- 1Obesity Center CGG, Erasmus MC, University Medical Center Rotterdam, Rotterdam, Netherlands
- 2Department of Internal Medicine, Division of Endocrinology, Erasmus MC, University Medical Center Rotterdam, Rotterdam, Netherlands
- 3Groningen Institute for Evolutionary Life Sciences, University of Groningen, Groningen, Netherlands
- 4Department of Clinical Chemistry, Erasmus MC, University Medical Center Rotterdam, Rotterdam, Netherlands
Background: Weight loss can induce changes in appetite-regulating hormone levels, possibly linked to increases in appetite and weight regain. However, hormonal changes vary across interventions. Here, we studied levels of appetite-regulating hormones during a combined lifestyle intervention (CLI: healthy diet, exercise and cognitive behavioral therapy).
Methods: We measured levels of long-term adiposity-related hormones (leptin, insulin, high-molecular-weight (HMW) adiponectin) and short-term appetite hormones (PYY, cholecystokinin, gastric-inhibitory polypeptide, pancreatic polypeptide, FGF21, AgRP) in overnight-fasted serum of 39 patients with obesity. Hormone levels were compared between T0 (baseline), T1 (after 10 weeks) and T2 (end of treatment, 1.5 years). T0-T1 hormone changes were correlated with T1-T2 anthropometric changes.
Results: Initial weight loss at T1 was maintained at T2 (−5.0%, p < 0.001), and accompanied by decreased leptin and insulin levels at T1 and T2 (all p < 0.05) compared to T0. Most short-term signals were not affected. Only PP levels were decreased at T2 compared to T0 (p < 0.05). Most changes in hormone levels during initial weight loss did not predict subsequent changes in anthropometrics, except for T0-T1 decreases in FGF21 levels and T0-T1 increases in HMW adiponectin levels tended to be associated with larger T1-T2 increases in BMI (p < 0.05 and p = 0.05, respectively).
Conclusion: CLI-induced weight loss was associated with changes in levels of long-term adiposity-related hormones towards healthy levels, but not with orexigenic changes in most short-term appetite signals. Our data indicates that the clinical impact of alterations in appetite-regulating hormones during modest weight loss remains questionable. Future studies should investigate potential associations of weight-loss-induced changes in FGF21 and adiponectin levels with weight regain.
1 Introduction
The increasing prevalence of obesity [body-mass-index (BMI) ≥30.0 kg/m2] challenges healthcare providers worldwide to counteract the disease (GBD 2015 Obesity Collaborators, 2017; Chooi et al., 2019). When trying to achieve long-lasting treatment success, the mode of weight loss intervention might be essential. In clinical practice, very-low-energy diets are commonly used as they can induce substantial weight loss in a short amount of time (Mustajoki and Pekkarinen, 2001). However, hypocaloric diets are often accompanied by increases in self-reported appetite and followed by weight regain, making long-term weight loss maintenance a critical issue in obesity treatment (Mustajoki and Pekkarinen, 2001; Sumithran et al., 2011). An alternative approach could be to induce a more modest, but sustainable weight loss via multidisciplinary treatment.
This commonly observed recidivism in response to diet-induced weight loss has been partly attributed to a physiological compensatory ‘starvation’ response, ranging from a more orexigenic (appetite-inducing) hormonal profile to increased metabolic efficiency favoring energy storage (Sumithran et al., 2011; Sumithran and Proietto, 2013; Greenway, 2015; Lean and Malkova, 2016). Amongst others, this compensatory mechanism may be reflected by normalized levels of long-term adiposity-related hormones (decreases in leptin and insulin levels; increases in adiponectin levels). Although these normalizations indicate metabolic improvements (Haffner et al., 1992; Zhu et al., 2010; Leon-Cabrera et al., 2013), they may favor hunger signaling, decreased energy expenditure and increased energy storage into subcutaneous fat (Kim et al., 2007; Sumithran and Proietto, 2013; Greenway, 2015). Additional contributors may be found in short-term regulators of energy balance which control appetite and metabolism by regulating inter-meal interval (satiety; fasting levels) and meal-termination (satiation; postprandial response) (Lean and Malkova, 2016; Steinert et al., 2017). Important actors in this context include orexigenic hypothalamus-derived agouti-related protein (AgRP), the anorexigenic gut peptides cholecystokinin (CCK), peptide tyrosine-tyrosine (PYY) and pancreatic polypeptide (PP) (Rossi et al., 1998; Sumithran et al., 2011; Sumithran and Proietto, 2013; Lean and Malkova, 2016). In addition, fibroblast growth factor-21 (FGF21) and gastric-inhibitory polypeptide (GIP) are important regulators of glucose metabolism and insulin sensitivity (Seino and Yabe, 2013; Talukdar et al., 2016; Jastreboff et al., 2022).
Normalizations of the long-term adiposity-related hormones leptin, insulin and (HMW) adiponectin levels have been described consistently across different weight loss modalities (Lean and Malkova, 2016; Khosravi-Largani et al., 2019). However, findings are less consistent for short-term regulators of energy balance (Lean and Malkova, 2016). Hypocaloric (very-low-energy) diets seem to induce weight-regain-favoring hormonal alterations (Essah et al., 2010; Sumithran et al., 2011; Greenway, 2015; Lean and Malkova, 2016). In contrast, results from exercise intervention studies rather point towards increased satiety signaling (Lean and Malkova, 2016; Sheikholeslami-Vatani and Rostamzadeh, 2022). A study investigating changes in response to a lifestyle intervention combining a hypocaloric diet and exercise showed no effects on fasting PYY or CCK levels (Coutinho et al., 2018). Thus, weight-loss-induced alterations in fasting levels of appetite-regulating hormones may reflect chronic changes in meal-to-meal appetite regulation which likely vary across interventions and may be relevant in the context of long-term weight-loss-maintenance (Lean and Malkova, 2016).
To our knowledge, no study has yet investigated the changes in levels of appetite-regulating hormones in response to a healthy diet (whereby patients are instructed not to follow a low-calorie scheme); combined with exercise and cognitive behavioral therapy (CBT). Moreover, the clinical impact of the proposed weight-loss-induced compensatory mechanism has recently been questioned. Others have noted that previous studies did not clearly demonstrate a link between the shift towards a theoretically orexigenic hormonal disposition and subsequent weight regain (Sumithran et al., 2011; Strohacker et al., 2014; Coutinho et al., 2018; Martins et al., 2020). The aim of this study was to describe changes in serum levels of hormonal long-term and short-term regulators of energy balance during a 1.5-year combined lifestyle intervention (CLI) for obesity which comprises a healthy (‘normocaloric’) diet, exercise and CBT. We also investigated whether changes in hormone levels after initial weight loss may predict subsequent changes in anthropometrics.
