- 1College of Horticulture and Plant Protection, Henan University of Science and Technology, Luoyang, China
- 2Institute of Plant Protection, Henan Academy of Agricultural Sciences, Zhengzhou, China
Although most of the damage caused by lepidopteran insects to plants is caused by the larval stage, chemosensory systems have been investigated much more frequently for lepidopteran adults than for larvae. The fall armyworm Spodoptera frugiperda (J. E. Smith) (Lepidoptera: Noctuidae) is a polyphagous and worldwide pest. To understand the larval chemosensory system in S. frugiperda, we sequenced and assembled the antennae and maxillae transcriptome of larvae in the sixth instar (larval a-m) using the Illumina platform. A total of 30 putative chemosensory receptor genes were identified, and these receptors included 11 odorant receptors (ORs), 4 gustatory receptors (GRs), and 15 ionotropic receptors/ionotropic glutamate receptors (IRs/iGluRs). Phylogeny tests with the candidate receptors and homologs from other insect species revealed some specific genes, including a fructose receptor, a pheromone receptor, IR co-receptors, CO2 receptors, and the OR co-receptor. Comparison of the expression of annotated genes between S. frugiperda adults and larvae (larval a-m) using RT-qPCR showed that most of the annotated OR and GR genes were predominantly expressed in the adult stage, but that 2 ORs and 1 GR were highly expressed in both the adult antennae and the larval a-m. Although most of the tested IR/iGluR genes were mainly expressed in adult antennae, transcripts of 3 iGluRs were significantly more abundant in the larval a-m than in the adult antennae of both sexes. Comparison of the expression levels of larval a-m expressed chemosensory receptors among the first, fourth, and sixth instars revealed that the expression of some of the genes varied significantly among different larval stages. These results increase our understanding of the chemosensory systems of S. frugiperda larvae and provide a basis for future functional studies aimed at the development of novel strategies to manage this pest.
Introduction
Chemical communication is essential for various insect behaviors such as mating, feeding, foraging, and oviposition (Hildebrand and Shepherd, 1997). The chemosensory process consists of several major events, including the conversion of compounds into electrical signals at the periphery, the integration of the electrical signals into the antennae lobes (for olfaction) or the subesophageal ganglion (for gustation), and ultimately the production of behavioral signals in brain centres (Leal, 2013; Wilson, 2013; He et al., 2022). At the periphery level, the chemosensory process is mainly mediated by three families of receptor genes including odorant receptors (ORs), gustatory receptors (GRs), and ionotropic receptors (IRs) (Dahanukar et al., 2005; Hansson and Stensmyr, 2011).
Insect GRs and ORs were first identified in the model insect Drosophila melanogaster (Gao and Chess, 1999; Clyne, 2000). These GRs and ORs are seven transmembrane proteins (350–500 amino acids), which have a similar motif at the C-terminal (Robertson et al., 2003). Compared to the classic vertebrate G-protein coupled receptors, insect GRs and ORs have an inverted topology (Benton et al., 2006). Receptors in the OR family are classified into two types: highly conserved OR co-receptors (ORco) and a variable “tuning” receptor (ORx). Insect ORs are heterodimers composed of a unique OR and the ORco. The heterodimer acts as a ligand-gated ion channel and may also function in metabolic signal transduction pathways (Sato et al., 2008; Wicher et al., 2008; Stengl, 2010). Insect ORs have also been reported to be heterotetramers based upon the cryo-electron microscopy structure of an ORco homomer from the parasitic wasp Apocrypta bakeri (Butterwick et al., 2018). Insect GRs occur mostly in gustatory sensilla that contact food or other substances being tasted. GRs can detect bitter and sweet compounds as well as carbon dioxide (Scott et al., 2001). Genes in the GR family can be divided into several major clades, which include genes that encode GRs that detect carbon dioxide (Jones, et al., 2007), sugar compounds (Fujii et al., 2015), fructose (Miyamoto et al., 2012), and bitter compounds (Delventhal and Carlson, 2016).
Like studies of insect GRs and ORs, studies of insect IRs were initiated with D. melanogaster. Insect IRs have evolved from the neurotransmitter receptors for glutamate, i.e., ionotropic glutamate receptors (iGluRs) (Benton et al., 2009; Croset et al., 2010; Robertson, 2019). Insect IRs are expressed in various tissues, have diverse functions, and can be classified into two groups based on their expression profile and sequence type. The “antennal IRs” are mainly expressed in the antennae and especially in neurons of coeloconic sensilla, which have been implicated in the sensing of amino acids, acids, and amines (Silbering et al., 2011; Ai et al., 2013; Hussain et al., 2018). Most insect IRs are “divergent IRs”. Receptors in this subgroup have variable sequences and are expressed in different tissues of the insects (Croset et al., 2010). Although the majority of divergent IRs are involved in olfaction and gustation (Koh et al., 2014), some function in the sensing of sound, temperature, and humidity (Knecht et al., 2016; Frank et al., 2017). Similar to the ORs, co-expression is also observed among IRs. Insect IRs can function in complex combinations, with at least three members (IR8a, IR25a, and IR76b) acting as co-receptors with tuning IRs (Abuin et al., 2011; Chen et al., 2015; Ni et al., 2016; Ganguly et al., 2017).
Moths constitute a large group of members in the Lepidoptera. During the lifetime of moths, their adults mainly function in mating and oviposition, while the larvae mainly function in feeding and thereby cause substantial damage to crops and other plants. Control of moth pests depends on understanding the chemosensory systems of both adults and larvae. Most researchers have focused on the chemosensory mechanisms of adult moths (Montagné et al., 2012; Chang et al., 2017; Liu et al., 2020), and relatively little is known about the chemosensory mechanisms of moth larvae. However, because they have a simple chemosensory system, with antennae and maxillae being their main chemosensory organs (Dethier and Schoonhoven, 1969; Itagaki and Hildebrand, 1990), moth larvae are ideal models for research on insect chemoreception. Identifications of larval chemosensory receptor repertoires had been reported in several moth species, including (but not limited to) Bombyx mori (Tanaka et al., 2009), Spodoptera littoralis (Poivet et al., 2013), Helicoverpa armigera (Liu et al., 2014), H. assulta (Xu et al., 2015), and S. litura (Li et al., 2021). Among which, functions for a few specific chemosensory receptors have been characterized. Comprehensive investigation of ORs in the larval stage of the silkworm B. mori indicated that a specifically receptor, BmOR56, may mediate the attraction of the mulberry leave volatile cis-jasmone to the caterpillars (Tanaka et al., 2009). Tissue-specific expression using RT-PCR found nine ORs in larval S. littoralis, and behavioral investigation revealed that these ORs are related to the caterpillar attraction to nine plant volatiles (De Fouchier et al., 2018). A recent study of a putative bitter GR (BmorGR66) from B. mori shows that BmorGR66 is mainly expressed in the larval maxilla and is responsible for the larvae feeding preference (Zhang et al., 2019).
The fall armyworm Spodoptera frugiperda (Lepidoptera: Noctuidae) is an important pest that originated in tropical and subtropical regions in North and South America (Sparks, 1979). It invaded the United States and Canada in the middle of the 19th century (Johnson, 1987; Early et al., 2018). Because of its strong migratory ability, S. frugiperda has spread to over 40 African countries within the last 6 years (Goergen et al., 2016; Cock et al., 2017). It has also recently invaded and rapidly spread across China (Wu et al., 2019). S. frugiperda is highly polyphagous, and its larvae can feed on many important cultivated crops such as rice, corn, peanuts, and soybeans (Meagher and Nagoshi, 2012). Pair-wise choice tests showed that S. frugiperda larvae can discriminate among inbred lines of maize, and are attracted to or not attracted to larvae-infested plants depending on the plant variety (Yactayo-Chang et al., 2021). The molecular basis underlying the chemosensory system in S. frugiperda larvae, however, is unknown.
In this study, we sequenced the transcriptome of the antennae and maxillae of S. frugiperda larvae and identified 11 ORs, 4 GRs, and 15 IRs/iGluRs. We constructed phylogenetic trees of these receptors and homologs in other insect species in order to determine the putative functions of the candidate genes. We then used real-time quantitative-PCR (RT-qPCR) to investigate the expressional profiles of these genes in S. frugiperda larvae and adults. Furthermore, we analyzed the expression levels of varoius chemosensory receptor genes at different larval development stages using RT-qPCR. The results provide a foundation for further studies of the functions of the chemoreceptors in S. frugiperda larvae and for the development of novel methods to control this pest.
Materials and methods
Insect rearing
Spodoptera frugiperda larvae were collected from a corn field in Shidian County, Baoshan, Yunnan Province, China. They were then reared in the laboratory at Henan University of Science and Technology, Luoyang, China. The insects were reared in a climatic cabinet at 26 ± 1°C with a relative humidity of 70% ± 5% and a photoperiod of 16 h: 8 h (L: D). The larvae were fed with an artificial diet that mainly contained wheat germ, corn leaf powder, and yeast powder. Male and female pupae were placed in separate cages for emergence. Adults were supplied with a 15% honey solution (v/v). Seven male moths and eight female moths were kept in a net cage for mating and oviposition.
Tissue collection and total RNA extraction
For transcriptome sequencing and RT-qPCR, we dissected the head of 200 S. frugiperda larvae in the first instar (1 day after hatching), the antennae and maxillae of 400 larvae in the fourth instar (second day in the fourth instar), the antennae and maxillae of 400 larvae in the sixth instar (second day in the sixth instar), the antennae of 80 adult males (3 days after eclosion), and the antennae of 80 adult females (3 days after eclosion). The five kinds of samples were immediately immersed in liquid nitrogen and were subjected to total RNA extraction with the RNeasy Plus Mini Kit (Qiagen, Venlo, Netherlands) according to the manufacturer’s instructions. The purity and concentration of the total RNA were determined based on the OD230, OD260, and OD280 values as measured with a spectrophotometer (Nano Drop 2000; Nano-Drop Products, Wilmington, DE, United States). The extracted RNA was used for transcriptome sequencing and RT-qPCR as described in the following sections. The following sections also indicate the numbers of replications used for each determination.
