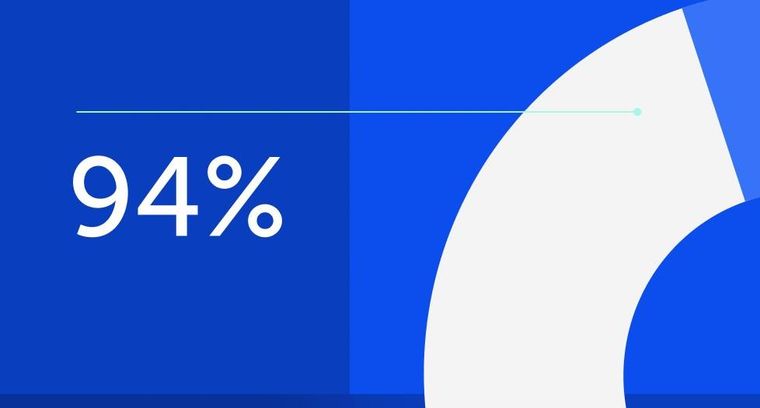
94% of researchers rate our articles as excellent or good
Learn more about the work of our research integrity team to safeguard the quality of each article we publish.
Find out more
ORIGINAL RESEARCH article
Front. Physiol., 14 September 2022
Sec. Invertebrate Physiology
Volume 13 - 2022 | https://doi.org/10.3389/fphys.2022.968047
This article is part of the Research TopicMethods and Applications in Invertebrate PhysiologyView all 12 articles
The inclusion of cephalopods in the legislation related to the use of animals for experimental purposes has been based on the precautionary principle that these animals have the capacity to experience pain, suffering, distress, and lasting harm. Recent studies have expanded this view and supported it. Handling cephalopod mollusks in research is challenging and whenever more invasive procedures are required, sedation and/or anesthesia becomes necessary. Therefore, finding adequate, safe, and effective anesthetics appears mandatory. Several substances have been considered in sedating cephalopods, in some instances applying those utilized for fish. However, species-specific variability requires more detailed studies. Despite long-lasting experience being linked to classic studies on squid giant axons, evidence of action on putative anesthetic substances is scarce for Loligo vulgaris and particularly for their embryos. The aim of the current study was to evaluate effects elicited by immersion of squid embryos in anesthetic solutions and examine whether these forms display a similar reaction to anesthetics as adults do. Different concentrations of ethanol (EtOH; 2, 2.5, and 3%) and magnesium chloride (MgCl2; 1, 1.5, and 1.8%) were tested by adopting a set of indicators aimed at exploring the physiological responses of squid embryos. Forty-two embryos of the common squid Loligo vulgaris (stages 27–28) were assigned to three conditions (EtOH, MgCl2, and controls) and video recorded for 15 min (5 min before, 5 min during, and 5 min after immersion in the anesthetic solutions). In each group, the heart rate, respiratory rate, buoyancy, chromatophore activity, and tentacles/arms responses were assessed to evaluate the embryos' vitality and responsiveness to stimulation. Both substances provoked a decrease in heart and respiratory rates and inhibited buoyancy, chromatophores, and tentacles/arms responses; no adverse effects were observed. EtOH had a faster onset of action and faster recovery than MgCl2, being potentially more adequate as an anesthetic for shorter procedures. Even though MgCl2 caused a longer muscle relaxation, the reversibility was not confirmed for the 1.8% concentration; however, lower concentrations triggered similar results as the ones obtained with the highest EtOH concentrations. We have shown that the late developmental stages of Loligo vulgaris embryos could represent a good model to evaluate anesthetics for cephalopods since they can display similar reactions to anesthetics as adults animals do.
In the last decade, cephalopod mollusks have been included in European Union regulation for the use of live animals by transposition into the national legislation of the Directive 2010/63/EU “on the protection of animals used for scientific purposes.” In Switzerland, the Animal Protection Ordinance (Schweizerische-Tierschutzverordnung, 2018) includes cephalopods in Chapter 1 (General Provisions—Aims) and Chapter 6 (Experiments on animals) to be protected by law since 2018. In particular, “Chapter 1” defines the categories of animals that will be protected by the ordinance; “Chapter 6” deals with animal experimentation and defines three types of cephalopods according to their sizes (small, medium, and large), no species-specific recommendation has been provided. The inclusion was based on the precautionary principle (Birch, 2017, 2021) that these animals possess the capacity to experience pain, suffering, distress, and lasting harm, further supported by some more recent findings (EFSA Panel, 2005; Crook et al., 2011; Crook and Walters, 2011; Andrews et al., 2013; Crook et al., 2013; Crook, 2021). Over the last years, an increasing number of studies have been focused on various aspects of the biology, physiology, and ecology of cephalopods. This induced an expansion of the number of species studied and also an increase in the number of studies. Therefore, an increase in attention toward the welfare of these animals is mostly required by the scientific community as well as fisheries. It is remarkable to mention that in the EU Member States, the number of cephalopods used in research in 2018 accounted for over four thousand animals according to (EU, 2019).
To perform animal handling such as for hemolymph withdrawal (Jozet-Alves et al., 2008; Locatello et al., 2013), surgeries such as limb amputation for biomechanical studies, pain or nerve regeneration studies (Imperadore et al., 2019b; Howard et al., 2019; Nesher et al., 2019), or tissue sampling (Mackie, 2008; Ponte and Fiorito, 2015; Zatylny-Gaudin et al., 2016; Baldascino et al., 2017; Imperadore et al., 2019a), the animal should be anesthetized. In mammals, the application of sedation protocols must ensure the “AALLR,” meaning analgesia, amnesia, loss of consciousness, loss of control reflexes, and muscle relaxation (Katzung et al., 2021). In cephalopods and, more particularly, in the common squid Loligo vulgaris, other criteria are needed to define satisfactory anesthesia, since their physiological characteristics are unique and cannot be easily compared to those of mammals under similar circumstances (Sen and Tanrikul, 2009). Previous work of various laboratories have urged the use of standardized protocols with specific criteria to evaluate satisfactory anesthesia in cephalopods: ventilation rate, chromatophore coloration change, loss of sucker adhesiveness, loss of voluntary or provoked movement, and unresponsiveness to noxious stimuli (Pagano et al., 2011; Gleadall, 2013; Butler-Struben et al., 2018). On the other hand, the major criteria to be observed during sedation on the basis of those used in other aquatic animals (McFarland and Klontz, 1969) include breathing depression, pupil constriction due to flash of light, reaction to pinching eye skin, flabbiness of arms, and the lack of reaction to stimulus. But are the same parameters used for adult animals also comparable to those used for embryos? Do cephalopod embryos display similar reactions as their adults do during anesthesia?
To date, ethanol (EtOH) and magnesium chloride (MgCl2) have been the most used substances to anesthetize cephalopods (Messenger et al., 1985; Butler-Struben et al., 2018; Abbo et al., 2021). Indeed, according to a recent review, out of 48 studies in which procedures for “anesthetizing” cephalopods were mentioned, 17 reported MgCl2, 23 EtOH in seawater, 3 a combination of both, and only 5 a different agent (Fiorito et al., 2015). The wide acceptance of EtOH and MgCl2 is linked to the fact that these substances are easy to use, not reported to be toxic for the user, and do not require special permits for purchase (depending on the country). Furthermore, they are cheap and considered more “eco-friendly” than other substances that have been suggested to be utilized with cephalopods (e.g., isoflurane; Polese et al., 2014) where special equipment is needed, and particularly, attention to disposal is required (Kumar et al., 2013; Varughese and Ahmed, 2021).
Detailed information about the physiological effects of EtOH and MgCl2 in cephalopods remains scarce, especially for Loligo vulgaris and, more particularly, for embryos at their latest stages. Most of the recent studies on Loligo spp. embryos are either of ecological interest or related to development (Burbach et al., 2014; Rosa et al., 2014; Crawford et al., 2020). Therefore, it is important to not only establish anesthesia protocols but also assess whether the same indicators used for adults may be applied to embryos and determine if embryos respond in the same way to the anesthetics as adults do.
