- 1Hubei Engineering Technology Center for Pest Forewarning and Management, Institute of Insect Sciences, Yangtze University, Jingzhou, Hubei, China
- 2Institute of Agricultural Quality Standards and Testing Technology Research, Hubei Academy of Agricultural Sciences/Hubei Key Laboratory of Nutritional Quality and Safety of Agro Products, Wuhan, Hubei, China
- 3Department of Plant Protection, Institute of Vegetables and Flowers, Chinese Academy of Agricultural Sciences, Beijing, China
Bradysia odoriphaga (Diptera: Sciaridae) is a serious pest of Chinese chives cultivated in China. Chemosensory proteins (CSPs) are important components of insect olfactory systems that capture and bind environmental semiochemicals which are then transported to olfactory receptors. Despite their importance, the mechanism of olfaction and related behavioral processes in B. odoriphaga have not been characterized. Here, we found that BodoCSP4 has an important olfactory function. RT-qPCR indicated that BodoCSP4 expression was highest in the heads (antennae removed) of adult males, followed by the antennae of adult males. Competitive binding assays with 33 ligands indicated that BodoCSP4 binds well with methyl allyl disulfide, diallyl disulfide, and n-heptadecane; the corresponding dissolution constants (Ki) were as high as 5.71, 5.71, and 6.85 μM, respectively. 3D-structural and molecular docking indicated that BodoCSP4 has five α-helices and surrounds the ligand with certain hydrophobic residues including Leu60, Leu63, Leu64, Ala67, Val28, Ile30, Ile33, Leu34, and Val86, suggesting these residues help BodoCSP4 bind to ligands. Silencing of BodoCSP4 significantly decreased the attraction of B. odoriphaga males to diallyl disulfide and n-heptadecane but not to methyl allyl disulfide in Y-tube olfaction assays. These results increase our understanding of how BodoCSP4 contributes to host and female localization by B. odoriphaga males.
Introduction
Insects depend on their olfactory systems to perceive chemical signals including host plant volatiles, sex pheromones, and alarm pheromones (Visser, 1986; Hansson, 2002; French et al., 2015). In insect olfactory systems, chemosensory proteins (CSPs) participate in the transmission of semiochemicals through the sensillar lymph fluid to the olfactory receptors (ORs) (Pelosi et al., 2005; Oliveira et al., 2018), and then transduce electrical signals in order to activate physiological functions and regulate insect behavioral responses (Pelosi et al., 2006; Antony et al., 2016). Therefore, insect CSPs have an indispensable role in the recognition of semiochemicals and help ensure that insects make timely and effective behavioral responses to such chemical signals (Pelosi et al., 2018).
Many studies have shown that insect CSPs are found in multiple tissues, suggesting that CSPs have additionally functions to chemosensation (Picimbon, 2003). In addition to being involved in host volatile recognition (Younas et al., 2022), CSPs are also involved in development (Maleszka et al., 2007), reproduction (Cheng et al., 2015; Zeng et al., 2020), circadian cycle regulation (Claridge-Chang et al., 2001), leg regeneration (Kitabayashi et al., 1998), insecticide resistance (Xu et al., 2022), vision (Zhu et al., 2016), and physiological shifts such as transition from the solitary to gregarious phase of Locusta migratoria (Chen et al., 2010; Guo et al., 2011). Therefore, studying how insect CSPs function will help to enrich our understanding of the potential roles of CSPs, and advance the development of comprehensive control methods using insect CSPs as molecular targets.
The Gnat Bradysia odoriphaga is a pest of both vegetables and mushrooms and is especially damaging to Chinese chives cultivated in Asian countries (Feng and Zheng, 1987). Feeding by B. odoriphaga larvae reduces the growth, development, and edibility of Chinese chives (Li et al., 2014). This pest is difficult to control because of its high fecundity and the soil-borne habitat of the larvae (Dang et al., 2001; Ma et al., 2013). At present, B. odoriphaga is mainly controlled via the application of insecticides, but overuse of chemical pesticides results in serious problems including insecticide resistance, environmental pollution, pesticide residues on host plants, and negative effects on human health (Yang et al., 2014). As a result, safe and effective non-chemical means to control B. odoriphaga are greatly needed.
Previous studies have shown that B. odoriphaga uses host plant volatiles to find hosts, mates, and oviposition sites (Yang et al., 2019). Li H. J. et al. (2007) found that B. odoriphaga females release n-heptadecane to attract males. Because the larvae have limited dispersal abilities, they rely on the adults to select suitable host plants for larval feeding, growth, and development. Although olfactory proteins are clearly important for regulating the behaviors of B. odoriphaga adults, most research concerning the functions of such proteins has focused on odorant binding proteins (BodoOBPs) (Tang et al., 2019; Yang et al., 2021a; Yang et al., 2021b) rather than on BodoCSPs.
To date, five BodoCSP genes have been identified from the B. odoriphaga antennae transcriptome (Zhao et al., 2018), but only the function of BodoCSP1 has been studied (Zhang et al., 2021). As determined by the latter authors, BodoCSP1 is highly expressed in antennae and is involved in the perception of host plant volatiles. The present work we conducted an extensive study to explore the potential functions of the CSP gene, BodoCSP4. We found that the BodoCSP4 expression levels were highest in the heads (without antennae) of males, followed by the antennae of males. To determine the specific physiological functions of BodoCSP4, we characterized its binding affinity with Chinese chive volatiles, modeled its protein structure, and investigated its potential binding sites. We also used a Y-tube olfaction assay to measure the behavioral responses of BodoCSP4-silenced B. odoriphaga adult males to host plant volatiles. These results increase our understanding of chemo-sensation by B. odoriphaga and could identify new molecular targets for controlling this important pest.
Materials and methods
Insect rearing
A population of B. odoriphaga was obtained from a Chinese chive field in the ShunYi District of Beijing, in 2019. The population has been maintained and fed on fresh Chinese chives (not treated with insecticides) in a climate-controlled chamber at 25–28°C, 70–80% relative humidity (RH), and a 16:8 h light/dark photoperiod.
RNA extraction and cDNA synthesis
Total RNA was extracted from eggs (n = 100), larvae (n = 10), pupae (n = 10), and adults (females and males that were <2 days old; n = 10 for each sex), and also from the antennae (n = 500), male heads (without antennae; n = 500), abdomens (n = 10), and carcasses (thoraxes, wings, and legs mixed; n = 10) of males and females. Extraction was performed with the Trizol kit (Invitrogen, CA, United States) according to the manufacturer’s instructions. The RNA samples were quantified using a Nanodrop ND-2000 spectrophotometer (Nanodrop, Wilmington, DE, United States), and the integrity was confirmed by 2% agarose gel electrophoresis.
