- 1Key Laboratory of Physical Fitness and Exercise, Ministry of Education, Beijing Sport University, Beijing, China
- 2Department of Sports Performance, Beijing Sport University, Beijing, China
- 3China Institute of Sport and Health Science, Beijing Sport University, Beijing, China
Background: Current research suggests that continuous aerobic exercise can be effective in improving vascular endothelial function, while the effect between different intensities and durations of exercise is unclear. The aim of this study was to explore the effect of different durations and intensities of aerobic exercise on vascular endothelial function in different populations.
Methods: Searches were performed in PubMed, Web of Science, and EBSCO databases. We included studies that satisfied the following criteria: 1) randomized controlled trials (RCTs); 2) including both an intervention and control group; 3) using flow-mediated dilation (FMD) as the outcome measure; and 4) testing FMD on the brachial artery.
Results: From 3,368 search records initially identified, 41 studies were eligible for meta-analysis. There was a significant effect of continuous aerobic exercise on improving flow-mediated dilation (FMD) [weighted mean difference (WMD), 2.55, (95% CI, 1.93–3.16), p < 0.001]. Specifically, moderate-intensity [2.92 (2.02–3.825), p < 0.001] and vigorous-intensity exercise [2.58 (1.64–3.53), p < 0.001] significantly increased FMD. In addition, a longer duration [<12 weeks, 2.25 (1.54–2.95), p < 0.001; ≥12 weeks, 2.74 (1.95–3.54), p < 0.001], an older age [age <45, 2.09 (0.78–3.40), p = 0.002; 45 ≤ age <60, 2.25 (1.49–3.01), p < 0.001; age ≥60, 2.62 (1.31–3.94), p < 0.001], a larger basal body mass index (BMI) [20 < BMI < 25, 1.43 (0.98–1.88), p < 0.001; 25 ≤ BMI < 30, 2.49 (1.07–3.90), p < 0.001; BMI ≥ 30, 3.05 (1.69–4.42), p < 0.001], and a worse basal FMD [FMD < 4, 2.71 (0.92–4.49), p = 0.003; 4 ≤ FMD < 7, 2.63 (2.03–3.23), p < 0.001] were associated with larger improvements in FMD.
Conclusion: Continuous aerobic exercise, especially moderate-intensity and vigorous-intensity aerobic exercise, contributed to improving FMD. The effect of continuous aerobic exercise on improving FMD was associated with duration and participant’s characteristics. Specifically, a longer duration, an older age, a larger basal BMI, and a worse basal FMD contributed to more significant improvements in FMD.
Systematic Review Registration: [https://www.crd.york.ac.uk/PROSPERO/display_record.php?RecordID=341442], identifier [CRD42022341442].
Introduction
The most common cause of mortality and morbidity worldwide is cardiovascular disease (CVD) (Townsend et al., 2015; Timmis et al., 2018; Kajikawa and Higashi, 2021), and studies have shown that CVD increases the incidence and mortality of other diseases (Clerkin et al., 2020), e.g., patients with underlying CVD may be at an increased risk for COVID-19 infection and mortality (Huang et al., 2020). Early evidence suggests that endothelial dysfunction, especially impaired endothelium-dependent vasodilation, is closely correlated with the emergence of various CVDs, such as hypertension, atherosclerosis, cardiac failure, and apoplexia (Lloyd-Jones et al., 2010; Tornero-Aguilera et al., 2022). Endothelial dysfunction is the initiating link of atherosclerosis (Thijssen et al., 2011), and early intervention in this link can effectively reverse the development of atherosclerosis (Thijssen et al., 2019). Flow-mediated dilation (FMD) describes the vasodilatory response to increasing shear stress in the brachial artery, which is currently used as a golden standard in evaluating endothelial function (Anderson and Mark, 1989). Several studies have shown that brachial artery FMD is a freestanding predictor of cardiovascular events (Gokce et al., 2002) and all-cause mortality (Xu et al., 2014a).
There is no doubt that absence of physical activity is one of the most significant CVD risk factors that can be modified (Haskell et al., 2007; Lee et al., 2012). The epidemiologic studies have shown that regular exercise, especially regular aerobic exercise, can promote cardiovascular function and reduce overall disease mortality (Bernardo et al., 2018; Wu et al., 2019). And even substantial evidence suggests that people who are physically active have a much higher percentage of survivors after a cardiovascular incident than those who are sedentary, and also describes the beneficial effects of physical activity on heart failure (Brandt et al., 2018). Previous studies have shown that aerobic exercise is superior to other types of exercise in improving vascular endothelial function (Kwon et al., 2011; O'Brien et al., 2020). Short-term aerobic exercise has been shown to significantly ameliorate the function of the endothelium of the brachial artery in male patients with chronic stable heart failure (Belardinelli et al., 2005). Meanwhile, a randomized controlled study found that 12 weeks of continuous aerobic exercise did not significantly improve endothelial function (Molmen-Hansen et al., 2012). And the relationship between aerobic exercise intensity and improvement in endothelial function is currently controversial. One study found that vigorous-intensity aerobic exercise significantly ameliorated endothelial function, whereas moderate-intensity aerobic exercise did not improve FMD (You et al., 2021). However, some studies show that low- or vigorous-intensity aerobic exercise does not improve FMD, a moderate-intensity aerobic exercise program can improve endothelial-dependent vasodilation (Goto et al., 2003; Goto et al., 2007). Therefore, the duration and the intensity of the continuous aerobic exercise are the key factors that affect the effects of intervene. Additionally, aerobic exercise affects vascular endothelial function differently based on the characteristics of the individual. However, some studies showed that aerobic exercise has no significant associations with FMD and baseline endothelial function in non-elderly healthy men and obese patients with type 2 diabetes (Wycherley et al., 2008; Swift et al., 2012; Shenouda et al., 2017).
Therefore, this systematic review and meta-analysis was conducted to investigate the effect of different durations and intensities of continuous aerobic exercise on the vascular endothelial function in people with different characteristics.
Methods
Design
According to PRIMA guidelines, this meta-analysis was conducted following preferred reporting items for systematic reviews and meta-analysis (PRISMA) (Page et al., 2021). The protocol for this systematic review has been registered on PROSPERO (CRD42022341442).
Search strategy
For this systematic review and meta-analysis, we searched PubMed, Web of Science, and EBSCO electronic databases, through December 2021. The initial search consisted of the following MESH terms and keywords: aerobic exercise, flow-mediated dilation, and vascular endothelial function. We also hand-searched reference lists of all identified studies and, in addition, references of reviews and meta-analyses for any additional relevant studies that could be added to the relevant literature. Two authors (XT and YC) completed the process independently using a standardized form. If there was disagreement between the two authors, a third author (LY) would join the discussion until the three reach a consensus.
Eligibility criteria
We included studies that satisfied the following criteria: eligible studies should 1) be randomized controlled trial (RCTs); 2) include both an intervention and control group with the only difference between them being the addition of continuous aerobic exercise in the intervention group; 3) use FMD as the outcome measure; and 4) the location of the FMD test was the brachial artery. In the analysis, non-English published articles, animal model publications, reviews, and conference articles were excluded.
Data extraction
Two authors (XT and YC) independently performed the data extraction, mainly including: 1) characteristics of included studies (first author’s last name, year of study publication); 2) characteristics of continuous aerobic exercise (intensity, frequency, duration, session duration); 3) participant’s characteristics [n, gender, age, basal FMD, and basal body mass index (BMI)]; 4) treatment effects [mean and standard deviation (SD) values reflecting the change in FMD from baseline and to post-intervention in the continuous aerobic exercise and control groups. The corresponding authors of the original study were emailed when the results of the original study were incomplete.
Methodological quality assessment
According to the Cochrane risk of bias criteria, we assessed the methodological quality of the studies included in this review (Higgins et al., 2011), and the quality assessment of eligible studies was based on factors including selection bias, performance bias, detection bias, attrition bias, reporting bias, and other biases. Independent assessments of methodological quality were conducted by two authors (XT and YC), and disagreements were resolved through discussion and consensus with a third author (LY).
Statistical analysis
Each study’s mean and SD values reflecting the change in FMD from baseline to post-intervention have been extracted for pooling purposes. Data were pooled using random-effects models to obtain the weighted mean differences (WMDs) and 95% confidence intervals (CIs). When I2 is < 50%, data were pooled using fixed effects models to obtain the WMD and 95% CIs; when I2 is ≥ 50%, data were pooled using random effects models to obtain the WMD and 95% CIs (Li et al., 2022). If there was a high heterogeneity (I2 > 60%), sensitivity analysis and subgroup analysis were used to interpret the results (Shamseer et al., 2015; Zhang et al., 2022; Zhen et al., 2022). In the subgroup analyses, we attempted to use durations of continuous aerobic exercise (<12 weeks, ≥12 weeks), intensities of continuous aerobic exercise (low-intensity, moderate-intensity, vigorous-intensity), participants’ age (young, age < 45; middle-aged, 45 ≤ age <60; elderly, age ≥60), basal BMI (normal weight, 20 < BMI < 25; overweight, 25 ≤ BMI < 30; obese, BMI ≥ 30) (Pi-Sunyer et al., 1998), and basal FMD (abnormal, FMD < 4; critical, 4 ≤ FMD < 7; normal, FMD ≥ 7) (Tanaka et al., 2018) to explore the impact on FMD. The analysis result, funnel plots, and forest plots were generated using RevMan 5.0 software. In terms of overall impact, p < 0.05 was considered statistically significant.
Results
Study selection
Figure 1 showed the situation, preliminary results have been retrieved for 3,346 search records, and 22 records have been identified through other means. Following the exclusion of duplicate studies, 2,758 studies remained, and 2,104 studies were not eligible for inclusion through the title and abstract screening. Six hundred and four studies were excluded by reading the full text of 654 studies: 1) non-RCTs (n = 245); 2) no control group (n = 94); 3) the intervention was resistance training (n = 58); 4) a combination of treatments was used in the experimental group (n = 56); 5) no relevance was found in the outcomes (n = 94); 3); and 6) data could not be extracted (n = 57). Finally, 50 studies examining the effect of continuous aerobic exercise on FMD were considered eligible for systematic review, of which 41 studies were considered eligible for meta-analysis.