Late-stage embryos provide an interesting life stage to explore, considering that their nervous system is well developed and their capacity to learn also appears weeks before hatching as shown for Sepia embryos (Darmaillacq et al., 2006; Lee et al., 2020). Another important fact is that embryos can be available in reasonable numbers.
Here, we utilized L. vulgaris embryos to evaluate responses to immersion in EtOH and MgCl2 solutions and assess the adequacy of these substances as anesthetics. In our aims, we 1) provide baseline heart rate and respiratory rate data for embryos; 2) describe changes in the heart and respiratory rates, buoyancy, chromatophore activity, and tentacle/arms responses induced by immersion in EtOH (2, 2.5, and 3% in seawater) and MgCl2 (1, 1.5, 1.8% in seawater); 3) compare the responses to anesthetic solutions of squid embryos with the adult data from other studies; and 4) assess the reversibility of anesthesia on the embryos. To the best of our knowledge, our study provides a first baseline and reference for physiological parameters of respiration and heart rate of late-stage L. vulgaris embryos.
Eggs from the species Loligo vulgaris were collected during a sampling project of the European Marine Biological Resource Center (EMBRC) France at the Bay of Morlaix on the 22 January 2021. The eggs which are normally attached to a substrate were found in a rocky sandy habitat. The eggs were transported by a commercial courier in 30-L plastic bags with one-thirds seawater and two-thirds oxygen following the procedure by Fuentes et al. (2005). The duration of the transport was 24 h at a temperature of 14°C, and the density was three branches per bag (i.e., less than 3,000 embryos/bag).
Upon arrival in the laboratory, the eggs were incubated in a closed artificial seawater system [Red Sea REEFER 170 Deluxe Aquarium (Black)]. The tank contained 130 L of artificial seawater at a temperature of 14 ± 0.5°C (salinity range: 34–36 ppt; pH range: 7.99–8.13). A 12-h light/dark cycle was set with a crepuscular and a dawn light regime, and Maxspect éclairage LED (RSX 100W) was utilized. The eggs were suspended in bundles (see Figure 1A) in a plastic structure made with PVC. Each bundle comprised between 15 and 30 arms. Each arm hosted between 100 and 150 embryos. Taking into account that the oxygen consumption of one egg mass of octopus is twice the consumption of an adult of an average weight size (Parra et al., 2000) and to provide the best care to warrant adequate care, squid eggs' oxygenation was further facilitated by adding two additional bubblers with air diffusers (Tetra APS 100 and Tetratec AS45).
FIGURE 1. (A) Eggs of Loligo vulgaris suspended in bundles; each bundle contains between 15 and 30 arms. Each arm contains between 100 and 150 embryos. (B) Embryo stages 27–28; ys: external yolk sac, a: arms, ey: eyes, chr: chromatophores, fu: funnel, ma: mantel, is: ink sac. (C) Experiment timeline.
Each procedure was conducted by the same person and under the same conditions. The observations of the embryos were carried out using a stereoscope (Leica Microsystems; Leica Plan APO 1.0×, 10450028; CLS100 LED).
We utilized L. vulgaris embryos at developmental stages 27–28 that were identified following the procedure by Arnold et al. (1974) (Figure 1B). At this stage, the yolk matches the length of the arms or the head; the latter representing one-third of the mantle length. Hatching could occur if stimulated artificially. At this stage, the ink sac is pigmented.
The eggs were placed in a Petri dish containing artificial seawater. Salinity and temperature were kept constant at 34 ppt and 14°C, respectively. The eggs were dissected carefully with a forceps (F. S. T, no. 11254-20), and the hatchlings were placed in a three-compartment culture glassware containing artificial seawater (salinity: 34 ppt; temperature: 14°C; formulation of the artificial seawater was 4 kg of artificial sea salt per 100 L of deionized water). The individuals were chosen randomly from three different egg branches; no sex differentiation was performed. The egg cases were dissected to remove the embryos, and once the embryos were out of their cases, no further development was observed.
Samples were randomly attributed to three groups. The first group (N = 18 embryos; EtOH group) served to test three different EtOH concentrations; the second group (N = 18, MgCl2 group) was used to test three different concentrations of MgCl2; and the third group represented the control. Each embryo was tested only for one concentration. Anesthetic solutions were made in artificial seawater, and we did not determine any potential difference in the osmotic pressure of the solutions utilized in the different treatments.
We identified three phases (5 min each): the control phase (t0), the anesthesia phase (t1), and the recovery phase (t2; Figure 1C). According to the Guidelines for the Care and Welfare of Cephalopods in Research, different times were proposed for anesthesia purposes for different species, but no references exist for Loligo and specifically for embryos (Fiorito et al., 2015). The averages suggested were between 8 and 19 min while using a single substance and according to the body size. In addition, the findings of Marking and Meyer (1985) regarding aquatic animals propose 3 min for sedation and 5 min for recovery. Therefore, we decided to use 5 min for each procedure. During the first 5 minutes (control phase; t0), the health status of the embryos was assessed. Then, artificial seawater was removed by a pipette and immediately replaced with the anesthetic solutions (anesthesia phase; t1). Afterward, the anesthetic was changed back to fresh artificial seawater (recovery phase, t2). During the three phases, the heart and respiratory rates were recorded, while the remaining parameters were evaluated only during t1 and t2. We recorded
- heart rate “hr”;
- respiratory rate “rr”;
- time until loss of buoyancy “TL B” and time to recover buoyancy “TR B″;
- time until loss of chromatophores activity “TL CR” and time to recover chromatophores activity “TR CR”;
- time until loss of tentacles/arms response “TL T/A R” and time to recover tentacles/arms response “TR T/A R″; and
- typical adverse response pattern “TARP” (as defined by Gleadall, 2013).
Anesthetic concentrations suggested for adult cephalopods ranged between 1% and 3% for EtOH and between 1.5% and 3.75% for MgCl2 depending on the species (reviewed in Fiorito et al., 2015). As no specific reference has been identified for L. vulgaris, we decided to investigate the effects of 2%, 2.5%, and 3% EtOH, as well as of 1%, 1.5%, and 1.8% MgCl2 (dissolved in 34 ppm artificial seawater). We did not assess the weight of the embryos. The whole procedure was video recorded, and the sequences were used to extract parameters.
Like any other cephalopod, Loligo vulgaris possess three hearts, the systemic heart and two branchial hearts. Since the beating of three hearts is synchronized, the heart rate of only one heart was counted (typically the right branchial heart). During t0, the heart rate was recorded (as beats/minute) during minutes 1, 3, and 5, then a mean of the three values was calculated. During t1, the heart rate was recorded at minutes 6, 8, and 10, and a mean was then calculated. Finally, during t2, the heart rate was assessed at minutes 11, 13, and 15, and the mean was calculated.
Cephalopod respiration is a complex movement of the mantle, increases or decreases its size, the first one corresponding to an inspiration (Figures 3A,B) and the increase of pressure in the mantle (Figure 3B) triggering water expulsion through the funnel (expiration) (Bone et al., 1994). During t0, the contractions of the mantle combined with the funnel movements were counted during minutes 1, 3, and 5, then the mean of the three values was calculated. Similarly, during t1, respirations were counted during minutes 6, 8, and 10, and the mean value was calculated. During t2, respirations were counted at minutes 11, 13, and 15, and the mean was calculated.
Buoyancy is the capacity of the embryo to swim in a physiological manner and, if put in an unphysiological position, to return to a physiological one. Buoyancy should be present as proof of good health (Sherrill et al., 2000; Moltschaniwskyj et al., 2007) and thus a core criterion for embryos inclusion in the study. During t0, buoyancy was verified and defined as present or absent. During t1, the time until loss of buoyancy was measured in seconds once the embryo went from a present to an absent state. During t2, embryos were stimulated every 10 s by a gentle touch to the mantle, head, tentacles, and arms, and the time to recovery of buoyancy was recorded.
Chromatophores are pigmented sacs connected to radial muscles, and each contraction and relaxation of these muscles trigger the pigmented sac to increase or decrease its size, respectively (Mathger and Hanlon, 2007). During t0, the chromatophores were observed, and three states were defined: a relaxed state, half-contracted state, and a contracted state (Figure 5A–C). During t1, the time until loss of chromatophore activity was defined as the time from immersion in the anesthetic bath to loss of chromatophore activity or until a relaxed state was observed and maintained. During t2, the time to recovery of the chromatophore activity was defined as the time from immersion in an anesthetic-free solution to the return of chromatophore pattern changes/change from a relaxed to contracted state.
