. Mol. Cell. Endocrinol. 452, 138–147. doi:10.1016/j.mce.2017.05.030
Yang, C., Guo, W. B., Zhang, W. S., Bian, J., Yang, J. K., Zhou, Q. Z., et al. (2017). Comprehensive proteomics analysis of exosomes derived from human seminal plasma. Andrology-US. 5(5), 1007–1015. doi:10.1111/andr.12412
Yang, W., Zhang, J., Xu, B., He, Y., Liu, W., Li, J., et al. (2020). HucMSC-Derived exosomes mitigate the Age-Related retardation of fertility in female mice. Mol. Ther. 28(4), 1200–1213. doi:10.1016/j.ymthe.2020.02.003
Yao, G., Yin, M., Lian, J., Tian, H., Liu, L., Li, X., et al. (2010). MicroRNA-224 is involved in transforming growth Factor-β-Mediated mouse granulosa cell proliferation and granulosa cell function by targeting smad4. Mol. Endocrinol. 24(3), 540–551. doi:10.1210/me.2009-0432
Yuan, C., Li, Z., Zhao, Y., Wang, X., Chen, L., Zhao, Z., et al. (2021). Follicular fluid exosomes: Important modulator in proliferation and steroid synthesis of porcine granulosa cells. FASEB J. 35(5), e21610. doi:10.1096/fj.202100030RR
Yuan, D., Luo, J., Sun, Y., Hao, L., Zheng, J., and Yang, Z. (2021). PCOS follicular fluid derived exosomal miR-424-5p induces granulosa cells senescence by targeting CDCA4 expression. Cell. Signal. 85, 110030. doi:10.1016/j.cellsig.2021.110030
Zaborowski, M. P., Balaj, L., Breakefield, X. O., and Lai, C. P. (2015). Extracellular vesicles: Composition, biological relevance, and methods of study. BioScience. 65(8), 783–797. doi:10.1093/biosci/biv084
Zeng, H., Fu, Y., Shen, L., and Quan, S. (2021). MicroRNA signatures in plasma and plasma exosome during window of implantation for implantation failure following in-vitro fertilization and embryo transfer. Reprod. Biol. Endocrinol. 19(1), 180. doi:10.1186/s12958-021-00855-5
Zhang, L., Li, H. H., Yuan, M., Li, D., and Wang, G. Y. (2020a). Exosomal miR-22-3p derived from peritoneal macrophages enhances proliferation, migration, and invasion of ectopic endometrial stromal cells through regulation of the SIRT1/NF-κB signaling pathway. Eur. Rev. Med. Pharmacol. Sci. 24(2), 571–580. doi:10.26355/eurrev_202001_20033
Zhang, L., Li, H., Yuan, M., Li, D., Sun, C., and Wang, G. (2020b). Serum exosomal MicroRNAs as potential circulating biomarkers for endometriosis. Dis. Markers. 2020, 2456340. doi:10.1155/2020/2456340
Zhang, M., Wang, X., Xia, X., Fang, X., Zhang, T., and Huang, F. (2022). Endometrial epithelial cells-derived exosomes deliver microRNA-30c to block the BCL9/Wnt/CD44 signaling and inhibit cell invasion and migration in ovarian endometriosis. Cell. Death Discov. 8(1), 151. doi:10.1038/s41420-022-00941-6
Zhang, N., Wang, Y., Liu, H., and Shen, W. (2020). Extracellular vesicle encapsulated microRNA-320a inhibits endometrial cancer by suppression of the HIF1α/VEGFA axis. Exp. Cell. Res. 394(2), 112113. doi:10.1016/j.yexcr.2020.112113
Zhang, Y., Chang, X., Wu, D., Deng, M., Miao, J., and Jin, Z. (2021). Down-regulation of exosomal miR-214-3p targeting CCN2 contributes to endometriosis fibrosis and the role of exosomes in the horizontal transfer of miR-214-3p. Reprod. Sci. 28(3), 715–727. doi:10.1007/s43032-020-00350-z
Zhao, G., Yang, C., Yang, J., Liu, P., Jiang, K., Shaukat, A., et al. (2018). Placental exosome-mediated Bta-miR-499-Lin28B/let-7 axis regulates inflammatory bias during early pregnancy. Cell. Death Dis. 9(6), 704. doi:10.1038/s41419-018-0713-8
Zhao, Y., Tao, M., Wei, M., Du S, Wang H., and Wang, X. (2019). Mesenchymal stem cells derived exosomal miR-323-3p promotes proliferation and inhibits apoptosis of cumulus cells in polycystic ovary syndrome (PCOS). Artif. Cells Nanomed Biotechnol. 47(1), 3804–3813. doi:10.1080/21691401.2019.1669619
Zheng, D., Huo, M., Li, B., Wang, W., Piao, H., Wang, Y., et al. (2020). The role of exosomes and exosomal MicroRNA in cardiovascular disease. Front. Cell. Dev. Biol. 8, 616161. doi:10.3389/fcell.2020.616161
Zhou, W., and Dimitriadis, E. (2020). Secreted MicroRNA to predict embryo implantation outcome: From research to clinical diagnostic application. Front. Cell. Dev. Biol. 8. 586510, doi:10.3389/fcell.2020.586510
Zhou, W., Lian, Y., Jiang, J., Wang, L., Ren, L., Li, Y., et al. (2020). Differential expression of microRNA in exosomes derived from endometrial stromal cells of women with endometriosis-associated infertility. Reprod. Biomed. Online. 41(2), 170–181. doi:10.1016/j.rbmo.2020.04.010
Zhou, Z., Tu, Z., Zhang, J., Tan, C., Shen, X., Wan, B., et al. (2022). Follicular Fluid-Derived exosomal MicroRNA-18b-5p regulates PTEN-Mediated PI3K/Akt/mTOR signaling pathway to inhibit polycystic ovary syndrome development. Mol. Neurobiol. 59(4), 2520–2531. doi:10.1007/s12035-021-02714-1
Keywords: exosome, pregnancy-related diseases, embryo implantation, follicular development, endocrine regulation
Citation: Guo X-R, Ma Y, Ma Z-M, Dai T-S, Wei S-H, Chu Y-K and Dan X-G (2023) Exosomes: The role in mammalian reproductive regulation and pregnancy-related diseases. Front. Physiol. 14:1056905. doi: 10.3389/fphys.2023.1056905
Received: 29 September 2022; Accepted: 01 March 2023;
Published: 10 March 2023.
Edited by:
Jean Feugang, Mississippi State University, United StatesReviewed by:
Laura Alessandra Favetta, University of Guelph, CanadaBeatrice Aramini, University of Bologna, Italy
Copyright © 2023 Guo, Ma, Ma, Dai, Wei, Chu and Dan. This is an open-access article distributed under the terms of the Creative Commons Attribution License (CC BY). The use, distribution or reproduction in other forums is permitted, provided the original author(s) and the copyright owner(s) are credited and that the original publication in this journal is cited, in accordance with accepted academic practice. No use, distribution or reproduction is permitted which does not comply with these terms.
*Correspondence: Yuan-Kui Chu, Y2h1eXVhbmt1aUBueG11LmVkdS5jbg==; Xin-Gang Dan, eGdkYW5Abnh1LmVkdS5jbg==