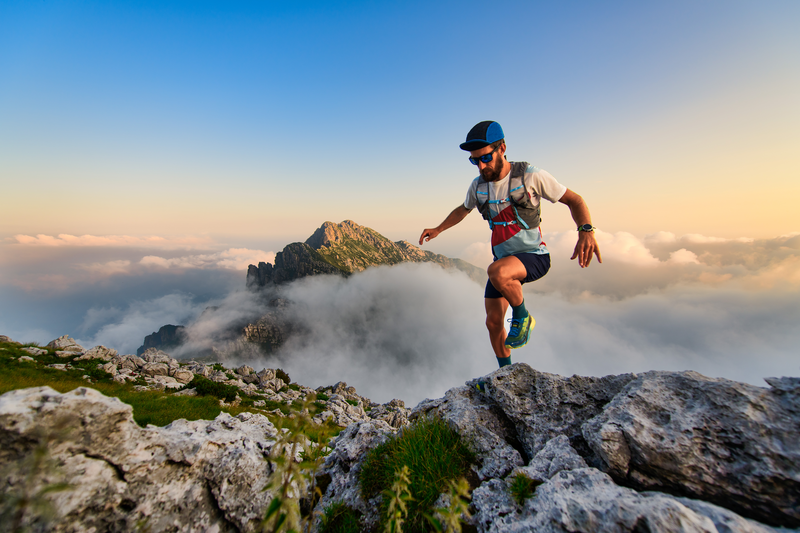
94% of researchers rate our articles as excellent or good
Learn more about the work of our research integrity team to safeguard the quality of each article we publish.
Find out more
ORIGINAL RESEARCH article
Front. Physiol. , 03 February 2023
Sec. Avian Physiology
Volume 13 - 2022 | https://doi.org/10.3389/fphys.2022.991318
This article is part of the Research Topic Insights into the role of trace minerals in poultry nutrition: implications on animal physiology and metabolism, performances, environmental issues and product quality. View all 6 articles
Copper (Cu) is widely used at high levels as growth promoter in poultry, the alternative source of Cu to replace the high level of inorganic Cu at poultry farm remains to be determined. Three floor pen experiments were conducted to evaluate the effects of Cu methionine hydroxy-analogue chelate (Cu-MHAC, MINTREX®Cu, Novus International, Inc.) on growth performance and gut health in broilers in comparison to CuSO4 and/or tribasic copper chloride (TBCC). There were 3 treatments in experiment#1 (0, 30 and 75 ppm Cu-MHAC) and experiment#2 (15 and 30 ppm Cu-MHAC, and 125 ppm CuSO4), and 4 treatments in experiment #3 (15 and 30 ppm Cu-MHAC, 125 ppm CuSO4 and 125 ppm TBCC) with nine replicates pens of 10–13 birds in each treatment. The levels of other minerals were equal among all treatments within each experiment. All birds were orally gavaged with a coccidiosis vaccine at 1x recommended dose on d0 in experiment#1 and #2 and 10x recommended dose on d15 in experiment #3. Data were analyzed by one-way ANOVA, means were separated by Fisher’s protected LSD test. A p ≤ 0.05 was considered statistically different. In experiment #1, 30 and 75 ppm Cu-MHAC improved FCR during grower phase, increased jejunal villus height and reduced jejunal crypt depth, 30 ppm Cu-MHAC increased cecal Lactobacillus spp. abundance in 41 days broilers. In experiment #2, compared to CuSO4, 15ppm Cu-MHAC increased cumulative performance index in 28 days broilers, 15 and/or 30 ppm Cu-MHAC improved gut morphometry, and 30 ppm Cu-MHAC reduced the abundance of E. coli and Enterobacteriaceae in cecum in 43 days broilers. In experiment #3, 15 ppm and 30 ppm Cu-MHAC improved FCR vs. CuSO4 during starter phase, reduced the percentage of E. coli of total bacteria vs. TBCC, 30 ppm Cu-MHAC increased the percentages of Lactobacillus acidophilus, Lactobacillus spp. and Clostridium cluster XIVa of total bacteria vs. both CuSO4 and TBCC in the cecum of 27 days broilers. In summary, low doses of Cu-MHAC had comparable growth performance to high dose of TBCC and CuSO4 while improving gut microflora and gut morphometry in broilers subject to coccidiosis vaccination or coccidia challenge, indicating that low doses of bis-chelated Cu could be used as a complimentary strategy to improve animal gut health.
Copper (Cu) is widely used in concentrations in excess of the nutritional requirements as growth promoter and antimicrobial in poultry industry, however, the total amounts and concentrations of Cu used in feed may differ among countries due to different restrictions imposed by national legislation. The mechanism by which high levels of Cu promote growth remains to be determined. One of the possible mechanisms by which Cu may benefit birds is by shifting gut microbiota, thereby reducing susceptibility of birds to diseases and decreasing inflammation (Arias and Koutsos, 2006) and therefore increasing nutrient absorption (Hawbaker et al., 1961; Bunch et al., 1965). Although it’s commonly recognized that Cu exerts anti-microbial effect in the gut (Borkow and Gabbay, 2005; Borkow and Gabbay, 2009), there is not much direct and consistent data showing that Cu alters the population of bacterial species in poultry. Kim et al. (2011) showed that 100 ppm Cu-methionine and Cu-soy proteinate increased the population of Lactobacillus and decreased the population of E. coli and Clostridium perfringens in ileum, but 50 ppm Cu-methionine and Cu-soy proteinate were not effective. Pang et al. (2009) showed that 187.5 ppm CuSO4 and tribasic copper chloride (TBCC) increased Lactobacilli and decreased E. Coli in vitro, but did not impact the number of ileal Lactobacilli in birds. 150 ppm Cu2O decreased the abundance of some pathogenic bacterial families such as Streptococcaceae and Corynebacteriaceae and increased the abundance of some commensal bacteria like Clostridiaceae and Peptostreptococcaceae in broilers (Forouzandeh et al., 2021). More studies are needed to better understand how different sources of Cu modulate gut microbiota in broilers.