Transcriptome sequencing and assembly
According to the age-stage analysis of S. frugiperda, there are six instars during the larval period, the fifth and sixth instars are assigned to gluttonous stage, and duration of the sixth instar is the longest among all the larval development stages (Dai et al., 2020; Wang et al., 2020; Xie et al., 2021). Similar development stages were observed on artificial diet-fed S. frugiperda in our study. Therefore, Illumina sequencing of the total RNA from the larval antennae and maxillae of S. frugiperda on the second day in the sixth instar (the tissue is hereafter specifically referred to as the “larval a-m”) was performed at Sangon Biotech (Shanghai, China) using methods that were similar to those previously described (Sun et al., 2020). A 10-µg quantity (concentration ≥50 ng/μl) of total RNA was used for the synthesis of cDNA. Three biological replications were conducted. The cDNA libraries were then prepared, and paired-end reads were obtained from the cDNA libraries using the Illumina HiSeq 2000 platform. Sequence assembly was carried out with the transcriptome de novo assembly program (https://github.com/trinityrnaseq/trinityrnaseq/). TGICL (TGI Clustering) and Cap3 tools were then used to cluster the Trinity outputs and to thereby produce the unigenes.
Identification and annotation of chemosensory receptors
Annotation of unigenes was performed as previously described (Sun et al., 2020). To retrieve proteins with the highest sequence similarity (E-values < 1e-5), we conducted BLAST searches against the sequences in the non-redundant (Nr) database in the NCBI, and in the COG (Clusters of Orthologous Groups of proteins), Swiss-Prot (http://www.ebi.ac.uk/uniprot), KEGG (Kyoto encyclopedia of genes and genomes), and GO (gene ontology) databases (Ashburner et al., 2000; Kanehisa, 2004; Xie et al., 2011).
Alignments comparing transcripts encoding putative larval ORs, GRs, and IRs/iGluRs were manually performed using the BLASTx tool in NCBI. The open reading frames (ORFs) of candidate chemosensory receptor genes were predicted using the Translate program (http://web.expasy.org/translate/). The full-length transcripts were determined by considering the BLASTx results as well as the start and stop codons.
The expression levels of annotated genes were estimated using the transcripts per kilobase of exon per million mapped (TPM) method (http://deweylab.github.io/RSEM/). The average TPM value of three biological replications of the sample was calculated.
Phylogenetic analysis
The OR phylogenetic tree was built based on amino acid sequences from the datasets of insect species including S. frugiperda (this study), H. armigera, and B. mori. The GR phylogenetic tree was built based on amino acid sequences from the datasets of S. frugiperda (this study), B. mori, H. armigera, and Danaus plexippus. The IR/iGluR phylogenetic tree was built based on the amino acid sequences from the datasets of S. frugiperda (this study), Dendrolimus punctatus, H. armigera, and D. melanogaster. Sequences were aligned and neighbor-joining trees were constructed using MEGA11 as previously described (Sun et al., 2021). The trees were subsequently visualized and edited with Figtree v1.4.2 (http://tree.bio.ed.ac.uk/software/figtree/). The amino acid sequences of ORs, GRs, and IRs/iGluRs used in phylogenetic analyses are listed in Supplementary Table S1.
RT-qPCR
RT-qPCR was firstly conducted to compare the relative expression levels of annotated S. frugiperda chemosensory receptor genes in the larval a-m, adult male antennae, and adult female antennae. Expression level analyses of the larval a-m expressed genes were then conducted in the first instar larval head (too small to separate the antennae and maxillae), the fourth instar larval antennae and maxillae, and the sixth instar larval antennae and maxillae. The S. frugiperda β-actin gene was used as the internal reference for normalizing the expression levels of the target genes. Three biological reactions were conducted for each of the three kinds of tissue samples, and each reaction was performed three times as technical replicates. Melting curves were then observed, and five PCR products were randomly selected for sequencing to avoid non-specific amplifications. Relative expression levels of all tested genes were calculated using the 2−ΔCt method (Schmittgen and Livak, 2008).
The data were analyzed and figures were made with GraphPad Prism 6 (GraphPad Software Inc., San Diego, CA). The primers used in the RT-qPCR assays were designed using Primer Premier 5.0 software (PREMIER Biosoft International) and are listed in Supplementary Table S2.
Results
Larval transcriptome and annotation
In this study, we generated a transcriptome of the S. frugiperda larval a-m using Illumina sequencing. An average of 56.52 million clean reads were collected, and the average Q30 base ratio and GC base ratio were 90.32% and 46.98%, respectively (Supplementary Table S3). A total of 96,197 unigenes were finally assembled, with a mean length of 633 bp and an N50 length of 972 bp. Among the 96,197 unigenes, 14,171 (14.73%) were >1000 bp (Supplementary Table S4). Among the 96,197 unigenes, 44.23% (42,548) had matches (E-value < 1e-5) in the NR database. The highest homology was with S. frugiperda (33,165 unigenes, 77.95%), followed by S. litura (2,185 unigenes, 5.14%), S. exigua (1063 unigenes, 2.50%), and H. armigera (845 unigenes, 1.98%) (Supplementary Figure S1).
Among the 96,197 unigenes in S. frugiperda larval a-m, 16,227 (16.87%) corresponded to at least one GO category. Among the 16,227 unigenes, 7,902 were assigned to the “molecular function” (48.7%), 11,829 to the “biological process” (72.9%), and 9,719 to the “cellular component” (59.9%). The three most represented terms were “binding”, “cellular process”, and “cell” (Supplementary Figure S2).
Candidate ORs
A total of 11 candidate OR genes were identified in the S. frugiperda larval a-m transcriptome (Table 1). For convenience, we numbered the identified chemosensory receptors in this study according to the numbers previously used for S. frugiperda sequences (whenever possible) or for best matched homologs in other moth species. The putative chemosensory receptors displayed 99%–100% amino acid sequence identities to the sequences of the chemosensory receptors reported in the genome analysis of S. frugiperda (Gouin et al., 2017). All of the identified SfruORs have complete ORFs based on the presence of start and stop codons, and on the results of BLASTx alignment to other lepidopteran ORs. Their nucleotide/amino acid sequences and GenBank accession numbers are listed in Supplementary Table S5.
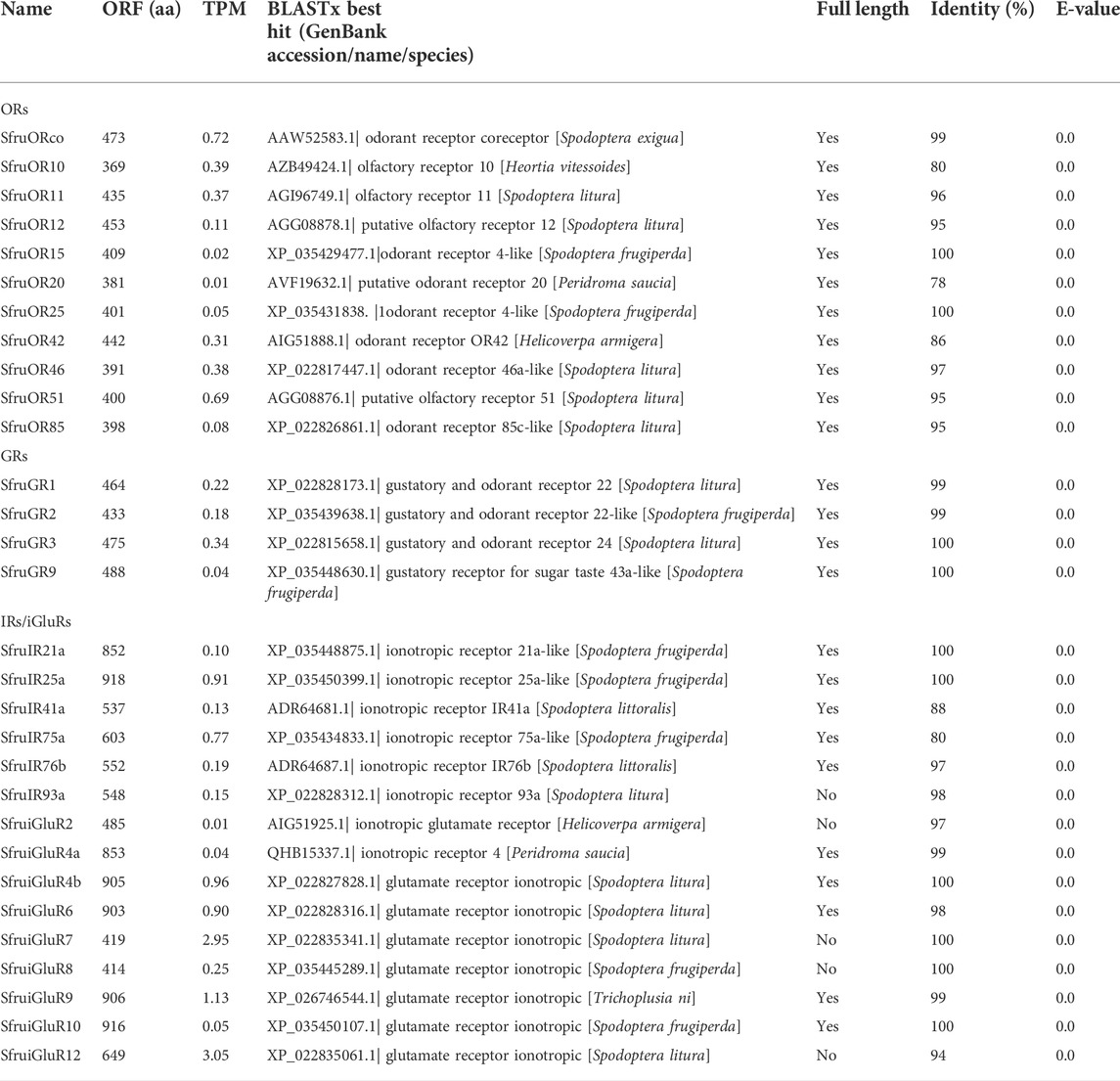
TABLE 1. Unigenes of candidate chemosensory receptors in larval antennae and maxilla of S. frugiperda.