Tested ligands
N-phenyl-1-naphthylamine (1-NPN) was used as the competitive fluorescent reporter. The following volatiles were used in binding assays with BodoCSP4: alkanes (nonane, dodecane, tetradecane, hexadecane, n-heptadecane); terpenes (ocimene, ß-pinene, ß-caryophyllene, (R)-(+)-limonene, a-humulene); alcohols ((Z)-3-hexen-1-ol, 1,8-cineole, citronellol, linalool); esters (butyl levulinate, methyl phenylacetate, butyl acrylate); ketones (2-hexanone, beta-Ionone); aldehydes (decanal, valeric aldehyde, octanal, benzaldehyde, heptanal, nonanal); sulfur compounds (diallyl disulfide, methyl allyl disulfide) and others (acetophenone, carvaceol, h-11 indole). All of these compounds and 1-NPN were purchased from Sigma-Aldrich (St. Louis, MO, United States) and had 98% purity. Other background information on the ligands is provided in Supplementary Table S1.
Identification, sequence analysis, and phylogenetic tree construction of BodoCSP4
Open reading frames (ORFs), the conserved domains, and N-terminal signal peptides of B. odoriphaga RNA were predicted using ORF Finder (http://www.ncbi.nlm.nih.gov/gorf/gorf.html), SMART software (http://smart.emblheidelberg.de/) (Letunic et al., 2015), and Signalp V5.1 (http://www.cbs.dtu.dk/services/SignalP/) (Jannick et al., 2004). The ExPASy Proteomics Server (http://cn.expasy.org/tools/pi_tool.html) was used to compute the isoelectric points and molecular weights of the deduced protein sequences. Primers used to validate the BodoCSP4 sequences are listed in Table 1. Purified PCR products were cloned into the pEASY-T1 vector (TransGen, China) and sequenced.
We used phylogenetic analysis to compare the resulting sequences of the putative CSP4 from B. odoriphaga with published orthologous CSP sequences (minus the signal peptides) from three other dipterans (Drosophila melanogaster, Culex quinquefasciatus, and Anopheles gambiae), two hemipterans (Adelphocoris lineolatus, Aphis gossypii), one hymenopteran (Apis mellifera), and one lepidopteran (Bombyx mori). Based on the predicted amino acid sequences, we used the Multhithreaded Maxmimum Likelihood method in MEGA6.0 to construct a phylogenetic tree for each gene; this was done with 1,000 bootstrap replications (Tamura et al., 2013) and with Poisson correction of distances.
Expression of the BodoCSP4 gene
Reverse transcription quantitative PCR (RT-qPCR) was used to quantify BodoCSP4 expression in different developmental stages of B. odoriphaga (egg, larva, pupa, and adult) and in different tissues of B. odoriphaga adults including male heads without antennae, female antennae, male antennae, abdomens, and carcasses (thoraxes, wings, and legs mixed). RNA was extracted according to reagent protocols. Primers designed based on cDNA sequences were used for RT-qPCR (Table 1). RPL18 and RPS15 were used as reference genes for relative expression analysis of BodoCSP4 at different life stages, and EF1 and ACT were used as reference genes for expression analysis in different tissues (Shi et al., 2016).
Recombinant protein expression and purification of BodoCSP4 protein
The BodoCSP4 cDNA was PCR amplified with specific primers (Table 1). The PCR products were ligated into the expression vector pBM30 according to the manufacturer’s instructions (Biomed, Beijing, China). The ligation products containing the pBM30/BodoCSP4 sequence were used to transform Escherichia coli BL21 (DE3) cells (TransGen Biotech) for protein expression and sequencing. Positive colonies were used for expression and purification of the recombinant BodoCSP4 protein. The results showed that, after sonication and centrifugation, the BodoCSP4 proteins were mainly expressed in insoluble bodies. Protein refolding was performed according to redox methods (Prestwich, 1993). In brief, 50 mM Tris buffer (pH 6.8) containing 0.2% Triton X-100 was used to wash the insoluble inclusion body, which was then dissolved in 6 M guanidine hydrochloride. The refolded protein was then collected and purified by Ni2+ ion affinity chromatography (GE-Healthcare, United States). The His-tag was removed by recombinant enterokinase (rEK) (Novagen, Beijing, China). The size and the concentration of BodoCSP4 were determined using SDS-PAGE and the BCA protein assay kit (CoWinbiotech, Beijing, China), respectively.
Fluorescence binding assays
An F-380 fluorescence spectrophotometer (Tianjin, China) was used to measure the binding affinity of BodoCSP4 to the selected volatiles, using 1-N-phenyl-naphthylamine (1-NPN) as the fluorescent probe. 1-NPN and 33 ligands were selected for this assay based on previous studies (Li H. L. et al., 2007; Zhang et al., 2016; Yang et al., 2019) (Supplementary Table S1). Both 1-NPN and the volatile compounds were diluted in chromatographic-grade methanol to make 1 mM stock solutions. Recombinant BodoCSP4 was dissolved in 20 mM Tris-HCl (pH 7.4) and diluted to a 2 µM stock solution. The dissociation constants (Kd) between 1-NPN and the recombinant BodoCSP4 were calculated by Scatchard analysis and the ligand binding affinity (Ki) was calculated with the following equation: Ki = [IC50]/(1 + [1-NPN]/K1- NPN), in which IC50 is the maximum concentration at which the ligand replaces 50% of the fluorescence value of 1-NPN [1-NPN] is the free concentration of 1-NPN; and K1-NPN is the dissociation constant of 1-NPN (Campanacci et al., 2003; Wei et al., 2008).
3D structure and molecular docking of BodoCSP4
The 3D structure of BodoCSP4 was obtained with a template of CSPMbraA6 (1N8V) and Swiss-Model software. The binding cavity of BodoCSP4 was predicted using SYBYL 7.3 software. Based on the results of the fluorescence binding assays, the ligands diallyl disulfide, methyl allyl disulfide, and n-heptadecane were selected to construct the molecular conformations by Sketch mode and were optimized using the Tripos force field and Gasteiger-Hückel charge. In addition, Surflex-Dock (SYBYL 7.3) was applied to the molecular docking modeling between the BodoCSP4 protein and three ligands.
dsRNA synthesis and Y-tube olfactometer assays
The primer pairs dsCSP4 and dsGFP, containing the T7 promoter sequence, are listed in Table 1, and the T7 RiboMAX ™ Express RNAi System was used to synthesize double stranded RNAs (dsRNAs). The dsRNAs were microinjected into 3-day-old pupae of B. odoriphaga, and newly eclosed male adults were selected for dsRNA extraction and behavioral assays as previously described (Yang et al., 2021b; Zhang et al., 2021). According to the fluorescence binding results, after BodoCSP4 was silenced, a Y-tube olfactometer was used to assess the behavioral responses of B. odoriphaga male adults to the ligands diallyl disulfide, methyl allyl disulfide, and n-heptadecane as described in our previous report (Yang et al., 2019). In brief, a 10-μL solution of diallyl disulfide, methyl allyl disulfide, or n-heptadecane in hexane was placed on a strip (4 × 40 mm) of filter paper; after 10 s, the strip was placed at the end of one of the two Y-tube arms. As a control, a hexane-treated filter paper strip was placed at the end of the other arm. Two streams of clean air were passed through the Y-tube arms to the base, and one adult male that had been previously injected (at the pupal stage, 12 h before emergence) with dsBodoCSP4 or dsGFP was placed in the base of the Y-tube. If the male adult moved at least half way up one of the Y-tube arms, the result was recorded and considered an indication of attraction or repulsion depending on which arm was selected. Each of six treatment combinations (3 host plant volatiles with controls and dsBodoCSP4 or dsGFP microinjected adults) are represented by 60 replicate assays.