Cu is a cofactor of lysyl oxidase, an enzyme that crosslinks collagen subunits into mature protein forms to increase their strength, and it plays an important role in collagen crosslinking (Vallet and Ricard-Blum, 2019), therefore, Cu could help to maintain and improve the structural integrity of connective tissue such as skin, skeletal muscle, intestine and tendon (Richards et al., 2010). 100 and 200 ppm of Cu hydroxychloride supplementation increased jejunal villus height (Nguyen et al., 2022). Pharmacological levels (188 ppm) of CuSO4 and TBCC decreased the number of lamina propia lymphocytes and/or intraepithelial lymphocytes in duodenum and jejunum and duodenal crypt depth in comparison to negative control without Cu supplementation in birds raised on used litter, TBCC reduced jejunal crypt depth and increased ileal intraepithelial lymphocytes compared to antibiotics positive control and increased duodenal intraepithelial lymphocytes compared to negative control in broilers raised on fresh litter (Arias and Koutsos, 2006). These results suggest that Cu improved gut morphometry and reduced intestinal inflammation when birds were under “high” microbial environment challenge (Arias and Koutsos, 2006). Previous studies showed that Cu methionine hydroxy analog chelate (Cu-MHAC, MINTREX®Cu, Novus International, Inc.), a bis-chelated Cu, improved ileal breaking strength compared to Cu sulfate, Cu lysine and Cu proteinate (Richards et al., 2010), indicating that Cu could also improve mechanical property of intestine probably by promoting collagen cross-linking.
There is growing concern that heavy metals, such as Cu, could act as a selective pressure to force the proliferation and evolution of Cu-resistant and antibiotic-resistant bacteria at the farm and in the environment, both commensal and pathogenic enteral bacteria in farmed animals that developed resistance to trace elements such as Zn and Cu and concomitant cross-resistance to antimicrobial agents may be transferred to other animals and human (Yazdankhah et al., 2014). Trace elements such as Cu could also be toxic to bacteria due to their chemical affinity to the thiol groups of macro-biomolecules and their solubility under physiological conditions (Yazdankhah et al., 2014). Yu et al. (2017) commented that possible solutions to reduce the impact of heavy metal resistance include using organic trace mineral and reducing the supplemental levels of trace minerals in animal feed rations. In addition, the inclusion levels of Cu in complete poultry feed with a moisture level of 12% was approved to be less than 25 mg/kg feed in Europe Union (EU copper reduction plans officially approved, 2018) (https://www.allaboutfeed.net/animal-feed/feed-additives/eu-copper-reduction-plans-officially-approved), which indicates that highly bioavailable sources of Cu are needed to supplement at low levels to provide sufficient Cu for the growth of birds and in the meantime to comply the EU regulation.
Coccidiosis is a major intestinal challenge that causes economic loss to the broiler industry. The global cost of coccidiosis including losses during production and costs for prophylaxis and treatment in chickens is estimated to have been ∼ £10.36 billion at 2016 prices, which is equivalent to £0.16/chicken produced (Blake et al., 2020). In recent years, more and more poultry producers are looking into natural approaches to control coccidiosis, such as essential oils, probiotics, prebiotics, trace minerals, and other gut health enhancing products, which could be complimentary to the coccidiosis control programs. Chen et al. (2022) showed that supplementation of both bis-chelated mineral methionine hydroxy analog chelates (MMHAC) and high levels of total sulfur amino acid could overcome the growth performance challenges due to coccidiosis. It has been reported that Cu requirements were higher for chickens experiencing an acute phase response or pathological challenge compared to healthy chickens (Koh et al., 1996). For example, the chicks infected with Eimeria (E.) tenella had significantly elevated levels of serum copper and ceruloplasmin and liver copper than their pair-fed counterparts (Richards and Augustine., 1988). Different sources of organic and inorganic Cu sources are commercially available for poultry producers to use at different doses. Although there are numerous studies investigating the role of Cu supplementation in broilers subjected to coccidiosis (Rochell et al., 2017; Bortoluzzi et al., 2020; Dos Santos et al., 2020; Zaghari et al., 2022), the efficacy of different sources and doses of Cu supplementation in growth performance and gut health parameters of broilers subjected to coccidiosis vaccination or coccidia challenge is still inconsistent. The objective of this study was to determine an alternative source of Cu to replace high level of inorganic Cu in broilers subject to coccidiosis vaccination or coccidia challenge in poultry farm. Bis-chelated Cu, Cu-MHAC, has been reported to be a highly bioavailable Cu source (Wang et al., 2007), multiple low doses of Cu-MHAC were tested in three experiments to determine whether the low doses of Cu-MHAC could have similar growth performance as high dose of CuSO4 and/or TBCC while improving gut morphology and cecal microbiota in broilers subjected to coccidiosis vaccination or coccidia challenge.