Description of the included studies
The main characteristics of interventions and participants were presented in Table 1. There were 1959 participants enrolled in the 41 studies. Of the 41 studies, six studies involved diabetes participants (Wycherley et al., 2008; Kwon et al., 2011; Choi et al., 2012; Mitranun et al., 2014; Ghardashi et al., 2018; Boff et al., 2019), five studies involved healthy participants (Yoshizawa et al., 2010; Pierce et al., 2011; Shenouda et al., 2017; King and Pyke, 2020; Haynes et al., 2021), five studies involved high blood pressure participants (Westhoff et al., 2007; Westhoff et al., 2008; Molmen-Hansen et al., 2012; Beck et al., 2013; Boeno et al., 2020), four studies involved obese participants (Kelly et al., 2004; Meyer et al., 2006; Swift et al., 2012; Bhutani et al., 2013), four studies involved heart failure participants (Kobayashi et al., 2003; Belardinelli et al., 2005; Wisløff et al., 2007; Erbs et al., 2010), three studies involved metabolic syndrome participants (Lavrencic et al., 2000; Tjønna et al., 2008; Tjønna et al., 2011), three studies involved coronary artery disease participants (Sixt et al., 2008; Vona et al., 2009; Desch et al., 2010), two studies involved heart disease participants (Blumenthal et al., 2005; Braith et al., 2008), two studies involved hormonal diseases participants (Sprung et al., 2013; Jones et al., 2014), one study involved myocardial infarction participants (Vona et al., 2004), one study involved coronary heart disease (CHD) participants (Swift et al., 2012), one study involved peripheral arterial disease participants (McDermott et al., 2009), one study involved depression participants (Sherwood et al., 2016), one study involved kidney disease participants (Kirkman et al., 2019), one study involved non-alcoholic fatty liver disease (NAFLD) participants (Pugh et al., 2014), and one study involved high cardiovascular risk participants (Jo et al., 2020). In studies examining the effect of continuous aerobic exercise on endothelial function in diseased participants, drug use did not change throughout the intervention period. In addition, gender was not a factor in about 80% of studies, with only five studies targeting women (Yoshizawa et al., 2010; Kwon et al., 2011; Choi et al., 2012; Sprung et al., 2013; Jo et al., 2020) and four studies targeting men (Lavrencic et al., 2000; Jones et al., 2014; Shenouda et al., 2017; King and Pyke, 2020).
The overall duration varied from 4 weeks to 24 weeks. As stated in the position statement regarding physical activity and training intensity (Norton et al., 2010), following are the modifications we made to the classification of continuous aerobic exercise intensity according to the included research situation: 1.6 < metabolic equivalents (METs) < 3, 20% < maximal oxygen uptake (VO2max) < 40%, 40% < maximal heart rate (HRmax) < 55%, 20% < heart rate reserve (HRR) < 40%, or 8 < rating of perceived exertion (RPE) < 10 were determined as low-intensity; 3 < METs < 6, 40% < VO2max < 60%, 55% < HRmax < 70%, 40% < HRR < 60%, or 11 < RPE < 13 were determined as moderate-intensity; 6 < METs < 9, 60% < VO2max < 85%, 70% < HRmax < 90%, 60% < HRR < 85%, or 14 < RPE < 16 were determined as vigorous-intensity.
Risk of bias
Evaluation of the methodological quality of the included literature was conducted using the Cochrane risk assessment tool, mainly from selection bias, performance bias, detection bias, attrition bias, reporting bias and other biases. Quality was scored according to three levels (low risk, high risk and unclear). The quality of the included literature is divided into three levels from high to low: high quality, medium quality and low quality (Figures 2, 3). Publication bias was assessed visually by inspecting the funnel plot (Figure 4).
Meta-analysis results
The effect of continuous aerobic exercise on FMD
We found that continuous aerobic exercise had a significant effect on increasing FMD when compared to the control group [WMD, 2.55 (95% CI, 1.93–3.16), p < 0.001, Figure 5], while there was a significant heterogeneity (I2 = 95%). Hence, a sensitivity analysis in which 1 study was removed at a time, was performed to evaluate the stability of the results. Sensitivity analysis results were shown in Supplementary Figure S1, the pooled effect changed slightly by removing each study, which confirmed the stability of our results. Therefore, we used subgroup analyses to interpret the results.
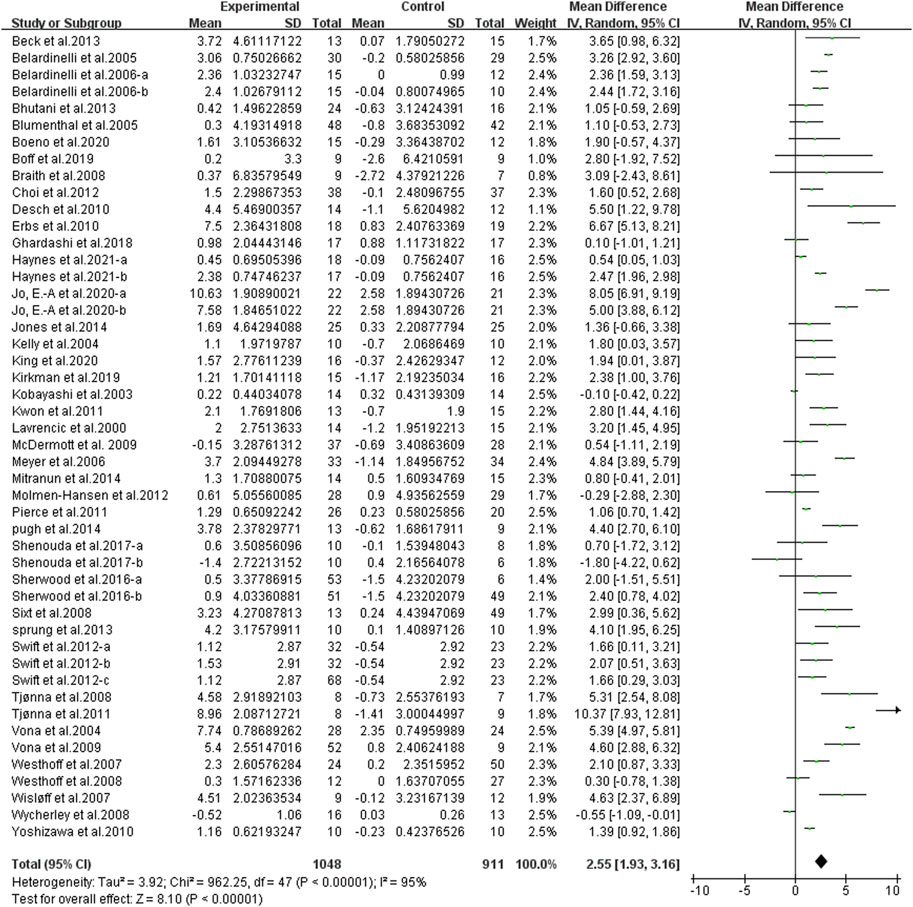
FIGURE 5. Meta-analysis results of the effect of continuous aerobic exercise on FMD. The pooled estimates were obtained from random effects analysis. Diamonds indicated the effect size of each study summarized as WMD. The size of the shaded squares was proportional to the percentage weight of each study. Horizontal lines represented the 95% CI and the vertical line represented the overall effect.
Subgroup analysis
Different results were shown when considering duration of intervention (Figure 6). The subgroup analysis indicated that a longer duration was associated with larger improvements in FMD [< 12 weeks, WMD, 2.25 (95%CI, 1.54–2.95), p < 0.001, I2 = 88%; ≥ 12 weeks, WMD, 2.74 (95%CI, 1.95–3.54), p < 0.001, I2 = 95%].
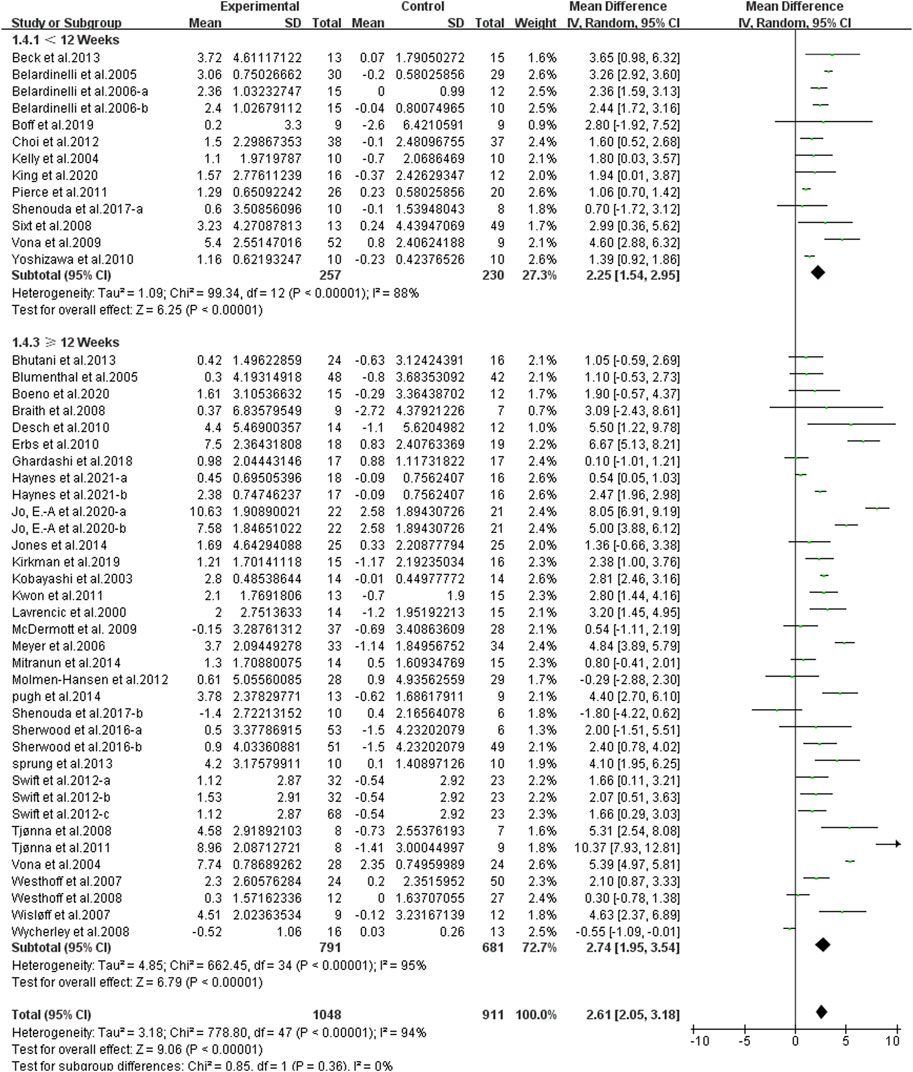
FIGURE 6. Meta-analysis results of the effect of different durations of continuous aerobic exercise on FMD. The pooled estimates were obtained from random effects analysis. Diamonds indicated the size of the effect of each study summarized as WMD. The size of the shaded square was proportional to the percentage weight of each study. Horizontal lines represented the 95% CI and the vertical dashed line represented the overall effect.