In cephalopods, such as octopus, a measure of the state of anesthesia is sucker adhesiveness (Sen and Tanrikul, 2009; Pagano et al., 2011). However, for smaller cephalopods, sucker adhesiveness is difficult to evaluate. Moreover, this is particularly difficult to assess in late-stage L. vulgaris embryos. Therefore, as an alternative proxy for the responsiveness of the embryo, we assessed tentacles/arms response following a gentle pinch. During t0, the tentacles/arms response was classified as absent or present. During t1, the time until loss of tentacles/arms response or the absence of it was measured. In t2, the time to recovery of tentacles/arms response or its presence was recorded.
The typical adverse response pattern (TARP) corresponds to an excitatory phase of immersion in the anesthetic solution that in some circumstances may be particularly marked, including inking, escape reactions, increased or erratic ventilation, rapid switch in coloration or of the chromatophore tone, rapid jetting movements, convulsions, and defecation (Gleadall, 2013). Any observed adverse reactions were recorded during induction and recovery.
Video recordings of L. vulgaris embryos at stages 27–28 (Figure 1B) under different treatments were obtained during experiments, and parameters were noted from video playback. Each video consisted of a 5-min control phase (t0), a 5-min anesthesia phase (t1), and a 5-min recovery phase (t3), as mentioned above.
Statistical analyses were performed using standard statistical functions in jamovi (https://www.jamovi.org/). For all experiments where two conditions are directly compared, we used a t-test (buoyancy, chromatophores activity, and tentacles response), while for the experiments with multiple conditions, we used ANOVA with a Tukey post hoc test (heart and respiratory rates). A paired samples t-test was used to determine if there was a significant difference between the means of the embryos anesthetized with EtOH and those anesthetized with MgCl2, for the following parameters: buoyancy, chromatophores activity, and tentacles response. The heart and respiratory rates were assessed using repeated measures ANOVA within factors; three conditions and three repeated measures with a Tukey post hoc test. All data are presented as means ± standard error of the mean (SE).
Ethical review and approval were not required for this study because only embryos were used, which do not fall under the Swiss Animal Protection Ordinance. However, we followed the principles stated in the Guidelines for the Care and Welfare of Cephalopods in Research (Fiorito et al., 2015). After the experiment, the embryos were humanely killed by an increased dose of EtOH every 5 min, starting with 1.5%, then 2.5% until a final concentration of 3.75% was reached, and death was confirmed by mechanical brain/head destruction.
During the experiments, we did not observe mortality at short term, induced from treatment in L. vulgaris embryos.
During the control/baseline phase (t0), the heart rate was comparable in all experimental groups: mean ± SE, 79.04 ± 2.92 beats/minute (EtOH group) and 72.07 ± 5.06 beats/minute (MgCl2 group; Figure 2 A and B). It is noteworthy to report that we recorded the lowest observed rate at t0 of 67.67 beats/minute (±3.86) in animals assigned to the 1.8% MgCl2 group, and the highest values noted reached 82.67 beats/minute (±5.83; 2% EtOH group; see Table 1).
FIGURE 2. (A). Heart rate (beats/minute) before, during, and after anesthesia for the three concentrations of EtOH (3%, 2.5%, and 2%), as well as the control group. The y-axis represents the heart rate in beats per minute, the x-axis displays the three phases of the experiment each lasting 5 min: T0, control phase; T1, sedation phase; and T2, recovery phase. (B) Heart rate (beats/minute) before, during, and after anesthesia for the three concentrations of MgCl2 (1.8%, 1.5%, and 1%), as well as the control group. The y-axis represents the heart rate in beats per minute, the x-axis displays the three phases of the experiment, each lasting 5 min: T0, control phase; T1, sedation phase; and T2, recovery phase.
TABLE 1. Heart rate (beats/minute) of L. vulgaris embryos exposed to different EtOH and MgCl2 concentrations and the controls (no anesthetic solution). Measures are summarized (mean, SE, and 95% confidence interval) at the three different phases: baseline, t0; anesthesia, t1; and recovery, t2.
During the anesthesia phase (t1), the lowest heart rate was reached under 1.8% MgCl2 at 30.83 beats/minute (±3.61), followed by the rate noted under 1.5% MgCl2 (37.83 beats/minute) and 1% MgCl2 (50.67 beats/minute). The lowest heart rate for the EtOH group was triggered by the 3% EtOH concentration with 50.00 beats/minute (±4.84) followed by the 2.5% EtOH group with 63.17 beats/minute, and finally the 2% EtOH group recorded the highest heart rate for both groups with 65.17 beats/minute (Figure 2 A, B, and Tables 1).
During the recovery phase (t2), animals returned to values comparable to the baseline with the highest heart rates observed for the EtOH group with 79.67 beats/minute (±6.88), 72.33 beats/minute, and 72.17 beats/minute for the 3%, 2%, and 2.5% concentrations, respectively. For the MgCl2 group, the highest values of the heart rate were observed in the 1.5% concentration condition (65.17 ± 4.65 beats/minute), followed by the 1% concentration condition with 59.17 beats/minute and the 1.8% concentration condition with 33.50 beats/minute (see Table 1).
Overall, no significant differences emerged among the groups (p = 1, 3% EtOH and 2% EtOH; p = 0.999 for the 2.5% EtOH group). By comparing the heart rates between t0 and t1, significant differences resulted (3% EtOH and 2.5% EtOH, p < 0.001 and p = 0.003, respectively), confirming the heart rate depression during anesthesia. However, no variations existed between t0 and t1 for the 2% EtOH group, confirming that the embryos were too lightly anesthetized, as were those in the control group with p = 0.113 and p = 1, respectively. During the recovery phase, significant variations were found only for the 3% EtOH group (p < 0.001) (see Supplementary Table S1).
Overall, no significant differences were observed during t0 (baseline) between different treatments (p > 0.05). However, significant differences existed when comparing before anesthesia (t0) and during anesthesia (t1; p < 0.001 for 1.8% and 1.5%, p = 0.002 for 1% MgCl2) except for the control group (p = 1), thus confirming the heart rate depression induced by anesthesia. When comparing the heart rate between the anesthesia (t1) and recovery (t2) phases, no significant differences were observed for animals exposed to 1.8% MgCl2 since the animals never recovered and the 1% appeared to be only light sedated. p < 0.001 for the 1.5% MgCl2 proved to be the more satisfying concentration (Supplementary Table S1).
Table 2 summarizes the respiratory rate observed in L. vulgaris embryos in different phases and conditions of the experiment. During the t0 phase, the average respiratory rate ranged between 56.29 ± 5.50 and 63.40 ± 6.70 mantle contractions per minute (EtOH and MgCl2 groups, respectively (Figure 3C), with a peak of 68.83 ± 3.86 mantle contractions per minute (mcm), and the lowest values recorded were 51.33 mantle contractions per minute. During anesthesia phase (t1), the respiratory rate was reduced to 12.83 ± 3.64 mcm (3% EtOH) and 14.20 ± 4.49 mcm (1.8% MgCl2; Figure 3 C; Table 2). The second lowest respiratory rates were recorded with 1.5% MgCl2 concentration (18.33) followed by 2.5% EtOH and 2% EtOH groups (30.83 and 31.70 mantle contractions per minute, respectively). The less depressed respiratory rate in squid embryos was observed with the 1% MgCl2 concentration, reaching 36.70 mantle contractions per minute (50.33 mantle contractions per minute for the control group; Table 2). During recovery (t2), the respiratory rate returned to high values of 52.0 ± 4.37 mcm (1% MgCl2), 51.67 mcm (1.5% MgCl2), and 51.68 (3% EtOH group) mantle contractions per minute; 49.17 mcm (2.5% EtOH) and 47.70 mcm for L. vulgaris embryos exposed to 2% EtOH concentrations. We registered 49.67 mantle contractions per minute in the control group (Table 2).