Guide for the Care and Use of Agricultural Animals in Research and Teaching (FASS, 2010) was followed for housing and care of the animals throughout the experiments. All research procedures were reviewed and approved by the animal ethics committee composed of members from Novus International Inc (St. Charles, MO 63304) and a licensed veterinarian from Bridgeton Animal hospital (Bridgeton, MO 63044). Ross 308 male broilers were purchased from a local hatchery (Stover Hatchery, Stover, MO). Upon arrival, birds were placed immediately in 36 floor pens of 6 sqft with 10–13 birds per pen in an environmentally controlled room. Water was supplied to each pen via a nipple drinker system. Each pen had two drinkers and one hanging plastic feeder. Heat was furnished via natural gas fired radiant tube heaters and each pen was equipped with a brooding heat lamp. Test diets and water were provided for ad libitum consumption throughout the experiments. A 3 cm layer of used litter as bedding material was applied uniformly in each pen across all treatments on the first day of the experiment. The room was preheated to 27°C 2 days prior to study and kept at 27°C from 0 to 14 days. Room temperature was reduced to 26°C on d 14, 24°C on d 21, and 21°C on d 24 and kept at 21°C until the end of the experiment. For the first week, 23 h of light was provided. The light period was reduced to 20 h from d 8 to 39 and back to 23 h on d 40 until the end of the experiment.
Experimental diets were formulated to meet nutritional requirements of broiler chickens except for minerals. Composition and calculated nutrient profile of starter, grower, and finisher diets in three experiments were shown in Tables 1–3. Formulated and analyzed Cu concentration were shown in Table 4. For each phase, a common basal diet was made to reduce variation among test diets from weighing and mixing; aliquots of the basal diet were then supplemented with different sources of minerals. The methionine contribution from 2-hydroxy-4-methylthiobutyric acid (HMTBa) in Cu methionine hydroxy-analogue chelate (Cu-MHAC, MINTREX®Cu, Novus International, Inc.) was taken into consideration, and all final test diets were formulated to contain the same amount of supplemental HMTBa within each experiment. The starter diets were offered in crumbled, grower and finisher diets in pellet form with the pelleting temperature of 85°C. Birds were weighed by pen on d0, 14, 28 and 40 (Experiment #1) or d14, 28 and 42 (Experiment #2 and #3). On each weigh day other than d 0, feed consumption on pen basis was also determined. Mortality was checked and recorded twice daily, and weights of the dead birds were used to adjust feed conversion ratio (FCR). Cumulative Performance Index (cPI) was calculated as (cumulative livability*body weight*100/day of study)/cumulative FCR.
TABLE 1. Composition (%) and calculated nutrient content of basal diets during starter, grower and finisher phases in experiment #1.
TABLE 2. Composition (%) and calculated nutrient content of basal diets during starter, grower and finisher phases in experiment #2.
TABLE 3. Composition (%) and calculated nutrient content of basal diets during starter, grower and finisher phases in experiment #3.
A total of 351 day-old Ross 308 male broilers were randomly assigned to one of the 3 treatments: 0, 30 or 75 ppm Cu-MHAC (MINTREX®Cu, Novus International, Inc.). Supplemental levels of Zn (32 ppm) and Mn (32 ppm) from MHAC (MINTREX®Zn:Mn, Novus International, Inc.), Fe (40 ppm), I (1.25 ppm), and Se (0.3 ppm) from inorganic sources were equal among 3 treatments. Each diet was fed to nine replicate pens of 13 broilers with randomized complete block design. Broilers were vaccinated with 1× recommended dose of coccidiosis vaccine (mixed species of E. acervulina, E. tenella, and E. maxima from Huvepharma, Inc.) by oral gavage on d0. On d41, 1 bird/pen was sacrificed to collect duodenum and jejunum tissue for gut morphometry measurement and cecal content for bacteria quantitation.
A total of 270 day-old Ross 308 male broilers were randomly assigned to one of the 3 treatments: 15 or 30 ppm Cu-MHAC (MINTREX®Cu, Novus International, Inc) or 125 ppm CuSO4. Supplemental levels of Zn (100 ppm), Mn (90 ppm), Fe (40 ppm), I (1.25 ppm), and Se (0.3 ppm) from inorganic sources were equal among 3 treatments. Each diet was fed to nine replicate pens of 10 broilers with randomized complete block design. Broilers were vaccinated with 1× recommended dose of coccidiosis vaccine as described above in Experiment #1 by oral gavage on d0. On d43, 1 bird/pen was sacrificed to collect duodenum, jejunum and ileum tissue for gut morphometry measurement and cecal content for bacteria quantitation.
A total of 468 day-old Ross 308 male broilers were randomly assigned to one of the 4 treatments: 15 or 30 ppm Cu-MHAC (MINTREX®Cu, Novus International, Inc.), 125 ppm CuSO4, or 125 ppm TBCC. Supplemental levels of Zn (100 ppm), Mn (90 ppm), Fe (40 ppm), I (1.25 ppm), and Se (0.3 ppm) from inorganic sources were equal among 3 treatments. Each diet was fed to nine replicate pens of 13 broilers with randomized complete block design. On d15, broilers were challenged with 10× recommended dose of coccidiosis vaccine as described above in Experiment #1 by oral gavage. On d29, 1 bird/pen was sacrificed to collect cecal content for bacteria quantitation.
Duodenum, jejunum and ileum tissue were taken, flushed with formalin-free histology fixative (NOTOXhisto Fixative; Scientific Device Laboratory; Des Plaines. IL) and fixed with NOTOXhisto for 4 weeks. Fixed intestine tissue was trimmed in cross-sections, processed, embedded in paraffin and sliced in cross-sections. A 5 μm section of each sample was placed on a glass slide and stained with hematoxylin and eosin for morphometry examination and measurement under Olympus light microscope. In each intestine cross-section, five replicates of each variable were measured from each sample using ZEN lite 2012 Imaging software (ZEISS Microscopy; Thornwood, NY). A total of representative 5 villus and 5 crypts in each slide were selected to measure villus height, villus width, crypt depth. Muscularis layer thickness, which includes muscularis propria, subserosa and serosa, was measured at 5 representative locations of each intestine slide. Villus height was measured from the top of the villus to the top of the lamina propria. Crypt depth was measured from the base upwards to the region of transition between the crypt and villus. Villus width was measured at the middle of each villus, whereas crypt depth to villus height ratio (CVR) was determined as the ratio of crypt depth to villus height, while villus height to villus width ratio (HWR) was determined as the ratio of villus height to villus width.
