- Key Laboratory of Freshwater Fisheries and Germplasm Resources Utilization, Ministry of Agriculture and Rural Affairs, Freshwater Fisheries Research Center, Chinese Academy of Fishery Sciences, Wuxi, China
Corbicula fluminea (C. fluminea) is an important freshwater economy shellfish in China, but it often suffers from air exposure during transportation. In this study, we investigated the histological, physiological (mainly including respiratory metabolism, antioxidant capacity, and immune function), and transcriptomic responses of hepatopancreas in C. fluminea to different times of air exposure. At histological level, air exposure caused vacuolation of digestive cells (24–96 h) and enlargement of digestive tubule lumen (6–96 h) in hepatopancreas. At physiological level, the activities of enzymes related to glycolysis (hexokinase and pyruvate kinase) and anaerobic respiration (lactate dehydrogenase) were increased first (6–24 h) of air exposure, then came back to normal level or even decreased. The activity of aerobic respiration-related enzyme (succinic dehydrogenase) began to reduce from 24 h of air exposure. The activities of antioxidant enzymes (superoxide dismutase and catalase) were enhanced during 6–48 h of air exposure and then returned to control level or even inhibited. The content of malondialdehyde (MDA) increased from 96 h of air exposure. The activities of immune-related enzymes (acid phosphatase and alkaline phosphatase) increased during 6–48 h, then returned to normal or began to decline. At transcriptome level, 44 differentially expressed genes (DEGs) in the hepatopancreas were identified after 96-h air exposure. Among these DEGs, 8 were associated with glycolysis, TCA cycle, immune, and antioxidant, and were downregulated after 96-h air exposure. Taken together, these findings illuminated the response of C. fluminea to air exposure at histological, physiological, and transcriptomic levels, which will be beneficial to the aquaculture and transportation of C. fluminea.
Introduction
Aquatic animals often suffer from many stresses during the aquaculture process, which will harm their health and the aquaculture industry. Among many stresses, air exposure is unavoidable stress for most aquatic animals and may occur during ebb tide, harvest, and transportation (Yin et al., 2017; Zhou et al., 2021). When exposed to air, aquatic animals have less access to oxygen and face severe stress of hypoxia. Lots of evidence indicate that severe air exposure will cause several adverse effects on aquatic animals, such as oxidative stress injury, cell apoptosis, destruction of the histological structure, disruption of energy homeostasis, poor muscle quality, reduced growth, and even death (Arlinghaus and Hallermann, 2007; Gu et al., 2017; Lu et al., 2021; Harianto et al., 2022). To cope with desiccation and hypoxia stress of air exposure, aquatic animals can mobilize multiple adaptive physiological and molecular mechanisms, including changing respiratory metabolic strategies, enhancing immune function, increasing antioxidant defense, and altering gene expression (Romero et al., 2007; Bao et al., 2019). At present, there are many studies on the response of various aquatic animals, including shellfish, to air exposure. Whereas most studies on shellfish mainly focus on intertidal marine shellfish (Meng et al., 2018; Nie et al., 2020), there are a few studies on freshwater shellfish.
The freshwater shellfish Asian clam Corbicula fluminea (C. fluminea), which belongs to the class Bivalvia, the family Corbiculidae, and the genus Corbicula, is distributed in many rivers, estuaries, and lakes in China (Rosa et al., 2011). It is an important part of freshwater fisheries resources in China due to its excellent product performance and high economic value (Abaychi and Mustafa, 1988). In recent years, C. fluminea and its processing products are selling well in Japan and Southeast Asia countries, making it become one of the important export aquatic products in China (Liao et al., 2013). However, it takes a long time to transport C. fluminea from harvest to market. To reduce transport costs, the transportation of C. fluminea is usually waterless, which makes C. fluminea face the risk of air exposure. Therefore, it is necessary to clarify the responses of C. fluminea to air exposure for their aquaculture and transportation. Although the previous study has elucidated the behavior and metabolic response of C. fluminea to air exposure (Byrne et al., 1990), the histological, physiological, and molecular responses have been poorly studied.
