- 1Research Institute for Agriculture, Fisheries and Food (ILVO), Merelbeke, Belgium
- 2Department of Pathobiology, Pharmacology and Zoological Medicine, Ghent University, Merelbeke, Belgium
- 3Zinpro Corporation, Boxmeer, Netherlands
- 4Department of Agricultural and Food Sciences, Alma Mater Studiorum, University of Bologna, Cesena, Italy
- 5Department of Animal Sciences and Aquatic Ecology, Ghent University, Ghent, Belgium
The objective of this study was to evaluate the effect of the interaction of the zinc source (ZnSO4 vs. zinc amino acid complex) and vitamin E level (50 IU/kg vs. 100 IU/kg) on meat yield and quality in broilers subjected to chronic cyclic heat stress in the finisher phase. A total of 1224 one-day-old male Ross 308 broilers were randomly distributed among four dietary treatments. Each treatment contained nine replicates of 34 birds, housed in floor pens in a temperature- and lighting-controlled room. Treatments were organized in a 2 × 2 factorial arrangement: two sources of zinc, 60 mg/kg of Zn as ZnSO4 or 60 mg/kg of Zn as zinc amino acid complexes (ZnAA), combined with two levels of vitamin E (50 or 100 IU/kg). From day 28 until day 37 (finisher phase), all birds were subjected to chronic cyclic heat stress (32 ± 2°C for 6 h daily). In the present study, it was observed that replacing ZnSO4 with ZnAA increased breast meat weight and yield of broilers reared under chronic cyclic heat stress conditions, whereas total slaughter yield was not affected. Moreover, it was observed that replacing ZnSO4 with ZnAA resulted in breast meat with a lower drip and thawing loss and a higher marinade uptake. In conclusion, replacing ZnSO4 with more readily available ZnAA can improve breast meat yield and increase the water-holding capacity of breast meat of broilers exposed to chronic cyclic heat stress at the end of the production cycle. However, as no thermoneutral group was included in the present study, the observed effects of the zinc source cannot be generalized as a solution for heat stress. Moreover, the beneficial effects of ZnAA on breast meat yield and quality seem to be independent of the vitamin E level, and increasing vitamin E level has no additional beneficial effects.
1 Introduction
Heat stress is a major concern in poultry production because it has a profound effect on animal health and performance. Modern broiler breeds display reduced heat tolerance because of a lack of sweat glands and the high metabolism associated with low feed conversion and rapid growth (Lara and Rostagno, 2013; He et al., 2018). Moreover, chronic heat stress leads to the deterioration of meat quality by changing the aerobic metabolism and by increasing glycolysis and fat deposition (Petracci et al., 2015; Lu et al., 2017; Wang et al., 2017). Consequently, the meat from broilers reared under high environmental temperatures is characterized by a pale color, low water-holding capacity (WHC), and therefore also increased cook and drip losses (Wang et al., 2017). The impaired WHC is detrimental to the valorization of broiler meat which is further processed by marination, tumbling, and cooking (Zaboli et al., 2019). Supplementation of vitamin A, C, and E can improve heat tolerance ability and animal performance during heat stress (Khan et al., 2011; Khan et al., 2012; Rehman et al., 2017). Some antioxidant minerals, including chromium (Akbari and Torki, 2014; Attia et al., 2017), selenium (Habibian et al., 2015), and zinc, (Sahin et al., 2009; Chand et al., 2014) are also used to prevent negative effects of heat stress. Zinc is an essential component of many enzymes, and it has both structural and catalytic functions in metalloenzymes. Furthermore, zinc is required for normal immune function as well as proper skeletal development and maintenance (Sahin et al., 2009). One of the most important functions of zinc is its antioxidant role and its participation in the antioxidant defense system. An increased level of reactive oxygen species is one of the main causes of decreased meat quality due to heat stress (Zaboli et al., 2019). In broiler diets, ZnSO4 and ZnO are two of the main inorganic zinc sources. There are also organic zinc sources available that are characterized by improved bioavailability (Star et al., 2012; Swiatkiewicz et al., 2014). A more readily available zinc source might be more efficient in reducing the adverse effects of stressors in broiler production, such as heat stress. Both zinc and vitamin E are frequently used antioxidants to alleviate the negative impact of heat stress; however, vitamin E is expensive, and lower levels may be sufficient in combination with a more readily available zinc source. To the best of our knowledge, there is no information available concerning the interaction of zinc sources, as opposed to different zinc levels, on meat quality of broilers subjected to a temperature challenge, and on the interaction with the vitamin E level. Therefore, the objective of this study was to evaluate the effect of the interaction of the zinc source (ZnSO4 vs. zinc amino acid complex) and vitamin E level (50 vs. 100 IU) on meat quality and yield of broilers exposed to chronic cyclic heat stress in the finisher phase.