Cecal content was collected from each bird and snap-frozen in liquid nitrogen and stored at -20°C freezer until sample analysis. Genomic DNA was extracted from cecal content using PowerLyzer® PowerSoil® DNA Isolation Kit (Qiagen, Germantown, MD). DNA concentration was quantified and 8 ng of cecal DNA was used to measure the abundance of bacteria by quantitative polymerase chain reaction (qPCR) using SYBR Green in the QuantStudio 5 RealTime PCR System (Applied Biosystems; Foster City, CA) in a 384-well plate and primers described in Table 5. All primers were verified for efficiency (90% ± 10%) and linearity of amplification (r2 ≥ 0.99). The abundance of different bacteria per ng DNA from cecal contents at family or specie levels was expressed as Ct value per ng DNA, lower Ct means higher abundance, and vice versa, higher Ct means lower abundance. The relative abundance of bacteria was expressed as the percentage % of total bacteria by calculating delta Ct of different bacteria verse (vs) total bacteria with 1 Ct difference equaling 2-fold difference in bacterial abundance.
All data were subjected to analysis of variance as a randomized complete block design using the PROC MIXED procedure of SAS 9.4. Pen was used as the experimental unit for the analysis. Data were analyzed by one-way ANOVA, means were separated by Fisher’s protected LSD test. The incidences of wooden breast were analyzed by Chi-square test. A p-value ≤ 0.05 was considered statistically different. A p-value 0.05< p ≤ 0.10 was considered a numerical trend.
In experiment #1, 30 ppm and 75 ppm Cu-MHAC supplementation improved period FCR by 2.8 and 2.6 points, respectively, during grower phase (d15-27) in comparison to control without Cu supplementation and had no effect on other growth performance parameters at different time points (Table 6). Compared to control, 30 ppm and 75 ppm Cu-MHAC increased (p < 0.05) villus height and HWR and reduced (p < 0.05) crypt depth and CVR in jejunum but not in duodenum (Table 7).
Cecal DNA was extracted to measure bacterial population by qPCR. Supplementation of 30 ppm Cu-MHAC significantly (p < 0.05) decreased the Ct of Lactobacillus spp per ng of cecal DNA (Table 8), indicating that it increased the abundance of Lactobacillus spp in cecum. No differences in the abundance and relative abundance of other bacteria were detected between treatments. It’s unexpected that 75 ppm Cu-MHAC did not alter the abundance of Lactobacillus spp and Lactobacillus acidophilus.
TABLE 8. Ct of E. coli, Lactobacillus acidophilus, Lactobacillus spp. and Enterobacteriaceae per ng of cecal DNA, and percentage (%) of E. Coli, Lactobacillus L.) Acidophilus, L.spp. and Enterobacteriaceae (% of total bacteria) in cecum of broilers at 41 days of age of experiment #1.
There was no significant treatment difference of growth performance during all phases except for the improvement (p < 0.04) of d28 cumulative performance index by supplementation of 15 ppm Cu-MHAC in comparison to 125 ppm CuSO4, which resulted from the numerical increase of body weight and cumulative FCR (Table 9).
In birds at 42 days of age, compared to 125 ppm CuSO4, 15 ppm Cu-MHAC reduced the thickness of muscularis layer in jejunum (p = 0.0243) and ileum (p = 0.0424), and crypt depth in ileum (p = 0.0493); 30 ppm Cu-MHAC reduced the muscular layer thickness in duodenum (p = 0.0405) (Table 10).
Compared with 125 ppm CuSO4, 30 ppm Cu-MHAC significantly (p < 0.05) reduced the abundance of Escherichia coli (E. coli) and Enterobacteriaceae in cecum of birds of 42 days of age (Table 11).
TABLE 11. Ct of Firmicutes, E. Coli, Lactobacillus Acidophilus, Lactobacillus spp. and Enterobacteriaceae per ng of cecal DNA, and percentage (%) of Firmicutes, E. Coli, Lactobacillus Acidophilus, Lactobacillus spp. and Enterobacteriaceae (% of total bacteria) in broilers at 43 days of age of experiment #2.
15 and 30 ppm of Cu-MHAC significantly improved (p < 0.05) d0-14 FCR by 2.8 and 3 points, respectively, compared to CuSO4 treatment, TBCC was intermediate (Table 12). No significant difference was observed for body weight, period FCR, cumulative FCR, feed intake and performance index on d14. Neither dose of Cu-MHAC improved growth performance parameters during grower and finisher phase (Table 12).
The abundance of different bacteria including total bacteria per ng DNA of cecal contents was not different among 4 treatments (Table 13). The relative abundance of these bacteria expressed as their percentage in total bacteria were also compared among 4 treatments (Table 13). 30 ppm Cu-MHAC increased (p < 0.05) the relative abundance of Lactobacillus acidophilus, Lactobacillus spp. and Clostridium cluster XIVa in comparison to CuSO4 and TBCC treatments. Both 15 and 30 ppm Cu-MHAC decreased (p < 0.05) the relative abundance of E. Coli compared to TBCC, but not different from CuSO4 treatment. The relative abundance of Firmicutes and Bacteroidetes at phylum levels were not different among 4 treatments.