In this study, we investigated the effects of air exposure on the histology, physiology (mainly including respiratory metabolism, antioxidant capacity, and immune function) as well as transcriptome of the hepatopancreas of C. fluminea for the first time. This study will provide valuable data to clarify the coping mechanism of C. fluminea to air exposure and be beneficial to the aquaculture and transportation of C. fluminea.
Materials and methods
Animals
C. fluminea (wet weight: 4.10 ± 0.90 g; shell length: 25.32 ± 2.02 mm; shell height: 20.88 ± 1.99 mm; shell width: 13.37 ± 1.24 mm) were obtained from the Anhui Shuiyun Environmental Protection Technology Co., LTD. (China, Wuhu). Before exposure experiments, all C. fluminea were kept in dechlorinated freshwater with a temperature of 20°C ± 0.5°C, dissolved oxygen of 6.16 ± 0.13 mg/L, and a pH of 8.3 ± 0.1 for 2 weeks of acclimation. In this period, clams were fed twice daily with chlorella. All animal procedures followed the Animal Experiments Ethics Committee of Freshwater Fisheries Research Center, Chinese Academy of Fishery Sciences.
Air exposure and sampling
The C. fluminea were randomly divided into seven groups: 0, 6, 12, 24, 48, 72, and 96 h. Three replications were set up in each group. Clams in the 0 h group were used as controls and reared as they had been during acclimation. The individuals of other groups were placed into aquariums without water to undergo the indicated times of air exposure. The hepatopancreas tissues of clams in each group were collected for further analysis.
Histopathological analysis
The hepatopancreas of four clams from each group was fixed in Bouin’s fixative solution (Phygene, China). After being dehydrated and transparentized, tissues were embedded in paraffin wax and cut into 6-μm-thick sections. Then, the paraffin sections were stained with hematoxylin/eosin (H & E) and observed under a microscope (Olympus, Japan) (Mi et al., 2021).
Biochemical detection
The hepatopancreas tissues were homogenized with 0.9% saline solution at a ratio of 1:9 on ice and centrifuged at 936 × g at 4°C for 10 min. The supernatants were collected in new tubes to detect the activities of lactate dehydrogenase (LDH), succinate dehydrogenase (SDH), hexokinase (HK), pyruvate kinase (PK), superoxide dismutase (SOD), catalase (CAT), acid phosphatase (ACP) and alkaline phosphatase (AKP), and the contents of glutathione (GSH) and malonaldehyde (MDA). All detection procedures followed the manufacturer’s instructions for commercial assay kits (LDH: A076-1-1; SDH: A022-1-1; HK: A077-3-1; PK: A076-1-1; SOD: A001-3-2; CAT: A007-1-1; ACP: A060-2-2; AKP: A059-2-2; GSH: A006-2-1; MDA: A003-1-2) (Nanjing Jiancheng Bioengineering Institute, China). The protein contents were measured using a Bradford Protein Assay Kit (Beyotime, China). The enzyme activity was normalized by protein concentration.
RNA extraction, cDNA library construction, and sequencing
The RNA samples of the hepatopancreas tissues in the 0 h group (control: CL) and 96 h group (air exposure: AE) were used for the Illumina transcriptome sequence. The hepatopancreas tissues of 3 clams were mixed into one sample, and 3 samples from each group were utilized for transcriptome sequence. High-quantity RNAs were used for the construction of Illumina cDNA libraries. Then 6 cDNA libraries were sequenced on the Illumina HiSeqX10 platform at Magigene (Guangdong, China). Fragments Per Kilobase Million mapped reads (FPKM) method was utilized to calculate the relative expressions of transcripts. The differentially expressed genes (DEGs) were identified by a threshold of false discovery rate (FDR) <0.05 and |log2 (fold change)| >1.
Real-time quantitative PCR
The RNA-seq results were verified by real-time quantitative PCR (qRT-PCR). RNA was extracted from the hepatopancreas of six clams from each group using TaKaRa MiniBEST Universal RNA (TaKaRa, Japan). The cDNA was synthesized using PrimeScript™ RT reagent Kit with gDNA Eraser (TaKaRa). The qRT-PCR was performed using B Green™ Premix Ex Taq™ (Tli RNaseH Plus) (TaKaRa). The primers used in qRT-PCR were designed by Primer 5.0 software and their sequence information was shown in Supplementary Table S1. The transcription elongation factor 1 alpha (EF1α) was utilized as a reference gene.