2 Materials and methods
All experimental procedures in this study were in compliance with the European guidelines for the care and use of animals in research (Directive 2010: 63: EU) and were approved by the Ethical Committee of the Research Institute for Agriculture, Fisheries and Food (ILVO), Merelbeke, Belgium, under authorization number 2017: 308.
2.1 Experimental design, animals, and diets
A total of 1224 one-day-old male Ross 308 broilers (Belgabroed, Merksplas, Belgium) were randomly allocated to 36 floor pens (9 pens per treatment and 34 broilers per pen) in an alternating block design, with one replicate per treatment in each block. Up to day 7, the broilers were subjected to a light schedule of 23 hours of light and 1 hour of darkness. From day 7 onwards, the animals were subjected to a light schedule of 18 hours of light and 6 hours of darkness. The temperature was kept at 29°C during the first week of the experiment and reduced thereafter until a final temperature of 22°C was reached at day 28. From day 28 until day 37 (slaughter age), the temperature and relative humidity (RH) in the stable were raised up to 32 ± 2°C and 55–65%, respectively, for 3 h and subsequently maintained for 6 h before cooling down again to the initial temperature of 22°C. Heat stress was confirmed empirically on randomly selected birds from different pens equally divided over treatments by measuring the rectal temperature, in order to confirm that the temperature was raised above the physiological range of 41.2–42.2°C. The house was equipped with a dynamic ventilation system with an air entrance on one side and air extraction on the other side. The temperature and RH were constantly monitored and steered by the adjustment of the heating system and ventilation rate. Humidity was increased by nebulizing water via water nozzles and decreased by increasing the ventilation rate.
Dietary treatments were organized in a 2 × 2 factorial design with two sources of zinc (Zn). Treatments contained equal amounts of elemental zinc (60 mg/kg), either originating from ZnSO4 (ZnSO4.7H2O; containing 22% of elemental zinc, Sigma-Aldrich, St. Louis, United States) or originating as zinc amino acid complexes (ZnAA; containing 10% of elemental zinc, Availa®Zn, Zinpro Corporation, Eden Prairie, United States), and two levels of vitamin E (50 or 100 IU/kg; dl-α-tocopheryl acetate). The dietary treatments were provided in a wheat–rye-based diet (Table 1). Availa®Zn is a zinc chelate based on single amino acids from hydrolyzed soy protein and zinc bound in a one-to-one molar ratio. All dietary treatments contained equal zinc levels that comply with the dietary needs as described by NRC (1994). A vitamin E level of 50 IU/kg is recommended in the Ross 308 manual (Aviagen, 2018) and was set at the standard dose, and 100 IU/kg was selected as the elevated level of vitamin E. The total levels of zinc included in the experimental diets were determined using inductively coupled plasma atomic emission spectroscopy with a method derived from NEN 15763, ISO 21033, and ISO 27085 at the ECCA laboratory (Merelbeke, Belgium) (Table 2). The total levels of vitamin E included in the experimental diets were determined according to Claeys et al. (2016) (Table 2). A three-phase feeding scheme was applied, and dietary treatments were applied in all phases. The starter diet was fed from day 0 up to day 10 and was provided as a crumble. The grower and finisher diets were fed as pellets from day 10 up to day 28 and from day 28 up to day 37, respectively. Feed and drinking water were provided ad libitum.
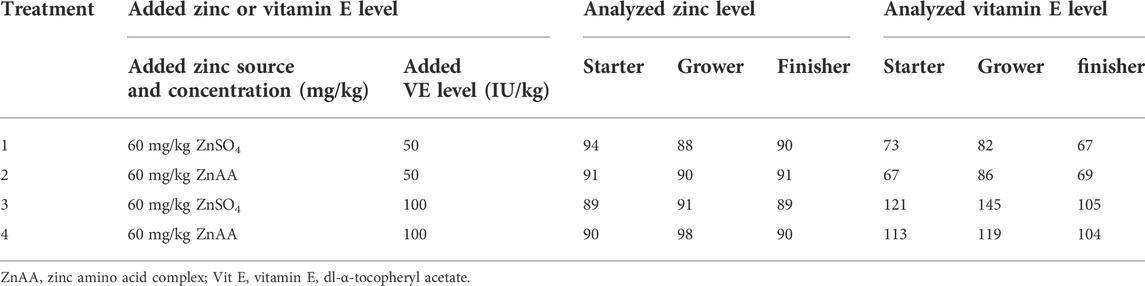
TABLE 2. Analyzed zinc and vitamin E concentrations in the different dietary treatments for broilers.