2 Methods
2.1 Participants
The study subjects included adult patients with lifestyle-induced obesity who underwent a multidisciplinary CLI between October 2013 and October 2019. The treatment program was provided by the Obesity Center CGG (‘Centrum Gezond Gewicht’) at Erasmus MC, University Medical Center Rotterdam. Exclusion criteria were other causes of obesity (e.g. genetic or endocrine diseases), inability to speak Dutch, intellectual disability (IQ < 80), current wish for pregnancy and severe physiological or behavioral problems impeding functioning in a group. An inclusion criterion was the presence of ≥1 obesity-related comorbidity (e.g., dyslipidemia, hypertension, non-alcoholic fatty liver disease, type 2 diabetes, obstructive sleep apnea or osteoarthritis). Before starting a trajectory, patients were screened by a medical doctor, a dietician, a physical therapist, and a psychologist. If inclusion criteria were met and no factors necessitating additional treatment were detected, patients were enrolled in the program. All participants provided written informed consent before the start. The study protocol was approved by the local medical ethics committee of the Erasmus MC Rotterdam (MEC 2012–257).
2.2 Combined lifestyle intervention (CLI)
Twice a year groups of 10–12 participants started a 1.5-year CLI trajectory. Every group session comprised 1.5 h of combined CBT and nutritional advice which were followed by 1.5 h by exercise sessions. A detailed description is provided in Table 1.
Evaluation moments were at baseline (before the start of the program, T0), after the first 10 weeks of (intensive) treatment (T1) and at 1.5 years at the end of the program (T2). At each time point, anthropometrics and metabolic parameters were assessed for clinical care and additional fasting blood samples were drawn for research purposes. The present analysis represents a retrospective analysis of all available follow-up serum samples in March 2020.
2.3 Hormone measurements
For 45 patients, blood serum samples were available at follow-up. The samples were stored after centrifugation at −20 or −80°C for a maximum duration of 7 years. Commercially available enzyme-linked immunosorbent assay (ELISA) kits were used to determine levels of leptin (Mediagnost, Reutlingen, Germany), total PYY, PP, GIP, FGF21 (Millipore Corporation, Billerica, MA, United States) as well as AgRP and CCK (Sigma Aldrich, St. Louis, MO, United States). HMW adiponectin was determined using a chemiluminescent enzyme immunoassay (CLEIA, Lumipulse G1200, Fujirebio, Gent, Belgium). All analyses for leptin, PYY, GIP, FGF21, AgRP, CCK and HMW adiponectin were performed at the same time at the same laboratory at the end of the study. For each patient, samples from all time points were analyzed in the same plate to prevent inter-assay bias. Insulin was measured as part of standard clinical care using a commercially available CLEIA kit for in vitro diagnostics immediately after blood drawing (Lumipulse G1200, Fujirebio, Gent, Belgium). Part of this insulin data has been reported in previous articles of our group (e.g. (van der Zalm et al., 2020).
2.4 Statistical analysis
All statistical analyses were performed using SPSS version 24 (IBM Corp. 2019). If hormone levels were below the detectable range of the ELISA analyses, missing values were replaced by the limit of detection stated in the protocol. This occurred for PYY (one sample at T2), and PP (two samples at T0, three samples at T1, three samples at T2). To detect within-subject changes across the three time points, repeated-measures ANOVAs with Bonferroni-corrected post hoc t-tests were used or Friedman’s test with Bonferroni-corrected post hoc Wilcoxon-signed rank tests in case of non-normality. Data is reported as means ± standard error (SEM) or, if not normally distributed, median and interquartile range (IQR). Chi-squared test of independence was used to test for sex differences in proportions of responder status at T1 and T2. Percentage changes of all outcomes were computed using the following formula:
3 Results
3.1 Baseline sample characteristics
Of the 45 patients for whom follow-up blood samples were available, six were excluded: two patients were not fasting at blood drawing, one patient became pregnant, one was later diagnosed to have a genetic form of obesity, one patient underwent bariatric surgery and one developed a severe recurring pancreatitis. Thus, we included a total of 39 patients (31 women, 79.5%) who had a mean BMI of 40.6 kg/m2 (±0.9 SEM) and a mean age of 41.8 years (±2.1 SEM). Details are provided in Table 2.
3.2 Changes in anthropometrics in response to CLI
After 10 weeks (T1), patients showed a mean weight loss of −6.1 kg (5.1%) which was maintained after 1.5 years of treatment (T2, -6.0 kg; −5.0%). This was accompanied bya mean decrease in waist circumference (WC) of −6.8 cm (−5.8%) at T1 and -6.1 cm (−5.2%) at T2 (all p < 0.001). In addition, we saw improvements in the patients’ blood lipid profile (see Table 2). At T1, 19 patients (48.7%) lost ≥5% weight (‘responder’, of whom 16 were women) and at T2 there were 20 (51.3%) responders (of whom 17 were women). There was no sex effect on responder status at T1 (p = 0.378) or T2 (p = 0.317).
3.3 Changes in levels of appetite-regulating hormones in response to CLI
We investigated changes in hormone levels across the three measurement time points. In Figure 1A, we show that levels of the long-term adiposity-related hormones leptin and insulin were decreased at T1 (n = 39, 33.4 ng/ml ±2.4 SEM, p < 0.001; n = 37, 108 pmol/l [81–147 IQR], p = 0.011, respectively) as well as at T2 (39.7 ng/ml ± 2.6 SEM, p = 0.009; 124 pmol/l [74–157 IQR], p = 0.013, respectively) compared to baseline (45.8 ng/ml ± 2.5 SEM, 141 pmol/l [95–216 IQR], respectively). Leptin levels significantly increased between T1 and T2 (p = 0.004), which was not observed for insulin levels (p > 0.05). At all three time points, leptin and insulin levels of many patients remained above the normal reference ranges used for clinical diagnostics at the Erasmus MC. Specifically, hyperleptinemia (1.5–33.9 ng/ml for women, 0.2–12.9 ng/ml for men) was observed in 34 patients (87.2%) at T0, in 21 patients (53.8%) at T1 and in 27 patients (69.2%) at T2. Similarly, a total of 23 patients (62.2%) had hyperinsulinemia (>100 pmol/l) at T0, 18 (48.6%) at T1 and 19 patients (51.4%) at T2. HMW adiponectin levels did not change significantly between T0 and T1 (n = 39, 3.66 μg/ml [1.65–4.67 IQR] vs 3.05 μg/ml [1.77–4.50 IQR], respectively), but were increased at T2 (3.32 μg/ml [1.86–5.23 IQR]) compared to T1 (p = 0.003).