A phylogenetic tree conducted with the OR dataset of S. frugiperda (this study), H. armigera, and B. mori revealed that we identified the ORco gene in the S. frugiperda larval a-m. We also identified a member (SfruOR11) of the lepidopteran PR subfamily, whose homologs in H. armigera and B. mori are involved in sex pheromone perception (Sakurai et al., 2004; Nakagawa et al., 2005; Liu et al., 2013; Jiang et al., 2014). Five ORs (SfruOR20, SfruOR25, SfruOR42, SfruOR46, and SfruOR51) appeared in different branches of the phylogenetic tree. The other four ORs (SfruOR10, SfruOR12, SfruOR15, and SlitIR85) clustered in an S. frugiperda-specific OR branch (Figure 1A).
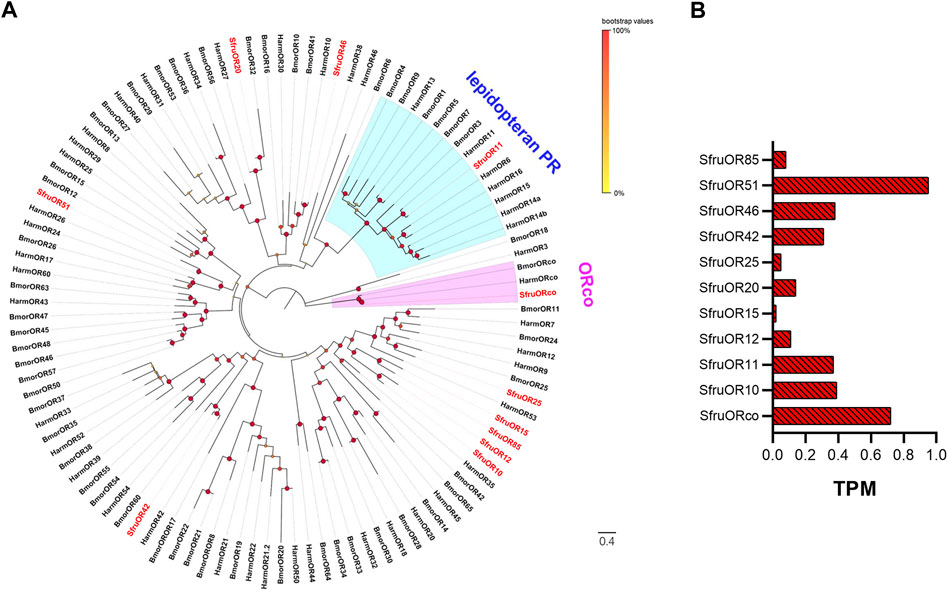
FIGURE 1. Candidate ORs of S. frugiperda larvae. (A) Phylogenetic relationships of ORs from S. frugiperda (Sfru, this study), B. mori (Bmor), and H. armigera (Harm). The neighbor-joining tree was constructed using MEGA11 (1000 bootstrap replicates). The tree was rooted by the ORco orthologs. The ORco clade is highlighted in pink; the lepidopteran pheromone receptor (PR) branches are highlighted in blue. (B) TPM values of candidate ORs in antennae and maxillae of S. frugiperda larvae.
The expression levels of the 11 candidate SfruOR genes in the larval a-m were evaluated using the TPM method. The results indicated that SfruORco was the most abundantly expressed OR (TPM = 0.72). SfruOR51 had the second highest expression level, with a TPM value of 0.69. The candidate PR, SfruOR11, had a modest TPM value of 0.37, which was only slightly lower than the value of the third most highly expressed OR, SfruOR46 (TPM = 0.38) (Table 1; Figure 1B).
Expression patterns of the 11 candidate SfruORs in S. frugiperda adult male antennae, adult female antennae, and the larval a-m were examined by RT-qPCR. The results showed that the expression levels of most of the tested SfruORs were higher in the adult antennae than in larval a-m, that expression of SfruOR46 and SfruOR85 was greater in female than in male antennae, and that the expression of SfruOR11 was greater in male than in female antennae. Three transcripts, SfruOR11/12/20, appeared to be adult-specific, i.e., their expression was barely detected in larval a-m. The expression levels of two SfruORs (SfruOR10 and SfruOR51) were similar to or tended to be slightly higher in the larval a-m than in the adult antennae of both sexes (Figure 2).
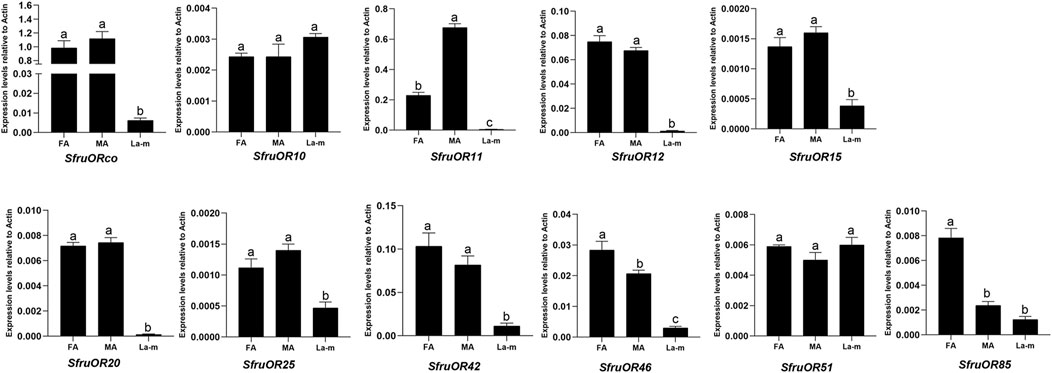
FIGURE 2. Expression profiles of candidate SfruORs in S. frugiperda larvae and adults. RT-qPCR analysis of candidate OR genes in male antennae (MA), female antennae (FA), and larval antennae and maxillae (L a-m). Values are means ± SE. Different letters indicate significant differences (Tukey’s multiple comparison test. after a one-way ANOVA, p < 0.05, n = 3).
Candidate GRs
We identified a total of 4 candidate unigenes encoding SfruGRs in the S. frugiperda larval a-m transcriptome, and all four had full-length ORFs (Table 1, Supplementary Table S5). Phylogenetic analysis based on amino acid sequences of GRs from S. frugiperda (this study), H. armigera, B. mori, and D. plexippus showed that three SfruGRs (SfruGR1/2/3) were grouped with HarmGR1/2/3 and BmorGR1/2/3, which are candidate “CO2 receptors”. Another GR, SfruGR9, was clustered in the “fructose receptors” branch (Table1; Figure 3A), whose orthologs in H. armigera (amino acid identity 96%) and B. mori (amino acid identity 64%) were previously demonstrated to be involved in the perception of fructose (Sato et al., 2011; Xu et al., 2012; Jiang et al., 2015).
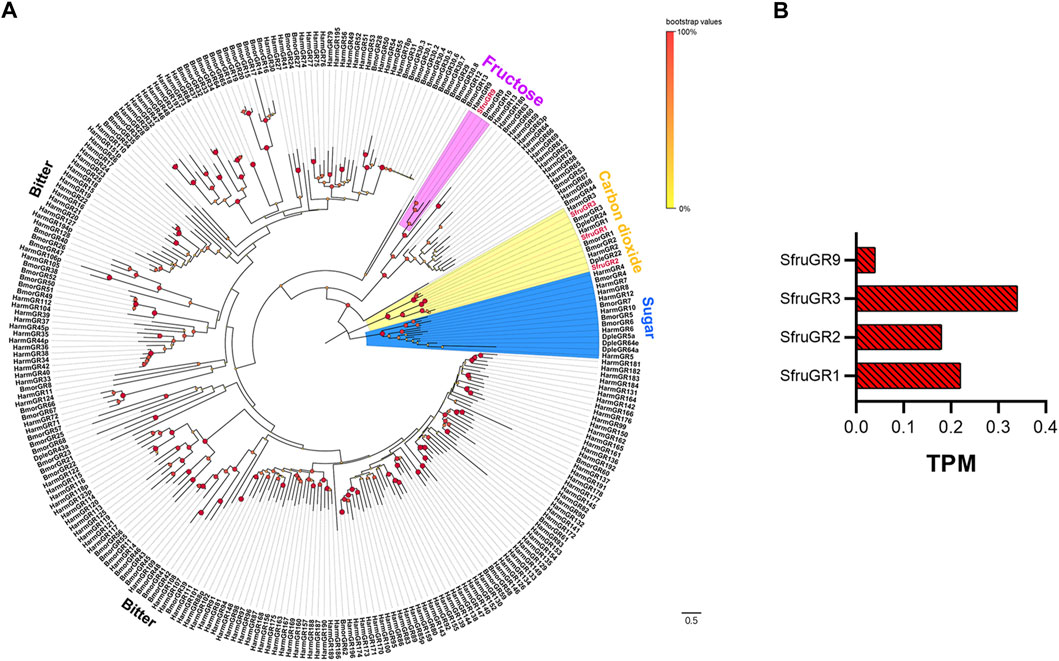
FIGURE 3. Candidate GRs of S. frugiperda larvae. (A) Phylogenetic relationships of GRs from S. frugiperda (Sfru, this study), B. mori (Bmor), H. armigera (Harm), and D. plexippus (Dple). The neighbor-joining tree was constructed using MEGA11 (1000 bootstrap replicates). The tree was rooted with the conservative fructose receptors. The “carbon dioxide receptor” branches are highlighted in yellow; the “fructose receptors” are highlighted in pink; the “sugar-taste receptors” are highlighted in blue; and the “bitted-taste receptor” branches are not highlighted. (B) TPM values of candidate GRs in antennae and maxillae of S. frugiperda larvae.