2.2 Slaughter yield and meat quality analysis
On day 37, three broilers per pen were selected, and live weight was determined by weighing the animals on a scale suited for animal use before transport to the slaughterhouse, where they were commercially slaughtered. Carcasses were immediately chilled after processing. Slaughter yield was determined approximately 24 h after slaughter (108 birds in total, 27 from each treatment group). The broilers were manually dissected by trained personnel to determine carcass, wing, leg (thigh and drumstick) and breast meat weight, and total yield. Carcass yield was calculated as eviscerated carcass weight relative to live weight before slaughter. The carcasses were cut as shown in Figure 1, and the breast, thigh, drumstick, and wing yields were calculated as their weight relative to eviscerated carcass weight. All parts were skin-on and bone-in after cut-up, except for the breast.
The different meat quality parameters were determined using the breast (pectoralis major muscles) (Figure 2). Left breast fillets (n = 9 per treatment) were weighed, and color measurements were performed using a MiniScan EZ colorimeter (Hunterlab, Reston, VA) to record CIE L* (lightness), a* (redness), and b* (yellowness) values. Temperature and pH ultimate were measured using a Portamess® 910 (Knick, Berlin, Germany). Following these measurements, breast fillets were vacuum-packed and stored at -20°C in order to determine protein solubility at a later time point. The remaining left breast fillets (n = 18 per treatment) were vacuum-packed and transported to the University of Bologna (Italy, Cesena, Department of Agricultural and Food Sciences) in order to determine marinade uptake. The right breast fillets (n = 18 per treatment) were removed from the carcass and put in a polypropylene bag, hung for 24 h at 4 ± 2°C, and then blotted dry, and weighed again to measure drip loss. Drip loss was calculated as the difference between the weight before storage (W1) and the weight after storage (W2) relative to the weight before storage. Drip loss was calculated using the following formula: drip loss (%) = [(W1–W2)/W1 ] × 100. The remaining right (n = 9 per treatment) breast fillets were weighed (W3), vacuum-packed, and stored at -20°C for 4 days. They were then defrosted for 24 h at 5°C, blotted dry, and weighed (W4) in order to determine thawing loss. Thawing loss was calculated using the following formula: thawing loss = [(W3–W4)/W3 ] × 100. After the thawing loss was determined, the fillets were cooked in a warm water bath (80°C) for 30 min. Afterward, they were blotted dry and weighed (W5) to record cooking loss. Cooking loss was calculated using the following formula: cooking loss (%) = [(W4—W5)/W4 ] × 100. Drip loss, thawing loss, and cooking loss were used to evaluate the water-holding capacity.
2.2.1 Myofibrillar and sarcoplasmic protein solubility
Protein solubility was determined based on the difference in extractability of proteins in solutions at different ionic strengths. Sarcoplasmic protein solubility was determined by homogenizing (Ultraturrax, T25 basic, New Brunswick NJ) 3 g of minced meat in 80 ml of extraction medium (150 mM sodium chloride and 0.01 mM iodo acetic acid). The supernatant was centrifuged (3,000 g, 10 min) and filtered (Schleicher & Schuell nr. 597½), and the protein concentration of this supernatant was determined using the biuret method (Layne, 1957). To determine myofibrillar protein solubility, the remaining pellet was suspended in 45 ml of extraction buffer (0.1 M citric acid, 1 mM EDTA, 0.4 M sodium chloride, and 0.01 mM iodo acetic acid). The suspension was incubated at room temperature for 2 h. The supernatant was centrifuged (5,000 g, 20 min) and filtered (Schleicher & Schuell nr. 597½), and the protein concentration of this supernatant was determined using the biuret method (Layne, 1957).
2.2.2 Marinade uptake
In order to assess marinade performances, meat was cut in order to obtain parallel cut samples (8 × 4 × 2 cm, weighing about 80 g), which were individually labeled and marinated by the addition of 20% marinade solution (6% sodium chloride and 1.8% sodium tripolyphosphate) using a small-scale vacuum tumbler (model MGH-20, Vakona Qualitat, Lienen, Germany). The tumbling time was 40 min under vacuum (-0.95 bar) (two working cycles of 20 min/cycle and one pause cycle of 5 min). After tumbling, samples were weighed again, and the difference in weight was used to determine marinade uptake. Marinade uptake was calculated based on carcass weight before marination (W1) and its weight after marination (W2), according to the following equation: marinade uptake (%) = [(W2 –W1)/W1] × 100.
2.3 Statistical analysis
Statistical analysis was performed in R for Windows (version 3.5.1). All data were checked for outliers and the normality of the residuals. Slaughter yield and meat quality were analyzed using a general linear model (GLM) with “zinc source” and “vitamin E level” as fixed factors and block as a random factor (factorial analysis). In the two-factorial analyses, when there was no significant interaction or no trend, only the main effects were taken into account. The differences were considered statistically significant at p < 0.05 and considered tendency at 0.05 < p < 0.1.