Different results were shown when considering exercise intensity (Figure 7). Specifically, compared with the control group, moderate-intensity exercise [WMD, 2.92 (95%CI, 2.02–3.82), p < 0.001, I2 = 87%] and vigorous-intensity exercise [WMD, 2.58 (95%CI, 1.64–3.53), p < 0.001, I2 = 95%] significantly increased FMD, while low-intensity exercise had no significant effect on FMD [WMD, 2.10 (95%CI, −1.36–5.55), p = 0.23, I2 = 87%].
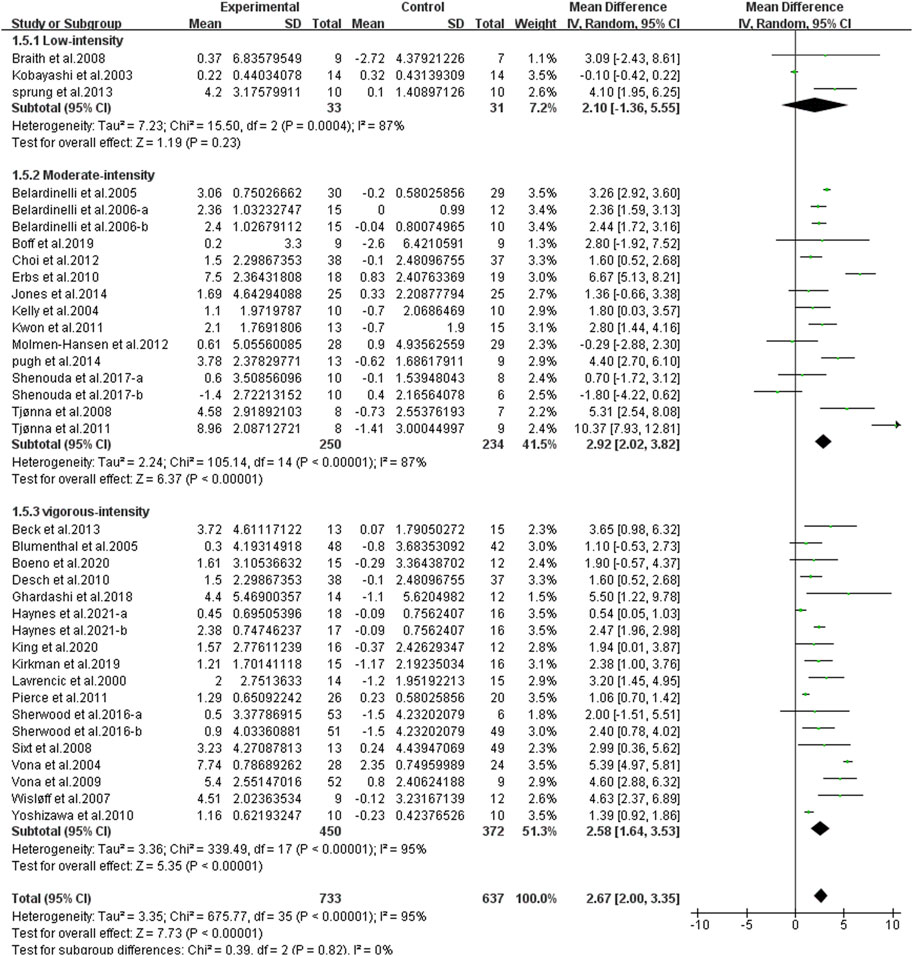
FIGURE 7. Meta-analysis results of the effect of different intensities of continuous aerobic exercise on FMD. The pooled estimates were obtained from random effects analysis. Diamonds indicated the size of the effect of each study summarized as WMD. The size of the shaded square was proportional to the percentage weight of each study. Horizontal lines represented the 95% CI and the vertical dashed line represented the overall effect.
Different results were shown when considering participants’ characteristics. The subgroup analysis indicated that an older age [young, age < 45, WMD, 2.09 (95%CI, 0.78–3.40), p = 0.002, I2 = 81%; middle-aged, 45 ≤ age <60, WMD, 2.25 (95%CI, 1.49–3.01), p < 0.001, I2 = 94%; elderly, age ≥60, WMD, 2.62 (95%CI, 1.31–3.94), p < 0.001, I2 = 97%, Figure 8], a larger basal BMI [normal weight, 20 < BMI < 25, WMD, 1.43 (95%CI, 0.98–1.88), p < 0.001, I2 = 0%; overweight, 25 ≤ BMI < 30, WMD, 2.49 (95%CI, 1.07–3.90), p < 0.001, I2 = 92%; obese, BMI ≥ 30, WMD, 3.05 (95%CI, 1.69–4.42), p < 0.001, I2 = 92%, Figure 9], and a worse basal FMD [abnormal, FMD < 4, WMD, 2.71 (95%CI, 0.92–4.49), p = 0.003, I2 = 99%; critical, 4 ≤ FMD < 7, WMD, 2.63 (95%CI, 2.03–3.23), p < 0.001, I2 = 90%, Figure 10] were associated with larger improvements in FMD. However, continuous aerobic exercise had no significant effect on FMD in people with normal basal FMD [WMD, 1.66 (95%CI, −0.28–3.59), p = 0.09, I2 = 60%, Figure 10].
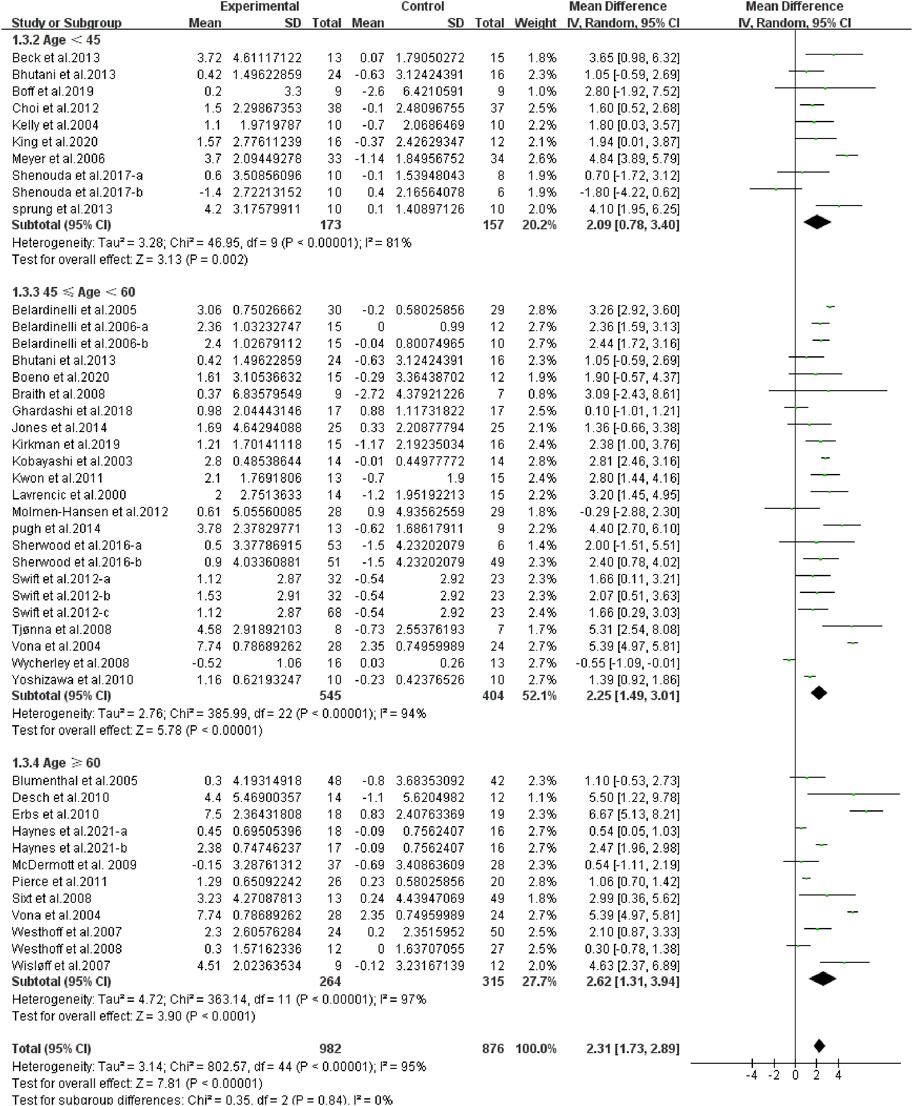
FIGURE 8. Meta-analysis results of the effect of continuous aerobic exercise on FMD in young, middle-aged, or elderly people. The pooled estimates were obtained from random effects analysis. Diamonds indicated the size of the effect of each study summarized as WMD. The size of the shaded square was proportional to the percentage weight of each study. Horizontal lines represented the 95% CI and the vertical dashed line represented the overall effect.
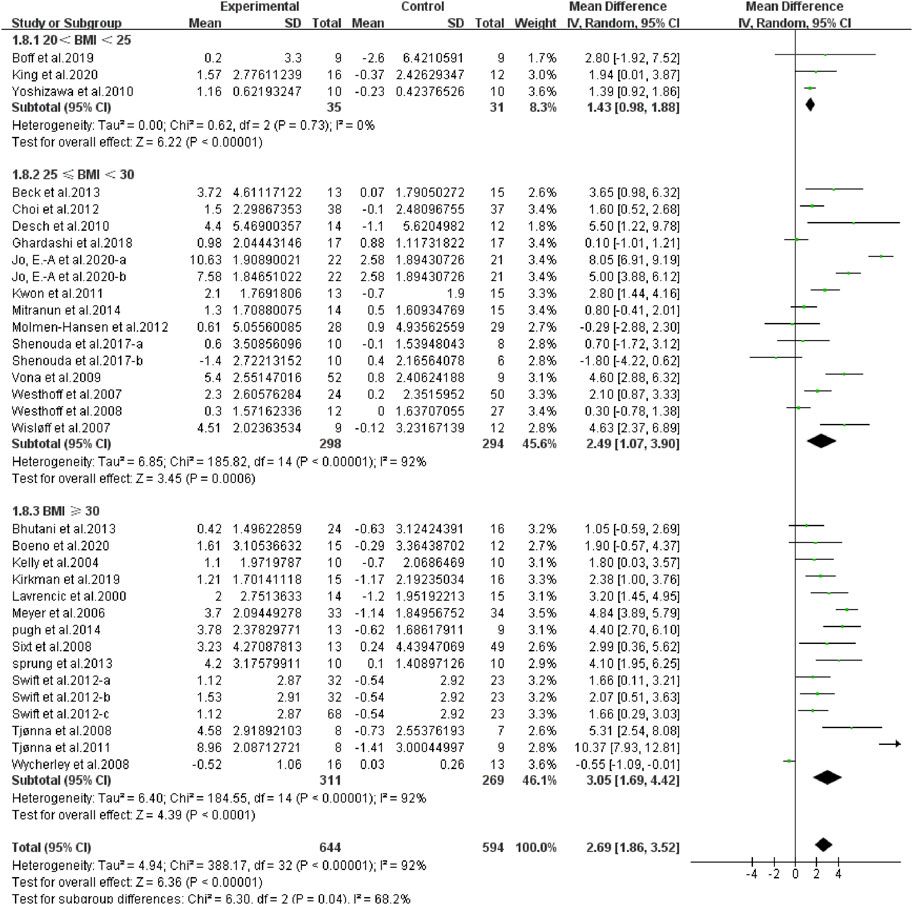
FIGURE 9. Meta-analysis results of the effect of continuous aerobic exercise on FMD in normal weight, overweight, or obese people. The pooled estimates were obtained from random effects analysis. Diamonds indicated the size of the effect of each study summarized as WMD. The size of the shaded square was proportional to the percentage weight of each study. Horizontal lines represented the 95% CI and the vertical dashed line represented the overall effect.