Statistical analysis
Data analyses were performed using SPSS 22.0 software. All data were expressed as mean ± standard deviation (SD). One-way ANOVA combined with the LSD post-test was employed to compare the differences among groups. A p-value < 0.05 indicated statistical significance.
Results
Histopathological changes in hepatopancreas
The hepatopancreas of normal C. fluminea (0 h group) mainly consisted of the digestive tubule and connective tissue. In the control group, the lumens of the digestive tubule were small or closed and the epithelial cells and digestive cells were in alignment. In air exposure groups, the enlargement of the lumen was observed in the hepatopancreas of C. fluminea from 6 to 96 h. After 24 h, the epithelial cells and digestive cells were arranged irregularly and even exfoliated. The vacuolation occurred after 48 h (Figure 1).
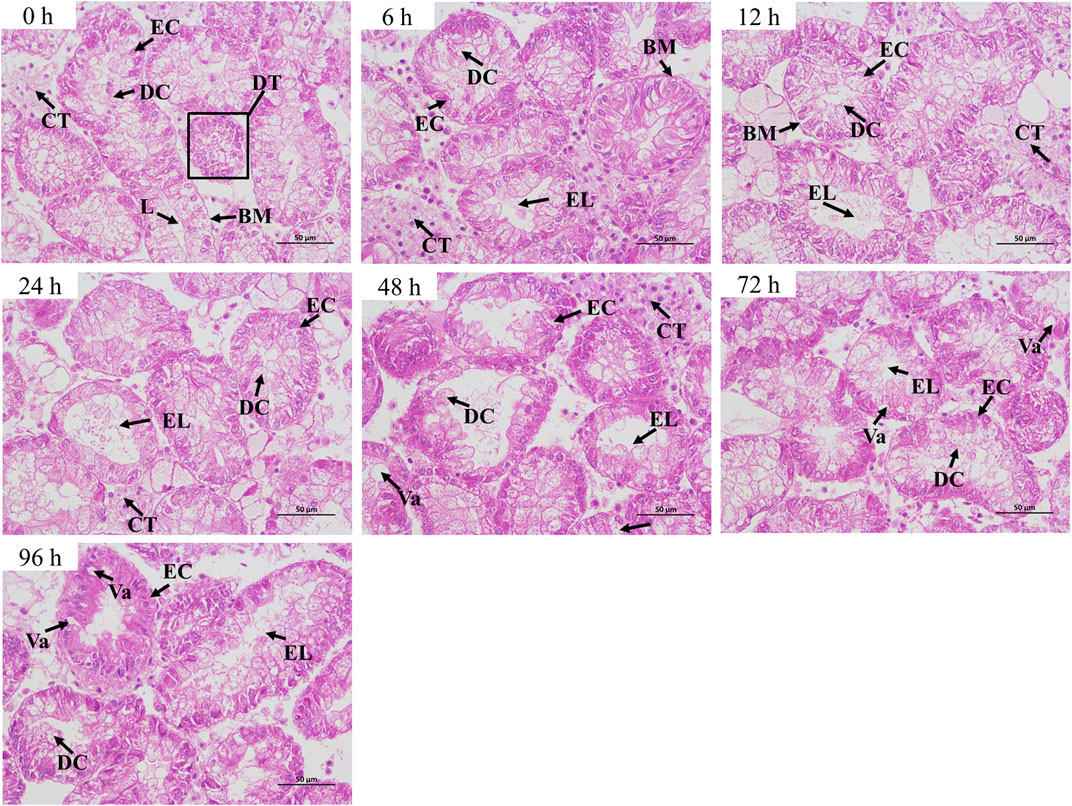
FIGURE 1. Histopathological changes in the hepatopancreas of C. fluminea under air exposure. DT, digestive tubule; L, lumen; EC, epithelial cell; DC, digestive cell; CT, connective tissue; BM, basement membrane; Va, vacuole; EL, enlargement of lumen.