3 Results
3.1 Slaughter yield and meat quality
There were no interactions observed between the dietary zinc source and vitamin E level for total slaughter yield and the different meat quality parameters (Table 3). A tendency (p = 0.052) for live body weight was observed for the zinc source. Broilers that were fed a diet supplemented with ZnAA tended to have a higher slaughter weight than broilers that were fed diets supplemented with ZnSO4.
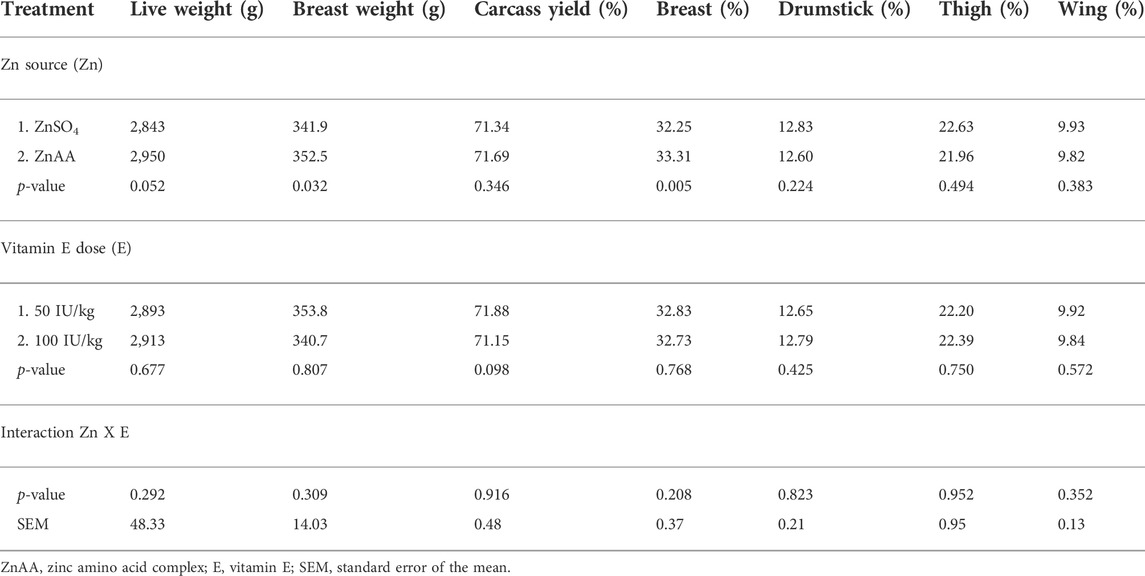
TABLE 3. Effect of the supplemental zinc (Zn) source and vitamin E level (Vit E) on live weight and carcass composition of broilers at slaughter age (day 37).
Only a main effect of the zinc source on breast yield and certain meat quality parameters was observed, whereas no main effect of the vitamin E level was observed. The zinc source significantly affected the breast yield and water-holding capacity of the breast meat. A higher breast meat yield was observed for birds that fed a diet supplemented with ZnAA than birds that fed a diet supplemented with ZnSO4. Breast meat of birds that were fed a diet supplemented with ZnAA was characterized by a significantly lower drip loss and thawing loss than breast meat of birds that were fed a diet supplemented with ZnSO4. No effect of dietary treatment on cooking loss was observed. Dietary treatment did not significantly influence protein solubility and marinade uptake in breast meat (Table 4).