Statistical analysis
All RT-qPCR experiments were analyzed using one-way ANOVA followed by Tukey-HSD tests with SPSS 20.0 software. All RT-qPCR experiments were conducted using three independent biological replicates. In each of the six Y-tube olfactometer assays (3 ligands and controls without ligand × two types of microinjected B. odoriphaga adult males), behavioral responses to filter paper with or without ligand were analyzed by a chi-square test (χ 2).
Results
Identification and phylogenetic analysis of BodoCSP4 in B. odoriphaga
We identified a CSP gene named BodoCSP4 (Accession number: MG544173) in a previously published B. odoriphaga transcriptome dataset, and we verified its identity by RT-PCR (Table 2; Fig. S1A). The full-length BodoCSP4 sequence contains an ORF of 357 bp. The predicted amino acid sequence has the typical four-cysteine signature (Wanner et al., 2004) with a motif of C1-X6-8-C2-X16-21-C3-X2-C4 (Supplementary Figure S1B) and an 18 amino acid-signal peptide in the N terminus (Supplementary Figure S1B). BodoCSP4 has a molecular weight of 13.58 kDa and an isoelectric point of 9.42 (Table 2). In a phylogenetic tree of BodoCSP4 and other insect CSPs, BodoCSP4 was clustered with the CSPs of the dipterans D. melanogaster and A. gambiae (Supplementary Figure S2).
Expression profile analysis of BodoCSP4
Among developmental stages, the expression level of BodoCSP4 was lowest in eggs and highest in adult males (F = 11.711, df = 4,14, p = 0.001) (Figure 1A). Regarding tissue-specific expression analysis in adults, expression levels of BodoCSP4 were highest in adult male heads (antennae removed), followed by adult male antennae (F = 14.68, df = 4,14, p = 0.000) (Figure 1B).
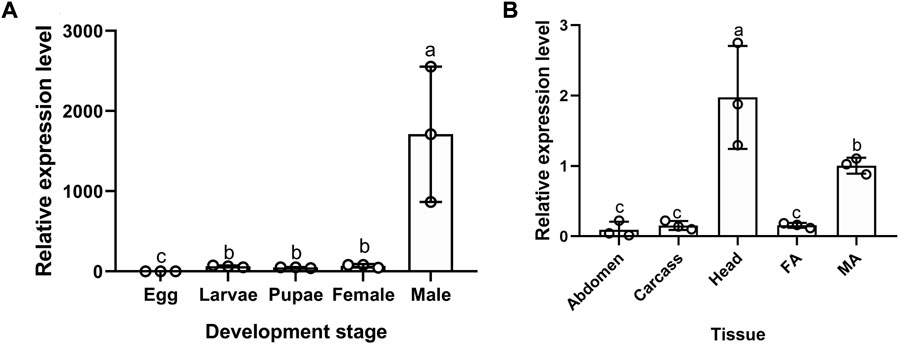
FIGURE 1. Gene expression profiling of CSP4 in different developmental stages (A) and tissues (B) of B. odoriphaga as determined by RT-qPCR. Egg; Larvae; Pupae; Female; Male. Fa: Female antenna; Ma: Male antenna; Male head (without antennae); Carcass: leg + wing + thorax; Abdomen. The expression levels were estimated using the 2−ΔΔCt method. The expression level in eggs was used as a standard to compare expression levels among developmental stages, and the expression level in male antennae was used as a standard to compare expression levels among tissues. Values are means ± SE; means with different letters are significantly different (p < 0.05).
Recombinant protein expression and purification of BodoCSP4
The recombinant BodoCSP4 protein was successfully expressed and purified in Escherichia coli, yielding a high concentration (up to 0.68 mg/ml). BodoCSP4 was mainly expressed in insoluble bodies and its molecular weight is < 15 kDa, which was consistent with the predicted results (Supplementary Figure S3).
Fluorescence binding assays with BodoCSP4
To determine the function of the recombinant protein, we measured the ligand-binding affinity of recombinant CSP4 in a competitive fluorescence binding assay with 33 ligands (Figure 2 and Supplementary Table S2). The dissociation constant (Kd) of BodoCSP4/1-NPN was 1.076 µM (Figure 2A). The binding affinity results showed that BodoCSP4 specifically bound to 3 of the 33 ligands: diallyl disulfide (5.71 µM), methyl allyl disulfide (5.71 µM), and n-heptadecane (6.85 µM) (Figure 2B). The binding affinities between BodoCSP4 and the other ligands are listed in Supplementary Table S2.
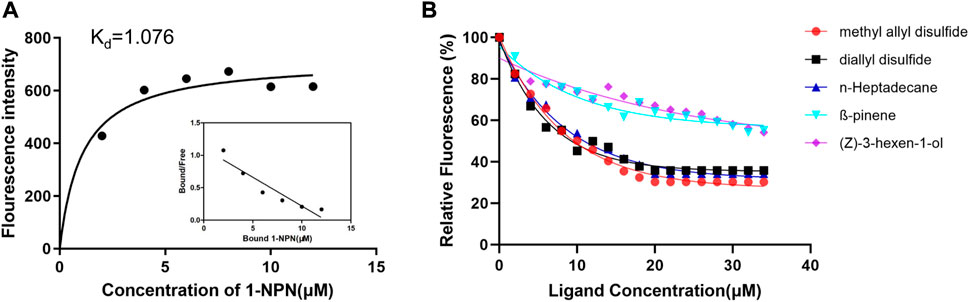
FIGURE 2. Binding curves for 1-NPN and Scatchard plots of recombinant BodoCSP4 (A) and fluorescence competitive binding curves of the recombinant protein BodoCSP4 with five ligands (B).
3D model structuring and molecular docking of BodoCSP4
Based on the high alignment and high sequence identity (42%) between BodoCSP4 and the template protein CSPMbraA6 (Figure 3A), BodoCSP4 has five α-helices located between residues Asn29-Asn36 (α1), Asp37-Lys50 (α2), Thr54-Thr70 (α3), Cys75-Arg93 (α4), and Arg94-Asp106 (α5) (Figure 3B).