TABLE 13. The Ct of E. coli, Lactobacillus acidophilus, Lactobacillus spp. and Clostridium cluster XIVa (% of total bacteria) per ng of cecal DNA and the relative population (%) of E. coli, Lactobacillus acidophilus, Lactobacillus spp. and Clostridium cluster XIVa (% of total bacteria) in the cecum of broilers at 29 days of age in experiment #3.
In all three experiments, Cu-MHAC improved a few growth performance parameters during either starter or grower phases, but not during finisher phase in Eimeria vaccinated or challenged birds.
Cu is required for the development and maintenance of immune system and Cu deficiency influences the ability of animals to maintain their immunity (Percival, 1998). Oxidative stress and inflammation are frequently involved in enteric diseases of broilers, and they are part of normal defense mechanisms against pathogens (Lauridsen, 2019). Inflammation is a generic response that is considered as a mechanism of innate immunity fighting against pathogens (Lauridsen, 2019). Individuals suffering from oxidative stress might correspondingly have weak immune responses in order to minimize oxidative damage (Cram et al., 2015).
Cu-MHAC is more bioavailable than ITM (Wang et al., 2007) and MMHAC has been reported to improve antioxidant status in broilers and lactating Holstein cow and vaccine-induced anti-M. hyopneumoniae antibody titers in gilts (Richards et., al., 2010; Zhao et al., 2015). On the other hand, inorganic Cu especially when supplemented at high levels could become pro-oxidants and increase reactive oxygen species and malondialdehyde leading to oxidative stress (Yang et al., 2019). Song et al., (2021) reported that immune system and its function are not well developed from d6 to d13, and not mature until d30 to 34 in the broiler chickens in cages, and it is necessary to enhance the immune function of the broiler chickens through nutritional supplementation from d1 to 30. In our studies, birds were reared in floor pens, and they would develop immunity to Eimeria after a few cycles of Eimeira infection, it’s possible that Cu-MHAC either improved immunity development before Eimeria challenge during starter phase or boosted immunity during grower phase, therefore, the growth performance was improved to a certain degree during starter and/or grower phase but not during finisher phase when birds gained full immunity against Eimeira. These hypotheses warrant further investigation in future studies.
Overall, low dose of Cu-MHAC supplementation had similar growth performance as high dose of CuSO4 and TBCC in broilers.
The villi-crypt unit in intestinal epithelium is responsible for nutrient absorption (GÜunther et al., 2013). The epithelial cells near the villous tip have the strongest digestion and absorption ability of nutrients (Hampson, 1986), therefore, more epithelial cells and longer villus height could increase nutrients absorption (Thomson and Keelean, 1986), increase of villus height and villus height/villus width ratio indicates greater villus absorption capacity, and vice versa, decrease of villus height and villus height/villus width ratio indicates lower villus absorption capacity. For example, the significant decrease of villi length contributes to reduced digestive capacity in postweaning piglets (Montagne et al., 2007). In study #1, 30 ppm and 75 ppm Cu-MHAC increased (p < 0.05) jejunal villus height and villus height/villus width ratio, suggesting that Cu-MHAC probably improved jejunal villus absorption capacity. Consistent with this study, Nguyen et al. (2022) reported that feeding 100 and 200 ppm Cu hydroxychloride increased jejunal villus height in broilers.
The enterocyte in intestinal epithelium has high turnover rate and renews itself every 4–5 days, the enterocyte turnover occurs with cell loss in the intestinal lumen due to apoptosis of epithelial cells near the villous tip, then the cells in the crypts will proliferate and migrate towards the apex of the villi to replace the cell loss (GÜunther et al., 2013). Therefore, decreased crypt depth and crypt depth/villus height ratio indicate slower enterocyte turnover, which would reduce the nutrient and energy needed for gut maintenance and reserve more nutrient and energy for animal growth and tissue development. 30 and 75 ppm Cu-MHAC reduced (p < 0.05) jejunal crypt depth and crypt depth/villus height ratio in experiment #1. 15 ppm Cu-MHAC reduced ileal crypt depth in experiment #2. These results suggest that Cu-MHAC likely slowed down the enterocyte turnover which could help save nutrients and energy for potential animal growth and tissue development.
Thickening of muscularis propria has been reported to be positively correlated with chronic inflammation in Crohn’s disease patients (Chen et al., 2017). 15 and/or 30 ppm Cu-MHAC decreased the thickness of muscularis layer in multiple sections of intestine compared to CuSO4 in experiment #2 suggesting that Cu-MHAC probably reduced intestinal inflammation.
Collectively, dietary inclusion of Cu-MHAC in broiler diets improved gut structural integrity with greater villus height, shorter crypt depth and/or thinner muscularis layer thickness.
One of the possible mechanisms by which Cu may benefit birds is by shifting the gut microbiota, thereby reducing susceptibility of birds to diseases and decreasing intestinal lymphocyte recruitment and infiltration (Arias and Koutsos, 2006) and thus increasing nutrient absorption (Hawbaker et al., 1961; Bunch et al., 1965). Although Cu has been widely accepted as growth promoter due to its antimicrobial effect (Borkow and Gabbay, 2005; Borkow and Gabbay, 2009), consistent data to demonstrate how Cu modulates gut microbiota is still lacking in poultry. Supplementation of high levels (187.5 ppm) of Cu from either CuSO4 or Cu hydroxychloride did not alter the number of ileal Lactobacillus in broiler chickens (Pang et al., 2009). Increasing the dose of dietary Cu hydroxychloride and CuSO4 non-selectively and linearly reduced the population of both beneficial bacteria Lactobacillus and pathogenic groups Bacteroides and Enterobacteriaceae in cecum of broilers (Nguyen et al., 2022), which is similar to a newly weaned pig study in which CuSO4 supplementation decreased the counts of Lactobacilli and Enterobacteriaceae in the cecum (Mei et al., 2010). Inclusion of 36.75 ppm of Cu-bearing montmorillonite in broiler diets reduced the total viable counts of E. coli and Clostridium in the small intestine and cecum, but CuSO4 had no effect (Xia et al., 2004). These findings suggest that different levels and sources of Cu have inconsistent effects on bacteria populations.