TABLE 2. Respiratory rate (mantle contractions/minute) of L. vulgaris embryos exposed to different EtOH and MgCl2 concentrations and the controls (no anesthetic solution). Measures are summarized (mean, SE, and 95% confidence interval) at the three different phases: baseline, t0; anesthesia, t1; and recovery, t2.
FIGURE 3. (A) Expansion of the mantle. (B) Contraction of the mantle. Both (A) and (B) correspond to one respiration. (C) Respiratory rate (mantle contractions/minute) before, during, and after anesthesia for the three concentrations of EtOH (3%, 2.5%, and 2%) and MgCl2 (1.8%, 1.5%, and 1%), as well as the control group. The y-axis represents the respiratory rate, the x-axis displays the three phases of the experiment, each lasting 5 min; T0, control phase; T1, sedation phase; and T2, recovery phase.
No differences were observed during t0 (baseline; see Supplementary Table S2). However substantial differences were noted when t0 (before anesthesia) and t1 (after anesthesia) phases were compared (p < 0.001, 1.8%, and 1.5% MgCl2 concentration groups). No differences emerged when embryos exposed to 1% MgCl2 were considered (p = 0.079; Supplementary Table S2). In addition, when baseline (t0) and the recovery (t2) phases were considered, we did not find significant differences except for the 1.8% MgCl2 group. Comparison between the anesthesia phase (t1) and the recovery (t2) revealed significant differences for the 1.5% (p < 0.001) and 1% (p = 0.078) MgCl2 groups, showing recovery which appears to be more efficient when the 1.8% concentration was considered (Supplementary Table S2).
Time until loss of buoyancy “TL B″ was shorter on average when using EtOH (54 ± 8.29 s) compared to values recorded with MgCl2 (194 ± 52.61 s; Figure 4 A and B). The faster time until loss of buoyancy was reached when embryos were exposed to 3% EtOH concentration (19.83 ± 3.66 s; Table 3), followed by the 2.5% EtOH concentration and the 2% EtOH concentration (68.33 ± 7.17, and 73.83 ± 10.44 s, respectively). For the MgCl2 group, the TL B took longer (TL B: 107.50 ± 41.60 s, 1.8% MgCl2; 174.50 ± 30.09 s, 1.5% MgCl2; 299.50 ± 3.69 s, 1% MgCl2; Table 3). During the recovery phase after anesthesia (t2), the average time to recovery buoyancy “TR B″ was 198.83 ± 33.25 s for the EtOH group but required 1700.06 ± 1185.52 s for the MgCl2 group, thus suggesting a marked interindividual variability in this case (Table 3). The highest TR B value was observed for embryos exposed to 1.8% MgCl2 (4721.67 ± 855.4 s), followed by animals of the 1.5% MgCl2 concentration (314 ± 21.60 s), then by the 3% EtOH group (223.33 ± 20.00 s) and the 1.5% and 2% EtOH groups (194.67 ± 42.70 and 178.50 ± 44.40 s, respectively). The faster TR B was obtained with 1% MgCl2 concentration at 64.50 ± 12.60 s (Table 3).
FIGURE 4. (A) Box and whiskers plot with the time until loss of buoyancy response in seconds. The y-axis represents the seconds until loss of buoyancy and the x-axis displays the three EtOH and MgCl2 concentrations. The upper and lower limits show the highest and lowest times, respectively, until the loss of buoyancy, and the mid-point shows the mean. (B) Box and whiskers plot with the time to recovery of buoyancy response in seconds. The y-axis represents the seconds to the recovery of buoyancy and the x-axis displays the three EtOH and MgCl2 concentrations. The upper and lower limits show the highest and lowest times, respectively, until recovery of buoyancy, and the mid-point shows the mean.
TABLE 3. Time until loss of buoyancy in seconds (TL B) and time to recovery of buoyancy in seconds (TR B) of L. vulgaris embryos exposed to different EtOH and MgCl2 concentrations and the controls (no anesthetic solution). Measures are summarized (mean, median, SD, and SE).
No significant differences were obtained for the time until loss of buoyancy among all three EtOH concentrations and the 1.8% MgCl2 concentration (Supplementary Table S3). However, significant differences were found in comparing EtOH concentrations and the 1.5% and 1% MgCl2 groups (Supplementary Table S3). Substantial differences existed among groups; the 3% EtOH concentration was significant when compared to the baseline, while the 1.8% and 1.5% MgCl2 concentrations had no significant differences (Supplementary Table S3).
Concerning the time to recover buoyancy, no significant differences existed between the EtOH concentrations and the 1.5% MgCl2 group (Supplementary Table S3). Among the EtOH concentrations, no significant difference emerged for time to recover buoyancy, in contrast to the case of the MgCl2 group (all significant; Supplementary Table S3).
Time until loss of chromatophore activity “TL CR” was faster on average with EtOH (147.89 ± 14.45 s) than with MgCl2 (168.44 ± 15.26 s; Figure 5D). The faster onset was with the 3% EtOH concentration at 80.50 ± 36.40 s, followed by the 1.8% MgCl2 group (108.50 ± 45.70 s; Table 4). The loss of chromatophore activity was reached in 130.17 ± 41.10 s when squid embryos were exposed to 1.5% MgCl2 concentration and in 163.17 ± 26.30 s when the animals were exposed to 2.5% EtOH concentration (Table 4). On the other hand, TL CR required more than 200 s when the squid embryos were tested with 2% EtOH (200 ± 36.70 s) and 1% MgCl2 (266.67 ± 33.33 s; Table 4).
FIGURE 5. (A) Contracted chromatophores corresponding to muscle relaxation. (B) A half-contracted chromatophores. (C) Completely relaxed chromatophores corresponding to full muscle contraction. (D) Box and whiskers plot with the time until the loss or to recovery of chromatophores activity in seconds. The y-axis represents the seconds until the loss or to recovery of chromatophores and the x-axis displays the three EtOH and MgCl2 concentrations. The upper and lower limits show the highest and lowest times, respectively, until loss or to recovery of chromatophore activity, and the mid-point shows the mean.
TABLE 4. Time until loss of chromatophore tone in seconds (TL CR) and time to recovery of chromatophore activity in seconds (TR CR) of L. vulgaris embryos exposed to different EtOH and MgCl2 concentrations and the controls (no anesthetic solution). Measures are summarized (mean, median, SD, and SE).
Recovery of chromatophore tone was quite fast with both MgCl2 and EtOH (61.89 ± 19.91; 70.44 ± 52.69 s, respectively). The fastest recovery was recorded when the lowest MgCl2 concentration (1%) was used (11.17 ± 2.47 s) and the 2.5% EtOH group (45.83 ± 16.13 s; Table 4). More time was required when animals were exposed to the 2% EtOH concentration (68.50 ± 12.03 s; Table 4). On the other hand, 3% EtOH concentration and 1.8% MgCl2 concentration resulted in a longer time to recovery of chromatophore tone (97 ± 51.17; 88.33 ± 17.13 s, respectively; Table 4). It is interesting to note that embryos that exhibited half-contracted or relaxed chromatophore states at the baseline performed a similar pattern during the whole immersion in EtOH until the change to fresh artificial seawater.
There were no significant differences in the time to recovery of chromatophore activity among the three concentrations of EtOH and the three concentrations of MgCl2. However, significant differences were observed between the 1.8% and 1% MgCl2 and between 1.5% and 1% MgCl2 (Supplementary Table S4) groups.