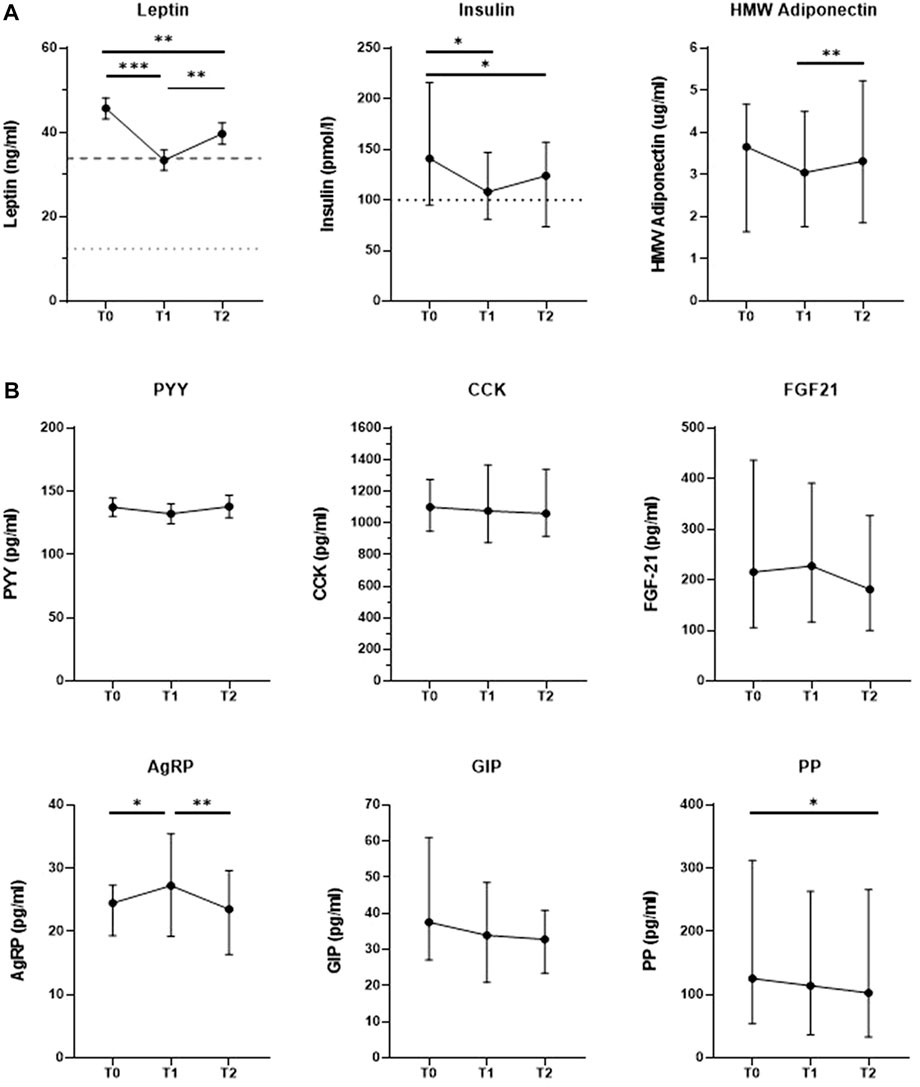
FIGURE 1. Changes in in levels of hormones regulating appetite and energy homeostasis in response to a combined lifestyle intervention from baseline (T)) to 10 weeks (T1) and 1.5 years of treatment (T2). (A) Changes in levels of long-term adiposity-related hormones, including decreases in leptin and insulin levels towards normal levels. Cut-off lines: Upper limit of reference range for healthy populations (dotted lower line: males: 0.2–12.4 ng/ml; dashed upper line: females: 1.5–33.9 ng/ml), as used for clinical diagnostics in the Erasmus MC. Cut-off line insulin: Upper limit of reference range for healthy populations (≤ 100 pmol/l for both sexes) as used for clinical diagnostics in the Erasmus MC. N-37–39. Data are expressed as mean+/-SD SEM (leptin) or median (IQR) (insulin, HMW adiponectin), depending on normal distribution. HMW Adiponectin = High molecular weight adiponectin./. (B) No change in levels of most short-term regulators of energy balance at the end of CLI. PYY = Peptide tyrosine-tyrosine-tyrosine, CCK = Cholecystokinin, FGF21 = Fibroblast growth factor 21, AgRP = Agouti-related protein, GIP = Gastric-inhibitory polypeptide, PP = Pancreatic polypeptide. Data are expressed as mean+/-SD SEM (PYY) or median (IQR) (PP, AgRP, GIP, PYY, CCK, FGF-21), depending on normal distribution. *p < .05, **p < .01, ***p < .001 after repeated-measures ANOVA with Bonferroni-corrected post hoc test or Fridman’s test with Bonferroni-corrected post hoc tests in case of non-normality. N = 38–39.
As depicted in Figure 1B, levels of most short-term regulators of energy balance did not change significantly in response to the intervention except for PP and AgRP. PP levels were significantly decreased at T2 (n = 38, 102.75 pg/ml [32.90–265.95 IQR]) compared to T0 (125.65 pg/ml [54.28–312.33 IQR], p = 0.049). AgRP levels increased significantly between T0 (n = 38, 24.50 pg/ml [19.33–27.29 IQR]) and T1 (27.25 pg/ml [19.24–35.43 IQR], p = 0.009), but decreased again between T1 and T2 (23.44 pg/ml [16.76–30.66 IQR], p = 0.012) so that AgRP levels did not significantly differ from baseline after 1.5 years of treatment (p < 0.05). We did not find significant between-time-point-differences for PYY, GIP, CCK or FGF21 levels (all p > 0.05).
Changes in anthropometrics were associated with changes in leptin, insulin and HMW adiponectin at the end of the program (see Supplementary Table S1). A sensitivity analysis including only women showed results closely paralleling those of the full group (see Supplementary Figure S2).
3.4 Associations of initial hormone changes (T0-T1) with subsequent changes in anthropometrics (T1-T2)
Table 3 shows the associations of initial hormone changes (%∆ T0-T1) with subsequent changes in BMI and WC (%∆ T1-T2) before and after correction for sex and age. In the crude analysis, initial decreases in insulin, FGF21 as well as PYY levels were associated with subsequent increases in BMI (p < 0.05). Initial increases in HMW adiponectin levels tended to be associated with subsequent increases in BMI, although this association did not reach statistical significance (p = 0.070). We did not see significant associations between initial hormone level changes and subsequent changes in WC.