According to TPM values, the most transcribed gene among the four putative SfruGRs was SfruGR3 (TPM = 0.34), which was followed by SfruGR1 (TPM = 0.22) and SfruGR2 (TPM = 0.18). The least transcribed of the putative SfruGRs was SfruGR9 (TPM = 0.04) (Table1; Figure 3B).
The four candidates SfruGRs were found to be expressed in all three of the tested tissues. Expression of SfruGR1 and SfruGR2 was significantly higher in male antennae than in female antennae or in larval a-m, and expression of SfruGR3 and SfruGR9 was tended to be higher in female antennae than in male antennae or in larval a-m. SfruGR3 was also highly expressed in the larval a-m, i.e., its expression was slightly lower (but not significantly lower) in the larval a-m than in the female antennae (Figure 4).

FIGURE 4. Expression profiles of candidate SfruGRs in S. frugiperda larvae and adults. RT-qPCR analysis of candidate GR genes in male antennae (MA), female antennae (FA), and larval antennae and maxillae (L a-m). Values are means ± SE. Different. letters indicate significant differences (Tukey’s multiple comparison test after a one-way ANOVA, p < 0.05, n = 3).
Candidate IRs/iGluRs
According to the transcriptome analysis, a total of 6 putative SfruIRs and 9 putative SfruiGluRs were identified (Table 1). Five of the 6 putative SfruIRs had full-length ORFs, and 5 of the 15 SfruIRs/iGluRs lacked a C-terminal (SfruiGluR2/7), an N-terminal (SfruIR93a/SfruiGluR8), or both a C- and an N-terminal (SfruiGluR12) (Supplementary Table S5). A phylogenetic tree built with these SfruIRs/iGluRs and homologs from D. melanogaster, H. armigera, and D. punctatus showed that the candidate SfruIR25a and SfruIR76b were distributed in the co-receptor IR25a and IR76b lineages, respectively. Four IRs (SfruIR21a/41a/75a/93a) were clustered in the “antennal IRs” sub-group. None of the SfruIRs appeared in the “divergent IRs” subgroup (Figure 5A).
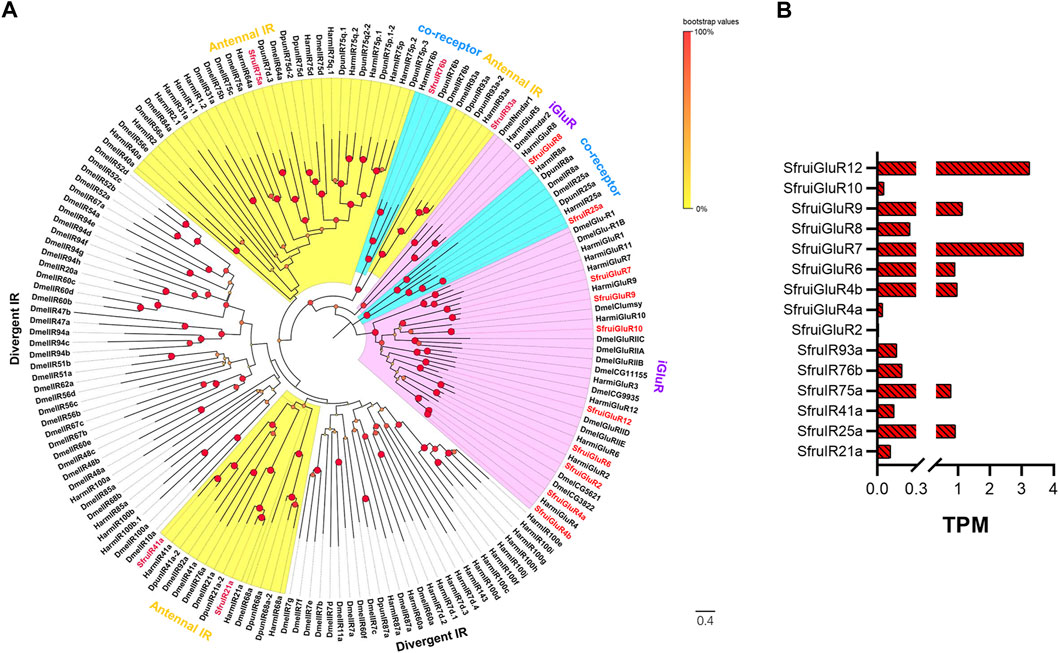
FIGURE 5. Candidate IRs/iGluRs of S. frugiperda larvae (A) Phylogenetic relationships of IRs/iGluRs from S. frugiperda (Sfru, this study), H. armigera (Harm), D. melanogaster (Dmel), and D. punctatus (Dpun). The neighbor-joining tree was constructed using MEGA11 (1000 bootstrap replicates). The tree was rooted with the conservative iGluRs genes. The IR co-receptor branches are highlighted in blue; the ionotropic glutamate receptor (iGluRs) branches are highlighted in purple; the “antennal IR” branches are highlighted in yellow; the “divergent IR” branches are not highlighted. (B) TPM values of candidate IRs/iGluRs in antennae and maxillae of S. frugiperda larvae.
TPM calculation demonstrated that the most enriched SfruIR/iGluR in the S. frugiperda larval a-m was SfruiGluR12 (TPM = 3.05), followed by SfruiGluR7 (TPM = 2.95) and SfruiGluR9 (TPM = 1.13). The co-receptor SfruIR25a had a low TPM value of 0.91, which was higher than that of the co-receptor SfruIR76b (TPM = 0.19) (Table1; Figure 5B).
According to RT-qPCR analysis, the expression of the 6 candidate SfruIR genes was greater in the antennae of S. frugiperda adult females and males than in the larval a-m. The expression of SfruiGluR2/4a/4b/8 was also greater in the antennae of S. frugiperda adult females and males than in the larval a-m. Although expression levels of SfruiGluR10/12 were similar between adult antennae and the larval a-m, the expression levels of SfruiGluR6/7/9 were significantly higher in the larval a-m than in the antennae of adult females and males (Figure 6).
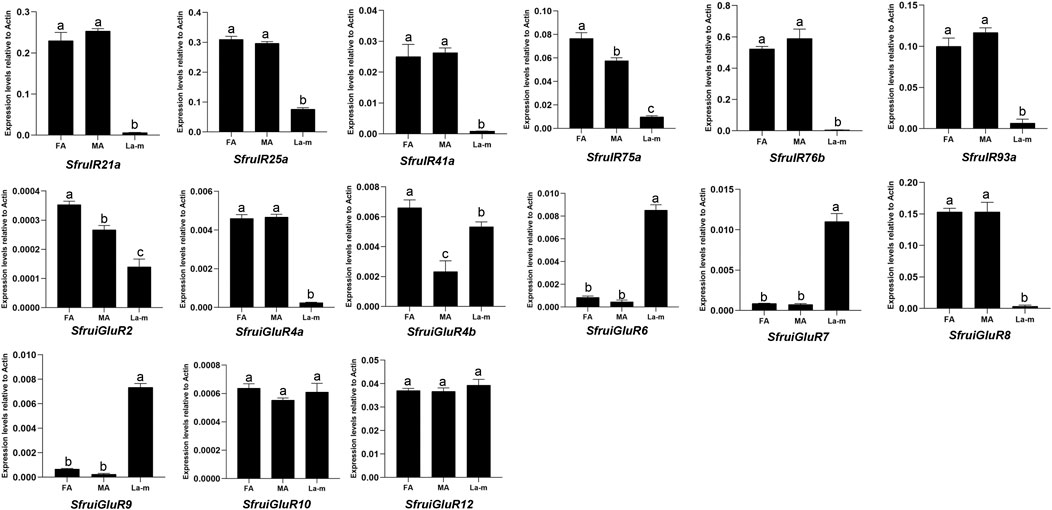
FIGURE 6. Expression profiles (as determined by RT-qPCR analysis) of candidate SfruIRs/iGluRs genes in S. frugiperda male antennae (MA), female antennae (FA), and larval antennae and maxillae (L a-m). Values are means ± SE. Different letters indicate significant differences (Tukey’s multiple comparison test.after a one-way ANOVA, p < 0.05, n = 3).
Expression profiles of chemosensory receptors in different stages of S. frugiperda larvae
To reveal expression levels of the chemosensory receptors during S. frugiperda larval development, we selected three stages, first, fourth, and sixth instars, to cover the larval developmental period. In total, 23 chemosensory receptor genes including 8 SfruORs, 4 SfruGRs, and 11 SfruIRs/iGluRs showed larval a-m expression were measured using RT-qPCR (not including the genes of which the expression in larval a-m was barely detected). According to the RT-qPCR results, 2 SfruORs (SfruORco/SfruOR42) and 1 SfruiGluR (SfruiGluR2) displayed higher expression in the first instar than in other two instars. While 3 SfruGRs (SfruGR1/3/9) and 4 SfruIR/iGluRs (SfruIR93a and SfruiGluR7/9/12) displayed higher expression in the fourth and sixth instars than in the first instar. In comparison, the expression of SfruOR15 and SfruGR2 was higher in the sixth instar than in other two instars, and the expression of SfruOR46 was higher in the first and fourth instars than in the sixth instar. Other measured genes exhibited similar expression levels among the three stages (Figure 7).