Time until loss of the tentacles/arms response “TL T/A R” was quicker on average for the MgCl2 concentrations with 96.94 ± 25.26 s and 117.83 ± 37.54 s for the EtOH concentrations (Figure 6 A and B). The faster TL T/AR was achieved by the 1.8% MgCl2 concentration with 44.83 ± 11.70 s, followed by 46.33 ± 7.43 s for the 3% EtOH concentration. The second fastest TL T/A R resulted in conditions where animals were exposed to 1.5% MgCl2 concentration (99 ± 16.54 s); this was followed by TL T/A R in animals exposed to 2% MgCl2 concentration (147.67 ± 29.66 s) and 2.5% EtOH (159.51 ± 36.81; Table 5). The slower TL T/A R was observed for squid embryos exposed to 1% MgCl2 concentrations (266.70 ± 33.33 s; Table 5). Time to the recovery of tentacles/arms response “TR T/A R″ was on average quicker for the EtOH (67.94 ± 37.85 s) than was for the MgCl2 (73.28 ± 28.37 s) group. We observed the quickest recovery in the 1% MgCl2 group, followed by the groups exposed to 2.5% EtOH and 2% EtOH (20.17 ± 8.35; 36.67 ± 11.30; 40.33 ± 11.55 s, respectively; Table 5). The longest TR T/A R was obtained for the 3% EtOH concentration (126.83 ± 38.19 s) and 1.8% MgCl2 (117.33 ± 21.75 s; Supplementary Table S5) groups.
FIGURE 6. (A) Box and whiskers plot with the time until loss of tentacles/arms response in seconds. The y-axis represents the seconds until loss of tentacles/arms response and the x-axis displays the three EtOH and MgCl2 concentrations. The upper and lower limits show the highest and the lowest times, respectively, until the loss of tentacles/arms response, and the mid-point shows the mean. (B) Box and whiskers plot with the time to recovery of tentacles/arms response in seconds. The y-axis represents the seconds to recovery of tentacles/arms response and the x-axis displays the three EtOH and MgCl2 concentrations. The upper and lower limits show the highest and the lowest times, respectively, until the recovery of tentacles/arms response, and the mid-point shows the mean.
TABLE 5. Time until loss of tentacles/arms response in seconds (TL T/A R) and time to recovery of tentacles/arms response in seconds (TR T/A R) of L. vulgaris embryos exposed to different EtOH and MgCl2 concentrations and the controls (no anesthetic solution). Measures are summarized (mean, median, SD, and SE).
No TARP reactions (as defined by Gleadall, 2013) were observed throughout the course of the study.
The embryos were monitored for 48 h after anesthesia to assess their vitality and any other adverse effects. Our data show that embryos exposed to MgCl2 can be divided into two groups: the first group are the embryos exposed to 1.8% concentration and the second group are those anesthetized with the 1.5% and 1% MgCl2 concentrations. None of the embryos exposed to 1.8% MgCl2 survived after 6 h. Besides buoyancy, all the other parameters considerably returned to values similar to the baseline; the heart and respiratory rates took between 40 min and 1 h to normalize. The chromatophore tone and tentacles/arms response also recovered relatively quickly within 5 min. However, the quickest recovery for the buoyancy was obtained after 2 h 46 min for one embryo and did not occur for the remaining embryos. On the other hand, 8 out of 12 embryos survived 24 h with a decreased heart rate and respiratory rate and only four survived 48 h. In any case, death appeared to be linked to respiratory depression followed by poor oxygenation.
The short life span can be explained by the type of anesthetic but also the fact that embryos had lost their external yolk, leaving them with only their internal yolk to feed them. For the EtOH group, for each concentration, only one embryo was kept for this follow-up. The three embryos those survived 24 h with a proper heart and respiratory rate, buoyancy, chromatophore, and tentacles/arms response were still present. After 48 h, the embryos were still present with a heart rate, but with a lower rate than the baseline, and the respiration was depressed with partial buoyancy.
The large diversity of cephalopods, with over 800 species, makes it difficult to standardize the use and dosage of anesthetic solutions. For research purposes, only a few species are currently being studied. However, these species have diverged, adapting to rather different lifestyles and differing in body sizes. While it is challenging to anesthetize different species, it is important to establish some common approaches and standardize the evaluation of anesthetic quality in these animals. In our study, the parameters proposed by Pagano et al. (2011) and Andrews et al. (2013) were investigated as much as the size and physiology of the Loligo embryos allowed.
The concentrations of EtOH and MgCl2 used in the present study were based on ranges previously utilized for adult animals (reviewed in Fiorito et al., 2015). Depending on the species and body weight, concentrations ranging from 1 to 3% were suggested for EtOH and from 1.5% to 3.75% for MgCl2. Therefore, in our study the concentrations for the EtOH solution tested were 2%, 2.5%, and 3% and were 1%, 1.5% and 1.8% for MgCl2 in seawater. It seems likely that the body size and age of the animal may affect the efficiency of a given anesthetic. In our study, the comparison of these substances revealed that for the heart rate and respiratory rate, EtOH had a faster onset and recovery than did MgCl2, confirming previous observations reported for adult squids. Indeed, a recent study found that EtOH is less depressive and easier to recover than MgCl2 for octopus and cuttlefish (Abbo et al., 2021). Nevertheless, the return of the chromatophore and tentacle/arm responses was faster for the MgCl2 groups than the EtOH groups.
The mechanisms of MgCl2 and EtOH anesthetic functions in cephalopods is still largely unknown. A recent study in Sepia pharaonis showed that MgCl2 enhances the amount of the inhibitory neurotransmitters glycine and tyrosine while reducing the level of dopamine (Yang et al., 2020). In contrast to EtOH, MgCl2 has a prolonged activity, being therefore potentially more suitable for longer procedures. In addition, MgCl2 has been reported to have not only immobilizing properties but also analgesic ones, by acting as a neurotransmitter release blocker and having local anesthetic properties (Butler-Struben et al., 2018). However, the mode of action and physiological mechanism of MgCl2 as an anesthetic have not been fully elucidated and remain to be explored. It has been studied extensively in mice (Wong et al., 1997; Sharko and Hodge, 2008). According to Wong et al. (1997), the use of EtOH resembles the use of volatile anesthetics such as desflurane, both depressing the muscle stretch reflex or monosynaptic reflex while having a quick onset and offset of action as well as an age-related rise of anesthetic potency. The younger the animal, the higher the required dosage; the latter is along the lines of the higher dosage used in our experiment. In addition, EtOH has a hypnotic and sedative effect modulated through metabotropic glutamate receptors requiring GABAA and NMDA receptors. Even though EtOH analgesic effects are not known in cephalopods, it is notorious to provoke a possible status of loss of consciousness in humans, followed in some cases of amnesia and analgesic effects (Tamerin et al., 1971; Thompson et al., 2017), but state dependency (Goodwin et al., 1969; Bettinger and McIntire, 2004; Rezayof et al., 2008) has been widely reported and may be the cause of such effects.
In the future, it will be possible to compare different concentrations, combinations of substances, or other anesthetics (e.g., isoflurane; Polese et al., 2014). In the present study, embryos were anesthetized by immersion, which is a minimally invasive approach, largely utilized in fish, cephalopods, and other aquatic organisms. Other administration methodologies exist, such as subcutaneous or intravenous injections, and might be explored for future studies, with appropriate care in terms of monitoring stress and severity degrees.
The heart rate and respiratory rate are basic vital parameters that can be easily recorded during anesthesia for cephalopods and, particularly for, Loligo vulgaris embryos, and no reference values are available for these vital parameters in the literature. Therefore, obtaining baseline data before and after anesthesia was one of our main goals.
During the baseline phase, the heart rate and respiratory rate were recorded, and they were consistent among all embryos. During anesthesia, the heart and respiratory rates were depressed for both anesthetics, however, important differences among embryos were observed, corroborating the idea that individual susceptibility to anesthetic concentration is present (Butler-Struben et al., 2018). The best EtOH concentration remained 3%, in comparison to the 2.5% and 2% EtOH concentrations (but mortality at the end of the experiment should be carefully considered). For the latter two, the embryos were lightly anesthetized, and the decrease in the heart and respiratory rates showed no significant differences between the three phases (Figure 2 and 3, t1–t2). EtOH 3% concentration displayed the quickest return to the initial baseline: within 1 min. The respiration rate at the 3% EtOH concentration resulted as the lowest of all concentrations and returned similarly to baseline within 3 min, similar to the most optimal MgCl2 concentration. Comparing the heart and respiratory rate parameters, the optimal MgCl2 solution was the 1.5% concentration; the heart rate obtained after anesthesia was equivalent to the 1.8% MgCl2 concentration but lower than the 3% EtOH concentration. The return to baseline was obtained within 6 min instead of nearly 40 min to 1 h for the 1.8% MgCl2 concentration. The respiratory rate attained with the 1.5% concentration was the third lowest rate after the 1.8% MgCl2 concentration and the 3% EtOH concentration. Concerning the recovery, it was reached within the observed 5 min.