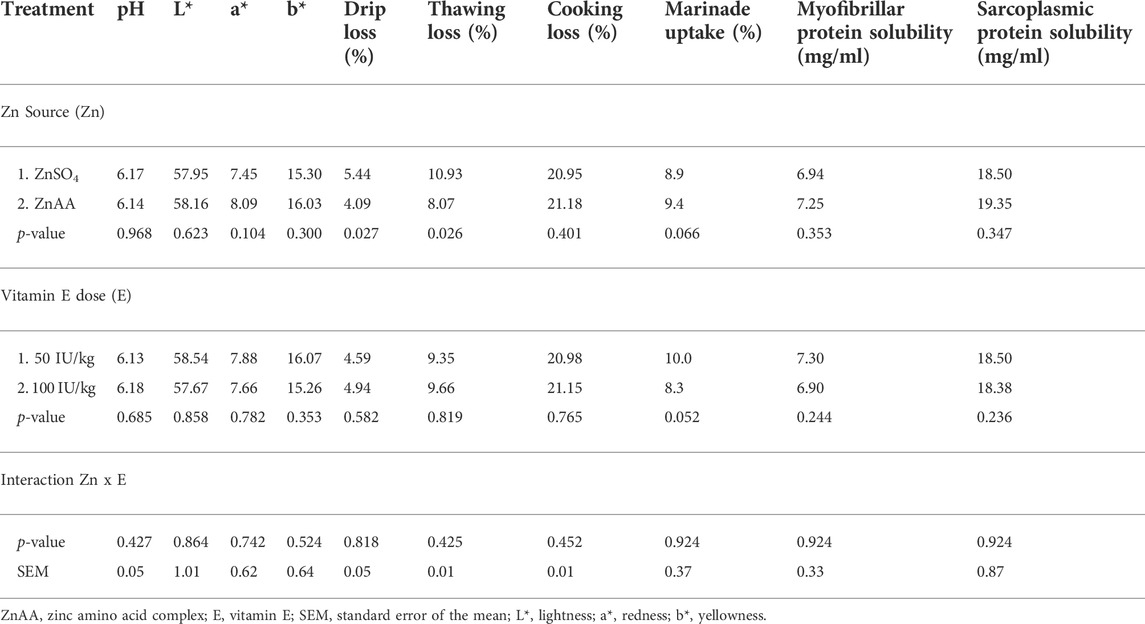
TABLE 4. Quality characteristics and functional properties of breast meat of broilers at slaughter age (day 37).
4 Discussion
Broilers reared under high temperatures often show lower meat yield and impaired meat quality (Zaboli et al., 2019). Breast meat of broilers exposed to chronic heat stress results in pale meat color (Petracci et al., 2004; Zhang et al., 2012), decreased WHC (Feng et al., 2008; Wang et al., 2009), and increased cook and drip losses (Woelfel et al., 2002) and is characterized by an increased denaturation of sarcoplasmic or myofibrillar proteins and a lower WHC (Zaboli et al., 2019). Additionally, higher breast meat yields are often associated with lower meat quality, characterized by a higher drip and cooking loss and lower marinade uptake (Petracci et al., 2015; Wang et al., 2017).
Interestingly, in the present study, it was observed that supplying zinc as ZnAA, resulted in increased yield in breast meat, characterized by lower drip and thawing losses, as compared to supplying zinc as ZnSO4. The lack of differences in cooking loss is probably due to the fact that fillets used to assess thawing loss were also used to assess the cooking loss. The increased breast yield might be partly attributed to the tendency for an increased live body weight of broilers supplemented with ZnAA, as modern broilers are selected for increased yield of pectoralis major muscles (Zuidhof et al., 2014). A previous study (De Grande et al., 2020) showed that ZnAA can improve performance under thermoneutral conditions. As zinc plays an important role in normal development and growth, it is possible that zinc supplements with increased bioavailability may better support growth under heat stress conditions. Although this could not be concluded from this study, no thermoneutral control group was included. Therefore, further research needs to be performed to confirm this hypothesis.
Zinc supplementation as such or increasing supplementation levels might decrease drip loss and improve the water-holding capacity of the meat under thermoneutral conditions (Liu et al., 2011; Yang et al., 2011). Trace minerals help to sustain the production in animals, improve nutrient utilization and at the same time effectively neutralize the oxidant stress and enhance the compromised immune system of heat-stressed birds (Mir et al., 2018). As the requirements for trace minerals increase during heat stress, the inclusion of a more readily available zinc source, such as ZnAA, might be more efficient in reducing the adverse effects of heat stress on meat quality (Sahin et al., 2009; Chand et al., 2014; Farag and Alagawany, 2018). In addition, Liu et al. (2015) reported that increased dietary supplementation of Zn can upregulate the expression of Zn-containing superoxide dismutase. As the negative impact of high ambient temperatures on meat quality is mainly caused by oxidative damage to the skeletal muscle (Zaboli et al., 2019), the improved quality traits when supplying ZnAA could be ascribed to improved support of the antioxidant defense system (Liu et al., 2015; Kamran Azad et al., 2018). Indeed, a previous study performed by De Grande et al. (2020) showed that ZnAA could decrease the activity of the glutathione peroxidase in plasma on day 36, while malondialdehyde levels did not differ, indicating that ZnAA might better support the oxidative status.
Although it has been acknowledged that vitamin E has a positive effect on meat quality by protecting membranes against lipid oxidation, thus reducing drip loss in meat (Estevez, 2015; Pompeu et al., 2018), no effects could be observed when the dietary vitamin E level was increased in the present study. It is possible that the increase in the level of vitamin E was insufficient to create an impact on meat quality and yield under these conditions; in the recent literature, supplementation at a level of 250 mg/kg was advised to improve meat quality in broiler chickens (Shakeri et al., 2020).