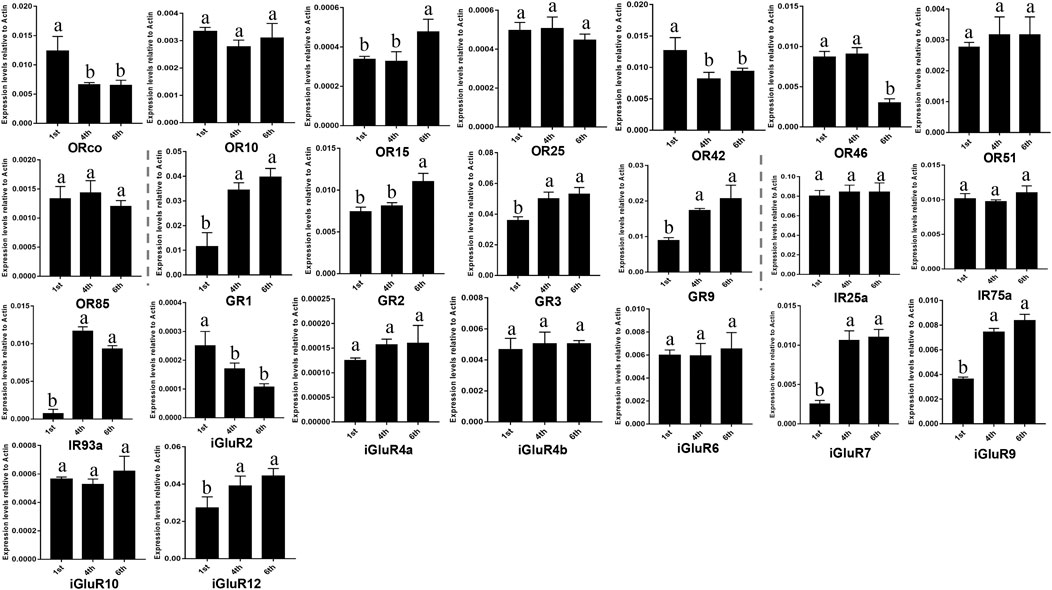
FIGURE 7. Expression profiles of chemosensory receptors in different stages of S. frugiperda larvae. RT-qPCR analyses of the genes displaying larval a-m expression were conducted in the first (1st) instar larval head, the fourth (4th) instar larval antennae and maxillae, and the sixth (6th) instar larval antennae and maxillae. Values are means ± SE. Different letters indicate significant differences (Tukey’s multiple comparison test after a one-way ANOVA, p < 0.05, n = 3).
Discussion
Despite the wide distribution of S. frugiperda and the severe damage to crops caused by its larvae, information concerning the larval chemosensory system is limited. In the current study, we focused on three groups of receptors (ORs, GRs, and IRs) because of their potential importance to the chemosensory system of S. frugiperda larvae and therefore to the development of new pest control strategies.
In this study, we analyzed the transcriptome of the S. frugiperda larval a-m by de novo sequencing. The total number of SfruORs identified here (11) is close to the number (16) of ORs found in the larval transcriptome of H. armigera (Di et al., 2017) but is much lower than the number of putative ORs reported in the larval transcriptome of S. littoralis (22) and S. litura (22) (Poivet et al., 2013; Li et al., 2021). The large difference in the numbers of ORs found in our study of S. frugiperda than in previous studies of S. littoralis and S. litura may have resulted from the sample collection methods. In the current study of S. frugiperda, the larval a-m were collected on the 2nd day in the 6th instar; in contrast, the larval a-m were collected in the 4th instar of S. littoralis (Poivet et al., 2013), and entire heads were collected from 1- to 6-day-old larvae of S. litura (Li et al., 2021). Furthermore, the assembled larval head transcriptome of S. littoralis revealed 34 ORs in the 1st instar but only 18 ORs in the 4th instar (Revadi et al., 2021).
Although we found 11 ORs in S. frugiperda larvae, 26 ORs were recently detected in the transcriptome of antennae of S. frugiperda adults (Qiu et al., 2020). This difference between the number of ORs in S. frugiperda larvae and adults is consistent with that observed for ORs in other lepidopteran species. For example, B. mori expressed 23 ORs in larvae but 35 in adults (Tanaka et al., 2009); H. armigera expressed 16 ORs in larvae but 47 in adults (Liu et al., 2012; Di et al., 2017); S. littoralis expressed 22 ORs in larvae but 47 in adults (Poivet et al., 2013); and S. litura expressed 22 ORs in larvae but 60 in adults (Li et al., 2021). These differences may be due to differences in the physiological and behavioral characteristics of larvae vs. adults regardless of species.
Consistent with the expression profile of ORco genes reported for other lepidopteran species (Di et al., 2017; de Fouchier et al., 2018), we found that the SfruORco was highly expressed in both the adult antennae and the larval a-m. This indicated the ability of olfaction in both the larvae and adults of S. frugiperda. In the study of model insect B. mori, deletion of the ORco gene using CRISPR/Cas9 system demonstrated that the homozygous mutant of adults was unable to respond to bombykol or bombykal, and larval feeding behavior assays revealed that the mutant larvae displayed defective selection for mulberry leaves and the mulberry leave volatile cis-jasmone (Liu et al., 2017). Like the OR expression profiles reported in other lepidopteran species (Di et al., 2017; de Fouchier et al., 2018; Li et al., 2021), expression of most of the annotated SfruORs in our study of S. frugiperda was greater in adult antennae (male/female) than in the larval a-m. The SfruORs (SfruOR11/12/20) that displayed adult antennae-specific expression profiles may be involved in the olfaction of S. frugiperda adults. For example, HarmOR27, an ortholog of SfruOR20, mainly expressed in the adult antennae of male and female H. armigera, was found triggering butyl salicylate when heterologously expressed in Drosophila empty neurons (Guo et al., 2021). The SfruORs (SfruOR10 and SfruOR51) that showed similar transcript levels in the larval a-m and adult antennae may have olfactory functions in both developmental stages. As an ortholog of SfruOR51, SlitOR31 showed expression in the antennae of both adult and larval S. littoralis (De Fouchier et al., 2018). Heterologous expression of this receptors in Drosophila exhibited narrow tuning to the plant volatile eugenol that commonly emitted by flowers (De Fouchier et al., 2017).
SfruOR42, an ortholog of HarmOR42 (amino acid identity: 86%), was identified in the current study. A previous study found that HarmOR42 was expressed in the antennae of H. armigera larvae and that this receptor responded strongly to the larval attractant phenylacetaldehyde in the Xenopus oocyte recording system (Di et al., 2017). The exact function of SfruOR42 (whether or not S. frugiperda larvae use it to detect phenylacetaldehyde) remains to be determined.
Because insect larvae do not mate, detection of sex pheromones by insect larvae has seldom been reported. However, research has shown that S. littoralis larvae were more attracted to a food source when it contained species-specific sex pheromone components (Poivet et al., 2012). Similar results were reported for larvae of Plutella xylostella (Zhu et al., 2016) and S. litura (Han et al., 2022). In the current study, we identified a putative pheromone receptor SfruOR11, which was clustered in the lepidopteran pheromone receptor OR11 clade. RT-qPCR analysis showed that SfruOR11 was expressed in both adult antennae and the larval a-m, with the expression level significantly higher in male antennae than in female antennae or the larval a-m. Given that the specific ligands for the OR11 clade in lepidopterans are still unknown (Yang and Wang, 2021), the role of sex pheromone perception by S. frugiperda larvae remains to be elucidated.
We identified three GRs (SfruGR1/2/3) that are homologous with B. mori or H. armigera CO2 receptors. It is well known that adult moths can use CO2 concentration to evaluate flower profitability (Thom et al., 2004). Specialized neurons that sense CO2 are predominantly enriched in the labial palps in adult moths, and three GRs, GR1/2/3, are responsible for the detection of CO2 (Xu and Anderson, 2015; Ning et al., 2016). In this study, we found that three CO2 receptor genes were abundantly expressed in the male and female adult antennae, and that SfurGR3 was also highly expressed in the larval a-m. Similar expression characteristics have been reported in H. armigera (Xu and Anderson, 2015). Because little is known about the ability of lepidopteran larvae to detect CO2, functional investigation is needed of SfruGR1/2/3 in the larval a-m of S. frugiperda. Another GR, SfruGR9, the homolog of H. armigera GR9 (HarmGR9) and B. mori GR9 (BmorGR9), was detected in the current study. HarmGR9 was previously found to have high transcription levels in H. armigera female antennae and larval foreguts, and to respond specifically to D-fructose (Jiang et al., 2015). Similarly, BmorGR9 in B. mori was identified as a fructose receptor (Sato et al., 2011). In the current study, SfruGR9 was expressed in both adults and larvae (although the expression level was higher in female antennae than in the other tissues), which is reasonable because both S. frugiperda larvae and adults can detect fructose. Further research is needed to determine the roles of SfruGR9 in adult antennae (especially female antennae) and in the larval chemosensory organs of S. frugiperda.
Insect IRs are expressed in chemosensory organs and other tissues involved in olfaction, gustation, and in the sensing of humidity and temperature (Rytz et al., 2013; Robertson, 2019; Hou et al., 2022). In the current study, four “antennal IRs” (SfruIR21a/41a/75a/93a) and two IR co-receptors (SfruIR25a/76b) were annotated in the larval a-m of S. frugiperda. RT-qPCR demonstrated that all of these annotated “antennal IRs” and co-receptors were highly expressed in adult antennae of both sexes; these results are consistent with those reported for H. armigera and Agrotis segetum (Liu et al., 2014; Hou et al., 2022). SfruIR25a and SfruIR75a were also highly expressed in that larval a-m (although at a significantly lower level than in adult antennae), indicating that these IRs are involved in the chemosensory reception in both larvae and adults of S. frugiperda. In D. melanogaster, IR25a and IR93a have been reported to be indispensable for the detection of cool temperatures by IR21a (Ni et al., 2016). The functional properties of their orthologs (SfruIR21a/25a/93a) identified in the current study remain to be investigated.