Effects of air exposure on respiratory metabolism
The activity of HK increased significantly from 6 to 12 h of air exposure, then kept normal level from 24 to 48 h, and dropped remarkably from 72 to 96 h (Figure 2A). The activity of PK was significantly elevated from 12 to 24 h of air exposure and then came back to the control level during 48–96 h (Figure 2B). As shown in Figure 2C, the activity of SDH significantly decreased from 24 to 96 h after air exposure. LDH activity elevated significantly from 6 to 24 h of air exposure, and then returned to normal level during 48–96 h (Figure 2D).
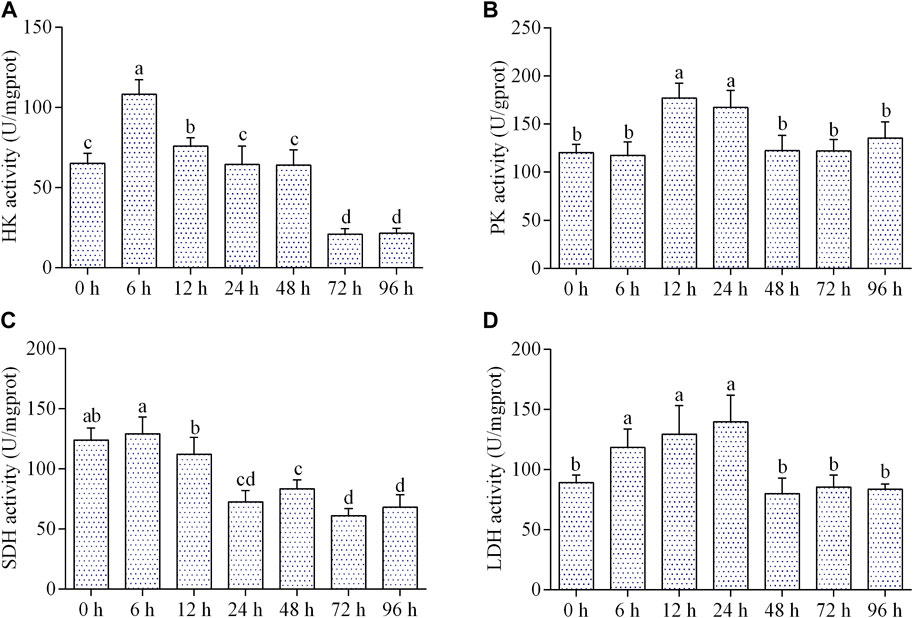
FIGURE 2. Effects of air exposure on respiratory metabolism. (A) HK activity (n = 5); (B) PK activity (n = 5); (C) SDH activity (n = 5); (D) LDH activity (n = 5). Data were expressed as mean ± SD. Different letters indicated statistical significance (p < 0.05).
Effects of air exposure on antioxidant and oxidative damage parameters
The SOD activity was significantly increased at 48 h of air exposure, and then returned to the control level from 72 to 96 h (Figure 3A). The CAT activity increased significantly during 6–12 h of air exposure, then returned to normal level from 24 to 48 h, and reduced markedly from 72 to 96 h (Figure 3B). The GSH content was increased between 12–24 h, and then come back to normal (Figure 3C). The content of MDA was remarkably increased after 96 h of air exposure, while did not change significantly at other time points (Figure 3D).
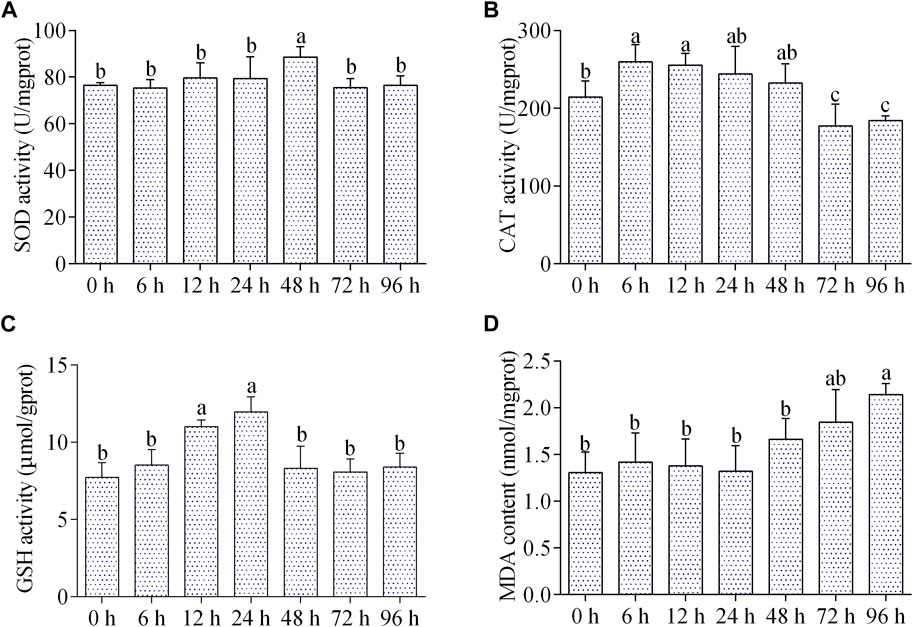
FIGURE 3. Effects of air exposure on antioxidant parameters in hepatopancreas. (A) SOD activity; (B) CAT activity; (C) GSH content; (D) MDA content. Data were expressed as mean ± SD (n = 5). Different letters indicated statistical significance (p < 0.05).