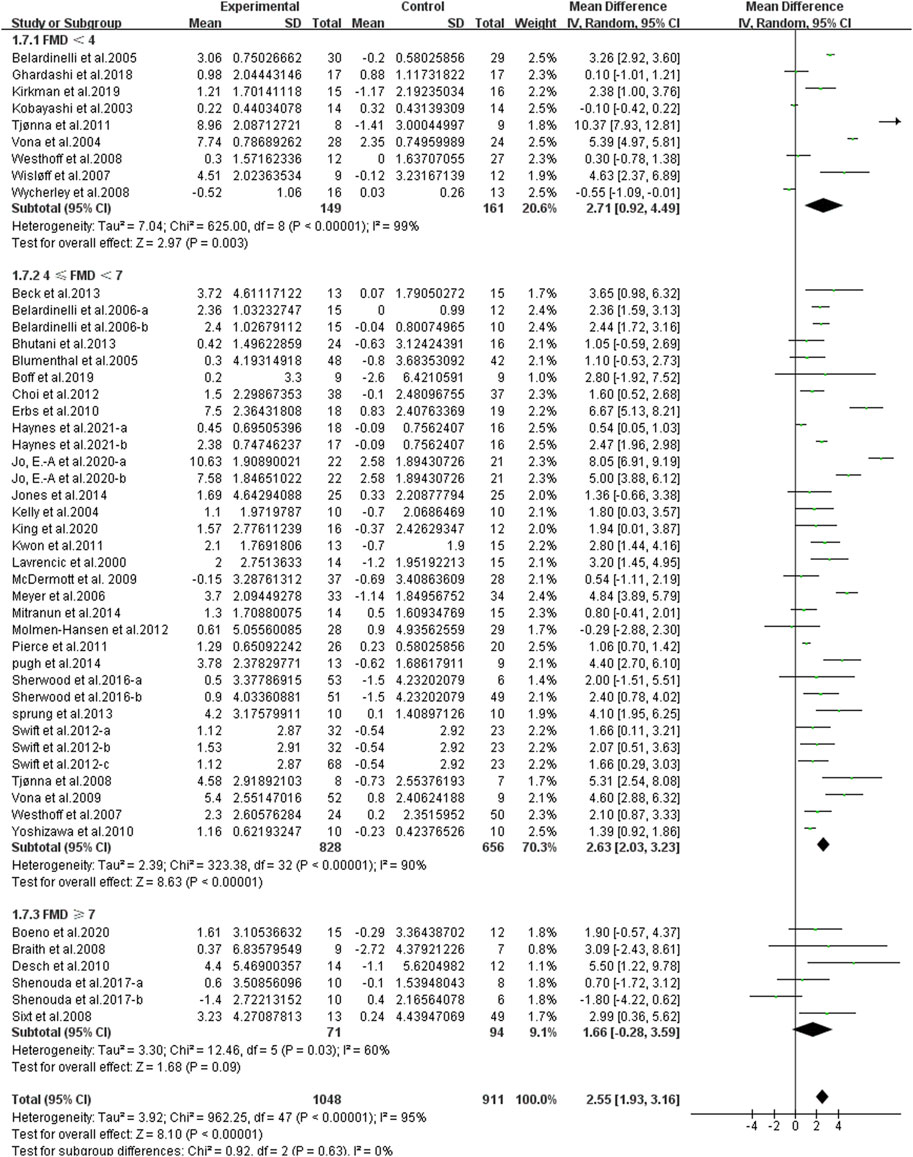
FIGURE 10. Meta-analysis results of the effect of continuous aerobic exercise on FMD in people with normal, critical, or abnormal basal FMD. The pooled estimates were obtained from random effects analysis. Diamonds indicated the size of the effect of each study summarized as WMD. The size of the shaded square was proportional to the percentage weight of each study. Horizontal lines represented the 95% CI and the vertical dashed line represented the overall effect.
Discussion
The effect of continuous aerobic exercise on endothelial function
This systematic review and meta-analysis indicated that continuous aerobic exercise significantly improved endothelial function, as revealed by increased FMD, which was consistent with the results of You et al. (2021), showing that aerobic exercise improved endothelial function. Our study showed that continuous aerobic exercise contributed to an overall improvement in the FMD by 2.55 (WMD), which has a clinical importance for public health. The mechanism of continuous aerobic exercise improving vascular endothelial function had not been fully revealed, the following potential mechanisms might explain the beneficial effects of continuous aerobic exercise on vascular endothelial function.
First, flow shear stress (FSS), the frictional force exerted by blood on the vessel wall, is considered a regulator of the vascular endothelium (Girard and Nerem, 1995). Numerous studies have shown that aerobic exercise increase blood flow shear stress (Goto et al., 2003; Tinken et al., 2010) that can directly induce increased NO synthesis and release from vascular endothelium (Green et al., 2004; Thijssen et al., 2010), increasing NO bioavailability (Taddei et al., 2000) and secondary enhancement of endothelial nitric oxide synthase (eNOS) expression (Hambrecht et al., 2003; Newcomer et al., 2011). Second, it has been suggested that inflammation plays a central role in endothelial dysfunction (a critical step in the progression of CVD) (Rathod et al., 2017), aerobic exercise can reduce plasma biomarkers of low-grade inflammation [highly sensitive C-reactive protein (hs-CRP), serum amyloid A (SAA), soluble intercellular adhesion molecule-1 (sICAM-1), interleukin-6 (IL-6), interleukin-8 (IL-8), and tumor necrosis factor-α (TNF-α)], endothelial dysfunction biomarkers [soluble vascular adhesion molecule-1 (sVCAM-1) and soluble E-selectin (sE-selectin)] (Vandercappellen et al., 2022), levels of l-arginine, and ratios of l-arginine to citrulline, citrulline to ornithine, and l-arginine to ornithine (Tsukiyama et al., 2017). Third, oxidative stress is one of the risk factors for vascular endothelial disorders (Yilmaz et al., 2020). Aerobic exercise can modulate oxidative stress, increase the expression of antioxidant enzymes, and regulate reactive oxygen species (ROS) in mitochondria, and ROS induces nuclear transcriptional coactivators PPAR-gamma co-activator-1 alpha/beta (PGC1α/β) and activates several nuclear transcription factors, including peroxisome proliferator-activated receptor gamma (PPARγ) and its targets superoxide dismutase (SOD) 1, SOD2, glutathione peroxidase (GPx) 1, and catalase (CAT) (St-Pierre et al., 2006). Fourth, studies have shown that regular aerobic exercise can normalize the basal overactivity of the sympathetic nervous system (Antunes-Correa et al., 2010), muscle sympathetic nerve activity (MSNA) is a method that records sympathetic nerve passage, which is reduced by aerobic exercise (Notarius et al., 2015). NO also regulates vascular endothelial function by interacting with the autonomic nervous system (Gamboa et al., 2012). Finally, aerobic exercise can increase endothelial progenitor cell (EPC) number and differentiation capacity (Landers-Ramos et al., 2019), as well as vascular endothelial growth factor (VEGF) and insulin growth factor-1 (IGF-1) (Vonderwalde and Kovacs-Litman, 2018), which can contribute to vascular regeneration and angiogenesis, and aerobic exercise can promote the secretion of active protective factors, such as endothelin (ET), prostacyclin I2 (PGI2), angiotensin II (Ang II), arginase, and other biologically active molecules (Beck et al., 2013), which have a positive effect on endothelial function. It may be possible to use endothelial progenitor cells as a surrogate biologic marker for cardiovascular function and cumulative morbidity (Hill et al., 2003; Koutroumpi et al., 2012).
Subgroup analysis
In the studies we included, continuous aerobic exercise significantly improved vascular endothelial function, while there was considerable heterogeneity between groups. Therefore, we used subgroup analysis to interpret the results. First, the effect of aerobic exercise is closely related to the duration and intensity of the intervention, so we performed subgroup analyses about intervention duration and exercise intensity. Our results showed that the duration of ≥ 12 weeks and < 12 weeks both improved the vascular endothelial function significantly, and ≥ 12 weeks of continuous aerobic exercise was more effective in improving FMD than < 12 weeks. Previous studies have found that short-term training can increase the bioavailability of eNOS and NO, thereby improving vascular endothelial function (Sessa et al., 1994; Sun et al., 1994; Kingwell et al., 1997). However, some studies have found that long-term training can expand the diameter of arteries (Leon and Bloor, 1968; Wyatt and Mitchell, 1978; Lash and Bohlen, 1992), the better effect of ≥ 12 weeks may be due to structural changes in the vessel caused by long-term repetitive training, a phenomenon sometimes referred to as “arterial remodeling’’ (Prior et al., 2003). Time-course studies have shown that training firstly induces functional changes, followed by structural remodeling that restores function to baseline levels (Laughlin, 1995). While some evidence suggests that diameter may not be significantly enlarged in all situation at rest, this may be due to compensatory increases in vasoconstrictor and relaxation tone to regulate blood pressure and vascular resistance, which can increase the flow shear stress, thereby improving the vascular endothelial function (Green et al., 2012). Aerobic exercise improves vascular endothelium, and there appears to be an optimal training intensity. In our study, moderate-intensity exercise and vigorous-intensity exercise significantly increased FMD, and moderate-intensity exercise having a greater effect than vigorous-intensity exercise, while low-intensity exercise had no significant effect on FMD, which was in line with the report by Goto et al. (2003), showing that low-intensity aerobic exercise has no effect on vascular endothelial function. In this context, low-intensity aerobic exercise may not provide the optimal shear stress stimulus with the potential to improve endothelial function (Green et al., 2004), whereas moderate-intensity and high-intensity aerobic exercise enhances endothelial-dependent vasodilation in humans by increasing NO production, blood flow, blood flow speed, and shear stress, and reducing ROS production (Goto et al., 2003; Harrison et al., 2006; Tinken et al., 2010; Ramos et al., 2015; Green et al., 2017). Therefore, we speculated that there may be a dose-response relationship between the duration of continuous aerobic exercise and FMD, and this relationship depends not only on duration but also on exercise intensity. Discovering the optimal effect of exercise duration and optimal intensity threshold may help to design more effective and safe clinical prescriptions for patients with endothelial dysfunction.