Unlike those findings, in the current experiments, 30 ppm Cu-MHAC increased (p < 0.05) the relative abundance of beneficial bacteria, Lactobacillus spp. Clostridium Cluster XIVa and/or Lactobacillus acidophilus in comparison to CuSO4 and TBCC or negative control, and reduced the abundance of E. Coli and/or Enterobacteriaceae in comparison to CuSO4 or TBCC, 15 ppm Cu-MHAC reduced the percentage of E. Coli of total bacteria compared to TBCC.
Lactobacilli are present throughout the gastrointestinal tract of poultry and have various biochemical properties, such as producing antibacterial compounds (Gomes and Malcata, 1999) and possessing potential anti-inflammatory and antioxidant activity (Wu et al., 2013; Oh et al., 2018; Li et al., 2019; Talib et al., 2019; Müller et al., 2021). Clostridium cluster XIVa includes many known butyrate-producing bacteria and plays beneficial roles in the regulation of intestinal inflammation in experimental mouse models (Van den Abbeele et al., 2013; Onrust et al., 2015). Increase of Lactobacillus and Clostridium cluster XIVa could potentially improve broiler health by exhibiting antimicrobial, anti-inflammatory and antioxidant benefits. These results revealed the benefits of Cu-MHAC over inorganic forms of Cu, CuSO4 or TBCC, by shifting the gut microbiota to more beneficial microflora. This is probably due to the greater bioavailability of Cu-MHAC (Wang et al., 2007) and additional benefits of the chelate ligand, HMTBa, an organic acid form of methionine that exhibits antimicrobial effect (Guo et al., 2022). The improvement of gut morphometry could be the indirect outcome of anti-microbial effect of Cu-MHAC.
In summary, dietary inclusion of low levels (15–30 ppm) of Cu-MHAC had comparable growth performance as high level of TBCC and CuSO4 while improving gut microflora and gut morphometry in broilers subject to coccidiosis vaccination or coccidia challenge. 15–30 ppm Cu-MHAC could be used to replace high levels of CuSO4 and or TBCC supplementation in broiler diets and used as a complimentary strategy to improve animal gut health.
The original contributions presented in the study are included in the article/supplementary material, further inquiries can be directed to the corresponding authors.
The animal study was reviewed and approved by Novus International, Inc. Animal Ethics Committee.
JC carried out the project, data analysis, interpreted the results, and prepared the manuscript. FY formulated diets. All authors contributed to experimental design, discussed the results, and reviewed the manuscript.
The studies were funded by Novus International Inc.
JC, FY, VK, KW, MV, and DH were employed by the company Novus International, Inc.
All claims expressed in this article are solely those of the authors and do not necessarily represent those of their affiliated organizations, or those of the publisher, the editors and the reviewers. Any product that may be evaluated in this article, or claim that may be made by its manufacturer, is not guaranteed or endorsed by the publisher.
Arias V. J., Koutsos E. A. (2006). Effects of copper source and level on intestinal physiology and growth of broiler chickens. Poult. Sci. 85, 999–1007. doi:10.1093/ps/85.6.999
Blake D. P., Knox J., Dehaeck B., Huntington B., Rathinam T., Ravipati V., et al. (2020). Re-calculating the cost of coccidiosis in chickens. Vet. Res. 51 (1), 115. doi:10.1186/s13567-020-00837-2
Borkow G., Gabbay J. (2005). Copper as a biocidal tool. Curr. Med. Chem. 12, 2163–2175. doi:10.2174/0929867054637617
Borkow G., Gabbay J. (2009). Copper, an ancient remedy returning to fight microbial, fungal and viral infections. Curr. Chem. Biol. 3, 272–278. doi:10.2174/187231309789054887
Bortoluzzi C., Vieira B. S., Applegate T. J. (2020). Influence of dietary zinc, copper, and manganese on the intestinal health of broilers under Eimeria challenge. Front. Vet. Sci. 7, 13. doi:10.3389/fvets.2020.00013
Bunch R. J., Mccall J. T., Speer V. C., Hays V. W. (1965). Copper supplementation for weanling pigs. J. Anim. Sci. 24, 995–1000. doi:10.2527/jas1965.244995x
Chen J., Yan F., Kuttappan V. A., Cook K., Buresh B., Roux M., et al. (2022). Effect of methionine and trace minerals (zinc, copper and manganese) supplementation on growth performance of broilers subjected to Eimeria challenge. Front. Physiol. Accept. 13, 991320. doi:10.3389/fphys.2022.991320
Chen W., Lu C., Hirota C., Iacucci M., Ghosh S., Gui X. (2017). Smooth muscle hyperplasia/hypertrophy is the most prominent histological change in crohn's fibrostenosing bowel strictures: A semiquantitative analysis by using a novel histological grading scheme. J. Crohns Colitis. 11 (1), 92–104. doi:10.1093/ecco-jcc/jjw126
Cram D. L., Blount J. D., York J. E., Young A. J. (2015). Immune response in a wild bird is predicted by oxidative status, but does not cause oxidative stress. PLoS One 10 (3), e0122421. doi:10.1371/journal.pone.0122421
Dos Santos T. S., Teng P. Y., Yadav S., Castro F. L. S., Gould R. L., Craig S. W., et al. (2020). Effects of inorganic Zn and Cu supplementation on gut health in broiler chickens challenged with Eimeria spp. Front. Vet. Sci. 7, 230. doi:10.3389/fvets.2020.00230
EU copper reduction plans officially approved (2018). Feed additives. Available at: https://www.allaboutfeed.net/animal-feed/feed-additives/eu-copper-reduction-plans-officially-approved/. [Accessed 30 07 2018].