Ionotropic glutamate receptors (iGluRs) are localized on the surface of neuron synapses and are involved in the transmission of signals in nervous systems (Benton et al., 2006; Abuin et al., 2011). Three subfamilies of iGluRs, including NMDA (N-methyl-D-aspartate), kainate, and AMPA (α-amino-3- hydroxy-5-methyl-4-isoxazolepropionic acid) have been well studied in their structural features and biophysical functions (Mayer, 2011; Rytz et al., 2013). In the current study, we identified 9 iGluRs in the larval a-m of S. frugiperda. We found that some iGluRs (SfruiGluR2/4a/4b/8) were mainly expressed in adult antennae, that expression of SfruiGluR2/4b was higher in female than in male antennae, that expression of SfruiGluR6/7/9 was higher in the larval a-m than in adult antennae, and that expression of SfruiGluR10/12 was similar in adult antennae and the larval a-m. These differences in expression levels of iGluRs are probably related to their importance in different tissues/stages. Among all of the annotated SfruIRs/iGluRs, the expression level was highest for SfruiGluR7. RT-qPCR analyses also showed that SfruiGluR7 was much more enriched in the larval a-m than in adult antennae. We therefore suggest that this gene may be especially important in the transmission of neuron signals in S. frugiperda larvae which should be further experimentally validated.
Expression levels of 23 larval a-m expressed chemosensory receptor genes during larval development were further analyzed using RT-qPCR. Three larval stages (first, fourth, and sixth instars) were selected to cover the larval developmental period (early, middle, and gluttonous stage) (Dai et al., 2020; Wang et al., 2020; Xie et al., 2021). We found that the expression of 13 chemosensory receptor genes including 4 SfruORs, 4 SfruGRs, and 5 SfruIRs/iGluRs varied significantly among the three larval stages. Similar findings had been reported by other researchers. Measurements of the dynamic expression of S. litura chemosensory genes demonstrated that some of the genes showed differential expression during larval developmental stages (Li et al., 2021). Studies on the expression profiles of ORs in the larvae of S. littoralis between the first and fourth instar revealed that some of the ORs exhibited instar-biased expression (Revadi et al., 2021). Differential expression investigation of chemosensory receptor genes during larval development of S. frugiperda in this study provides a good basis to understand odor-guided behavioral traits of the larvae, and to analyze the function of chemosensory receptor genes during larval feeding (Yactayo-Chang et al., 2021).
Furthermore, we found that expression levels of 4 SfruGR genes (SfruGR1/2/3/9) were higher in the sixth instar than in the first and fourth instars. While the expression of SfruORco was higher in the first instar than in the fourth and sixth instars. Xu and Anderson (2015) reported that expression levels of CO2 GR genes (HarmGR1/2/3) from the cotton bollworm H. armigera were higher in the fifth instar than in the third instar. Transcriptomic analysis combined with RT-PCR demonstrated that the ORco gene of S. littoralis had higher expression in the heads of first instar larvae than in the fourth instar (Revadi et al., 2021). Correlations between the expression levels of these genes and the larval development stages, however, need to be confirmed in more moth species. And functional importance of such expression patterns during larval feeding is worth further investigation.
In conclusion, this work represents the first annotation and expression profile analysis of the chemosensory receptors in larval antennae and maxillae of the lepidopteran pest S. frugiperda. Our findings provide a foundation for future studies of the functions of the chemosensory receptors in S. frugiperda larvae.
Data availability statement
The datasets presented in this study can be found in online repositories. The names of the repository/repositories and accession number(s) can be found in the article/Supplementary Material.
Author contributions
Y-LS, J-FD, and C-HT conceived and designed the study. P-SJ and B-XD reared the insect and collected the biological material. P-SJ extracted the RNA. J-FD, and C-HT performed the transcriptome data analysis. Y-LS and P-SJ constructed the phylogenetic trees. Y-LS, J-FD, and C-HT performed the molecular work. Y-LS wrote the manuscript. All authors read and approved the final version of the manuscript.
Funding
This work was funded by National Key R&D Programs of China (Grant Nos. 2021YFD1400701), College Students’ Innovative Entrepreneurial Training Plan Program (Grant Nos. S202110464060), Major Special Science and Technology Project of Henan Province (Grant Nos. 201300111500), and Doctoral Scientific Research Foundation of Henan University of Science and Technology (Grant Nos.13480064).
Conflict of interest
The authors declare that the research was conducted in the absence of any commercial or financial relationships that could be construed as a potential conflict of interest.
Publisher’s note
All claims expressed in this article are solely those of the authors and do not necessarily represent those of their affiliated organizations, or those of the publisher, the editors and the reviewers. Any product that may be evaluated in this article, or claim that may be made by its manufacturer, is not guaranteed or endorsed by the publisher.
Supplementary material
The Supplementary Material for this article can be found online at: https://www.frontiersin.org/articles/10.3389/fphys.2022.970915/full#supplementary-material
References
Abuin L., Bargeton B., Ulbrich M. H., Isacoff E. Y., Kellenberger S., Benton R. (2011). Functional architecture of olfactory ionotropic glutamate receptors. Neuron 69, 44–60. doi:10.1016/j.neuron.2010.11.042
Ai M., Blais S., Park J. Y., Min S., Neubert T. A., Suh G. S. B. (2013). Ionotropic glutamate receptors IR64a and IR8a form a functional odorant receptor complex in vivo in Drosophila. J. Neurosci. 33, 10741–10749. doi:10.1523/JNEUROSCI.5419-12.2013
Ashburner M., Ball C. A., Blake J. A., Botstein D., Butler H., Cherry J. M., et al. (2000). Gene ontology: Tool for the unification of biology. Nat. Genet. 25, 25–29. doi:10.1038/75556
Benton R., Sachse S., Michnick S. W., Vosshall L. B. (2006). Atypical membrane topology and heteromeric function of Drosophila odorant receptors in vivo. PLoS Biol. 4, e20. doi:10.1371/journal.pbio.0040020
Benton R., Vannice K. S., Gomez-Diaz C., Vosshall L. B. (2009). Variant ionotropic glutamate receptors as chemosensory receptors in Drosophila. Cell. 136, 149–162. doi:10.1016/j.cell.2008.12.001
Butterwick J. A., Del Mármol J., Kim K. H., Kahlson M. A., Rogow J. A., Walz T., et al. (2018). Cryo-EM structure of the insect olfactory receptor Orco. Nature 560, 447–452. doi:10.1038/s41586-018-0420-8
Chang H. T., Liu Y., Ai D., Jiang X., Dong S. L., Wang G. R. (2017). A pheromone antagonist regulates optimal mating time in the moth Helicoverpa armigera. Curr. Biol. 27, 1610–1615.e3. doi:10.1016/j.cub.2017.04.035
Chen C., Buhl E., Xu M., Croset V., Rees J. S., Lilley K. S., et al. (2015). Drosophila ionotropic receptor 25a mediates circadian clock resetting by temperature. Nature 527, 516–520. doi:10.1038/nature16148
Clyne P. J., Warr C. G., Carlson J. R. (2000). Candidate taste receptors in Drosophila. Science 287, 1830–1834. doi:10.1126/science.287.5459.1830
Cock M. J. W., Beseh P. K., Buddie A. G., Cafá G., Crozier J. (2017). Molecular methods to detect Spodoptera frugiperda in Ghana, and implications for monitoring the spread of invasive species in developing countries. Sci. Rep. 7, 4103. doi:10.1038/s41598-017-04238-y
Croset V., Rytz R., Cummins S. F., Budd A., Brawand D., Kaessmann H., et al. (2010). Ancient protostome origin of chemosensory ionotropic glutamate receptors and the evolution of insect taste and olfaction. PLoS Genet. 6, e1001064. doi:10.1371/journal.pgen.1001064
Dahanukar A., Hallem E. A., Carlson J. R. (2005). Insect chemoreception. Curr. Opin. Neurobiol. 15, 423–430. doi:10.1016/j.conb.2005.06.001
Dai Q. X., Li Z. Y., Tian Y. J., Zhang Z. F., Wang L., Lu Y. Y., et al. (2020). Effects of different corn varieties on development and reproduction of Spodoptera frugiperda. Chin. J. Appl. Ecol. 31, 3273–3281. doi:10.13287/j.1001-9332.202010.020