Overall, it can be argued that an organic form of Zn, in particular ZnAA, which is characterized by improved bioavailability (Star et al., 2012; De Grande et al., 2020), might be able to better mitigate lipid and protein oxidation in post-rigor breast muscles and increase both the water-holding capacity and water-binding ability. However, as no thermoneutral control group was incorporated in this study, the observed effects of the zinc source cannot be generalized as a solution for the negative effects of heat stress. Therefore, further research needs to be performed to elucidate the underlying mechanism concerning the effects of zinc sources on meat yield and quality.
In conclusion, comparing ZnSO4 with more readily available ZnAA shows improved breast meat yield and increased water-holding capacity in broilers exposed to chronic cyclic heat stress at the end of the production cycle. Moreover, the beneficial effects of ZnAA on breast meat yield and quality seem to be independent of the vitamin E level, and increasing the vitamin E level has no additional beneficial effects.
Data availability statement
The raw data supporting the conclusion of this article will be made available by the authors, without undue reservation.
Ethics statement
The animal study was reviewed and approved by the Ethical Committee of the Research Institute for Agriculture, Fisheries and Food (ILVO), Merelbeke, Belgium, under authorization number: 2017: 308.
Author contributions
All authors listed have made a substantial, direct, and intellectual contribution to the work and approved it for publication.
Funding
This study received funding from Zinpro Corporation. The funder was not involved in the study design, collection, analysis, interpretation of data, writing of this article, or decision to submit it for publication. All authors declare no other competing interests.
Acknowledgments
The authors thank Jolien Vander Linden, the animal caretaker at the ILVO, and the colleagues of the department at Ghent University for their help with feeding, taking care of the birds, and taking samples.
Conflict of interest
CR and CT are employed by Zinpro corporation. All authors declare no other competing interests.
The remaining authors declare that the research was conducted in the absence of any commercial or financial relationships that could be construed as a potential conflict of interest.
Publisher’s note
All claims expressed in this article are solely those of the authors and do not necessarily represent those of their affiliated organizations, or those of the publisher, the editors, and the reviewers. Any product that may be evaluated in this article, or claim that may be made by its manufacturer, is not guaranteed or endorsed by the publisher.
References
Akbari M., Torki M. (2014). Effects of dietary chromium picolinate and peppermint essential oil on growth performance and blood biochemical parameters of broiler chicks reared under heat stress conditions. Int. J. Biometeorol. 58, 1383–1391. doi:10.1007/s00484-013-0740-1
Attia Y. A., Al-Harthi M. A., El-Shafey A. S., Rehab Y. A., Kim W. K. (2017). Enhancing tolerance of broiler chickens to heat stress by supplementation with vitamin E, vitamin C and/or probiotics. Ann. Anim. Sci. 17, 1155–1169. doi:10.1515/aoas-2017-0012
Aviagen (2018). Ross broiler management handbook [Brochure]. Aviagen group. https://en.aviagen.com/assets/Tech_Center/Ross_Broiler/Ross-BroilerHandbook2018-EN.pdf.
Bowker B., Zhuang H. (2015). Relationship between water-holding capacity and protein denaturation in broiler breast meat. Poult. Sci. 94, 1657–1664. doi:10.3382/ps/pev120
Chand N., Naz S., Khan A., Khan S., Khan R. U. (2014). Performance traits and immune response of broiler chicks treated with zinc and ascorbic acid supplementation during cyclic heat stress. Int. J. Biometeorol. 58, 2153–2157. doi:10.1007/s00484-014-0815-7
Claeys E., Vossen E., De Smet S. (2016). Determination of -tocopherol by reversed-phase HPLC in feed and animal-derived foods without saponification. J. Sci. Food Agric. 96, 522–529. doi:10.1002/jsfa.7119
De Grande A., Leleu S., Delezie E., Rapp C., De Smet S., Goossens E., et al. (2020). Dietary zinc source impacts intestinal morphology and oxidative stress in young broilers. Poult. Sci. 99, 441–453. doi:10.3382/ps/pez525
Estevez M. (2015). Oxidative damage to poultry: From farm to fork. Poult. Sci. 94, 1368–1378. doi:10.3382/ps/pev094
Eu E. D. 2010. European Directive 2010/63/EU of the European Parliament and of the Council of 22 September 2010 on the protection of animals used for scientific purposes (Text with EEA relevance).