FASS (2010). Guide for care and use of agricultural animals in research and teaching. 3rd Edition. Champaign, IL: Federation of Animal Science Societies.
Forouzandeh A., Blavi L., Abdelli N., Melo-Duran D., Vidal A., Rodríguez M., et al. (2021). Effects of dicopper oxide and copper sulfate on growth performance and gut microbiota in broilers. Poult. Sci. 100 (8), 101224. doi:10.1016/j.psj.2021.101224
Gomes A. M. P., Malcata F. X. (1999). Bifidobacterium spp. and Lactobacillus acidophilus: Biological, biochemical, technological and therapeutical properties relevant for use as probiotics. Trends Food Sci. Technol. 10 (4-5), 139–157. doi:10.1016/s0924-2244(99)00033-3
Guo Y. J., Wang Z. Y., Wang Y. S., Chen B., Huang Y. Q., Li P., et al. (2022). Impact of drinking water supplemented 2-hydroxy-4-methylthiobutyric acid in combination with acidifier on performance, intestinal development, and microflora in broilers. Poult. Sci. 101 (3), 101661. doi:10.1016/j.psj.2021.101661
Güunther C., Neumann H., Neurath M. F., Becker C. (2013). Apoptosis, necrosis and necroptosis: Cell death regulation in the intestinal epithelium. Gut 62 (7), 1062–1071. doi:10.1136/gutjnl-2011-301364
Hampson D. J. (1986). Alterations in piglet small intestinal structure at weaning. Res. Vet. Sci. 40, 32–40. doi:10.1016/s0034-5288(18)30482-x
Hawbaker J. A., Speer V. C., Hayes V. W., Catron D. V. (1961). Effects of copper sulfate and other chemoprophylactics in growing swine rations. J. Anim. Sci. 20, 163–167.
Kim G. B., Seo Y. M., Shin K., Rhee A. R., Han K., Paik I. K. (2011). Effects of supplemental copper-methionine chelate and copper-soy proteinate on the performance, blood parameters, liver mineral content, and intestinal microflora of broiler chickens. J. Appl. Poult. Res. 20, 21–32. doi:10.3382/japr.2010-00177
Koh T. S., Peng R. K., Klasing K. C. (1996). Dietary copper level affects copper metabolism during lipopolysaccharide-induced immunological stress in chicks. Poult. Sci. 75 (7), 867–872. doi:10.3382/ps.0750867
Lauridsen C. (2019). From oxidative stress to inflammation: Redox balance and immune system. Poult. Sci. 98 (10), 4240–4246. doi:10.3382/ps/pey407
Li S. C., Hsu W. F., Chang J. S., Shih C. K. (2019). Combination of Lactobacillus acidophilus and Bifidobacterium animalis subsp. lactis shows a stronger anti-Inflammatory effect than individual strains in HT-29 Cells. Nutrients 11 (5), 969. doi:10.3390/nu11050969
Mei S. F., Yu Y., Ju C. F., Zhu D., Che D. W. (2010). Effect of different levels of copper on growth performance and cecal ecosystem of newly weaned piglets. Ital. J. Anim. Sci. 9, e71. doi:10.4081/ijas.2010.e71
Montagne L., Boudry G., Favier C., Le Huërou-Luron I., Lallès J. P., Sève B. (2007). Main intestinal markers associated with the changes in gut architecture and function in piglets after weaning. Br. J. Nutr. 97 (1), 45–57. doi:10.1017/S000711450720580X
Müller L., Kuhn T., Koch M., Fuhrmann G. (2021). Stimulation of probiotic bacteria induces release of membrane vesicles with augmented anti-inflammatory activity. ACS Appl. Bio. Mater. 4 (5), 3739–3748. doi:10.1021/acsabm.0c01136
Nguyen H. T. T., Kheravii S. K., Wu S. B., Roberts J. R., Swick R. A., Toghyani M. (2022). Sources and levels of copper affect liver copper profile, intestinal morphology and cecal microbiota population of broiler chickens fed wheat-soybean meal diets. Sci. Rep. 12 (1), 2249. doi:10.1038/s41598-022-06204-9
Oh N. S., Joung J. Y., Lee J. Y., Kim Y. (2018). Probiotic and anti-inflammatory potential of Lactobacillus rhamnosus 4B15 and Lactobacillus gasseri 4M13 isolated from infant feces. PLoS One 13 (2), e0192021. doi:10.1371/journal.pone.0192021
Onrust L., Ducatelle R., Van Driessche K., De Maesschalck C., Vermeulen K., Haesebrouck F., et al. (2015). Steering endogenous butyrate production in the intestinal tract of broilers as a tool to improve gut health. Front. Vet. Sci. 2, 75. doi:10.3389/fvets.2015.00075
Pang Y., Patterson J. A., Applegate T. J. (2009). The influence of copper concentration and source on ileal microbiota. Poult. Sci. 88 (3), 586–592. doi:10.3382/ps.2008-00243
Percival S. S. (1998). Copper and immunity. Am. J. Clin. Nutr. 67 (5), 1064S–1068S. doi:10.1093/ajcn/67.5.1064S
Richards J. D., Zhao J., Harrell R. J., Atwell C. A., Dibner J. J. (2010). Trace mineral nutrition in poultry and swine. Asian-Aust. J. Anim. Sci. 23 (11), 1527–1534. doi:10.5713/ajas.2010.r.07
Richards M. P., Augustine P. C. (1988). Serum and liver zinc, copper, and iron in chicks infected with Eimeria acervulina or Eimeria tenella. Biol. Trace Elem. Res. 17, 207–219. doi:10.1007/BF02795457