In mammals, the return to a responsive and conscious state following general anesthesia is typically evaluated by looking at eye reflexes, jaw tone, and occurrence of spontaneous movements. In cephalopods, tentacle adhesiveness as well as eye reflexes have been proposed as signs of recovery (Sen and Tanrikul, 2009; Pagano et al., 2011). Due to the smaller size of the embryos used in this study, these parameters would have been difficult to assess. Therefore, the tentacles/arms response to pinch was used instead. This response was lost quickly on average with MgCl2, especially at the 1.8% concentration, than with EtOH, but recovery was never reached for this concentration. Nevertheless, lower dosages of 1% MgCl2 attained the quickest recovery time. Corroborating the mechanism of action of MgCl2 (Yang et al., 2020), indeed MgCl2 not only releases inhibitory neurotransmitters but at the same time also reduces the release of excitatory neurotransmitters, and therefore lower concentrations could have less depressive effects.
Cephalopods possess unique features such as chromatophore activity, allowing adaptation to environmental conditions. Indeed, while submerging the embryos in both anesthetic agents, the chromatophore reacted until a complete contraction (paling). Both MgCl2 and EtOH showed a similar effect and no differences existed among the concentrations for the time until the loss and recovery of function. The faster TL CR was obtained with 3% EtOH, with the longer TR CR being registered for the 1.8% MgCl2 concentration, possibly indicating that at higher concentrations, MgCl2 is a better muscle relaxant than EtOH.
Buoyancy is another physiological mechanism that healthy squids should display. According to previously published guidelines for aquatic species, anesthesia is considered “effective” if it acts within 3 min of immersion in an anesthetic bath, while recovery should occur within 5 min after returning to an anesthetic-free solution (Marking and Meyer, 1985). Both anesthetics were quite efficient in inducing loss of buoyancy, no significant differences existed for the three EtOH solutions and 1.8% MgCl2. Regarding the recovery of function, it was quicker with EtOH than with MgCl2. Since the 1.8% MgCl2 concentration took longer than 300 s to recover, it indicates a non-recovery of function, and therefore according to the definition of Marking and Meyer (1985), does not fulfill the efficiency criteria. In addition, no significant differences existed between the 3% EtOH, 2.5% EtOH, and 1.5% MgCl2 concentrations for the time to recovery of buoyancy.
Any adverse response pattern should be documented for embryos. According to Gleadall (2013), there are different adverse responses for embryos, such as inking, defecating, or rapid changes of chromatophore patterns. Immersion in EtOH and MgCl2 solutions was well tolerated by embryos of the common squid Loligo vulgaris, and no adverse effects at the tested concentrations were observed, corroborating previous reports (Lange and Hartline, 1974; Garcia-Franco, 1992; Ikeda et al., 2009; Mooney et al., 2010; Estefanell et al., 2011).
Following various authors, cephalopods react to anesthesia by changes in the respiratory rate, which appear depressed with the magnitude, depending on the concentration. Similar results were obtained in our study in squid embryos since their respiratory rates went down with differences in depth, depending on the concentrations used. Furthermore, the loss of body color or chromatophore relaxation are the other indicators of the depth of anesthesia. According to the substances used, adults look paler or achromatic. Indeed, for the embryos, this was also the case; their chromatophores relaxed, not allowing the pigmentation to show. Sucking intensity is the only parameter that could not be measured in embryos in this study due to their relative size. Instead, pinching of arms and tentacles was used.
We found that both substances had similar effects on the heart and respiratory rates after immersion, as well as on chromatophores activity, tentacles/arms response, and buoyancy. The main differences between EtOH and MgCl2 appeared during the recovery. Buoyancy was on average more quickly restored following immersion in EtOH than it did in MgCl2. For the 1.8% MgCl2 concentration, buoyancy was not regained, indicating that the safety for MgCl2 at this concentration cannot be guaranteed. On the other hand, no significant differences were observed for anesthetic recovery of the chromatophore and tentacle/arms responses.
It should be noted that considering the marked interindividual variability (also observed here), we cannot exclude that a fixed time frame of exposure to the anesthetic solution and recovery may be insufficient for all animals to attain a similar state for each concentration tested. Further studies may help in disclosing such a possibility.
Even though no adverse response pattern was observed during the experiment for both EtOH and MgCl2 at the investigated concentrations, EtOH seemed to be a more efficient substance for Loligo vulgaris embryos than did MgCl2 in circumstances here tested. Not only did embryos regain their heart and respiratory rates quickly but had also recovered physiological responsiveness more rapidly after the EtOH treatment.
Moreover, both substances might at increasing concentrations also be relevant for euthanasia.
The physiological parameters used here represent a critical attempt to standardize the monitoring of embryos, and together with other techniques such as electrophysiology recordings from the pallial nerves (Abbo et al., 2021) or H-NMR spectroscopy (Yang et al., 2020), could elucidate the mechanism of action of MgCl2 and EtOH, in particular, the reversible inhibitory activity of these substances. In future studies, further measures could be included, such as for instance, skin irritation (linked with exposure to EtOH) or corticosteroids production in response to exposure to anesthetics (Chancellor et al., 2021). Such studies are critical to gain deeper knowledge on the effects and safety of anesthetic agents in cephalopods, possibly highlighting differences among species and peculiarities in mechanisms of action.
The original contributions presented in the study are included in the article/Supplementary Material; further inquiries can be directed to the corresponding author.
Ethical review and approval were not required for the animal study because only embryos were used for this study, which do not fall under the animal welfare directive.
MS, SS, and CS designed the study. MS performed the experiments and analyzed the data. MS, SS, and CS wrote the manuscript.
This work was funded by the Swiss National Science Foundation (31003A_149499 to SS.)
The authors would like to thank the Station Biologique de Roscoff, CNRS Sorbonne Université, especially R. Garnier and G. Schires. They thank R. Rohr of the Biostatistics platform of the Department of Biology for his help with the statistical analysis. They also thank C. Fritsch, J. Kaldun, and their colleagues at the University of Fribourg for the fruitful discussion and comments.
The authors declare that the research was conducted in the absence of any commercial or financial relationships that could be construed as a potential conflict of interest.
All claims expressed in this article are solely those of the authors and do not necessarily represent those of their affiliated organizations, or those of the publisher, the editors, and the reviewers. Any product that may be evaluated in this article, or claim that may be made by its manufacturer, is not guaranteed or endorsed by the publisher.
The Supplementary Material for this article can be found online at: https://www.frontiersin.org/articles/10.3389/fphys.2022.968047/full#supplementary-material
Abbo L. A., Himebaugh N. E., DeMelo L. M., Hanlon R. T., Crook R. J. (2021). Anesthetic efficacy of magnesium chloride and ethyl alcohol in temperate Octopus and cuttlefish species. J. Am. Assoc. Lab. Anim. Sci. 60 (5), 556–567. doi:10.30802/AALAS-JAALAS-20-000076
Andrews P. L. R., Darmaillacq A. S., Dennison N., Gleadall I. G., Hawkins P., Messenger J. B., et al. (2013). The identification and management of pain, suffering and distress in cephalopods, including anaesthesia, analgesia and humane killing. J. Exp. Mar. Biol. Ecol. 447, 46–64. doi:10.1016/j.jembe.2013.02.010
Arnold J. M., Summers W. C., Gilbert D. L., Manalis R. S., Daw N. W., Lasek R. J. (1974). A guide to laboratory use of the squid Loligo pealei. Woods Hole. Mass: Marine Biological Laboratory.