Studies have previously shown that increasing FMD is positively related to reducing the risk of CVD, and that every 1% increase in FMD in the brachial artery decreases risk by 13% (Inaba et al., 2010; Ras et al., 2013; Xu et al., 2014b; Matsuzawa et al., 2015; Thijssen et al., 2019). Therefore, the changes of CVD risk factors are closely related to the improvement of vascular endothelial function, so in the subgroup analyses, we also sought to determine the effects of participants’ characteristics (age, basal BMI, and basal FMD). Our results showed that a worse basal FMD was associated with a larger improvement in FMD, while continuous aerobic exercise had no significant effect on FMD in people with normal basal FMD, which was consistent with a previous study, showing that when FMD was normal at baseline, there was no significant difference in the effect of the intervention on FMD, but those with impaired endothelial function may have greater improvement, and aerobic exercise would benefit individuals most in need of improved endothelial function (Swift et al., 2012; Ashor et al., 2015).
In atddition, our results showed that an older age was associated with a larger improvement in FMD, which was consistent with previous studies (Skaug et al., 2013; Konigstein et al., 2022). There is no doubt that endothelial function is affected by age (Ungvari et al., 2018). During aging, the vascular system produces excess superoxide and hydrogen peroxide, both of which inhibit the vasodilatory activity of NO and lead to the formation of free radicals (e.g., proximities), whereas aging results in a low-grade inflammatory phenotype in the vascular system (El Assar et al., 2013). The vascular endothelial function is impaired due to the oxidative stress increase and other factors, while aerobic exercise reduces oxidative stress by inhibiting oxidation and stimulating antioxidant pathways (Seals et al., 2019). Obesity is an independent predictor of CVDs, with progressive increases in BMI associated with increased risk of CVD (Hubert et al., 1983; Donato et al., 2018). Therefore, we divided the included studies based on their participants’ basal BMI into three groups: normal weight, overweight, and obese. Our results showed that compared with the control group, continuous aerobic exercise significantly improved FMD in normal weight, overweight, and obese people. In addition, a larger basal BMI was associated with larger improvements in FMD, indicating that continuous aerobic exercise had a better effect on FMD in higher BMI people than in lower BMI people. The concept that obesity is characterized by low levels of chronic and subclinical inflammation is becoming more widely accepted (Hajer et al., 2008). Excessive BMI produces high levels of inflammatory factors, and the pro-inflammatory state in adipose tissue leads to local insulin resistance and impaired inhibition of free fatty acids (FFA) release by insulin (Gustafson, 2010). Continuous aerobic exercise can reduce inflammatory factors and increase antioxidant capacity (Gielen et al., 2010; Vandercappellen et al., 2022). Aerobic exercise reduces BMI in obese individuals, and endurance training is associated with increased circulating high-density lipoprotein (HDL) levels and decreased very low-density lipoprotein (LDL) (Halverstadt et al., 2007). It improves insulin sensitivity (Newsom et al., 2013) and insulin signaling within the vascular endothelium promotes Akt-dependent phosphorylation and activation of eNOS to produce the vasodilator NO (Steinberg et al., 1994), thereby improving vascular endothelial function. In short, age and obesity are the main factors causing vascular endothelial dysfunction, and the different effects of continuous aerobic exercise to FMD can be proved by the intervention effect to participants with different age basal BMI.
Limitations of the review
It is important to acknowledge some potential limitations of this meta-analysis. As for the inclusion criteria, all included studies were RCTs using continuous aerobic exercise interventions, which could not be completely blinded. As a result, subjective factors will contribute to a certain degree of deviation in quality evaluation. Additionally, there was a high degree of heterogeneity, which may be due to multiple risk factors such as smoking, food and alcohol intake, blood pressure, and blood lipids. Moreover, previous studies have shown that high-intensity intervals training (HIIT) can also be effective in improving FMD (Molmen-Hansen et al., 2012; Ghardashi et al., 2018; Boff et al., 2019), but our study only focused on the effect of continuous aerobic exercise on FMD, and future studies should take HIIT into account to make the results more comprehensive. Furthermore, gender differences were not considered due to the limited availability of separate male and female studies in this study. Finally, because of the significant heterogeneity in the results of the meta-analysis, the results of our study should be interpreted with caution.
Conclusion
Our analysis indicated that continuous aerobic exercise, especially moderate-intensity and vigorous-intensity aerobic exercise, contributed to improving FMD. The effect of continuous aerobic exercise on improving FMD was associated with duration and participant’s characteristics. Specifically, a longer duration, an older age, a larger basal BMI, and a worse basal FMD contributed to more significant improvements in FMD.
Data availability statement
The original contributions presented in the study are included in the article/Supplementary Material, further inquiries can be directed to the corresponding authors.
Author contributions
XT and YL conceived the idea and design for the article. XT, YC, KZ, and SR performed the literature search and data acquisition, analysis, and interpretation. XT and YC carried out the meta-analysis with assistance from YL and LY. XT drafted the manuscript and all authors contributed to editing and revising the manuscript and approved the final version prior to submission.
Funding
This study was supported by the National Key R&D Program of China (2022YFC3600201), the Chinese Universities Scientific Fund (2022QN015, LY), and Graduate Students' Innovative Scientific Research Program of Beijing Sport University (20221060, XT).
Conflict of interest
The authors declare that the research was conducted in the absence of any commercial or financial relationships that could be construed as a potential conflict of interest.
Publisher’s note
All claims expressed in this article are solely those of the authors and do not necessarily represent those of their affiliated organizations, or those of the publisher, the editors and the reviewers. Any product that may be evaluated in this article, or claim that may be made by its manufacturer, is not guaranteed or endorsed by the publisher.
Supplementary material
The Supplementary Material for this article can be found online at: https://www.frontiersin.org/articles/10.3389/fphys.2023.1043108/full#supplementary-material
References
Anderson E. A., Mark A. L. (1989). Flow-mediated and reflex changes in large peripheral artery tone in humans. Circulation 79 (1), 93–100. doi:10.1161/01.cir.79.1.93
Antunes-Correa L. M., Melo R. C., Nobre T. S., Ueno L. M., Franco F. G., Braga A. M., et al. (2010). Impact of gender on benefits of exercise training on sympathetic nerve activity and muscle blood flow in heart failure. Eur. J. Heart Fail 12 (1), 58–65. doi:10.1093/eurjhf/hfp168
Ashor A. W., Lara J., Siervo M., Celis-Morales C., Oggioni C., Jakovljevic D. G., et al. (2015). Exercise modalities and endothelial function: A systematic review and dose-response meta-analysis of randomized controlled trials. Sports Med. 45 (2), 279–296. doi:10.1007/s40279-014-0272-9
Beck D. T., Casey D. P., Martin J. S., Emerson B. D., Braith R. W. (2013). Exercise training improves endothelial function in young prehypertensives. Exp. Biol. Med. (Maywood) 238 (4), 433–441. doi:10.1177/1535370213477600
Belardinelli R., Lacalaprice F., Faccenda E., Purcaro A., Perna G. (2005). Effects of short-term moderate exercise training on sexual function in male patients with chronic stable heart failure. Int. J. Cardiol. 101 (1), 83–90. doi:10.1016/j.ijcard.2004.05.020
Belardinelli R., Capestro F., Misiani A., Scipione P., Georgiou D. (2006). Moderate exercise training improves functional capacity, quality of life, and endothelium-dependent vasodilation in chronic heart failure patients with implantable cardioverter defibrillators and cardiac resynchronization therapy. Eur. J. Cardiovasc. Prev. Rehabil. 13 (5), 818–825. doi:10.1097/01.hjr.0000230104.93771.7d
Bernardo B. C., Ooi J. Y. Y., Weeks K. L., Patterson N. L., McMullen J. R. (2018). Understanding key mechanisms of exercise-induced cardiac protection to mitigate disease: Current knowledge and emerging concepts. Physiol. Rev. 98 (1), 419–475. doi:10.1152/physrev.00043.2016
Bhutani S., Klempel M. C., Kroeger C. M., Trepanowski J. F., Phillips S. A., Norkeviciute E., et al. (2013). Alternate day fasting with or without exercise: Effects on endothelial function and adipokines in obese humans. e-SPEN J. 8 (5), e205–e209. doi:10.1016/j.clnme.2013.07.005
Blumenthal J. A., Sherwood A., Babyak M. A., Watkins L. L., Waugh R., Georgiades A., et al. (2005). Effects of exercise and stress management training on markers of cardiovascular risk in patients with ischemic heart disease: A randomized controlled trial. JAMA 293 (13), 1626–1634. doi:10.1001/jama.293.13.1626
Boeno F. P., Ramis T. R., Munhoz S. V., Farinha J. B., Moritz C. E. J., Leal-Menezes R., et al. (2020). Effect of aerobic and resistance exercise training on inflammation, endothelial function and ambulatory blood pressure in middle-aged hypertensive patients. J. Hypertens. 38 (12), 2501–2509. doi:10.1097/HJH.0000000000002581