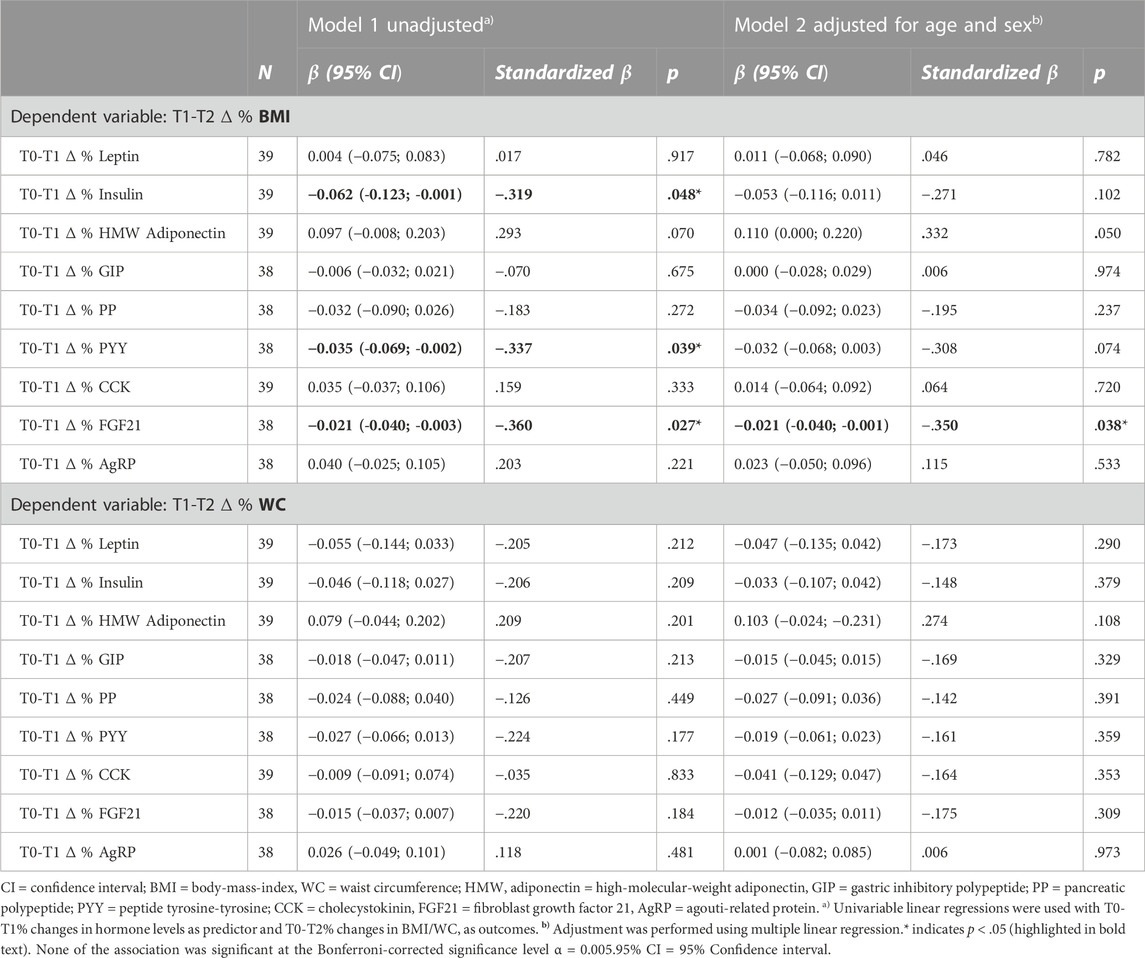
TABLE 3. Associations between initial (T0-T1) % changes in hormone levels and subsequent (T1-T2) % changes in anthropometrics.
After correction for sex and age, only the association of T0-T1% decrease in FGF21 levels with T1-T2% increase in BMI remained significant (p < 0.05). T0-T1% increase in HMW adiponectin was in trend associated with subsequently higher T1-T2% increase in BMI (p = 0.05). No other associations were observed between initial (T0-T1) % change in hormone levels and subsequent (T1-T2) % change in BMI or WC (see Table 3). Results did not change notably after an additional sensitivity analysis, correcting for T0-T1%∆ in BMI or WC respectively (see Supplementary Table S3). Neither did the results change notably after a sensitivity analysis including only women (see Supplementary Table S4). When applying the Bonferroni-corrected significance level of p < 0.0055, none of the associations remained statistically significant.
4 Discussion
Here, we describe changes in fasting levels of long-term and short-term regulators of energy homeostasis in response to a CLI combining a healthy normocalorc diet, exercise and cognitive behavioral therapy. We also addressed the question whether changes in hormonal regulators of energy balance during initial weight loss (T0-T1) predict subsequent changes in anthropometrics (T1-T2).
We found that the modest CLI-induced weight loss (≈5%) (Jensen et al., 2014) was accompanied by changes in levels of long-term adiposity-related hormones (leptin, insulin and HMW adiponectin) towards normal values. Importantly, after 1.5 years, levels of most short-term regulators of energy homeostasis were not altered, except for a decrease in fasting PP levels. When corrected for sex and age, most hormonal changes during initial weight loss did not predict subsequent changes in anthropometrics. Only initial decreases in FGF21 levels and increases in HMW adiponectin levels tended to be associated with larger subsequent increases in BMI.
The observed decrease in leptin and insulin levels, along with the (small) increase in HMW adiponectin levels are likely beneficial for metabolic health (Haffner et al., 1992; Kim et al., 2007; Zhu et al., 2010; Leon-Cabrera et al., 2013). Reductions in anorexigenic leptin and insulin levels may be interpreted as potentially hunger-inducing (Sumithran et al., 2011; Sumithran and Proietto, 2013). However, they may also indicate reduced leptin and insulin resistance, both of which are reflected by high peripheral hormone levels and are associated with metabolic dysfunction as well as disrupted brain appetite signaling (Matheny et al., 2011; Könner and Brüning, 2012; Heni et al., 2014). Consequently, the observed reductions may reflect improved central hormone sensitivity, and thus improved long-term appetite regulation. Indeed, we did see improved whole-body insulin sensitivity at the end of the program, as indicated by decreased HOMA-IR (see Table 1). At any rate, neither initial changes in leptin nor insulin predicted weight regain in the corrected model, suggesting that their influence on long-term weight management was, if present, very limited. It must be noted that throughout the whole intervention, levels of leptin and insulin remained above the normal reference ranges in many of the patients. This is likely due to the fact that, despite weight loss, these patients still had obesity.