Baldascino E., Di Cristina G., Tedesco P., Hobbs C., Shaw T. J., Ponte G., et al. (2017). The gastric ganglion of Octopus vulgaris: Preliminary characterization of gene- and putative neurochemical-complexity, and the effect of Aggregata octopiana digestive tract infection on gene expression. Front. Physiol. 8 (1001). doi:10.3389/fphys.2017.01001
Bettinger J. C., McIntire S. L. (2004). State-dependency in C. elegans. Genes. Brain Behav. 3 (5), 266–272. doi:10.1111/j.1601-183X.2004.00080.x
Birch J. (2017). Animal sentience and the precautionary principle. Anim. Sentience 2 (16), 1. doi:10.51291/2377-7478.1200
Birch J., Burn C., Schnell A., Browning H., Crump A. (2021). Review of the evidence of sentience in cephalopod molluscs and decapod Crustaceans. London: London School of Economics and Political Science.
Bone Q., Brown E., Travers G. (1994). On the respiratory flow in the cuttlefish sepia officinalis. J. Exp. Biol. 194, 153–165. doi:10.1242/jeb.194.1.153
Burbach J. P., Grant P., Hellemons A. J., Degiorgis J. A., Li K. W., Pant H. C. (2014). Differential expression of the FMRF gene in adult and hatchling stellate ganglia of the squid Loligo pealei. Biol. Open 3 (1), 50–58. doi:10.1242/bio.20136890
Butler-Struben H. M., Brophy S. M., Johnson N. A., Crook R. J. (2018). In vivo recording of neural and behavioral correlates of anesthesia induction, reversal, and euthanasia in cephalopod molluscs. Front. Physiol. 9, 109. doi:10.3389/fphys.2018.00109
Chancellor S., Abbo L., Grasse B., Sakmar T., Brown J. S., Scheel D., et al. (2021). Determining the effectiveness of using dermal swabs to evaluate the stress physiology of laboratory cephalopods: A preliminary investigation. Gen. Comp. Endocrinol. 314, 113903. doi:10.1016/j.ygcen.2021.113903
Crawford K., Diaz Quiroz J. F., Koenig K. M., Ahuja N., Albertin C. B., Rosenthal J. J. C. (2020). Highly efficient knockout of a squid pigmentation gene. Curr. Biol. 30 (17), 3484–3490. e3484. doi:10.1016/j.cub.2020.06.099
Crook R. J. (2021). Behavioral and neurophysiological evidence suggests affective pain experience in octopus. iScience 24 (3), 102229. doi:10.1016/j.isci.2021.102229
Crook R. J., Hanlon R. T., Walters E. T. (2013). Squid have nociceptors that display widespread long-term sensitization and spontaneous activity after bodily injury. J. Neurosci. 33 (24), 10021–10026. doi:10.1523/JNEUROSCI.0646-13.2013
Crook R. J., Lewis T., Hanlon R. T., Walters E. T. (2011). Peripheral injury induces long-term sensitization of defensive responses to visual and tactile stimuli in the squid Loligo pealeii, Lesueur 1821. J. Exp. Biol. 214 (19), 3173–3185. doi:10.1242/jeb.058131
Crook R. J., Walters E. T. (2011). Nociceptive behavior and physiology of molluscs: Animal welfare implications. ILAR J. 52 (2), 185–195. doi:10.1093/ilar.52.2.185
Darmaillacq A.-S., Chichery R., Shashar N., Dickel L. (2006). Early familiarization overrides innate prey preference in newly hatched Sepia officinalis cuttlefish. Anim. Behav. 71 (3), 511–514. doi:10.1016/j.anbehav.2005.04.019
Efsa Panel o. A. H. a. W. (2005). Opinion of the Scientific Panel on Animal Health and Welfare (AHAW) on a request from the Commission related to the "Aspects of the biology and welfare of animals used for experimental and other scientific purposes. EFSA J. 292, 1–136. doi:10.2903/j.efsa.2005.292
Estefanell J., Socorro J., Afonso J. M., Roo J., Fernández-Palacios H., Izquierdo M. S. (2011). Evaluation of two anaesthetic agents and the passive integrated transponder tagging system in Octopus vulgaris (Cuvier 1797). Aquac. Res. 42 (3), 399–406. doi:10.1111/j.1365-2109.2010.02634.x
EU (2019). Report on the statistics on the use of animals for scientific purposes in the Member States of the European Union in 2015-2017. Available at: https://op.europa.en/en/publication-detail/-/publication/04a890d4-47ff-11ea-b81b-01aa75ed71a1
Fiorito G., Affuso A., Basil J., Cole A., de Girolamo P., D'Angelo L., et al. (2015). Guidelines for the care and welfare of cephalopods in research -A consensus based on an initiative by CephRes, FELASA and the boyd group. Lab. Anim. 49 (2), 1–90. doi:10.1177/0023677215580006
Fuentes L., Iglesias J., Sánchez F. J., Otero J. J., Moxica C., Lago M. J. (2005). Técnicas de transporte de paralarvas y adultos de pulpo (Octopus vulgaris). Bol. Inst. Esp. Oceanogr. 21 (1-4), 155–162.
Garcia-Franco M. (1992). Anaesthetics for the squid sepioteuthis sepioidea (mollusca: Cephalopoda). Comp. Biochem. Physiology Part C Comp. Pharmacol. 103 (1), 121–123. doi:10.1016/0742-8413(92)90239-4
Gleadall I. G. (2013). The effects of prospective anaesthetic substances on cephalopods: Summary of original data and a brief review of studies over the last two decades. J. Exp. Mar. Biol. Ecol. 447 (11), 23–30. doi:10.1016/j.jembe.2013.02.008
Goodwin D. W., Powell B., Bremer D., Hoine H., Stern J. (1969). Alcohol and recall: State-dependent effects in man. Science 163 (3873), 1358–1360. doi:10.1126/science.163.3873.1358
Howard R. B., Lopes L. N., Lardie C. R., Perez P. P., Crook R. J. (2019). Early-life injury produces lifelong neural hyperexcitability, cognitive deficit and altered defensive behaviour in the squid Euprymna scolopes. Philos. Trans. R. Soc. Lond. B Biol. Sci. 374 (1785), 20190281. doi:10.1098/rstb.2019.0281
Ikeda Y., Sugimoto C., Yonamine H., Oshima Y. (2009). Method of ethanol anaesthesia and individual marking for oval squid (Sepioteuthis lessoniana Férussac, 1831 in Lesson 1830â “1831). Aquac. Res. 41 (1), 157–160. doi:10.1111/j.1365-2109.2009.02305.x
Imperadore P., Lepore M. G., Ponte G., Pflüger H.-J., Fiorito G. (2019a). Neural pathways in the pallial nerve and arm nerve cord revealed by neurobiotin backfilling in the cephalopod mollusk Octopus vulgaris. Invert. Neurosci. 19 (2), 5. doi:10.1007/s10158-019-0225-y
Imperadore P., Parazzoli D., Oldani A., Duebbert M., Buschges A., Fiorito G. (2019b). From injury to full repair: Nerve regeneration and functional recovery in the common octopus, Octopus vulgaris. J. Exp. Biol. 222 (19), jeb209965. doi:10.1242/jeb.209965
Jozet-Alves C., Moderan J., Dickel L. (2008). Sex differences in spatial cognition in an invertebrate: The cuttlefish. Proc. Biol. Sci. 275 (1646), 2049–2054. doi:10.1098/rspb.2008.0501
Katzung B., Kruidering-Hall M., Tuan R. L., Vanderah T. W., Trevor A. (2021). Katzung & trevor's pharmacology: Examination and board review. New York: McGraw-Hill Education.