de Fouchier A., Sun X., Caballero-Vidal G., Travaillard S., Jacquin-Joly E., Montagné N. (2018). Behavioral effect of plant volatiles binding to Spodoptera littoralis Larval odorant receptors. Front. Behav. Neurosci. 12, 264. doi:10.3389/fnbeh.2018.00264
de Fouchier A., Walker W. B., Montagné N., Steiner C., Binyameen M., Schlyter F., et al. (2017). Functional evolution of Lepidoptera olfactory receptors revealed by deorphanization of a moth repertoire. Nat. Commun. 8, 15709. doi:10.1038/ncomms15709
Delventhal R., Carlson J. R. (2016). Bitter taste receptors confer diverse functions to neurons. eLife 5, e11181. doi:10.7554/eLife.11181
Dethier V. G., Schoonhoven L. M. (1969). Olfactory coding by Lepidopterous larvae. Entomol. Exp. Appl. 12, 535–543. doi:10.1111/j.1570-7458.1969.tb02551.x
Di C., Ning C., Huang L. Q., Wang C. Z. (2017). Design of larval chemical attractants based on odorant response spectra of odorant receptors in the cotton bollworm. Insect biochem. Mol. Biol. 84, 48–62. doi:10.1016/j.ibmb.2017.03.007
Early R., González-Moreno P., Murphy S., Day R. (2018). Forecasting the global extent of invasion of the cereal pest Spodoptera frugiperda, the fall armyworm. NeoBiota 40, 25–50. doi:10.1101/391847
Frank D. D., Enjin A., Jouandet G. C., Zaharieva E. E., Para A., Stensmyr M. C., et al. (2017). Early integration of temperature and humidity stimuli in the Drosophila brain. Curr. Biol. 27, 2381–2388.e4. doi:10.1016/j.cub.2017.06.077
Fujii S., Yavuz A., Slone J., Jagge C., Song X., Amrein H. (2015). Drosophila sugar receptors in sweet taste perception, olfaction, and internal nutrient sensing. Curr. Biol. 25, 621–627. doi:10.1016/j.cub.2014.12.058
Ganguly A., Pang L., Duong V. K., Lee A., Schoniger H., Varady E., et al. (2017). A molecular and cellular context-dependent role for Ir76b in detection of amino acid taste. Cell. Rep. 18, 737–750. doi:10.1016/j.celrep.2016.12.071
Gao Q., Chess A. (1999). Identification of candidate Drosophila olfactory receptors from genomic DNA sequence. Genomics 60, 31–39. doi:10.1006/geno.1999.5894
Goergen G., Kumar P. L., Sankung S. B., Togola A., Tamò M., Luthe D. S. (2016). First report of outbreaks of the fall armyworm Spodoptera frugiperda (J.E. Smith) (Lepidoptera, Noctuidae), a new alien invasive pest in west and central Africa. PLoS ONE 11, e0165632. doi:10.1371/journal.pone.0165632
Gouin A., Bretaudeau A., Nam K., Gimenez S., Aury J. M., Duvic B., et al. (2017). Two genomes of highly polyphagous lepidopteran pests (Spodoptera frugiperda, Noctuidae) with different host-plant ranges. Sci. Rep. 7, 11816. doi:10.1038/s41598-017-10461-4
Guo M. B., Du L. X., Chen Q. Y., Feng Y. L., Zhang J., Zhang X. X., et al. (2021). Odorant receptors for detecting flowering plant cues are functionally conserved across moths and butterflies. Mol. Biol. Evol. 38, 1413–1427. doi:10.1093/molbev/msaa300
Han W. K., Yang Y. L., Si Y. X., Wei Z. Q., Liu S. R., Liu X. L., et al. (2022). Involvement of GOBP2 in the perception of a sex pheromone component in both larval and adult Spodoptera litura revealed using CRISPR/Cas9 mutagenesis. Insect biochem. Mol. Biol. 141, 103719. doi:10.1016/j.ibmb.2022.103719
Hansson B. S., Stensmyr M. C. (2011). Evolution of insect olfaction. Neuron 72, 698–711. doi:10.1016/j.neuron.2011.11.003
He P., Liu Y., Hull J. J., Zhang Y. N., Zhang J., Guo X. J., et al. (2022). Editorial: Insect olfactory proteins (from gene identification to functional characterization), volume II. Front. Physiol. 13, 858728. doi:10.3389/fphys.2022.858728
Hildebrand J. G., Shepherd G. M. (1997). Mechanisms of olfactory discrimination: Converging evidence for common principles across phyla. Annu. Rev. Neurosci. 20, 595–631. doi:10.1146/annurev.neuro.20.1.595
Hou X. Q., Zhang D. D., Powell D., Wang H. L., Löfstedt C., Lofstedt C. (2022). Ionotropic receptors in the turnip moth Agrotis segetum respond to repellent medium-chain fatty acids. BMC Biol. 20, 34. doi:10.1186/s12915-022-01235-0
Hussain A., Zhang M., Üçpunar H. K., Svensson T., Quillery E., Gompel N., et al. (2018). Ionotropic chemosensory receptors mediate the taste and smell of polyamines. PLoS Biol. 14, e1002454. doi:10.1371/journal.pbio.1002454
Itagaki H., Hildebrand H. (1990). Olfactory interneurons in the brain of the larval sphinx moth Manduca sexta. J. Comp. Physiol. A 167, 309–320. doi:10.1007/BF00192566
Jiang X. J., Guo H., Di C., Yu S., Zhu L., Huang L. Q., et al. (2014). Sequence similarity and functional comparisons of pheromone receptor orthologs in two closely related Helicoverpa species. Insect biochem. Mol. Biol. 48, 63–74. doi:10.1016/j.ibmb.2014.02.010
Jiang X. J., Ning C., Guo H., Jia Y. Y., Huang L. Q., Qu M. J., et al. (2015). A gustatory receptor tuned to D-fructose in antennal sensilla chaetica of Helicoverpa armigera. Insect biochem. Mol. Biol. 60, 39–46. doi:10.1016/j.ibmb.2015.03.002
Johnson J. S. (1987). Migration and the life history strategy of the fall armyworm, Spodoptera frugiperda in the western hemisphere. Int. J. Trop. Insect Sci. 8, 543–549. doi:10.1017/S1742758400022591
Jones W. D., Cayirlioglu P., Kadow I. G., Vosshall L. B. (2007). Two chemosensory receptors together mediate carbon dioxide detection in Drosophila. Nature 445, 86–90. doi:10.1038/nature05466
Kanehisa M., Goto S., Kawashima S., Okuno Y., Hattori M. (2004). The KEGG resource for deciphering the genome. Nucleic Acids Res. 32, 277–280. doi:10.1093/nar/gkh063
Knecht Z. A., Silbering A. F., Ni L., Klein M., Budelli G., Bell R., et al. (2016). Distinct combinations of variant ionotropic glutamate receptors mediate thermosensation and hygrosensation in Drosophila. eLife 5, e17879. doi:10.7554/eLife.17879
Koh T. W., He Z., Gorur-Shandilya S., Menuz K., Larter N. K., Stewart S., et al. (2014). The Drosophila IR20a clade of ionotropic receptors are candidate taste and pheromone receptors. Neuron 83, 850–865. doi:10.1016/j.neuron.2014.07.012
Leal W. S. (2013). Odorant reception in insects: Roles of receptors, binding proteins, and degrading enzymes. Annu. Rev. Entomol. 58, 373–391. doi:10.1146/annurev-ento-120811-153635
Li L. L., Xu J. W., Yao W. C., Yang H. H., Dewer Y., Zhang F., et al. (2021). Chemosensory genes in the head of Spodoptera litura larvae. Bull. Entomol. Res. 111, 454–463. doi:10.1017/S0007485321000109
Liu N. Y., Xu W., Papanicolaou A., Dong S. L., Anderson A. (2014). Identification and characterization of three chemosensory receptor families in the cotton bollworm Helicoverpa armigera. BMC Genomics 15, 597. doi:10.1186/1471-2164-15-597
Liu Q., Liu W., Zeng B. S., Wang G. R., Hao D. J., Huang Y. P. (2017). Deletion of the Bombyx mori odorant receptor co-receptor (BmOrco) impairs olfactory sensitivity in silkworms. Insect biochem. Mol. Biol. 86, 58–67. doi:10.1016/j.ibmb.2017.05.007
Liu X. L., Zhang J., Qi Y., Wang C. Z., Dong S. L., Knaden M., et al. (2020). The molecular basis of host selection in a crucifer-specialized moth. Curr. Biol. 30, 4476–4482.e5. doi:10.1016/j.cub.2020.08.047
Liu Y., Gu S. H., Zhang Y. J., Guo Y. Y., Wang G. R. (2012). Candidate olfaction genes identified within the Helicoverpa armigera antennal transcriptome. PLoS One 7, e48260. doi:10.1371/journal.pone.0048260
Liu Y., Liu C. C., Lin K. J., Wang G. R. (2013). Functional specificity of sex pheromone receptors in the cotton bollworm Helicoverpa armigera. PLoS One 8, e62094. doi:10.1371/journal.pone.0062094
Mayer M. L. (2011). Emerging models of glutamate receptor ion channel structure and function. Structure 19, 1370–1380. doi:10.1016/j.str.2011.08.009
Meagher R. L., Nagoshi R. N. (2012). Differential feeding of fall armyworm Lepidoptera (Lepidoptera: Noctuidae) host strains on meridic and natural diets. Ann. Entomol. Soc. Am. 105, 462–470. doi:10.1603/AN11158
Miyamoto T., Slone J., Song X., Amrein H. (2012). A fructose receptor functions as a nutrient sensor in the Drosophila brain. Cell. 151, 1113–1125. doi:10.1016/j.cell.2012.10.024
Montagné N., Chertemps T., Brigaud I., François A., François M. C., De Fouchier A., et al. (2012). Functional characterization of a sex pheromone receptor in the pest moth Spodoptera littoralis by heterologous expression in Drosophila. Eur. J. Neurosci. 36, 2588–2596. doi:10.1111/j.1460-9568.2012.08183.x
Nakagawa T., Sakurai T., Nishioka T., Touhara K. (2005). Insect sex-pheromone signals mediated by specific combinations of olfactory receptors. Science 307, 1638–1642. doi:10.1126/science.1106267