Effects of air exposure on immune enzyme activities
The ACP activity was significantly increased at 24 and 48 h of air exposure, and then significantly dropped at 96 h (Figure 4A). The activity of AKP increased markedly at 6 h of air exposure, and then maintained a control level from 12 to 96 h (Figure 4B).
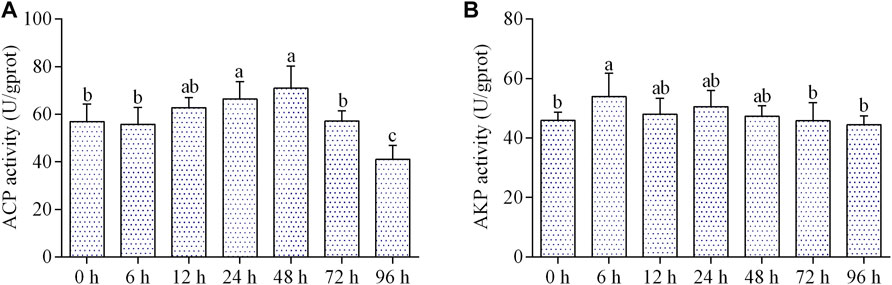
FIGURE 4. Effects of air exposure on immune parameters in hepatopancreas. (A) ACP activity; (B) AKP activity. Data were expressed as mean n ± SD (n = 5). Different letters indicated statistical significance (p < 0.05).
Effects of air exposure on transcriptomic
The transcriptome data were submitted to the SRA database (accession number: PRJNA817020). As shown in Table 1, a mean of 23,496,823 clean reads was obtained in all samples. The ranges of Q20 and Q30 were 98.16%–98.64% and 93.63%–94.94%, respectively. A total of 36,772 transcripts were assembled and 25,086 of them were annotated in six databases, including Uniprot, Pfam, GO, KEGG, KOG, and Nr (Table 2).
As shown in Figure 5A, principal component analysis (PCA) of transcript expressions showed that samples from the control and exposure groups were clustered separately, indicating significant differences between the two groups. A total of 44 DEGs were identified in the hepatopancreas after 96 h of air exposure, including 6 upregulated genes and 38 downregulated genes (Figure 5B). To verify the results of transcriptome analysis, 8 transcripts were randomly selected to perform qRT-PCR. Figure 5C showed similar change tendencies from RNA-seq and qRT-PCR, suggesting the reliable results of RNA-seq.