Kumar P. S., Kumar S. S., Mekala P., Arivuchelvan A., Jagadeeswaran A. (2013). ICT in local self governance: A study of rural India. Int. J. Comput. Appl. 83(5), 31–36. doi:10.5120/14453-2714
Lange G. D., Hartline P. H. (1974). Retinal responses in squid and octopus. J. Comp. Physiol. 93 (1), 19–36. doi:10.1007/BF00608757
Lee Y. C., Darmaillacq A. S., Dickel L., Chiao C. C. (2020). Effects of embryonic exposure to predators on the postnatal defensive behaviors of cuttlefish. J. Exp. Mar. Biol. Ecol. 524, 151288. doi:10.1016/j.jembe.2019.151288
Locatello L., Fiorito G., Finos L., Rasotto M. B. (2013). Behavioural and immunological responses to an immune challenge in Octopus vulgaris. Physiol. Behav. 122, 93–99. doi:10.1016/j.physbeh.2013.08.029
Mackie G. O. (2008). Immunostaining of peripheral nerves and other tissues in whole mount preparations from hatchling cephalopods. Tissue Cell. 40 (1), 21–29. doi:10.1016/j.tice.2007.08.005
Marking L. L., Meyer F. P. (1985). Fisheries 10 (6), 2–5. doi:10.1577/1548-8446(1985)010<0002:abanif>2.0.co;2
Mathger L. M., Hanlon R. T. (2007). Malleable skin coloration in cephalopods: Selective reflectance, transmission and absorbance of light by chromatophores and iridophores. Cell. Tissue Res. 329 (1), 179–186. doi:10.1007/s00441-007-0384-8
Messenger J. B., Nixon M., Ryan K. P. (1985). Magnesium chloride as an anaesthetic for cephalopods comparative biochemistry and physiology Part C: Comparative pharmacology 82(1), 203–205. doi:10.1016/0742-8413(85)90230-0
Moltschaniwskyj N. A., Hall K., Lipinski M. R., Marian J. E. A. R., Nishiguchi M., Sakai M., et al. (2007). Ethical and welfare considerations when using cephalopods as experimental animals. Rev. Fish. Biol. Fish. 17, 455–476. doi:10.1007/s11160-007-9056-8
Mooney A. T., Lee W.-J., Hanlon R. T. (2010). Long-duration anesthetization of squid (Doryteuthis pealeii). Mar. Freshw. Behav. Physiology 43 (4), 297–303. doi:10.1080/10236244.2010.504334
Nesher N., Maiole F., Shomrat T., Hochner B., Zullo L. (2019). From synaptic input to muscle contraction: Arm muscle cells of Octopus vulgaris show unique neuromuscular junction and excitation-contraction coupling properties. Proc. Biol. Sci. 286 (1278). doi:10.1098/rspb.2019.1278
Pagano E., Ponte G., Andrews P., Fiorito G. (2011). A comparative analysis of different anesthetics in Octopus: Towards true anesthesia? J. Shellfish Res. 30 (3), 1016.
Parra G., Villanueva R., Yúfera M. (2000). Respiration rates in late eggs and early hatchlings of the common octopus, Octopus vulgaris. J. Mar. Biol. Assoc. U. K. 80 (3), 557–558. doi:10.1017/S0025315400002319
Polese G., Winlow W., Di Cosmo A. (2014). Dose-dependent effects of the clinical anesthetic isoflurane on Octopus vulgaris: A contribution to cephalopod welfare. J. Aquat. Anim. Health 26 (4), 285–294. doi:10.1080/08997659.2014.945047
Ponte G., Fiorito G. (2015). in Immunohistochemical analysis of neuronal networks in the nervous system of Octopus vulgaris. Editors A. Merighi, and L. Lossi, 61–77.
Rezayof A., Alijanpour S., Zarrindast M.-R., Rassouli Y. (2008). Ethanol state-dependent memory: Involvement of dorsal hippocampal muscarinic and nicotinic receptors. Neurobiol. Learn. Mem. 89 (4), 441–447. doi:10.1016/j.nlm.2007.10.011
Rosa R., Trubenbach K., Pimentel M. S., Boavida-Portugal J., Faleiro F., Baptista M., et al. (2014). Differential impacts of ocean acidification and warming on winter and summer progeny of a coastal squid (Loligo vulgaris). J. Exp. Biol. 217 (4), 518–525. doi:10.1242/jeb.096081
Schweizerische-Tierschutzverordung (2018). Animal protection ordinance: Chapter 1: General Provisions Art 1 Scope et Art 2 Terms. Chapter 6: Animal Experiments, Genetically Modified Animals and Mutants having a Clinical Pathological Phenotype Section 1: Scope, Permitted Deviations Article 112 Scope. Avialable at: https://www.fedlex.admin.ch/eli/cc/2008/416/fr#art_1https://www.fedlex.admin.ch/eli/cc/2008/416/fr#art_2https://www.fedlex.admin.ch/eli/cc/2008/416/de#art_112.
Sen H., Tanrikul T. T. (2009). Efficacy of 2-phenoxyethanol as an anaesthetic for the musky octopus, Eledone moschata (lamarck 1799, cephalopoda: Octopodidae). Turk. J. Vet. Anim. Sci. 33 (6), 463–467.
Sharko A. C., Hodge C. W. (2008). Differential modulation of ethanol-induced sedation and hypnosis by metabotropic glutamate receptor antagonists in C57BL/6J mice. Alcohol. Clin. Exp. Res. 32 (1), 67–76. doi:10.1111/j.1530-0277.2007.00554.x
Sherrill J., Spelman L. H., Reidel C. L., Montali R. J. (2000). Common cuttlefish (sepia officinalis) mortality at the national zoological park: Implications for clinical management. J. Zoo. Wildl. Med. 31 (4), 523–531. doi:10.1638/1042-7260(2000)031[0523:CCSOMA]2.0.CO;2
Tamerin J. S., Weiner S., Poppen R., Steinglass P., Mendelson J. H. (1971). Alcohol and memory: Amnesia and short-term memory function during experimentally induced intoxication. Am. J. Psychiatry 127 (12), 1659–1664. doi:10.1176/ajp.127.12.1659
Thompson T., Oram C., Correll C. U., Tsermentseli S., Stubbs B. (2017). Analgesic effects of alcohol: A systematic review and meta-analysis of controlled experimental studies in healthy participants. J. Pain 18 (5), 499–510. doi:10.1016/j.jpain.2016.11.009
Varughese S., Ahmed R. (2021). Environmental and occupational considerations of anesthesia: A narrative review and update. Anesth. Analg. 133 (4), 826–835. doi:10.1213/ANE.0000000000005504
Wong S. M., Fong E., Tauck D. L., Kendig J. J. (1997). Ethanol as a general anesthetic: Actions in spinal cord. Eur. J. Pharmacol. 329 (2-3), 121–127. doi:10.1016/s0014-2999(97)89174-1
Yang H., Zhao Y., Song W., Ye Y., Wang C., Mu C., et al. (2020). Evaluation of the efficacy of potential anesthetic agents on cuttlefish (Sepia pharaonis) juveniles. Aquaculture Reports 1005242.
Zatylny-Gaudin C., Cornet V., Leduc A., Zanuttini B., Corre E., Le Corguille G., et al. (2016). Neuropeptidome of the cephalopod sepia officinalis: Identification, tissue mapping, and expression pattern of neuropeptides and neurohormones during egg laying. J. Proteome Res. 15 (1), 48–67. doi:10.1021/acs.jproteome.5b00463
Keywords: anesthesia, cephalopods embryos, Loligo vulgaris, ethanol, administration and dosage, magnesium chloride (MgCl2)
Citation: Sprecher M, Sprecher SG and Spadavecchia C (2022) A pilot investigation of the efficacy and safety of magnesium chloride and ethanol as anesthetics in Loligo vulgaris embryos. Front. Physiol. 13:968047. doi: 10.3389/fphys.2022.968047
Received: 13 June 2022; Accepted: 18 August 2022;
Published: 14 September 2022.
Edited by:
Graziano Fiorito, Stazione Zoologica Anton Dohrn, ItalyReviewed by:
Paul Andrews, St George’s, University of London, United KingdomCopyright © 2022 Sprecher, Sprecher and Spadavecchia. This is an open-access article distributed under the terms of the Creative Commons Attribution License (CC BY). The use, distribution or reproduction in other forums is permitted, provided the original author(s) and the copyright owner(s) are credited and that the original publication in this journal is cited, in accordance with accepted academic practice. No use, distribution or reproduction is permitted which does not comply with these terms.
*Correspondence: Marta Sprecher, bWFydGEuc3ByZWNoZXJAdW5pZnIuY2g=
Disclaimer: All claims expressed in this article are solely those of the authors and do not necessarily represent those of their affiliated organizations, or those of the publisher, the editors and the reviewers. Any product that may be evaluated in this article or claim that may be made by its manufacturer is not guaranteed or endorsed by the publisher.
Research integrity at Frontiers
Learn more about the work of our research integrity team to safeguard the quality of each article we publish.