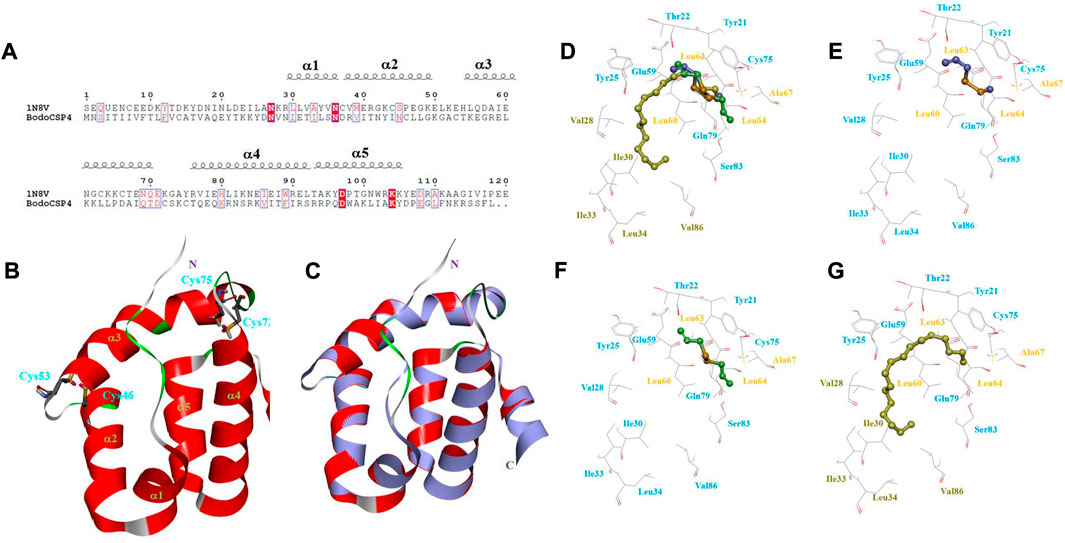
FIGURE 3. 3D structure model and binding cavity of Bradysia odoriphaga chemosensory protein 4 (BodoCSP4). (A) Sequence alignment of BodoCSP4 and CSPMbraA6 (1N8V). The black spiral coils represent α-helices. (B) 3D model of the target protein BodoCSP4 based on the crystal structure of the template protein of CSPMbraA6; two disulfide bridges are labeled in blue, and the five α-helices are marked in green. (C) Alignment of the target protein BodoCSP4 (red) and the template protein CSPMbraA6 (purple, 1N8V). 3D models of interactions of the recombinant protein BodoCSP4 with (D) three ligands (diallyl disulfide, methyl allyl disulfide, and n-heptadecane), (E) diallyl disulfide, (F) methyl allyl disulfide, and (G) and n-heptadecane. In D-G, the yellow residues present hydrophobic residues, the blue residues represent hydrophilic residues, and the brown residues represent the hydrophobic residues of the long chain of n-heptadecane.
To better understand the potential key amino acid residues involved in the interaction between the BodoCSP4 protein and a ligand, we examined BodoCSP4 and ligand complexes by molecular docking (Figure 3). Some hydrophobic residues of BodoCSP4 surround the ligands (diallyl disulfide, methyl allyl disulfide, and n-heptadecane), including Leu60, Leu63, Leu64, and Ala67 (Figure 3C). Because diallyl disulfide, methyl allyl disulfide, and n-heptadecane have similar structures and conformations (diallyl disulfide and methyl allyl disulfide in particular have the same disulfide bond group), they have almost the same BodoCSP4 binding site (Figures 3D, E). Unlike diallyl disulfide and methyl allyl disulfide, however, n-heptadecane occupied a narrow and hydrophobic tunnel of BodoCSP4 owing to the ligand’s long hydrophobic tail; the hydrophobic tunnel included residues Val28, Ile30, Ile33, Leu34, Val86, among others (Figure 3F). The following hydrophobic residues of BodoCSP4 surround the three ligands and are likely binding sites: Leu60, Leu63, Leu64, Ala67, Val28, Ile30, Ile33, Leu34, and Val86.
Behavioral effect of BodoCSP4 knockdown by RNAi
To further determine whether BodoCSP4 is important for recognizing the above three ligands, we silenced the BodoCSP4 gene and measured the attraction of dsBodoCSP4- and dsGFP-treated adult males to each ligand or to a control without ligand in Y-tube olfaction assays. Treatment of adult males with dsBodoCSP4, but not with dsGFP, significantly reduced BodoCSP4 expression (F = 7.952,df = 2,11,p = 0.01) (Figure 4). The dsGFP-treated adults were attracted to methyl allyl disulfide (Figure 5A; χ2 = 5.952, df = 1, p = 0.025), diallyl disulfide (Figure 5B; χ2 = 4.849, df = 1, p = 0.028), and n-heptadecane (Figure 5C; χ2 = 4.604, df = 1, p = 0.032). The dsBodoCSP4-treated adults were attracted to methyl allyl disulfide (Figure 5A; χ2 = 3.998, df = 1, p = 0.046), however no olfactory attraction to diallyl disulfide (Figure 5B; χ2 = 0.08, df = 1, p = 0.777) and n-heptadecane (Figure 5C; χ2 = 0.501, df = 1, p = 0.479) was observed. These results suggest that BodoCSP4 is important for the behavioral response of B. odoriphaga adults to diallyl disulfide and n-heptadecane but not to methyl allyl disulfide.
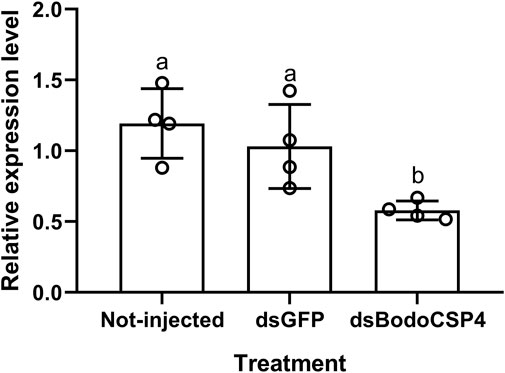
FIGURE 4. Effects of treatment of Bradysia odoriphaga male adults with dsGFP or dsBodoCSP4 on their mRNA levels of BodoCSP4 (as determined by RT-qPCR). The values are means ± SEM; means with different letters are significantly different (p < 0.05).

FIGURE 5. Behavioral responses of Bradysia odoriphaga male adults previously injected with dsRNAi to (A) diallyl disulfide (or a control), (B) methyl allyl disulfide (or a control), or (C) and n-heptadecane (or a control) in Y-tube olfactometer assays. In (A–C), one arm of the olfactometer contained the indicated ligand in solvent (hexane), and the other arm contained the solvent alone (control). For each assay, a treated male adult was placed at the base of the olfactometer, and its movement into one of the two arms was recorded; an asterisk indicates that significantly more adults responded to the ligand than to the control in the indicated assay. For each combination of ligand/control and dsGFP-treated or dsBodoCSP4-treated adult in (A–C), n = 60. Asterisks indicate statistically significant differences between the behavioral response to the ligand vs. the control as determined by chi-square tests: *p < 0.05, **p < 0.01.