Farag M. R., Alagawany M. (2018). Physiological alterations of poultry to the high environmental temperature. J. Therm. Biol. 76, 101–106. doi:10.1016/j.jtherbio.2018.07.012
Feng J., Zhang M., Zheng S., Xie P., Ma A. (2008). Effects of high temperature on multiple parameters of broilers in vitro and in vivo. Poult. Sci. 87, 2133–2139. doi:10.3382/ps.2007-00358
Habibian M., Sadeghi G., Ghazi S., Moeini M. M. (2015). Selenium as a feed supplement for heat-stressed poultry: A review. Biol. Trace Elem. Res. 165, 183–193. doi:10.1007/s12011-015-0275-x
He S. P., Arowolo M. A., Medrano R. F., Li S., Yu Q. F., Chen J. Y., et al. (2018). Impact of heat stress and nutritional interventions on poultry production. World's. Poult. Sci. J. 74, 647–664. doi:10.1017/S0043933918000727
ISO (2016). Animal and vegetable fats and oils—determination of trace elements by inductively coupled plasma optical emission spectroscopy (ICP-OES). Geneva, Switzerland: International Organization for Standardization. ISO standard no. 21033:2016).
ISO (2009). Animal feeding stuffs — determination of calcium, sodium, phosphorus, magnesium, potassium, iron, zinc, copper, manganese, cobalt, molybdenum, arsenic, lead and cadmium by ICP-AES. Geneva, Switzerland: International Organization for Standardization. (ISO standard no. 27085:2009).
Jensen C., Lauridsen C., Bertelsen G. 1998. Dietary vitamin E: Quality and storage stability of pork and poultry. Trends Food Sci. Technol. 9:62. doi:10.1016/S0924-2244(98)00004-1
Kamran Azad S., Shariatmadari F., Karimi Torshizi M. A., Ahmadi H. (2018). Effect of zinc concentration and source on performance, tissue mineral status, activity of superoxide dismutase enzyme and lipid peroxidation of meat in broiler chickens. Anim. Prod. Sci. 58, 1837–1846. doi:10.1071/AN15758
Khan R. U., Naz S., Nikousefat Z., Selvaggi M., Laudadio V., Tufarelli V. (2012). Effect of ascorbic acid in heat-stressed poultry. World's. Poult. Sci. J. 68, 477–490. doi:10.1017/S004393391200058x
Khan R. U., Naz S., Nikousefat Z., Tufarelli V., Javdani M., Rana N., et al. (2011). Effect of vitamin E in heat-stressed poultry. World's. Poult. Sci. J. 67, 469–478. doi:10.1017/S0043933911000511
Lara L. J., Rostagno M. H. (2013). Impact of heat stress on poultry production. Anim. (Basel) 3, 356–369. doi:10.3390/ani3020356
Layne E. 1957. [73] Spectrophotometric and turbidimetric methods for measuring proteins. Methods Enzymol. 3, 447. doi:10.1016/S0076-6879(57)03413-8
Leygonie C., Britz T. J., Hoffman L. C. (2012). Impact of freezing and thawing on the quality of meat: Review. Meat Sci. 91, 93–98. doi:10.1016/j.meatsci.2012.01.013
Liu Z. H., Lu L., Li S. F., Zhang L. Y., Xi L., Zhang K. Y., et al. (2011). Effects of supplemental zinc source and level on growth performance, carcass traits, and meat quality of broilers. Poult. Sci. 90, 1782–1790. doi:10.3382/ps.2010-01215
Liu Z. H., Lu L., Wang R. L., Lei H. L., Li S. F., Zhang L. Y., et al. (2015). Effects of supplemental zinc source and level on antioxidant ability and fat metabolism-related enzymes of broilers. Poult. Sci. 94, 2686–2694. doi:10.3382/ps/pev251
Lu Z., He X. F., Ma B. B., Zhang L., Li J. L., Jiang Y., et al. (2017). Chronic heat stress impairs the quality of breast-muscle meat in broilers by affecting redox status and energy-substance metabolism. J. Agric. Food Chem. 65, 11251–11258. doi:10.1021/acs.jafc.7b04428
Mir S., Pal R., Mani V., Malik T., Ojha L., Yadav S. (2018). Role of dietary minerals in heat-stressed poultry: A review. J. Entomology Zoology Stud. 6, 820
Nen_En_15763 (2010). Foodstuffs - determination of trace elements - determination of arsenic, cadmium, mercury and lead in foodstuffs by inductively coupled plasma mass spectrometry (icp-ms) after pressure digestion Netherlands Standards. Delft, Netherlands: Netherlands Standards.