Ni L., Klein M., Svec K. V., Budelli G., Chang E. C., Ferrer A. J., et al. (2016). The ionotropic receptors IR21a and IR25a mediate cool sensing in Drosophila. eLife 5, e13254. doi:10.7554/eLife.13254
Ning C., Yang K., Xu M., Huang L. Q., Wang C. Z. (2016). Functional validation of the carbon dioxide receptor in labial palps of Helicoverpa armigera moths. Insect biochem. Mol. Biol. 73, 12–19. doi:10.1016/j.ibmb.2016.04.002
Poivet E., Gallot A., Montagné N., Glaser N., Legeai F., Jacquin-Joly E. (2013). A comparison of the olfactory gene repertoires of adults and larvae in the noctuid moth Spodoptera littoralis. PLoS One 8, e60263. doi:10.1371/journal.pone.0060263
Poivet E., Rharrabe K., Monsempes C., Glaser N., Rochat D., Renou M., et al. (2012). The use of the sex pheromone as an evolutionary solution to food source selection in caterpillars. Nat. Commun. 3, 1047. doi:10.1038/ncomms2050
Qiu L., He L., Tan X., Zhang Z., Wang Y., Li X., et al. (2020). Identification and phylogenetics of Spodoptera frugiperda chemosensory proteins based on antennal transcriptome data. Comp. Biochem. Physiol. Part D. Genomics Proteomics 34, 100680. doi:10.1016/j.cbd.2020.100680
Revadi S. V., Giannuzzi V. A., Rossi V., Hunger G. M., Conchou L., Rondoni G., et al. (2021). Stage-specific expression of an odorant receptor underlies olfactory behavioral plasticity in Spodoptera littoralis larvae. BMC Biol. 19, 231. doi:10.1186/s12915-021-01159-1
Robertson H. M. (2019). Molecular evolution of the major arthropod chemoreceptor gene families. Annu. Rev. Entomol. 64, 227–242. doi:10.1146/annurev-ento-020117-043322
Robertson H. M., Warr C. G., Carlson J. R. (2003). Molecular evolution of the insect chemoreceptor gene superfamily in Drosophila melanogaster. Proc. Natl. Acad. Sci. U. S. A. 100, 14537–14542. doi:10.1073/pnas.2335847100
Rytz R., Croset V., Benton R. (2013). Ionotropic receptors (IRs): Chemosensory ionotropic glutamate receptors in Drosophila and beyond. Insect biochem. Mol. Biol. 43, 888–897. doi:10.1016/j.ibmb.2013.02.007
Sakurai T., Nakagawa T., Mitsuno H., Mori H., Endo Y., Tanoue S., et al. (2004). Identification and functional characterization of a sex pheromone receptor in the silkmoth Bombyx mori. Proc. Natl. Acad. Sci. U. S. A. 101, 16653–16658. doi:10.1073/pnas.0407596101
Sato K., Pellegrino M., Nakagawa T., Vosshall L. B., Touhara K. (2008). Insect olfactory receptors are heteromeric ligand-gated ion channels. Nature 452, 1002–1006. doi:10.1038/nature06850
Sato K., Tanaka K., Touhara K. (2011). Sugar-regulated cation channel formed by an insect gustatory receptor. Proc. Natl. Acad. Sci. U. S. A. 108, 11680–11685. doi:10.1073/pnas.1019622108
Schmittgen T. D., Livak K. J. (2008). Analyzing real-time PCR data by the comparative CT method. Nat. Protoc. 3, 1101–1108. doi:10.1038/nprot.2008.73
Scott K., Brady R., Cravchik A., Morozov P., Rzhetsky A., Zuker C., et al. (2001). A chemosensory gene family encoding candidate gustatory and olfactory receptor in Drosophila. Cell. 104, 661–673. doi:10.1016/s0092-8674(01)00263-x
Silbering A. F., Rytz R., Grosjean Y., Abuin L., Ramdya P., Jeferis G. S. X., et al. (2011). Complementary function and integrated wiring of the evolutionarily distinct Drosophila olfactory subsystems. J. Neurosci. 31, 13357–13375. doi:10.1523/JNEUROSCI.2360-11.2011
Sparks A. N. (1979). A review of the biology of the fall armyworm. Fla. Entomol. 62, 82–87. doi:10.2307/3494083
Stengl M. (2010). Pheromone transduction in moths. Front. Cell. Neurosci. 4, 133. doi:10.3389/fncel.2010.00133
Sun Y. L., Dong J. F., Gu N., Wang S. L. (2020). Identification of candidate chemosensory receptors in the antennae of the variegated cutworm, Peridroma saucia Hübner, based on a transcriptomic analysis. Front. Physiol. 11, 39. doi:10.3389/fphys.2020.00039
Sun Y. L., Dong J. F., Song Y. Q., Wang S. L. (2021). GOBP1 from the variegated cutworm Peridroma saucia (Hübner) (Lepidoptera: Noctuidae) displays high binding affinities to the behavioral attractant (Z)-3-hexenyl acetate. Insects 12, 939. doi:10.3390/insects12100939
Tanaka K., Uda Y., Ono Y., Nakagawa T., Suwa M., Yamaoka R., et al. (2009). Highly selective tuning of a silkworm olfactory receptor to a key mulberry leaf volatile. Curr. Biol. 19, 881–890. doi:10.1016/j.cub.2009.04.035
Thom C., Guerenstein P. G., Mechaber W. L., Hildebrand J. G. (2004). Floral CO2 reveals flower profitability to moths. J. Chem. Ecol. 30, 1285–1288. doi:10.1023/B:JOEC.0000030298.77377.7d
Wang W., He P., Zhang Y., Liu T., Jing X., Zhang S. (2020). The population growth of Spodoptera frugiperda on six cash crop species and implications for its occurrence and damage potential in China. Insects 11, 639. doi:10.3390/insects11090639
Wicher D., Schäfer R., Bauernfeind R., Stensmyr M. C., Heller R., Heinemann S. H., et al. (2008). Drosophila odorant receptors are both ligand-gated and cyclic-nucleotide-activated cation channels. Nature 452, 1007–1011. doi:10.1038/nature06861
Wilson R. I. (2013). Early olfactory processing in Drosophila: Mechanisms and principles. Annu. Rev. Neurosci. 36, 217–241. doi:10.1146/annurev-neuro-062111-150533
Wu Q. L., Jiang Y. Y., Wu K. M. (2019). Analysis of migration routes of the fall armyworm Spodoptera frugiperda (J.E. Smith) from Myanmar to China. Plant Prot. 45 (1–6), 18. doi:10.16688/j.zwbh.2019047
Xie C., Mao X., Huang J., Ding Y., Wu J., Dong S., et al. (2011). Kobas 2.0: A web server for annotation and identification of enriched pathways and diseases. Nucleic Acids Res. 39, 316–322. doi:10.1093/nar/gkr483
Xie W., Zhi J., Ye J., Zhou Y., Li C., Liang Y., et al. (2021). Age-stage, two-sex life table analysis of Spodoptera frugiperda (JE Smith) (Lepidoptera: Noctuidae) reared on maize and kidney bean. Chem. Biol. Technol. Agric. 8, 44. doi:10.1186/s40538-021-00241-8
Xu W., Anderson A. (2015). Carbon dioxide receptor genes in cotton bollworm Helicoverpa armigera. Naturwissenschaften 102, 11. doi:10.1007/s00114-015-1260-0
Xu W., Papanicolaou A., Liu N. Y., Dong S. L., Anderson A. (2015). Chemosensory receptor genes in the oriental tobacco budworm Helicoverpa assulta. Insect Mol. Biol. 24, 253–263. doi:10.1111/imb.12153
Xu W., Zhang H. J., Anderson A. (2012). A sugar gustatory receptor identified from the foregut of cotton bollworm Helicoverpa armigera. J. Chem. Ecol. 38, 1513–1520. doi:10.1007/s10886-012-0221-8
Yactayo-Chang J. P., Mendoza J., Willms S., Rering C. C., Beck J. J., Block A. K. (2021). Zea mays volatiles that influence oviposition and feeding behaviors of Spodoptera frugiperda. J. Chem. Ecol. 47, 799–809. doi:10.1007/s10886-021-01302-w
Yang K., Wang C. Z. (2021). Review of pheromone receptors in heliothine species: Expression, function, and evolution. Entomol. Exp. Appl. 169, 156–171. doi:10.1111/eea.12982
Zhang Z. J., Zhang S. S., Niu B. L., Ji D. F., Liu X. J., Li M. W., et al. (2019). A determining factor for insect feeding preference in the silkworm, Bombyx mori. PLoS Biol. 17, e3000162. doi:10.1371/journal.pbio.3000162
Keywords: larval transcriptome, odorant receptor, gustatory receptor, ionotropic receptor, real-time quantitative-PCR, Spodoptera frugiperda
Citation: Sun Y-L, Jiang P-S, Dong B-X, Tian C-H and Dong J-F (2022) Candidate chemosensory receptors in the antennae and maxillae of Spodoptera frugiperda (J. E. Smith) larvae. Front. Physiol. 13:970915. doi: 10.3389/fphys.2022.970915
Received: 16 June 2022; Accepted: 11 August 2022;
Published: 15 September 2022.
Edited by:
Ya-Nan Zhang, Huaibei Normal University, ChinaReviewed by:
Peng-Fei Lu, Beijing Forestry University, ChinaQian Ju, Shandong Peanut Research Institute (CAAS), China
Copyright © 2022 Sun, Jiang, Dong, Tian and Dong. This is an open-access article distributed under the terms of the Creative Commons Attribution License (CC BY). The use, distribution or reproduction in other forums is permitted, provided the original author(s) and the copyright owner(s) are credited and that the original publication in this journal is cited, in accordance with accepted academic practice. No use, distribution or reproduction is permitted which does not comply with these terms.
*Correspondence: Cai-Hong Tian, Y2FpaG9uZ3RpYW5AaG5hZ3JpLm9yZy5jbg==; Jun-Feng Dong, anVuZmVuZ2RvbmdAaGF1c3QuZWR1LmNu
†These authors have contributed equally to this work