Discussion
Like odorant-binding proteins (OBPs), CSPs are important components of insect olfactory systems, and are required by insects to search for and detect host plants, suitable oviposition sites, and mates (Vogt et al., 1985; Field et al., 2000; Sun et al., 2014). Previous research clarified the physiology and function of BodoCSP1, but the physiology and function of other CSPs in B. odoriphaga remained unknown prior to the current study.
That CSPs are widely distributed on insect chemosensory and non-chemosensory organs suggests that they have diverse functions (Jacquin-Joly et al., 2001). The current study with B. odoriphaga revealed that BodoCSP4 is mainly expressed in the heads (without antennae) of adult males, followed by the antennae of adult males. The broad expression profile of BodoCSP4 among B. odoriphaga tissues indicates that CSP4 may have other functions besides olfaction in this insect. Previous studies showed that OBP genes were highly expressed in insect heads, suggesting that they contribute to the detection of host plant volatiles and sex pheromones (Chang et al., 2015; Liu et al., 2015; Wang et al., 2016). The following studies also showed that the indicated CSPs are highly expressed in the male antennae of the following insects: CSP11 of Plutella xylostella (Fu et al., 2020), CSP8 of Nilaparvata lugens (Waris et al., 2018), CSP1 of Cylas formicarius (Hua et al., 2021), and CSP3 of Cnaphalocrocis medinalis (Zeng et al., 2018). High expression of CSP in male antennae suggests that they may be more involved in the perception of sex pheromones than plant volatiles. Based on the current results with B. odoriphaga, we speculate that BodoCSP4 is involved not only in the searching and localization of host plants by males, but also in finding females.
To explore the role of BodoCSP4, we determined the binding affinity of BodoCSP4 to 33 ligands. The results indicated that BodoCSP4 displayed high binding affinities (Ki < 10 μM) to two sulfur-containing volatiles, diallyl disulfide and methyl allyl disulfide, and to n-heptadecane. Liu et al. (2020) found that sulfur-containing secondary isothiocyanates (ITCs) affected the preference of P. xylostella for Arabidopsis thaliana; the latter study also reported that knockdown of PxylOr35 or PxylOr49 reduced the effect of ITCs on P. xylostella selection of oviposition sites, and that knockdown of both genes reduced the attraction of P. xylostella to ITCs. In addition, researchers recently reported that ITCs are not only chemical signals used by adult Scaptomyza flava to find host plants, but are also involved in the evolution of the olfactory receptor SflaOr67bs (Matsunaga et al., 2022). Our previous results showed that the sulfur-containing volatiles from Chinese chives, diallyl disulfide and methyl allyl disulfide, elicit strong electrophysiological responses from the antennae of both male and female B. odoriphaga (Yang et al., 2019). In the current study, however, the expression levels of BodoCSP4 were highest in male heads followed by male antennae. We therefore hypothesized that diallyl disulfide and methyl allyl disulfide are chemical cues that are specifically recognized by the olfactory gene BodoCSP4 and may help male B. odoriphaga adults locate Chinese chives. Interestingly, the current results indicated that BodoCSP4 also displayed a high binding affinity with n-heptadecane, which was obtained as an extract from the abdomen of B. odoriphaga females and elicits a behavioral response in male adults (Li H. J. et al., 2007). This finding is similar to that for CSPA6 of Mamestra brassicae (Jacquin-Joly et al., 2001) and SinfCSP19 of Sesamia inferen (Zhang et al., 2014), suggesting that BodoCSP4 may be involved in the recognition of sex pheromones by B. odoriphaga.
Homology modeling and molecular docking have been used to explore specific ligand-binding features (Liu et al., 2019). In the previous study, the modeled BodoCSP4 only had five α-helixes, which is similar to SfurCSP5 (Chen et al., 2018). BodoCSP4, however, differs from other insect CSPs, which contain six α-helixes (Campanacci et al., 2003; Tomaselli et al., 2006; Jean-François, 2014); as a result, it will be necessary to confirm or modify the actual 3D structure of BodoCSP4 in future studies. Kulmuni and Havukainen (2013) found that insect CSPs with five α-helixes are mostly involved in searching for mates. We therefore suggest that BodoCSP4 may be involved in finding females and in other olfactory functions.
Binding models in the current study showed that BodoCSP4 bound to the ligands of methyl allyl disulfide, diallyl disulfide, and n-heptadecane near many BodoCSP4 hydrophobic residues (including Leu60, Leu63, Leu64, and Ala67, Val28, Ile30, Ile33, Leu34, and Val86) but not the most polar hydrophobic residues (Figure 3). Mármol et al. (2021) reported that interactions with hydrophobic residues contribute to odor molecule binding and thereby mediate ligand recognition. In addition, previous studies have reported that leucine is the key amino acid for ligand binding, as was observed for Mamestra brassicae CSP6 (Campanacci et al., 2003), SfurCSP5 (Chen et al., 2018), and BminCSP3 (Cui et al., 2022). The hydrophobic residues of BodoCSP4 interacted with all three ligands, indicating their key involvement in ligand binding of BodoCSP4. This feature is consistent with other OBPs and CSPs (Tomaselli et al., 2006; Zheng et al., 2015; Northey et al., 2016). However, molecular docking can only assist in predicting binding mechanisms, and as such we will use site-directed mutagenesis to further investigate the specific functions of these hydrophobic amino acids. Based on both the homology modeling, molecular docking, and competitive binding results, we conclude that CSP4 can bind to both sulfur volatiles and sex pheromones, and that CSP4 therefore participates in multiple physiological functions in B. odoriphaga.
In the present study we used RNA interference and behavioral assays to further confirm the physiological functions of BodoCSP4 in B. odoriphaga. The results indicated that silencing of the BodoCSP4 gene significantly decreased the behavioral response of B. odoriphaga male adults to diallyl disulfide and n-heptadecane but did not decrease the behavioral response to methyl allyl disulfide. In contrast, Zhang et al. (2021) reported that silencing of BodoCSP1 reduced the behavioral response of B. odoriphaga male adults to diallyl disulfide and methyl allyl disulfide. In addition, previous reports indicated that BodoOBP1, BodoOBP2 (Tang et al., 2019), BodoOBP5 (Yang et al., 2021a), and BodoOBP8 (Yang et al., 2021b) bind well to diallyl disulfide and methyl allyl disulfide. We suggest that BodoCSP4, however, may work together with other OBPs or CSPs to recognize methyl allyl disulfide methyl allyl disulfide. For example, Sun et al. (2016) found that CmedOBP2 and CmedOBP3 co-regulate odorant recognition by C. medinalis adults, and Maguire et al. (2022) found that AgamOR2 and ORCO can together alter the olfactory preferences of Anopheles mosquitoes. Based on the current results, we speculate that diallyl disulfide and n-heptadecane but not methyl allyl disulfide can be specifically recognized by BodoCSP4, and that diallyl disulfide and n-heptadecane are the main chemical signals perceived by BodoCSP4 in host plant recognition and localization by males, as well as female localization.