Petracci M., Betti M., Bianchi M., Cavani C. (2004). Color variation and characterization of broiler breast meat during processing in Italy. Poult. Sci. 83, 2086–2092. doi:10.1093/ps/83.12.2086
Petracci M., Mudalal S., Soglia F., Cavani C. (2015). Meat quality in fast-growing broiler chickens. World's. Poult. Sci. J. 71, 363–374. doi:10.1017/S0043933915000367
Pompeu M. A., Cavalcanti L. F. L., Toral F. L. B. (2018). Effect of vitamin E supplementation on growth performance, meat quality, and immune response of male broiler chickens: A meta-analysis. Livest. Sci. 208, 5–13. doi:10.1016/j.livsci.2017.11.021
Rehman Z. U., Chand N., Khan R. U. (2017). The effect of vitamin E, L-carnitine, and ginger on production traits, immune response, and antioxidant status in two broiler strains exposed to chronic heat stress. Environ. Sci. Pollut. Res. Int. 24, 26851–26857. doi:10.1007/s11356-017-0304-8
Sahin K., Sahin N., Kucuk O., Hayirli A., Prasad A. S. (2009). Role of dietary zinc in heat-stressed poultry: A review. Poult. Sci. 88, 2176–2183. doi:10.3382/ps.2008-00560
Shakeri M., Oskoueian E., Le H. H., Shakeri M. (2020). Strategies to combat heat stress in broiler chickens: Unveiling the roles of selenium, vitamin E and vitamin C. Vet. Sci. 7, 71. doi:10.3390/vetsci7020071
Star L., van der Klis J. D., Rapp C., Ward T. L. (2012). Bioavailability of organic and inorganic zinc sources in male broilers. Poult. Sci. 91, 3115–3120. doi:10.3382/ps.2012-02314
Swiatkiewicz S., Arczewska-Wlosek A., Jozefiak D. (2014). The efficacy of organic minerals in poultry nutrition: Review and implications of recent studies. World's. Poult. Sci. J. 70, 475–486. doi:10.1017/s0043933914000531
Wang R. H., Liang R. R., Lin H., Zhu L. X., Zhang Y. M., Mao Y. W., et al. (2017). Effect of acute heat stress and slaughter processing on poultry meat quality and postmortem carbohydrate metabolism. Poult. Sci. 96, 738–746. doi:10.3382/ps/pew329
Wang R. R., Pan X. J., Peng Z. Q. (2009). Effects of heat exposure on muscle oxidation and protein functionalities of pectoralis majors in broilers. Poult. Sci. 88, 1078–1084. doi:10.3382/ps.2008-00094
Woelfel R. L., Owens C. M., Hirschler E. M., Martinez-Dawson R., Sams A. R. (2002). The characterization and incidence of pale, soft, and exudative broiler meat in a commercial processing plant. Poult. Sci. 81, 579–584. doi:10.1093/ps/81.4.579
Yang X. J., Sun X. X., Li C. Y., Wu X. H., Yao J. H. (2011). Effects of copper, iron, zinc, and manganese supplementation in a corn and soybean meal diet on the growth performance, meat quality, and immune responses of broiler chickens. J. Appl. Poult. Res. 20, 263–271. doi:10.3382/japr.2010-00204
Zaboli G., Huang X., Feng X., Ahn D. U. (2019). How can heat stress affect chicken meat quality? - a review. Poult. Sci. 98, 1551–1556. doi:10.3382/ps/pey399
Zhang Z. Y., Jia G. Q., Zuo J. J., Feng D. Y., Lei J., Ren L., et al. (2012). Effects of constant and cyclic heat stress on muscle metabolism and meat quality of broiler breast fillet and thigh meat. Poult. Sci. 91, 2931–2937. doi:10.3382/ps.2012-02255
Keywords: zinc, vitamin E, broiler, carcass yield, meat quality, heat stress
Citation: De Grande A, Ducatelle R, Leleu S, Rapp C, Torres C, Petracci M, De Smet S, Michiels J, Haesebrouck F, Van Immerseel F and Delezie E (2022) Effects of the dietary zinc source and vitamin E level on live weight and carcass yield and meat quality in male broilers reared under chronic cyclic heat stress conditions in the finisher phase. Front. Physiol. 13:992689. doi: 10.3389/fphys.2022.992689
Received: 12 July 2022; Accepted: 12 September 2022;
Published: 06 October 2022.
Edited by:
Krystyna Pierzchala-Koziec, University of Agriculture in Krakow, PolandReviewed by:
Cecep Hidayat, National research and innovation agency of Indonesia, IndonesiaGerardo Mendez-Zamora, Autonomous University of Nuevo Leon, Mexico
Copyright © 2022 De Grande, Ducatelle, Leleu, Rapp, Torres, Petracci, De Smet, Michiels, Haesebrouck, Van Immerseel and Delezie. This is an open-access article distributed under the terms of the Creative Commons Attribution License (CC BY). The use, distribution or reproduction in other forums is permitted, provided the original author(s) and the copyright owner(s) are credited and that the original publication in this journal is cited, in accordance with accepted academic practice. No use, distribution or reproduction is permitted which does not comply with these terms.
*Correspondence: Annatachja De Grande, QW5uYXRhY2hqYS5EZUdyYW5kZUBpbHZvLnZsYWFuZGVyZW4uYmU=