Boff W., da Silva A. M., Farinha J. B., Rodrigues-Krause J., Reischak-Oliveira A., Tschiedel B., et al. (2019). Superior effects of high-intensity interval vs. moderate-intensity continuous training on endothelial function and cardiorespiratory fitness in patients with type 1 diabetes: A randomized controlled trial. Front. Physiology 10, 450. doi:10.3389/fphys.2019.00450
Braith R. W., Schofield R. S., Hill J. A., Casey D. P., Pierce G. L. (2008). Exercise training attenuates progressive decline in brachial artery reactivity in heart transplant recipients. J. Heart Lung Transpl. 27 (1), 52–59. doi:10.1016/j.healun.2007.09.032
Brandt N., Gunnarsson T. P., Bangsbo J., Pilegaard H. (2018). Exercise and exercise training-induced increase in autophagy markers in human skeletal muscle. Physiol. Rep. 6 (7), e13651. doi:10.14814/phy2.13651
Choi K. M., Han K. A., Ahn H. J., Hwang S. Y., Hong H. C., Choi H. Y., et al. (2012). Effects of exercise on sRAGE levels and cardiometabolic risk factors in patients with type 2 diabetes: A randomized controlled trial. J. Clin. Endocrinol. Metab. 97 (10), 3751–3758. doi:10.1210/jc.2012-1951
Clerkin K. J., Fried J. A., Raikhelkar J., Sayer G., Griffin J. M., Masoumi A., et al. (2020). COVID-19 and cardiovascular disease. Circulation 141 (20), 1648–1655. doi:10.1161/CIRCULATIONAHA.120.046941
Desch S., Sonnabend M., Niebauer J., Sixt S., Sareban M., Eitel I., et al. (2010). Effects of physical exercise versus rosiglitazone on endothelial function in coronary artery disease patients with prediabetes. Diabetes, Obes. metabolism 12 (9), 825–828. doi:10.1111/j.1463-1326.2010.01234.x
Donato A. J., Machin D. R., Lesniewski L. A. (2018). Mechanisms of dysfunction in the aging vasculature and role in age-related disease. Circ. Res. 123 (7), 825–848. doi:10.1161/CIRCRESAHA.118.312563
El Assar M., Angulo J., Rodriguez-Manas L. (2013). Oxidative stress and vascular inflammation in aging. Free Radic. Biol. Med. 65, 380–401. doi:10.1016/j.freeradbiomed.2013.07.003
Erbs S., Hollriegel R., Linke A., Beck E. B., Adams V., Gielen S., et al. (2010). Exercise training in patients with advanced chronic heart failure (NYHA IIIb) promotes restoration of peripheral vasomotor function, induction of endogenous regeneration, and improvement of left ventricular function. Circ. Heart Fail 3 (4), 486–494. doi:10.1161/CIRCHEARTFAILURE.109.868992
Gamboa A., Okamoto L. E., Diedrich A., Choi L., Robertson D., Farley G., et al. (2012). Sympathetic activation and nitric oxide function in early hypertension. Am. J. Physiol. Heart Circ. Physiol. 302 (7), H1438–H1443. doi:10.1152/ajpheart.01020.2011
Ghardashi A., Izadi M. R., Rakhshan K., Mafi F., Biglari S., Gandomkar Bagheri H. (2018). Improved brachial artery shear patterns and increased flow-mediated dilatation after low-volume high-intensity interval training in type 2 diabetes. Exp. Physiol. 103 (9), 1264–1276. doi:10.1113/EP087005
Gielen S., Schuler G., Adams V. (2010). Cardiovascular effects of exercise training: Molecular mechanisms. Circulation 122 (12), 1221–1238. doi:10.1161/CIRCULATIONAHA.110.939959
Girard P. R., Nerem R. M. (1995). Shear stress modulates endothelial cell morphology and F-actin organization through the regulation of focal adhesion-associated proteins. J. Cell. Physiol. 163 (1), 179–193. doi:10.1002/jcp.1041630121
Gokce N., Keaney J. F., Hunter L. M., Watkins M. T., Menzoian J. O., Vita J. A. (2002). Risk stratification for postoperative cardiovascular events via noninvasive assessment of endothelial function: A prospective study. Circulation 105 (13), 1567–1572. doi:10.1161/01.cir.0000012543.55874.47
Goto C., Higashi Y., Kimura M., Noma K., Hara K., Nakagawa K., et al. (2003). Effect of different intensities of exercise on endothelium-dependent vasodilation in humans: Role of endothelium-dependent nitric oxide and oxidative stress. Circulation 108 (5), 530–535. doi:10.1161/01.Cir.0000080893.55729.28
Goto C., Nishioka K., Umemura T., Jitsuiki D., Sakagutchi A., Kawamura M., et al. (2007). Acute moderate-intensity exercise induces vasodilation through an increase in nitric oxide bioavailiability in humans. Am. J. Hypertens. 20 (8), 825–830. doi:10.1016/j.amjhyper.2007.02.014
Green D. J., Maiorana A., O'Driscoll G., Taylor R. (2004). Effect of exercise training on endothelium-derived nitric oxide function in humans. J. Physiol. 561 (1), 1–25. doi:10.1113/jphysiol.2004.068197
Green D. J., Spence A., Rowley N., Thijssen D. H., Naylor L. H. (2012). Vascular adaptation in athletes: Is there an 'athlete's artery. Exp. Physiol. 97 (3), 295–304. doi:10.1113/expphysiol.2011.058826
Green D. J., Hopman M. T., Padilla J., Laughlin M. H., Thijssen D. H. (2017). Vascular adaptation to exercise in humans: Role of hemodynamic stimuli. Physiol. Rev. 97 (2), 495–528. doi:10.1152/physrev.00014.2016
Gustafson B. (2010). Adipose tissue, inflammation and atherosclerosis. J. Atheroscler. Thromb. 17 (4), 332–341. doi:10.5551/jat.3939
Hajer G. R., van Haeften T. W., Visseren F. L. (2008). Adipose tissue dysfunction in obesity, diabetes, and vascular diseases. Eur. Heart J. 29 (24), 2959–2971. doi:10.1093/eurheartj/ehn387
Halverstadt A., Phares D. A., Wilund K. R., Goldberg A. P., Hagberg J. M. (2007). Endurance exercise training raises high-density lipoprotein cholesterol and lowers small low-density lipoprotein and very low-density lipoprotein independent of body fat phenotypes in older men and women. Metabolism 56 (4), 444–450. doi:10.1016/j.metabol.2006.10.019
Hambrecht R., Adams V., Erbs S., Linke A., Krankel N., Shu Y., et al. (2003). Regular physical activity improves endothelial function in patients with coronary artery disease by increasing phosphorylation of endothelial nitric oxide synthase. Circulation 107 (25), 3152–3158. doi:10.1161/01.CIR.0000074229.93804.5C
Harrison D. G., Widder J., Grumbach I., Chen W., Weber M., Searles C. (2006). Endothelial mechanotransduction, nitric oxide and vascular inflammation. J. Intern Med. 259 (4), 351–363. doi:10.1111/j.1365-2796.2006.01621.x
Haskell W. L., Lee I. M., Pate R. R., Powell K. E., Blair S. N., Franklin B. A., et al. (2007). Physical activity and public health: Updated recommendation for adults from the American college of sports medicine and the American heart association. Circulation 116 (9), 1081–1093. doi:10.1161/CIRCULATIONAHA.107.185649
Haynes A., Naylor L. H., Spence A. L., Robey E., Cox K. L., Maslen B. A., et al. (2021). Effects of land versus water walking interventions on vascular function in older adults. Med. Sci. Sports Exerc 53 (1), 83–89. doi:10.1249/MSS.0000000000002439
Higgins J. P., Altman D. G., Gotzsche P. C., Juni P., Moher D., Oxman A. D., et al. (2011). The Cochrane Collaboration's tool for assessing risk of bias in randomised trials. BMJ 343, d5928. doi:10.1136/bmj.d5928
Hill J. M., Zalos G., Halcox J. P., Schenke W. H., Waclawiw M. A., Quyyumi A. A., et al. (2003). Circulating endothelial progenitor cells, vascular function, and cardiovascular risk. N. Engl. J. Med. 348 (7), 593–600. doi:10.1056/NEJMoa022287
Huang C., Wang Y., Li X., Ren L., Zhao J., Hu Y., et al. (2020). Clinical features of patients infected with 2019 novel coronavirus in Wuhan, China. Lancet 395 (10223), 497–506. doi:10.1016/s0140-6736(20)30183-5
Hubert H. B., Feinleib M., McNamara P. M., Castelli W. P. (1983). Obesity as an independent risk factor for cardiovascular disease: A 26-year follow-up of participants in the framingham heart study. Circulation 67 (5), 968–977. doi:10.1161/01.cir.67.5.968
Inaba Y., Chen J. A., Bergmann S. R. (2010). Prediction of future cardiovascular outcomes by flow-mediated vasodilatation of brachial artery: A meta-analysis. Int. J. Cardiovasc Imaging 26 (6), 631–640. doi:10.1007/s10554-010-9616-1
Jo E. A., Wu S. S., Han H. R., Park J. J., Park S., Cho K. I. (2020). Effects of exergaming in postmenopausal women with high cardiovascular risk: A randomized controlled trial. Clin. Cardiol. 43 (4), 363–370. doi:10.1002/clc.23324
Jones L. W., Hornsby W. E., Freedland S. J., Lane A., West M. J., Moul J. W., et al. (2014). Effects of nonlinear aerobic training on erectile dysfunction and cardiovascular function following radical prostatectomy for clinically localized prostate cancer. Eur. Urol. 65 (5), 852–855. doi:10.1016/j.eururo.2013.11.009
Kajikawa M., Higashi Y. (2021). Promising assessment of vascular function for future cardiovascular events. J. Atheroscler. Thromb. 28 (11), 1123–1125. doi:10.5551/jat.ED154
Kelly A. S., Wetzsteon R. J., Kaiser D. R., Steinberger J., Bank A. J., Dengel D. R. (2004). Inflammation, insulin, and endothelial function in overweight children and adolescents: The role of exercise. J. Pediatr. 145 (6), 731–736. doi:10.1016/j.jpeds.2004.08.004
King T. J., Pyke K. E. (2020). Evidence of a limb- and shear stress stimulus profile-dependent impact of high-intensity cycling training on flow-mediated dilation. Appl. Physiol. Nutr. Metab. 45 (2), 135–145. doi:10.1139/apnm-2019-0151
Kingwell B. A., Sherrard B., Jennings G. L., Dart A. M. (1997). Four weeks of cycle training increases basal production of nitric oxide from the forearm. Am. J. Physiol. 272 (3), H1070–H1077. doi:10.1152/ajpheart.1997.272.3.H1070
Kirkman D. L., Ramick M. G., Muth B. J., Stock J. M., Pohlig R. T., Townsend R. R., et al. (2019). Effects of aerobic exercise on vascular function in nondialysis chronic kidney disease: A randomized controlled trial. Am. J. Physiology-Renal Physiology 316 (5), F898–F905. doi:10.1152/ajprenal.00539.2018
Kobayashi N., Tsuruya Y., Iwasawa T., Ikeda N., Hashimoto S., Yasu T., et al. (2003). Exercise training in patients with chronic heart failure improves endothelial function predominantly in the trained extremities. Circ. J. 67 (6), 505–510. doi:10.1253/circj.67.505