Overall, the results of this study suggest that BodoCSP4 may be involved in the recognition and localization of host plants and mates. Determining whether diallyl disulfide and n-heptadecane function as attractants to regulate B. odoriphaga behavior will require additional research.
Data availability statement
The original contributions presented in the study are included in the article/Supplementary Materials, further inquiries can be directed to the corresponding author.
Author contributions
ZY and YY designed the experiments. YY performed the experiment. ZJ, WF and HD contributed reagents/materials. YY wrote the manuscript. All authors gave final approval for publication.
Funding
This work was funded by the National Natural Science Foundation of China (Grant Nos. 32102202) to YY.; China Agriculture Research System (CARS-24-C-02) to ZY.
Acknowledgments
We would like to thanks to the Professor Hongxia Duan from China Agricultural University for her Homology modeling and molecular docking technical assistance in the laboratory.
Conflict of interest
The authors declare that the research was conducted in the absence of any commercial or financial relationships that could be construed as a potential conflict of interest.
Publisher’s note
All claims expressed in this article are solely those of the authors and do not necessarily represent those of their affiliated organizations, or those of the publisher, the editors and the reviewers. Any product that may be evaluated in this article, or claim that may be made by its manufacturer, is not guaranteed or endorsed by the publisher.
Supplementary material
The Supplementary Material for this article can be found online at: https://www.frontiersin.org/articles/10.3389/fphys.2022.989601/full#supplementary-material
References
Antony B., Soffan A., Jakše J., Abdelazim M. M., Aldosari S. A., Aldawood A. S., et al. (2016). Identification of the genes involved in odorant reception and detection in the palm weevil Rhynchophorus ferrugineus, an important quarantine pest, by antennal transcriptome analysis. BMC Genomics 17, 69. doi:10.1186/s12864-016-2362-6
Campanacci V., Lartigue A., Hallberg B. M., Jones T. A., Giudici-Orticoni M. T., Tegoni M., et al. (2003). Moth chemosensory protein exhibits drastic conformational changes and cooperativity on ligand binding. Proc. Natl. Acad. Sci. U. S. A. 100, 5069–5074. doi:10.1073/pnas.0836654100
Chang H. T., Liu Y., Yang T., Pelosi P., Dong S. L., Wang G. R. (2015). Pheromone binding proteins enhance the sensitivity of olfactory receptors to sex pheromones in Chilo suppressalis. Sci. Rep. 5, 13093. doi:10.1038/srep13093
Chen S., Yang P., Jiang F., Wei Y., Ma Z., Kang L. (2010). De novo analysis of transcriptome dynamics in the migratory locust during the development of phase traits. PLoS One 5, e15633. doi:10.1371/journal.pone.0015633
Chen G. L., Pan Y. F., Ma Y. F., Wang J., He M., He P. (2018). Binding affinity characterization of an antennae-enriched chemosensory protein from the white-backed planthopper, Sogatella furcifera (Horváth), with host plant volatiles.finity characterization of an antennae-enriched chemosensory protein from the white-backed planthopper, Sogatella furcifera (Horvath), with host plant volatiles. Pestic. Biochem. Physiol. 152, 1–7. doi:10.1016/j.pestbp.2018.09.006
Cheng D., Lu Y., Zeng L., Liang G., He X. (2015). Si-CSP9 regulates the integument and moulting process of larvae in the red imported fire ant. Solenopsis invicta. Sci. Rep. 5, 1–14. doi:10.1038/srep09245
Claridge-Chang A., Wijnen H., Naef F., Boothroyd C., Rajewsky N., Young M. W. (2001). Circadian regulation of gene expression systems in the Drosophila head. Neuron 32, 657–671. doi:10.1016/s0896-6273(01)00515-3
Cui Z. Y., Liu Y, P., Wang G, R., Zhou Q. (2022). Identification and functional analysis of a chemosensory protein from Bactrocera minax (Diptera: Tephritidae). Pest Manag. Sci. 78, 3479–3488. doi:10.1002/ps.6988
Dang Z. H., Dong J. Z., Gao Z. L., Jia H. M., Zhang K. J., Pan W. L. (2001). Biology and injury of Bradysia odoriphaga on leek in different types of cultivation. J. Agric. Univ. Hebei. 24, 65–68.
Feng H. Q., Zheng F. Q. (1987). Studies of the occurrence and control of Bradysia odoriphaga Yang et Zhang. J. Agri UnivK Shandong 18, 71–80.
Field L. M., Pickett J. A., Wadhams L. J. (2000). Molecular studies in insect olfaction. Insect Mol. Biol. 9, 545–551. doi:10.1046/j.1365-2583.2000.00221.x
French A., Ali Agha M., Mitra A., Yanagawa A., Sellier M. J., Marion-Poll F. (2015). Drosophila bitter taste(s). Front. Integr. Neurosci. 9, 207. doi:10.3389/fnint.2015.00058
Fu S. H., Li F. Y., Yan X. Z., Hao C. (2020). Expression profiles and binding properties of the chemosensory protein PxylCSP11 from the Diamondback Moth, Plutella xylostella (Lepidoptera: Plutellidae). J. Insect Sci. 20, 17–11. doi:10.1093/jisesa/ieaa107
Guo W., Wang X., Ma Z., Xue L., Han J., Yu D., et al. (2011). CSP and takeout genes modulate the switch between attraction and repulsion during behavioral phase change in the migratory locust. PLoS Genet. 7, e1001291. doi:10.1371/journal.pgen.1001291
Hansson B. S. (2002). A bug’s smell-research into insect olfaction. Trends Neurosci. 25, 270–274. doi:10.1016/s0166-2236(02)02140-9
Hua J. F., Pan C., H Y. M., Li Y. Q., Li H. F., Wu C. R., et al. (2021). Functional characteristic analysis of three odorant-binding proteins from the sweet potato weevil (Cylas formicarius) in the perception of sex pheromones and host plant volatiles. Pest Manag. Sci. 77, 300–312. doi:10.1002/ps.6019
Jacquin-Joly E., Vogt R. G., Francois M. C., Nagnan-Le M. P. (2001). Functional and expression pattern analysis of chemosensory proteins expressed in antennae and pheromonal gland of Mamestra brassicae. Chem. Senses 26, 833–844. doi:10.1093/chemse/26.7.833
Jannick D. B., Henrik N., Gunnar von H. J. (2004). Improved prediction of signal peptides: SignalP 3.0. J. Mol. Biol. 16, 783–795. doi:10.1016/j.jmb.2004.05.028
Jean-François P. (2014). Renaming Bombyx Mori chemosensory proteins. Int. J. Bioorg. Chem. Mol. Biol. 2, 1–4. doi:10.19070/2332-2756-140005e
Kitabayashi A. N., Arai T., Kubo T., Natori S. (1998). Molecular cloning of cDNA for p10, a novel protein that increases in the regenerating legs of Periplaneta americana (American cockroach). Insect biochem. Mol. Biol. 28, 785–790. doi:10.1016/s0965-1748(98)00058-7