At the end of the program, levels of most short-term regulators of energy homeostasis (PYY, CCK, GIP, AgRP and FGF21) were not significantly different from baseline, indicating that there were no major long-term alterations towards a potentially weight regain-inducing disposition. These results partly oppose findings regarding hypocaloric diets, most prominently including decreased PYY and CCK levels (Essah et al., 2010; Sumithran et al., 2011; Sumithran et al., 2013). However, our results are in line with previous studies regarding interventions comprising either increased physical activity/exercise or combinations of exercise and dietary advice (but excluding very-low-energy diets). These studies have reported unaltered levels or even increased PYY and CCK levels as well as decreased GIP levels (Jones et al., 2009; Vos et al., 2011; Guelfi et al., 2013; Martins et al., 2013; Lean and Malkova, 2016; Sheikholeslami-Vatani and Rostamzadeh, 2022). Here, it must be noted that weight loss in these studies was, as in our study, modest or not apparent and results are not completely consistent (Lien et al., 2009; Lean and Malkova, 2016; Ataeinosrat et al., 2022; Sheikholeslami-Vatani and Rostamzadeh, 2022). Thus, whether this (potentially beneficial) lack of strong hormonal alterations is due to the mode or the amount of weight loss/fat mass decrease, we cannot determine here.
A similar line of thought may be followed regarding the lack of changes in FGF21 levels in our study. FGF21 levels have been observed to be increased in obesity and to decrease after ≥9% diet- or surgery-induced weight loss (Christodoulides et al., 2009; Gómez-Ambrosi et al., 2017). This indicates that obesity-related increases in FGF21 levels may represent a compensatory mechanism for disease-related metabolic challenges and weight-loss-induced reductions might actually reflect improved metabolic health. In response to our intervention, FGF21 levels were not significantly altered, which is in line with previous observations in the context of modest weight loss (5.3%) (Mai et al., 2011). Thus, a more pronounced weight loss may be needed to significantly affect FGF21 levels.
The observed decrease in PP levels in our study may, meanwhile, point towards more hunger signaling. Varying effects of weight loss on fasting PP levels have been reported before, ranging from a decrease to an increase to the absence of changes (Reinehr et al., 2006; Sloth et al., 2008; Sumithran et al., 2011; Belinova et al., 2017). This variation in response may be due to differences in the interventions, amount of weight loss, study duration or sample composition. Thus, the effect of weight loss on PP secretion and the implications for weight management remain a subject of further investigation.
Even though we observed an increase in AgRP levels after 10 weeks of initial weight loss, levels had reverted to baseline values at the end of the program. This finding is partly in line with previous studies reporting increased levels of AgRP in response to diet- or lifestyle-induced weight reductions (Patkar et al., 2019; Kravchychyn et al., 2021). It also points towards increased hunger signaling in line with a potential weight-loss-induced physiological compensation (Sumithran et al., 2011; Sumithran and Proietto, 2013; Greenway, 2015). However, the reversal of AgRP levels back to baseline levels at the end of treatment also suggests that a potential acute ‘starvation’ response via increased AgRP levels can be silenced once energy balance is maintained on the long-term, i.e. once the body has had time to adapt to a new ‘set point’ of energy stores. Notably, there were no associations between initial increase in AgRP levels and subsequent changes in anthropometrics, indicating that a potential limiting effect on successful weight management was, if present, rather small.
In fact, after corrections for sex and age, most changes in hormone levels during initial weight loss (T0-T1) were not associated with subsequent changes in anthropometrics. Only initial decreases in FGF21 levels and increases in HMW adiponectin levels tended to be associated with subsequent increases in BMI, but not WC.
At first sight, this result seems puzzling since both a decrease in FGF21 levels as well as an increase in HMW adiponectin could indicate metabolic improvements in the context of obesity (Kim et al., 2007; Lee and Shao, 2014; Stern et al., 2016; Gómez-Ambrosi et al., 2017). Data from rodents suggests that both hormones act via a shared axis to exert their beneficial lipid- and insulin-lowering effects via actions on the adipose tissue and the liver (Holland et al., 2013; Stern et al., 2016). Indeed, FGF21 has been identified as an upstream effector of adiponectin (Holland et al., 2013). Nevertheless, their interaction is likely to be altered in the present context since, during weight loss, people with overweight or obesity usually decrease in their FGF21 levels while simultaneously increasing in adiponectin levels (Soni et al., 2011; Gómez-Ambrosi et al., 2017). Our findings are in agreement with studies suggesting that; in the context of obesity; weight-loss-induced metabolic and hormonal improvements such as increases in adiponectin levels can come at the expense of increased metabolic efficiency and increased energy storage into (especially subcutaneous) fat cells (Kim et al., 2007; Lee and Shao, 2014; Stern et al., 2016). Partly in line with this hypothesis, data from the Nurses’ Health study showed that higher baseline adiponectin levels predicted more subsequent weight gain in a large sample of healthy women (Hivert et al., 2011). Based on a study in rodents, the authors hypothesized that this association may reflect a state of adiponectin-induced improved metabolic efficiency and healthier adipocyte functioning via the expansion of subcutaneous body fat (instead of ectopic fat accumulation as e.g. in the pancreas or the liver) (Kim et al., 2007; Hivert et al., 2011). Since we do not have data on the size or functionality of our patients’ adipocytes, a deeper investigation of this hypothesis is unfortunately beyond the scope of this study.
At any rate, our results regarding the association between T0-T1 change in FGF21/HMW adiponectin levels and subsequent T1-T2 increase in BMI must be interpreted with caution. First, because of limited robustness: for both FGF21 as well as HMW adiponectin, the exclusion of the most extreme data points resulted in a non-significant association (see Supplementary Figure S3), indicating that the associations may have been driven by these two data points. Nevertheless, there was no other reason to exclude them, so we kept them in the dataset. Second, as we performed many statistical tests, there is a possibility that the observed association has occurred by chance. Indeed, the association did not remain statistically significant when applying the (however rather conservative) Bonferroni-correction for multiple testing. Finally, the unstandardized regression coefficient of changes in HMW adiponectin levels as a predictor was very small, indicating that for every 1% increase in T0-T1 HMW adiponectin levels, there was a 0.11% increase in BMI between T1 and T2 (see Table 3). The regression coefficient for T0-T1% changes in FGF21 levels was even smaller (see Table 3), indicating that the actual impact of initial hormone changes on subsequent weight gain might not be of strong clinical relevance. Thus, the role of (changes in) FGF21 and HMW adiponectin levels and weight management warrants further investigation in a larger sample.
4.1 Strengths
A clear strength of this study is the >1-year follow-up period after initial 10-week period of weight loss, which allowed us to investigate effects of hormonal alterations on long-term weight loss maintenance. Second, we measured a large number of appetite-regulating hormones, which provides a more comprehensive picture of the changes in the homeostatic appetite system than measuring few or single hormones alone.