Konigstein K., Wagner J., Infanger D., Knaier R., Neve G., Klenk C., et al. (2022). Cardiorespiratory fitness and endothelial function in aging healthy subjects and patients with cardiovascular disease. Front. Cardiovasc Med. 9, 870847. doi:10.3389/fcvm.2022.870847
Koutroumpi M., Dimopoulos S., Psarra K., Kyprianou T., Nanas S. (2012). Circulating endothelial and progenitor cells: Evidence from acute and long-term exercise effects. World J. Cardiol. 4 (12), 312–326. doi:10.4330/wjc.v4.i12.312
Kwon H. R., Min K. W., Ahn H. J., Seok H. G., Lee J. H., Park G. S., et al. (2011). Effects of aerobic exercise vs. resistance training on endothelial function in women with type 2 diabetes mellitus. Diabetes & metabolism J. 35 (4), 364–373. doi:10.4093/dmj.2011.35.4.364
Landers-Ramos R. Q., Sapp R. M., Shill D. D., Hagberg J. M., Prior S. J. (2019). Exercise and cardiovascular progenitor cells. Compr. Physiol. 9 (2), 767–797. doi:10.1002/cphy.c180030
Lash J. M., Bohlen H. G. (1992). Functional adaptations of rat skeletal muscle arterioles to aerobic exercise training. J. Appl. Physiol. (1985) 72 (6), 2052–2062. doi:10.1152/jappl.1992.72.6.2052
Laughlin M. H. (1995). Endothelium-mediated control of coronary vascular tone after chronic exercise training. Med. Sci. Sports Exerc 27 (8), 1135–1144. doi:10.1249/00005768-199508000-00006
Lavrencic A., Salobir B. G., Keber I. (2000). Physical training improves flow-mediated dilation in patients with the polymetabolic syndrome. Arterioscler. Thromb. Vasc. Biol. 20 (2), 551–555. doi:10.1161/01.atv.20.2.551
Lee I. M., Shiroma E. J., Lobelo F., Puska P., Blair S. N., Katzmarzyk P. T., et al. (2012). Effect of physical inactivity on major non-communicable diseases worldwide: An analysis of burden of disease and life expectancy. Lancet 380 (9838), 219–229. doi:10.1016/s0140-6736(12)61031-9
Leon A. S., Bloor C. M. (1968). Effects of exercise and its cessation on the heart and its blood supply. J. Appl. Physiol. 24 (4), 485–490. doi:10.1152/jappl.1968.24.4.485
Li G., Lv Y., Su Q., You Q., Yu L. (2022). The effect of aerobic exercise on pulse wave velocity in middle-aged and elderly people: A systematic review and meta-analysis of randomized controlled trials. Front. Cardiovasc Med. 9, 960096. doi:10.3389/fcvm.2022.960096
Lloyd-Jones D. M., Hong Y., Labarthe D., Mozaffarian D., Appel L. J., Van Horn L., et al. (2010). Defining and setting national goals for cardiovascular health promotion and disease reduction: The American heart association's strategic impact goal through 2020 and beyond. Circulation 121 (4), 586–613. doi:10.1161/circulationaha.109.192703
Matsuzawa Y., Kwon T. G., Lennon R. J., Lerman L. O., Lerman A. (2015). Prognostic value of flow-mediated vasodilation in brachial artery and fingertip artery for cardiovascular events: A systematic review and meta-analysis. J. Am. Heart Assoc. 4 (11), e002270. doi:10.1161/jaha.115.002270
McDermott M. M., Ades P., Guralnik J. M., Dyer A., Ferrucci L., Liu K., et al. (2009). Treadmill exercise and resistance training in patients with peripheral arterial disease with and without intermittent claudication: A randomized controlled trial. JAMA 301 (2), 165–174. doi:10.1001/jama.2008.962
Meyer A. A., Kundt G., Lenschow U., Schuff-Werner P., Kienast W. (2006). Improvement of early vascular changes and cardiovascular risk factors in obese children after a six-month exercise program. J. Am. Coll. Cardiol. 48 (9), 1865–1870. doi:10.1016/j.jacc.2006.07.035
Mitranun W., Deerochanawong C., Tanaka H., Suksom D. (2014). Continuous vs interval training on glycemic control and macro- and microvascular reactivity in type 2 diabetic patients. Scand. J. Med. Sci. Sports 24 (2), e69–e76. doi:10.1111/sms.12112
Molmen-Hansen H. E., Stolen T., Tjonna A. E., Aamot I. L., Ekeberg I. S., Tyldum G. A., et al. (2012). Aerobic interval training reduces blood pressure and improves myocardial function in hypertensive patients. Eur. J. Prev. Cardiol. 19 (2), 151–160. doi:10.1177/1741826711400512
Newcomer S. C., Thijssen D. H., Green D. J. (2011). Effects of exercise on endothelium and endothelium/smooth muscle cross talk: Role of exercise-induced hemodynamics. J. Appl. Physiol. (1985) 111 (1), 311–320. doi:10.1152/japplphysiol.00033.2011
Newsom S. A., Everett A. C., Hinko A., Horowitz J. F. (2013). A single session of low-intensity exercise is sufficient to enhance insulin sensitivity into the next day in obese adults. Diabetes Care 36 (9), 2516–2522. doi:10.2337/dc12-2606
Norton K., Norton L., Sadgrove D. (2010). Position statement on physical activity and exercise intensity terminology. J. Sci. Med. Sport 13 (5), 496–502. doi:10.1016/j.jsams.2009.09.008
Notarius C. F., Millar P. J., Floras J. S. (2015). Muscle sympathetic activity in resting and exercising humans with and without heart failure. Appl. Physiol. Nutr. Metab. 40 (11), 1107–1115. doi:10.1139/apnm-2015-0289
O'Brien M. W., Johns J. A., Robinson S. A., Bungay A., Mekary S., Kimmerly D. S. (2020). Impact of high-intensity interval training, moderate-intensity continuous training, and resistance training on endothelial function in older adults. Med. Sci. Sports Exerc 52 (5), 1057–1067. doi:10.1249/MSS.0000000000002226
Page M. J., McKenzie J. E., Bossuyt P. M., Boutron I., Hoffmann T. C., Mulrow C. D., et al. (2021). The PRISMA 2020 statement: An updated guideline for reporting systematic reviews. Bmj 372, n71. doi:10.1136/bmj.n71
Pi-Sunyer F. X., Becker D. M., Bouchard C., Carleton R. A., Colditz G. A., Dietz W. H., et al. (1998). Clinical guidelines on the identification, evaluation, and treatment of overweight and obesity in adults: Executive summary. Expert panel on the identification, evaluation, and treatment of overweight in adults. Am. J. Clin. Nutr. 68 (4), 899–917. doi:10.1093/ajcn/68.4.899
Pierce G. L., Eskurza I., Walker A. E., Fay T. N., Seals D. R. (2011). Sex-specific effects of habitual aerobic exercise on brachial artery flow-mediated dilation in middle-aged and older adults. Clin. Sci. (Lond) 120 (1), 13–23. doi:10.1042/CS20100174
Prior B. M., Lloyd P. G., Yang H. T., Terjung R. L. (2003). Exercise-induced vascular remodeling. Exerc Sport Sci. Rev. 31 (1), 26–33. doi:10.1097/00003677-200301000-00006
Pugh C. J. A., Sprung V. S., Kemp G. J., Richardson P., Shojaee-Moradie F., Umpleby A. M., et al. (2014). Exercise training reverses endothelial dysfunction in nonalcoholic fatty liver disease. Am. J. Physiology-Heart Circulatory Physiology 307 (9), H1298–H1306. doi:10.1152/ajpheart.00306.2014
Ramos J. S., Dalleck L. C., Tjonna A. E., Beetham K. S., Coombes J. S. (2015). The impact of high-intensity interval training versus moderate-intensity continuous training on vascular function: A systematic review and meta-analysis. Sports Med. 45 (5), 679–692. doi:10.1007/s40279-015-0321-z
Ras R. T., Streppel M. T., Draijer R., Zock P. L. (2013). Flow-mediated dilation and cardiovascular risk prediction: A systematic review with meta-analysis. Int. J. Cardiol. 168 (1), 344–351. doi:10.1016/j.ijcard.2012.09.047
Rathod K. S., Kapil V., Velmurugan S., Khambata R. S., Siddique U., Khan S., et al. (2017). Accelerated resolution of inflammation underlies sex differences in inflammatory responses in humans. J. Clin. Invest. 127 (1), 169–182. doi:10.1172/jci89429
Seals D. R., Nagy E. E., Moreau K. L. (2019). Aerobic exercise training and vascular function with ageing in healthy men and women. J. Physiol. 597 (19), 4901–4914. doi:10.1113/JP277764
Sessa W. C., Pritchard K., Seyedi N., Wang J., Hintze T. H. (1994). Chronic exercise in dogs increases coronary vascular nitric oxide production and endothelial cell nitric oxide synthase gene expression. Circ. Res. 74 (2), 349–353. doi:10.1161/01.res.74.2.349
Shamseer L., Moher D., Clarke M., Ghersi D., Liberati A., Petticrew M., et al. (2015). Preferred reporting items for systematic review and meta-analysis protocols (PRISMA-P) 2015: Elaboration and explanation. BMJ 350, g7647. doi:10.1136/bmj.g7647
Shenouda N., Gillen J. B., Gibala M. J., MacDonald M. J. (2017). Changes in brachial artery endothelial function and resting diameter with moderate-intensity continuous but not sprint interval training in sedentary men. J. Appl. PHYSIOLOGY 123 (4), 773–780. doi:10.1152/japplphysiol.00058.2017
Sherwood A., Blumenthal J. A., Smith P. J., Watkins L. L., Hoffman B. M., Hinderliter A. L. (2016). Effects of exercise and sertraline on measures of coronary heart disease risk in patients with major depression: Results from the SMILE-II randomized clinical trial. Psychosom. Med. 78 (5), 602–609. doi:10.1097/psy.0000000000000301
Sixt S., Rastan A., Desch S., Sonnabend M., Schmidt A., Schuler G., et al. (2008). Exercise training but not rosiglitazone improves endothelial function in prediabetic patients with coronary disease. Eur. J. Cardiovasc. Prev. rehabilitation 15 (4), 473–478. official journal of the European Society of Cardiology, Working Groups on Epidemiology & Prevention and Cardiac Rehabilitation and Exercise Physiology. doi:10.1097/HJR.0b013e3283002733
Skaug E. A., Aspenes S. T., Oldervoll L., Mørkedal B., Vatten L., Wisløff U., et al. (2013). Age and gender differences of endothelial function in 4739 healthy adults: The HUNT3 fitness study. Eur. J. Prev. Cardiol. 20 (4), 531–540. doi:10.1177/2047487312444234
Sprung V. S., Cuthbertson D. J., Pugh C. J., Aziz N., Kemp G. J., Daousi C., et al. (2013). Exercise training in polycystic ovarian syndrome enhances flow-mediated dilation in the absence of changes in fatness. Med. Sci. Sports Exerc 45 (12), 2234–2242. doi:10.1249/MSS.0b013e31829ba9a1