Kulmuni J., Havukainen H. (2013). Insights into the evolution of the CSP gene family through the integration of evolutionary analysis and comparative protein modeling. PLoS One 8, e63688. doi:10.1371/journal.pone.0063688
Letunic L., Doerks T., Bork P. (2015). Smart: Recent updates, new developments and status in 2015. Nucleic Acids Res. 43, 257–260. doi:10.1093/nar/gku949
Li H. J., He X. K., Zeng A. J., Liu Y. J., Jiang S. R. (2007a). Bradysia odoriphaga copulatory behavior and evidence of a female sex pheromone. J. Agri. Urban Entomol. 24, 27–34. doi:10.3954/1523-5475-24.1.27
Li H. L., Lou B., Cheng J. A., Gao Q. (2007b). The chemosensory protein of Chinese honeybee, Apis cerana cerana: Molecular cloning of cDNA, immunocytochemical localization and expression. Chin. Sci. Bull. 52, 1355–1364. doi:10.1007/s11434-007-0210-4
Li W. X., Yang Y. T., Xie W., Wu Q. J., Xu B. Y., Wang S. L., et al. (2014). Effects of temperature on the age-stage, two-sex life table of Bradysia odoriphaga (Diptera: Sciaridae). J. Econ. Entomol. 108, 126–134. doi:10.1093/jee/tou011
Liu N. Y., Yang K., Liu Y., Xu W., Anderson A., Dong S. L. (2015). Two general-odorant binding proteins in Spodoptera litura are differentially tuned to sex pheromones and plant odorants. Comp. Biochem. Physiol. A Mol. Integr. Physiol. 180, 23–31. doi:10.1016/j.cbpa.2014.11.005
Liu H. W., Duan H. X., Wang Q., Xiao Y., Wang Q., Xiao Q., et al. (2019). Key amino residues determining binding activities of the odorant binding protein AlucOBP22 to two host plant terpenoids of Apolygus lucorum. J. Agric. Food Chem. 67, 5949–5956. doi:10.1021/acs.jafc.8b05975
Liu X. L., Zhang J., Yan Q., Miao C. L., Han W. K., Hou W., et al. (2020). The molecular basis of host selection in a crucifer-specialized moth. Curr. Biol. 30, 4476–4482. doi:10.1016/j.cub.2020.08.047
Ma J., Chen S. L., Maurice M. (2013). Efficacy of entomopathogenic nematodes (Rhabditida: Steinernematidae and Heterorhabditidae) against the chive gnat, Bradysia odoriphaga. J. Pest Sci. 86, 556–561. doi:10.1007/s10340-013-0497-7
Maguire S. E., Afify A., Goff L. A., Potter C. J. (2022). Odorant-receptor-mediated regulation of chemosensory gene expression in the malaria mosquito Anopheles gambiae. Cell Rep. 38, 110494. doi:10.1016/j.celrep.2022.110494
Maleszka J., Foret S., Saint R., Maleszka R. (2007). RNAi-induced phenotypes suggest a novel role for a chemosensory protein CSP5 in the development of embryonic integument in the honeybee (Apis mellifera). Dev. Genes Evol. 217, 189–196. doi:10.1007/s00427-006-0127-y
Mármol J. del., Yedlin M. A., Ruta V. (2021). The structural basis of odorant recognition in insect olfactory receptors. Nature 597, 126–131. doi:10.1038/s41586-021-03794-8
Matsunaga T., Reisenman C. E., Goldman-Huertas B., Brand P., Miao K., Suzuki H. C., et al. (2022). Evolution of olfactory receptors tuned to mustard oils in herbivorous drosophilidae. Mol. Biol. Evol. 39, msab362. doi:10.1093/molbev/msab362
Northey T., Venthur H., De Biasio F., Chauviac F. X., Cole A., Ribeiro K. A. L., et al. (2016). Crystal structures and binding dynamics of odorant-binding protein 3 from two aphid species Megoura viciae and Nasonovia ribisnigri. Sci. Rep. 6, 24739. doi:10.1038/srep24739
Oliveira D. S., Brito N. F., Franco T. A., Moreira M. F., Leal W. S., Melo A. C. A. (2018). Functional characterization of odorant binding protein 27 (RproOBP27) from Rhodnius prolixus antennae. Front. Physiol. 9, 1175. doi:10.3389/fphys.2018.01175
Pelosi P., Calvello M., Ban L. (2005). Diversity of odorant-binding proteins and chemosensory proteins in insects. Chem. Senses 30, 291–292. doi:10.1093/chemse/bjh229
Pelosi P., Zhou J. J., Ban L. P., Calvello M. (2006). Soluble proteins in insect chemical communication. Cell. Mol. Life Sci. 63, 1658–1676. doi:10.1007/s00018-005-5607-0
Pelosi P., Iovinella I., Zhu J., Wang G., Dani F. R. (2018). Beyond chemoreception: Diverse tasks of soluble olfactory proteins in insects. Biol. Rev. Camb. Philos. Soc. 93, 184–200. doi:10.1111/brv.12339
Prestwich G. D. (1993). Bacterial expression and photo affinity labeling of a pheromone binding protein. Protein Sci. 2, 420–428. doi:10.1002/pro.5560020314
Shi C. H., Yang F. S., Zhu X., Du E. X., Yang Y. T., Wang S. L., et al. (2016). Evaluation of housekeeping genes for quantitative real-time PCR analysis of Bradysia odoriphaga (Diptera: Sciaridae). Int. J. Mol. Sci. 17, 1034. doi:10.3390/ijms17071034
Sun H., Guan L., Feng H., Yin J., Cao Y., Xi J., et al. (2014). Functional characterization of chemosensory proteins in the scarab beetle, Holotrichia oblita Faldermann (Coleoptera: Scarabaeida). PLoS One 9, e107059. doi:10.1371/journal.pone.0107059
Sun X., Zeng F. F., Yan M. J., Zhang A., Lu Z. X., Wang M. Q. (2016). Interactions of two odorant-binding proteins influence insect chemoreception. Insect Mol. Biol. 25, 712–723. doi:10.1111/imb.12256
Tamura K., Stecher G., Peterson D., Filipski A., Kumar S. (2013). MEGA6: Molecular evolutionary genetics analysis version 6.0. Mol. Biol. Evol. 30, 2725–2729. doi:10.1093/molbev/mst197
Tang B. W., Tai S. L., Dai W., Zhang C. N. (2019). Expression and functional analysis of two odorant-binding proteins from Bradysia odoriphaga (Diptera: Sciaridae). J. Agric. Food Chem. 67, 3565–3574. doi:10.1021/acs.jafc.9b00568
Tomaselli S., Crescenzi O., Sanfelice D., Ab E., Wechselberger R., Angeli S., et al. (2006). Solution structure of a chemosensory protein from the desert locust Schistocerca gregaria. Biochemistry 45, 10606–10613. doi:10.1021/bi060998w
Visser J. H. (1986). Host odor perception in phytophagous insects. Annu. Rev. Entomol. 31, 121–144. doi:10.1146/annurev.en.31.010186.001005
Vogt R. G., Riddiford L. M., Prestwich G. D. (1985). Kinetic properties of a sex pheromone-degrading enzyme: The sensillar esterase of Antheraea polyphemus. Proc. Natl. Acad. Sci. U. S. A. 82, 8827–8831. doi:10.1073/pnas.82.24.8827
Wang R., Zhang X. M., Li F. Q., Wu F., Li H. L., Luo C. (2016). Cloning and prokaryotic expression of odorant binding protein OBP8 in MED cryptic species Bemisia tabaci and the binding characteristics with plant volatiles. J. Plant Prot. 43, 32–39.