4.2 Limitations
We do acknowledge that this study also has some limitations. First, our results are limited to fasting hormone levels. Although this provides insight into the potential chronic effects of our intervention on long-term satiety (meal-to-meal interval), it would also be of interest to investigate the postprandial hormone release to assess the effects on short-term satiation (postprandial response) as both may affect food intake and long-term weight management in different ways. Second, the current study is limited to the demonstration of associations and does not provide evidence of causality. Third, although patients were instructed to follow a healthy normocaloric diet, we were not able to exactly determine to what degree patients had complied with the intervention regime. Finally, the limited sample size of our study may have resulted in to type 1 errors and our results should thus be replicated in a larger cohort.
4.3 Future directions
The limited sample size of the current study prevented us from investigating potential synergistic effects of changes in multiple hormones in a single statistical model. This could be included in future large-scale studies. It would also be highly interesting to prospectively compare the hormonal alterations in response to a normocaloric CLI to a control group and other interventions as well as single components of the intervention (e.g., CLI vs. very-low energy diets; vs. healthy diet only; vs. CBT only; vs. exercise only). Finally, future studies should investigate potential sex differences in this context which we could not assess in view of a sample size of only eight males.
5 Conclusion
Modest weight loss in response to a 1.5-year combined lifestyle intervention (comprising a healthy normocaloric diet, exercise and CBT) was accompanied by changes in levels of long-term adiposity-associated hormones towards healthy levels, and no changes in levels of most short-term regulators of energy homeostasis. The clinical impact of alterations in levels of appetite-regulating hormones on long-term weight loss maintenance during modest weight loss remains questionable. Especially changes in levels of long-term adiposity-related hormones seem to rather follow weight loss instead of governing it. Future studies should investigate the potential association of (changes in) FGF21 and adiponectin levels with subsequent weight gain in a larger sample, ideally including measures of adipocyte distribution and health.
Data availability statement
The original contributions presented in the study are included in the article/supplementary materials, further inquiries can be directed to the corresponding author.
Ethics statement
The studies involving human participants were reviewed and approved by Dutch Medical Ethics Review Committee (MERC). The patients/participants provided their written informed consent to participate in this study.
Author contributions
SK was responsible for developing the research question, performing the statistical analyses and writing the manuscript. She also planned and coordinated the hormone analyses of the blood samples and assisted in the laboratory work. EV contributed to developing the research question, helped with discussing the statistical analysis and gave feedback on the manuscript. AS helped with interpreting the results as well as writing the manuscript, whereby he provided substantial input regarding the theoretical framework. RL has helped with the statistical analysis, interpretation of the results and gave feedback on the manuscript. JV, AI, and MM helped with the interpretation of the results and have given feedback on the manuscript. SB was involved in organizing the laboratory analyses in his laboratory and in the interpretation of the results from a technical as well as theoretical perspective. He also gave feedback on the manuscript. ER was involved in the development of the research question, clinical care of the patients, and has supervised the coordination of the blood analyses, the statistical analyses, the interpretation of the results as well as the writing of the manuscript.
Funding
This work was funded by the Netherlands organization for scientific research (NWO) Vidi (grant No. 91716453) and the Elisabeth Foundation.
Acknowledgments
We would like to thank Mariëtte Boon for sharing her expertise on hormonal regulators of energy homeostasis. In addition, we would like to thank Mila Welling, Bibian van der Voorn, Hilguin Ruinemans, Kirsten Berk, Eva van Eijk, Arlette Vergunst for their medical treatments and professional guidance of the patients during the lifestyle intervention. We are also thankful to all patients included in the study as well as for the support of Renate Meeusen and Annemieke van der Zwaan regarding data management and study coordination.
Conflict of interest
The authors declare that the research was conducted in the absence of any commercial or financial relationships that could be construed as a potential conflict of interest.
Publisher’s note
All claims expressed in this article are solely those of the authors and do not necessarily represent those of their affiliated organizations, or those of the publisher, the editors and the reviewers. Any product that may be evaluated in this article, or claim that may be made by its manufacturer, is not guaranteed or endorsed by the publisher.
Supplementary material
The Supplementary Material for this article can be found online at: https://www.frontiersin.org/articles/10.3389/fphys.2023.1010858/full#supplementary-material
References
Ataeinosrat A., Haghighi M. M., Abednatanzi H., Soltani M., Ghanbari-Niaki A., Nouri-Habashi A., et al. (2022). Effects of three different modes of resistance training on appetite hormones in males with obesity. Front. Physiol. 13, 827335. doi:10.3389/fphys.2022.827335
Belinova L., Kahleova H., Malinska H., Topolcan O., Windrichova J., Oliyarnyk O., et al. (2017). The effect of meal frequency in a reduced-energy regimen on the gastrointestinal and appetite hormones in patients with type 2 diabetes: A randomised crossover study. PloS one 12, e0174820. doi:10.1371/journal.pone.0174820
Chooi Y. C., Ding C., Magkos F. (2019). The epidemiology of obesity. Metabolism 92, 6–10. doi:10.1016/j.metabol.2018.09.005