St-Pierre J., Drori S., Uldry M., Silvaggi J. M., Rhee J., Jäger S., et al. (2006). Suppression of reactive oxygen species and neurodegeneration by the PGC-1 transcriptional coactivators. Cell. 127 (2), 397–408. doi:10.1016/j.cell.2006.09.024
Steinberg H. O., Brechtel G., Johnson A., Fineberg N., Baron A. D. (1994). Insulin-mediated skeletal muscle vasodilation is nitric oxide dependent. A novel action of insulin to increase nitric oxide release. J. Clin. Invest. 94 (3), 1172–1179. doi:10.1172/JCI117433
Sun D., Huang A., Koller A., Kaley G. (1994). Short-term daily exercise activity enhances endothelial NO synthesis in skeletal muscle arterioles of rats. J. Appl. Physiol. (1985) 76 (5), 2241–2247. doi:10.1152/jappl.1994.76.5.2241
Swift D. L., Earnest C. P., Blair S. N., Church T. S. (2012). The effect of different doses of aerobic exercise training on endothelial function in postmenopausal women with elevated blood pressure: Results from the DREW study. Br. J. Sports Med. 46 (10), 753–758. doi:10.1136/bjsports-2011-090025
Taddei S., Galetta F., Virdis A., Ghiadoni L., Salvetti G., Franzoni F., et al. (2000). Physical activity prevents age-related impairment in nitric oxide availability in elderly athletes. Circulation 101 (25), 2896–2901. doi:10.1161/01.cir.101.25.2896
Tanaka A., Tomiyama H., Maruhashi T., Matsuzawa Y., Miyoshi T., Kabutoya T., et al. (2018). Physiological diagnostic criteria for vascular failure. Hypertension 72 (5), 1060–1071. doi:10.1161/HYPERTENSIONAHA.118.11554
Thijssen D. H., Maiorana A. J., O'Driscoll G., Cable N. T., Hopman M. T., Green D. J. (2010). Impact of inactivity and exercise on the vasculature in humans. Eur. J. Appl. Physiol. 108 (5), 845–875. doi:10.1007/s00421-009-1260-x
Thijssen D. H., Black M. A., Pyke K. E., Padilla J., Atkinson G., Harris R. A., et al. (2011). Assessment of flow-mediated dilation in humans: A methodological and physiological guideline. Am. J. Physiol. Heart Circ. Physiol. 300 (1), H2–H12. doi:10.1152/ajpheart.00471.2010
Thijssen D. H. J., Bruno R. M., van Mil A., Holder S. M., Faita F., Greyling A., et al. (2019). Expert consensus and evidence-based recommendations for the assessment of flow-mediated dilation in humans. Eur. Heart J. 40 (30), 2534–2547. doi:10.1093/eurheartj/ehz350
Timmis A., Townsend N., Gale C., Grobbee R., Maniadakis N., Flather M., et al. (2018). European society of cardiology: Cardiovascular disease statistics 2017. Eur. Heart J. 39 (7), 508–579. doi:10.1093/eurheartj/ehx628
Tinken T. M., Thijssen D. H., Hopkins N., Dawson E. A., Cable N. T., Green D. J. (2010). Shear stress mediates endothelial adaptations to exercise training in humans. Hypertension 55 (2), 312–318. doi:10.1161/hypertensionaha.109.146282
Tjønna A. E., Lee S. J., Rognmo Ø., Stølen T. O., Bye A., Haram P. M., et al. (2008). Aerobic interval training versus continuous moderate exercise as a treatment for the metabolic syndrome: A pilot study. Circulation 118 (4), 346–354. doi:10.1161/CIRCULATIONAHA.108.772822
Tjønna A. E., Rognmo Ø., Bye A., Stølen T. O., Wisløff U. (2011). Time course of endothelial adaptation after acute and chronic exercise in patients with metabolic syndrome. J. strength Cond. Res. 25 (9), 2552–2558. doi:10.1519/JSC.0b013e3181fb4809
Tornero-Aguilera J. F., Jimenez-Morcillo J., Rubio-Zarapuz A., Clemente-Suárez V. J. (2022). Central and peripheral fatigue in physical exercise explained: A narrative review. Int. J. Environ. Res. Public Health 19 (7), 3909. doi:10.3390/ijerph19073909
Townsend N., Nichols M., Scarborough P., Rayner M. (2015). Cardiovascular disease in Europe--epidemiological update 2015. Eur. Heart J. 36 (40), 2696–2705. doi:10.1093/eurheartj/ehv428
Tsukiyama Y., Ito T., Nagaoka K., Eguchi E., Ogino K. (2017). Effects of exercise training on nitric oxide, blood pressure and antioxidant enzymes. J. Clin. Biochem. Nutr. 60 (3), 180–186. doi:10.3164/jcbn.16-108
Ungvari Z., Tarantini S., Donato A. J., Galvan V., Csiszar A. (2018). Mechanisms of vascular aging. Circ. Res. 123 (7), 849–867. doi:10.1161/circresaha.118.311378
Vandercappellen E. J., Koster A., Savelberg H., Eussen S., Dagnelie P. C., Schaper N. C., et al. (2022). Sedentary behaviour and physical activity are associated with biomarkers of endothelial dysfunction and low-grade inflammation-relevance for (pre)diabetes: The Maastricht Study. Diabetologia 65 (5), 777–789. doi:10.1007/s00125-022-05651-3
Vona M., Rossi A., Capodaglio P., Rizzo S., Servi P., De Marchi M., et al. (2004). Impact of physical training and detraining on endothelium-dependent vasodilation in patients with recent acute myocardial infarction. Am. Heart J. 147 (6), 1039–1046. doi:10.1016/j.ahj.2003.12.023
Vona M., Codeluppi G. M., Iannino T., Ferrari E., Bogousslavsky J., von Segesser L. K. (2009). Effects of different types of exercise training followed by detraining on endothelium-dependent dilation in patients with recent myocardial infarction. Circulation 119 (12), 1601–1608. doi:10.1161/CIRCULATIONAHA.108.821736
Vonderwalde I., Kovacs-Litman A. (2018). Aerobic exercise promotes hippocampal neurogenesis through skeletal myofiber-derived vascular endothelial growth factor. J. Physiol. 596 (5), 761–763. doi:10.1113/JP275582
Westhoff T. H., Franke N., Schmidt S., Vallbracht-Israng K., Meissner R., Yildirim H., et al. (2007). Too old to benefit from sports? The cardiovascular effects of exercise training in elderly subjects treated for isolated systolic hypertension. Kidney & blood Press. Res. 30 (4), 240–247. doi:10.1159/000104093
Westhoff T. H., Schmidt S., Gross V., Joppke M., Zidek W., van der Giet M., et al. (2008). The cardiovascular effects of upper-limb aerobic exercise in hypertensive patients. J. Hypertens. 26 (7), 1336–1342. doi:10.1097/HJH.0b013e3282ffac13
Wisløff U., Støylen A., Loennechen J. P., Bruvold M., Rognmo Ø., Haram P. M., et al. (2007). Superior cardiovascular effect of aerobic interval training versus moderate continuous training in heart failure patients: A randomized study. Circulation 115 (24), 3086–3094. doi:10.1161/CIRCULATIONAHA.106.675041
Wu N. N., Tian H., Chen P., Wang D., Ren J., Zhang Y. (2019). Physical exercise and selective autophagy: Benefit and risk on cardiovascular health. Cells 8 (11), 1436. doi:10.3390/cells8111436
Wyatt H. L., Mitchell J. (1978). Influences of physical conditioning and deconditioning on coronary vasculature of dogs. J. Appl. Physiol. Respir. Environ. Exerc Physiol. 45 (4), 619–625. doi:10.1152/jappl.1978.45.4.619
Wycherley T. P., Brinkworth G. D., Noakes M., Buckley J. D., Clifton P. M. (2008). Effect of caloric restriction with and without exercise training on oxidative stress and endothelial function in obese subjects with type 2 diabetes. Diabetes Obes. Metab. 10 (11), 1062–1073. doi:10.1111/j.1463-1326.2008.00863.x
Xu G. F., Zhang J. Y., Pan H. T., Tian S., Liu M. E., Yu T. T., et al. (2014a). Cardiovascular dysfunction in offspring of ovarian-hyperstimulated women and effects of estradiol and progesterone: A retrospective cohort study and proteomics analysis. J. Clin. Endocrinol. Metab. 99 (12), E2494–E2503. doi:10.1210/jc.2014-2349
Xu Y., Arora R. C., Hiebert B. M., Lerner B., Szwajcer A., McDonald K., et al. (2014b). Non-invasive endothelial function testing and the risk of adverse outcomes: A systematic review and meta-analysis. Eur. Heart J. Cardiovasc Imaging 15 (7), 736–746. doi:10.1093/ehjci/jet256
Yilmaz M. I., Romano M., Basarali M. K., Elzagallaai A., Karaman M., Demir Z., et al. (2020). The effect of corrected inflammation, oxidative stress and endothelial dysfunction on FMD levels in patients with selected chronic diseases: A quasi-experimental study. Sci. Rep. 10 (1), 9018. doi:10.1038/s41598-020-65528-6
Yoshizawa M., Maeda S., Miyaki A., Misono M., Choi Y., Shimojo N., et al. (2010). Additive beneficial effects of lactotripeptides intake with regular exercise on endothelium-dependent dilatation in postmenopausal women. Am. J. Hypertens. 23 (4), 368–372. doi:10.1038/ajh.2009.270
You Q., Yu L., Li G., He H., Lv Y. (2021). Effects of different intensities and durations of aerobic exercise on vascular endothelial function in middle-aged and elderly people: A meta-analysis. Front. Physiol. 12, 803102. doi:10.3389/fphys.2021.803102
Zhang S., Zhen K., Su Q., Chen Y., Lv Y., Yu L. (2022). The effect of aerobic exercise on cognitive function in people with alzheimer's disease: A systematic review and meta-analysis of randomized controlled trials. Int. J. Environ. Res. Public Health 19 (23), 15700. doi:10.3390/ijerph192315700
Keywords: continuous aerobic exercise, endothelial function, FMD, systematic review, meta-analysis
Citation: Tao X, Chen Y, Zhen K, Ren S, Lv Y and Yu L (2023) Effect of continuous aerobic exercise on endothelial function: A systematic review and meta-analysis of randomized controlled trials. Front. Physiol. 14:1043108. doi: 10.3389/fphys.2023.1043108
Received: 13 September 2022; Accepted: 01 February 2023;
Published: 10 February 2023.
Edited by:
Nevena Jeremic, University of Kragujevac, SerbiaReviewed by:
Noelia Gonzalez-Galvez, Independent Researcher, Murcia, SpainFerdinando Iellamo, University of Rome Tor Vergata, Italy
Copyright © 2023 Tao, Chen, Zhen, Ren, Lv and Yu. This is an open-access article distributed under the terms of the Creative Commons Attribution License (CC BY). The use, distribution or reproduction in other forums is permitted, provided the original author(s) and the copyright owner(s) are credited and that the original publication in this journal is cited, in accordance with accepted academic practice. No use, distribution or reproduction is permitted which does not comply with these terms.
*Correspondence: Yuanyuan Lv, sunflowerlyy@bsu.edu.cn; Laikang Yu, yulaikang@126.com
†These authors have contributed equally to this work