Wanner K. W., Willis L. G., Theilmann D. A., Isman M. B., Feng Q., Plettner E. (2004). Analysis of the insect os-d-like gene family. J. Chem. Ecol. 30, 889–911. doi:10.1023/b:joec.0000028457.51147.d4
Waris M. I., Younas A., ul Qamar M. T., Hao L., Ameen A., Ali S., et al. (2018). Silencing of chemosensory protein gene NlugCSP8 by RNAi induces declining behavioral responses of Nilaparvata lugens. Front. Physiol. 9, 379. doi:10.3389/fphys.2018.00379
Wei Y., Brandazza A., Pelosi P. (2008). Binding of polycyclic aromatic hydrocarbons to mutants of odorant-binding protein: A first step towards biosensors for environmental monitoring. Biochim. Biophys. Acta 1784, 666–671. doi:10.1016/j.bbapap.2008.01.012
Xu H. F., Pan Y., Li J. Y., Yang F. T., Chen X. W., Gao X. W., et al. (2022). Chemosensory proteins confer adaptation to the ryanoid anthranilic diamide insecticide cyantraniliprole in Aphis gossypii Glover. Pestic. Biochem. Physiol. 184, 105076. doi:10.1016/j.pestbp.2022.105076
Yang Y. T., Li W. X., Xie W., Wu Q. J., Xu B. Y., Wang S. L., et al. (2014). Development of Bradysia odoriphaga (Diptera: Sciaridae) as affected by humidity: An age-stage, two-sex life table study. Appl. Entomol. Zool. 50, 3–10. doi:10.1007/s13355-014-0295-6
Yang Y. T., Su Q., Shi L. L., Chen G., Zeng Y., Shi C. H., et al. (2019). Electrophysiological and behavioral responses of Bradysia odoriphaga (Diptera: Sciaridae) to volatiles from its host plant, Chinese Chives (Allium tuberosum Rottler ex Spreng). J. Econ. Entomol. 112, 1638–1644. doi:10.1093/jee/toz057
Yang Y. T., Hua D. K., Shi C. H., Xie W., Zhang Y. J. (2021a). Molecilar and binding characteristic of OBP5 of Bradysia odoriphaga (Diptera: Sciaridae). J. Econ. Entomol. 114, 1509–1516. doi:10.1093/jee/toab095
Yang Y. T., Luo L., Tian L. X., Zhao C. W., Niu H. L., Hu Y. F., et al. (2021b). Function and characterization analysis of BodoOBP8 from Bradysia odoriphaga (Diptera: Sciaridae) in the recognition of plant volatiles and sex pheromones. Insects 12, 879. doi:10.3390/insects12100879
Younas A., Waris M. I., Shaaban M., Qamar M. T., Wang M. Q. (2022). Appraisal of MsepCSP14 for chemosensory functions in Mythimna separate. Insect Sci. 29, 162–176. doi:10.1111/1744-7917.12909
Zeng F. F., Liu H., Zhang A., Lu Z. X., Leal W. S., Abdelnabby H., et al. (2018). Three chemosensory proteins from the rice leaf folder Cnaphalocrocis medinalis involved in host volatile and sex pheromone reception. Insect Mol. Biol. 27, 710–723. doi:10.1111/imb.12503
Zeng Y., Merchant A., W Q. J., Wang S. L., Kong L., Zhou X., et al. (2020). A chemosensory protein BtabCSP11 mediates reproduction in Bemisia tabaci. Front. Physiol. 11, 709. doi:10.3389/fphys.2020.00709
Zhang Y. N., Ye Z. F., Yang K., Dong S. L. (2014). Antenna-predominant and male-biased CSP19 of Sesamia inferens is able to bind the female sex pheromones and host plant volatiles. Gene 536, 279–286. doi:10.1016/j.gene.2013.12.011
Zhang Z. J., Li W. X., He M., Zhu X. D., Lin W. C., Li X. W., et al. (2016). Behavioral responses of female Bradysiaod oriphaga Yang et Zhang to volatiles of conspecific larvae, pupae and eggs. Chin. J. Appl. Entom. 53, 1198–1204.
Zhang C. N., Tang B. W., Zhou T. L., Yu X. T., Hu M. F., Dai W. (2021). Involvement of chemosensory protein BodoCSP1 in perception of host plant volatiles in Bradysia odoriphaga. J. Agric. Food Chem. 69, 10797–10806. doi:10.1021/acs.jafc.1c02807
Zhao Y., Ding J., Zhang Z., Liu F., Zhou C., Mu W. (2018). Sex- and tissue-specific expression profiles of odorant binding protein and chemosensory protein genes in Bradysia odoriphaga (Diptera: Sciaridae).fic expression profiles of odorant binding protein and chemosensory protein genes in Bradysia odoriphaga (Diptera: Sciaridae). Front. Physiol. 9, 107. doi:10.3389/fphys.2018.00107
Zheng J., Li J., Han L., Wang Y., Wu W., Qi X., et al. (2015). Crystal structure of the Locusta migratoria odorant binding protein. Biochem. Biophys. Res. Commun. 456, 737–742. doi:10.1016/j.bbrc.2014.12.048
Keywords: Bradysia odoriphaga, chemosensory protein 4, competitive binding assays, RNAi, Y-tube olfaction assay
Citation: Yang Y, Hua D, Zhu J, Wang F and Zhang Y (2022) Chemosensory protein 4 is required for Bradysia odoriphaga to be olfactory attracted to sulfur compounds released from Chinese chives. Front. Physiol. 13:989601. doi: 10.3389/fphys.2022.989601
Received: 08 July 2022; Accepted: 12 September 2022;
Published: 27 September 2022.
Edited by:
Carolina E Reisenman, University of California, United StatesReviewed by:
Teruyuki Matsunaga, The University of Tokyo, JapanYoussef Dewer, Agricultural Research Center, Egypt
Copyright © 2022 Yang, Hua, Zhu, Wang and Zhang. This is an open-access article distributed under the terms of the Creative Commons Attribution License (CC BY). The use, distribution or reproduction in other forums is permitted, provided the original author(s) and the copyright owner(s) are credited and that the original publication in this journal is cited, in accordance with accepted academic practice. No use, distribution or reproduction is permitted which does not comply with these terms.
*Correspondence: Youjun Zhang, zhangyoujun@caas.cn