Christodoulides C., Dyson P Fau - Sprecher D., Sprecher D Fau - Tsintzas K., Tsintzas K Fau - Karpe F., Karpe F. (2009). Circulating fibroblast growth factor 21 is induced by peroxisome proliferator-activated receptor agonists but not ketosis in man. J. Clin. Endocrinol. Metab. 94, 3594–3601. doi:10.1210/jc.2009-0111
GBD 2015 Obesity Collaborators., Afshin A., Forouzanfar M. H., Reitsma M. B., Sur P., Estep K., et al. (2017). Health effects of overweight and obesity in 195 countries over 25 years. N. Engl. J. Med. 377, 13–27. doi:10.1056/NEJMoa1614362
Coutinho S. R., Rehfeld J. F., Holst J. J., Kulseng B., Martins C. (2018). Impact of weight loss achieved through a multidisciplinary intervention on appetite in patients with severe obesity. Am. J. Physiology-Endocrinology Metabolism 315, E91–E98. doi:10.1152/ajpendo.00322.2017
Essah P. A., Levy J. R., Sistrun S. N., Kelly S. M., Nestler J. E. (2010). Effect of weight loss by a low-fat diet and a low-carbohydrate diet on peptide YY levels. Int. J. Obes. 34, 1239–1242. doi:10.1038/ijo.2010.48
Gómez-Ambrosi J., Gallego-Escuredo J. M., Catalán V., Rodríguez A., Domingo P., Moncada R., et al. (2017). FGF19 and FGF21 serum concentrations in human obesity and type 2 diabetes behave differently after diet- or surgically-induced weight loss. Clin. Nutr. 36, 861–868. doi:10.1016/j.clnu.2016.04.027
Greenway F. L. (2015). Physiological adaptations to weight loss and factors favouring weight regain. Int. J. Obes. 39, 1188–1196. doi:10.1038/ijo.2015.59
Guelfi K. J., Donges C. E., Duffield R. (2013). Beneficial effects of 12 weeks of aerobic compared with resistance exercise training on perceived appetite in previously sedentary overweight and obese men. Metabolism 62, 235–243. doi:10.1016/j.metabol.2012.08.002
Haffner S. M., Valdez Ra Fau - Hazuda H. P., Hazuda Hp Fau - Mitchell B. D., Mitchell Bd Fau - Morales P. A., Morales Pa Fau - Stern M. P., Stern M. P. (1992). Prospective analysis of the insulin-resistance syndrome (syndrome X). Diabetes 41, 715–722. doi:10.2337/diab.41.6.715
Heni M., Schöpfer P., Peter A., Sartorius T., Fritsche A., Synofzik M., et al. (2014). Evidence for altered transport of insulin across the blood–brain barrier in insulin-resistant humans. Acta Diabetol. 51, 679–681. doi:10.1007/s00592-013-0546-y
Hivert M.-F., Sun Q., Shrader P., Mantzoros C. S., Meigs J. B., Hu F. B. (2011). Higher adiponectin levels predict greater weight gain in healthy women in the Nurses' Health Study. Obes. (Silver Spring, Md ) 19, 409–415. doi:10.1038/oby.2010.189
Holland W. L., Adams A. C., Brozinick J. T., Bui H. H., Miyauchi Y., Kusminski C. M., et al. (2013). An FGF21-adiponectin-ceramide axis controls energy expenditure and insulin action in mice. Cell Metab. 17, 790–797. doi:10.1016/j.cmet.2013.03.019
Jastreboff A. M., Aronne L. J., Ahmad N. N., Wharton S., Connery L., Alves B., et al. (2022). Tirzepatide once weekly for the treatment of obesity. N. Engl. J. Med. 387, 205–216. doi:10.1056/NEJMoa2206038
Jensen M. D., Ryan D. H., Apovian C. M., Ard J. D., Comuzzie A. G., Donato K. A., et al. (2014). 2013 AHA/ACC/TOS guideline for the management of overweight and obesity in adults: A report of the American college of cardiology/American heart association task force on practice guidelines and the obesity society. J. Am. Coll. Cardiol. 63, 2985–3023. doi:10.1016/j.jacc.2013.11.004
Jones T. E., Basilio Jl Fau - Brophy P. M., Brophy Pm Fau - Mccammon M. R., Mccammon Mr Fau - Hickner R. C., Hickner R. C. (2009). Long-term exercise training in overweight adolescents improves plasma peptide YY and resistin. Obesity 6, 1189–1195. doi:10.1038/oby.2009.11
Khosravi-Largani M., Nojomi M., Aghili R., Otaghvar H. A., Tanha K., Seyedi S. H. S., et al. (2019). Evaluation of all types of metabolic bariatric surgery and its consequences: A systematic review and meta-analysis. Obes. Surg. 29, 651–690. doi:10.1007/s11695-018-3550-z
Kim J. Y., Van De Wall E Fau - Laplante M., Laplante M Fau - Azzara A., Azzara A., Trujillo Me Fau - Hofmann S. M., Hofmann Sm Fau - Schraw T., et al. (2007). Obesity-associated improvements in metabolic profile through expansion of adipose tissue. J. Clin. Invest. 117, 2621–2637. doi:10.1172/JCI31021
Könner A. C., Brüning J. C. (2012). Selective insulin and leptin resistance in metabolic disorders. Cell Metab. 16, 144–152. doi:10.1016/j.cmet.2012.07.004
Kravchychyn A. C. P., Campos R., Oliveira E. S. L., Ferreira Y. A. M., Corgosinho F. C., Masquio D. C. L., et al. (2021). Adipocytokine and appetite-regulating hormone response to weight loss in adolescents with obesity: Impact of weight loss magnitude. Nutrition 14, 111188–88. doi:10.1016/j.nut.2021.111188
Lean M. E. J., Malkova D. (2016). Altered gut and adipose tissue hormones in overweight and obese individuals: Cause or consequence? IJO 40, 622–632. doi:10.1038/ijo.2015.220
Lee B., Shao J. (2014). Adiponectin and energy homeostasis. Rev. Endocr. metabolic Disord. 15, 149–156. doi:10.1007/s11154-013-9283-3
Leon-Cabrera S., Solís-Lozano L., Suárez-Álvarez K., González-Chávez A., Béjar Y., Robles-Díaz G., et al. (2013). Hyperleptinemia is associated with parameters of low-grade systemic inflammation and metabolic dysfunction in obese human beings. Front. Integr. Neurosci. 23, 62. doi:10.3389/fnint.2013.00062
Lien L. F., Haqq Am Fau - Arlotto M., Arlotto M Fau - Slentz C. A., Slentz Ca Fau - Muehlbauer M. J., Muehlbauer Mj Fau - Mcmahon R. L., Mcmahon Rl Fau - Rochon J., et al. (2009). The STEDMAN project: Biophysical, biochemical and metabolic effects of a behavioral weight loss intervention during weight loss, maintenance, and regain. OMICS 13, 21–35. doi:10.1089/omi.2008.0035
Mai K., Schwarz F., Bobbert T., Andres J., Assmann A., Pfeiffer A. F. H., et al. (2011). Relation between fibroblast growth factor–21, adiposity, metabolism, and weight reduction. Metabolism 60, 306–311. doi:10.1016/j.metabol.2010.02.016
Martins C., Dutton G. R., Hunter G. R., Gower B. A. (2020). Revisiting the Compensatory Theory as an explanatory model for relapse in obesity management. Am. J. Clin. Nutr. 112, 1170–1179. doi:10.1093/ajcn/nqaa243
Martins C., Kulseng B Fau - Rehfeld J. F., Rehfeld Jf Fau - King N. A., King Na Fau - Blundell J. E., Blundell J. E. (2013). Effect of chronic exercise on appetite control in overweight and obese individuals. Med. Sci. Sports Exerc 45, 805–812. doi:10.1249/MSS.0b013e31827d1618