Rochell S. J., Usry J. L., Parr T. M., Parsons C. M., Dilger R. N. (2017). Effects of dietary copper and amino acid density on growth performance, apparent metabolizable energy, and nutrient digestibility in Eimeria acervulina-challenged broilers. Poult. Sci. 96 (3), 602–610. doi:10.3382/ps/pew276
Song B., Tang D., Yan S., Fan H., Li G., Shahid M. S., et al. (2021). Effects of age on immune function in broiler chickens. J. Anim. Sci. Biotechnol. 12 (1), 42. doi:10.1186/s40104-021-00559-1
Talib N., Mohamad N. E., Yeap S. K., Hussin Y., Aziz M. N. M., Masarudin M. J., et al. (2019). Isolation and characterization of Lactobacillus spp. from kefir samples in Malaysia. Molecules 24 (14), 2606. doi:10.3390/molecules24142606
Thomson A. B., Keelan M. (1986). The development of the small intestine. Can. J. Physiol. Pharmacol. 164 (1), 13–29. doi:10.1139/y86-003
Vallet S. D., Ricard-Blum S. (2019). Lysyl oxidases: From enzyme activity to extracellular matrix cross-links. Essays Biochem. 63 (3), 349–364. doi:10.1042/EBC20180050
Van den Abbeele P., Belzer C., Goossens M., Kleerebezem M., De Vos W. M., Thas O., et al. (2013). Butyrate-producing Clostridium cluster XIVa species specifically colonize mucins in an in vitro gut model. ISME J. 7 (5), 949–961. doi:10.1038/ismej.2012.158
Wang Z., Cerrate S., Coto C., Yan F., Waldroup P. W. (2007). Evaluation of Mintrex® copper as a source of copper in broiler diets. Int. J. Poult. Sci. 6 (5), 308–313. doi:10.3923/ijps.2007.308.313
Wu Z., Pan D. D., Guo Y., Zeng X. (2013). Structure and anti-inflammatory capacity of peptidoglycan from Lactobacillus acidophilus in RAW-264.7 cells. Carbohydr. Polym. 96 (2), 466–473. doi:10.1016/j.carbpol.2013.04.028
Xia M. S., Hu C. H., Xu Z. R. (2004). Effects of copper-bearing montmorillonite on growth performance, digestive enzyme activities, and intestinal microflora and morphology of male broilers. Poult. Sci. 83 (11), 1868–1875. doi:10.1093/ps/83.11.1868
Yang F., Pei R., Zhang Z., Liao J., Yu W., Qiao N., et al. (2019). Copper induces oxidative stress and apoptosis through mitochondria-mediated pathway in chicken hepatocytes. Toxicol Vitro 54, 310–316. doi:10.1016/j.tiv.2018.10.017
Yazdankhah S., Rudi K., Bernhoft A. (2014). Zinc and copper in animal feed - development of resistance and co-resistance to antimicrobial agents in bacteria of animal origin. Microb. Ecol. Health Dis. 25, 25862. doi:10.3402/mehd.v25.25862
Yu Z., Gunn L., Wall P., Fanning S. (2017). Antimicrobial resistance and its association with tolerance to heavy metals in agriculture production. Food Microbiol. 64, 23–32. doi:10.1016/j.fm.2016.12.009
Zaghari M., Pouraghaali S., Zhandi M., Abbasi M. (2022). Effect of monovalent copper oxide and potentiated zinc oxide on growth performance and gut morphology of broiler chickens challenged with coccidiosis. Biol. Trace Elem. Res. doi:10.1007/s12011-022-03339-8
Keywords: chelated copper, CuSO4, TBCC, gut microbiota, gut morphometry, broiler
Citation: Chen J, Yan F, Kuttappan VA, Wedekind K, Vázquez-Añón M and Hancock D (2023) Effects of bis-chelated copper in growth performance and gut health in broiler chickens subject to coccidiosis vaccination or coccidia challenge. Front. Physiol. 13:991318. doi: 10.3389/fphys.2022.991318
Received: 11 July 2022; Accepted: 28 December 2022;
Published: 03 February 2023.
Edited by:
Federico Sirri, University of Bologna, ItalyReviewed by:
Kokou Tona, University of Lomé, TogoCopyright © 2023 Chen, Yan, Kuttappan, Wedekind, Vázquez-Añón and Hancock. This is an open-access article distributed under the terms of the Creative Commons Attribution License (CC BY). The use, distribution or reproduction in other forums is permitted, provided the original author(s) and the copyright owner(s) are credited and that the original publication in this journal is cited, in accordance with accepted academic practice. No use, distribution or reproduction is permitted which does not comply with these terms.
*Correspondence: Juxing Chen, anV4aW5nLmNoZW5Abm92dXNpbnQuY29t
Disclaimer: All claims expressed in this article are solely those of the authors and do not necessarily represent those of their affiliated organizations, or those of the publisher, the editors and the reviewers. Any product that may be evaluated in this article or claim that may be made by its manufacturer is not guaranteed or endorsed by the publisher.
Research integrity at Frontiers
Learn more about the work of our research integrity team to safeguard the quality of each article we publish.