Matheny M., Shapiro A., Tumer N., Scarpace P. J. (2011). Region-specific diet-induced and leptin-induced cellular leptin resistance includes the ventral tegmental area in rats. Neuropharmacology 60, 480–487. doi:10.1016/j.neuropharm.2010.11.002
Mustajoki P., Pekkarinen T. (2001). Very low energy diets in the treatment of obesity. Obes. Rev. 2, 61–72. doi:10.1046/j.1467-789x.2001.00026.x
Patkar P. P., Hao Z., Mumphrey M. B., Townsend R. L., Berthoud H. R., Shin A. C. (2019). Unlike calorie restriction, Roux-en-Y gastric bypass surgery does not increase hypothalamic AgRP and NPY in mice on a high-fat diet. Int. J. Obes. 43, 2143–2150. doi:10.1038/s41366-019-0328-x
Reinehr T., Enriori P. J., Harz K., Cowley M. A., Roth C. L. (2006). Pancreatic polypeptide in obese children before and after weight loss. Int. J. Obes. 30, 1476–1481. doi:10.1038/sj.ijo.0803393
Rossi M., Kim Ms Fau - Morgan D. G., Morgan Dg Fau - Small C. J., Small Cj Fau - Edwards C. M., Edwards Cm Fau - Sunter D., Sunter D Fau - Abusnana S., et al. (1998). A C-terminal fragment of Agouti-related protein increases feeding and antagonizes the effect of alpha-melanocyte stimulating hormone in vivo. Endocrinology 139, 4428–4431. doi:10.1210/endo.139.10.6332
Seino Y., Yabe D. (2013). Glucose-dependent insulinotropic polypeptide and glucagon-like peptide-1: Incretin actions beyond the pancreas. J. diabetes investigation 4, 108–130. doi:10.1111/jdi.12065
Sheikholeslami-Vatani D., Rostamzadeh N. (2022). Changes in appetite-dependent hormones and body composition after 8 Weeks of high-intensity interval training and vitamin D supplementation in sedentary overweight men. Front. Nutr. 9, 827630. doi:10.3389/fnut.2022.827630
Sloth B., Due A., Larsen T. M., Holst J. J., Heding A., Astrup A. (2008). The effect of a high-MUFA, low-glycaemic index diet and a low-fat diet on appetite and glucose metabolism during a 6-month weight maintenance period. Br. J. Nutr. 101, 1846–1858. doi:10.1017/S0007114508137710
Soni A. C., Conroy M. B., Mackey R. H., Kuller L. H. (2011). Ghrelin, leptin, adiponectin, and insulin levels and concurrent and future weight change in overweight, postmenopausal women. Menopause 18, 296–301. doi:10.1097/gme.0b013e3181f2e611
Steinert R. E., Feinle-Bisset C., Asarian L., Horowitz M., Beglinger C., Geary N. (2017). Ghrelin, CCK, GLP-1, and PYY(3-36): Secretory controls and physiological roles in eating and glycemia in health, obesity, and after RYGB. Physiol. Rev. 97, 411–463. doi:10.1152/physrev.00031.2014
Stern J. H., Rutkowski J. M., Scherer P. E. (2016). Adiponectin, leptin, and fatty acids in the maintenance of metabolic homeostasis through adipose tissue crosstalk. Cell metab. 23, 770–784. doi:10.1016/j.cmet.2016.04.011
Strohacker K., Mccaffery J. M., Maclean P. S., Wing R. R. (2014). Adaptations of leptin, ghrelin or insulin during weight loss as predictors of weight regain: A review of current literature. Int. J. Obes. 38, 388–396. doi:10.1038/ijo.2013.118
Sumithran P., Prendergast L. A., Delbridge E., Purcell K., Shulkes A., Kriketos A., et al. (2013). Ketosis and appetite-mediating nutrients and hormones after weight loss. Eur. J. Clin. Nutr. 67, 759–764. doi:10.1038/ejcn.2013.90
Sumithran P., Prendergast L. A., Delbridge E., Purcell K., Shulkes A., Kriketos A., et al. (2011). Long-term persistence of hormonal adaptations to weight loss. N. Engl. J. Med. 365, 1597–1604. doi:10.1056/NEJMoa1105816
Sumithran P., Proietto J. (2013). The defence of body weight: A physiological basis for weight regain after weight loss. Clin. Sci. (Lond) 124, 231–241. doi:10.1042/CS20120223
Talukdar S., Zhou Y., Li D., Rossulek M., Dong J., Somayaji V., et al. (2016). A long-acting FGF21 molecule, PF-05231023, decreases body weight and improves lipid profile in non-human primates and type 2 diabetic subjects. Cell Metab. 23, 427–440. doi:10.1016/j.cmet.2016.02.001
Van der Zalm I. J. B., Van Der Valk E. S., Wester V. L., Nagtzaam N. M. A., Van Rossum E. F. C., Leenen P. J. M., et al. (2020). Obesity-associated T-cell and macrophage activation improve partly after a lifestyle intervention. Int. J. Obes. 44, 1838–1850. doi:10.1038/s41366-020-0615-6
VOEDINGSCENTRUM (2015). Richtlijnen goede voeding 2015 [Online]. Voedingscentrum. (Accessed 05 01, 2022 2022). Available at: https://www.gezondheidsraad.nl/documenten/adviezen/2015/11/04/richtlijnen-goede-voeding-2015
Vos R. C., Pijl H., Wit J. M., Van Zwet E. W., Van Der Bent C., Houdijk E. C. A. M. (2011). The effect of multidisciplinary lifestyle intervention on the pre- and postprandial plasma gut Peptide concentrations in children with obesity. ISRN Endocrinol. 2011, 353756. doi:10.5402/2011/353756
Zhu N., Pankow Js Fau - Ballantyne C. M., Ballantyne Cm Fau - Couper D., Couper D Fau - Hoogeveen R. C., Hoogeveen Rc Fau - Pereira M., Pereira M Fau - Duncan B. B., et al. (2010). High-molecular-weight adiponectin and the risk of type 2 diabetes in the ARIC study. J. Clin. Endocrinol. Metab. 95, 5097–5104. doi:10.1210/jc.2010-0716
Keywords: appetite regulation, lifestyle intervation, gut hormones, weight regain, gut-brain axis
Citation: Kuckuck S, van der Valk ES, Scheurink AJW, Lengton R, Mohseni M, Visser JA, Iyer AM, van den Berg SAA and van Rossum EFC (2023) Levels of hormones regulating appetite and energy homeostasis in response to a 1.5-Year combined lifestyle intervention for obesity. Front. Physiol. 14:1010858. doi: 10.3389/fphys.2023.1010858
Received: 03 August 2022; Accepted: 30 January 2023;
Published: 20 February 2023.
Edited by:
Ahmad Alkhatib, University of Taipei, TaiwanReviewed by:
Kleber Souza, Federal University of Rio de Janeiro, BrazilHélène Volkoff, Memorial University of Newfoundland, Canada
Copyright © 2023 Kuckuck, van der Valk, Scheurink, Lengton, Mohseni, Visser, Iyer, van den Berg and van Rossum. This is an open-access article distributed under the terms of the Creative Commons Attribution License (CC BY). The use, distribution or reproduction in other forums is permitted, provided the original author(s) and the copyright owner(s) are credited and that the original publication in this journal is cited, in accordance with accepted academic practice. No use, distribution or reproduction is permitted which does not comply with these terms.
*Correspondence: Elisabeth F. C. van Rossum, ZS52YW5yb3NzdW1AZXJhc211c21jLm5s