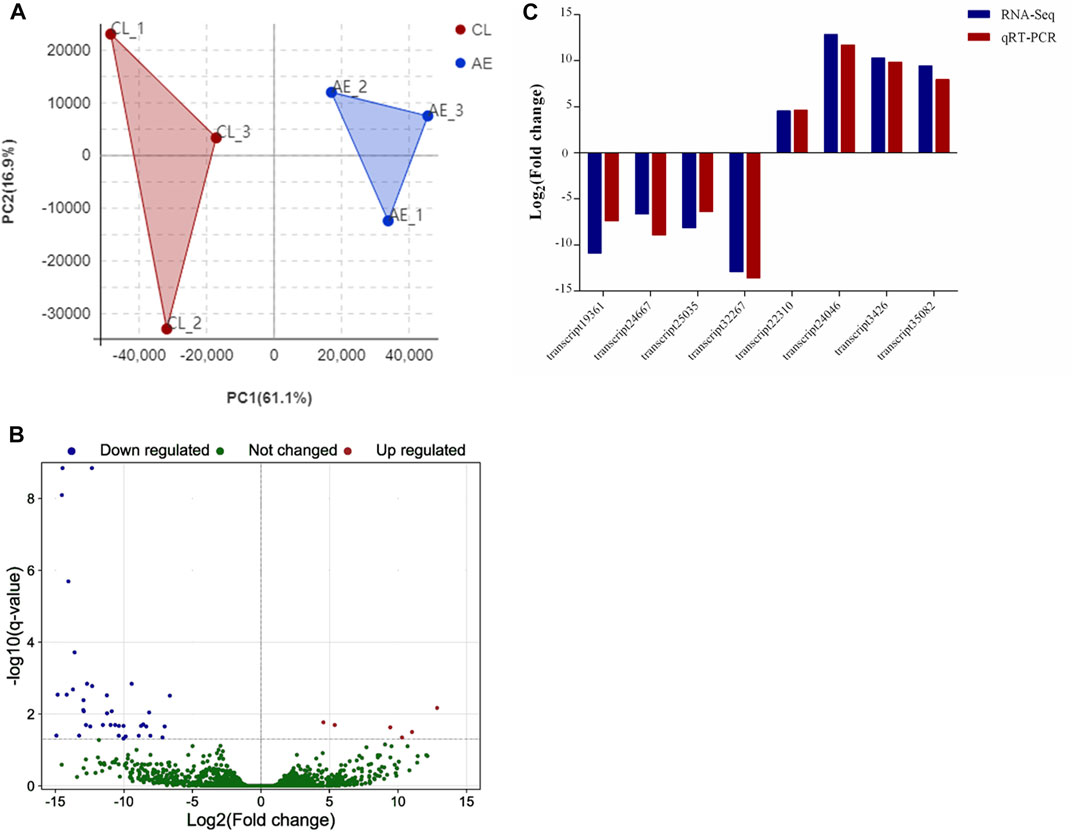
FIGURE 5. Identification and verification of DEGs. (A) PCA analysis of transcript expression in control (CL, 0 h) and air exposure groups (AE, 96 h); (B) Volcano map of DEGs; (C) Comparison of the relative fold change of RNA-Seq and qRT-PCR results.
The GO analysis was performed to understand the functional difference of DEGs. The results showed that DEGs were mainly enriched in the terms of intracellular non−membrane−bounded organelle and non−membrane−bounded organelle for cellular component ontology and the term of structural molecule activity for molecular function ontology (Supplementary Figure S1). Based on the KEGG pathways analysis, we found that DEGs were mainly enriched in pathways associated with the phagosome, pathogenic Escherichia coli infection, fluid shear stress and atherosclerosis, antigen processing and presentation, apoptosis, etc., (Figure 6). Eight DEGs involved in respiratory metabolism, immunity, and antioxidants were identified in this study and all of these genes were downregulated after 96-h air exposure (Table 3).
Discussions
Previous studies showed that air exposure can destroy the histological structures of many tissues, including gill, skin, intestines, and hepatopancreas, in aquatic animals (Lu et al., 2021; Harianto et al., 2022). In the current study, we observed the histopathological alterations of the hepatopancreas in C. fluminea under air exposure. We found that air exposure (6–96 h) caused the enlargement of the lumen and the vacuolation of the hepatopancreas. This finding indicated that air exposure induced tissue injury of the hepatopancreas in C. fluminea.
Respiration metabolism is the fundamental way for an organism to obtain energy. Aquatic animals usually perform aerobic respiration to provide energy for the organisms. However, when they undergo stress by adverse factors such as air exposure or hypoxia, they activate anaerobic respiration to adapt to the anoxic environment (Pihl et al., 1991; Ali and Nakamura, 2000). SDH is a key rate-limiting enzyme of aerobic respiration and is responsible for catalyzing the oxidation of succinic acid to fumaric acid and releasing ATP (Hägerhäll, 1997). In this study, the activity of SDH reduced from 24 h of air exposure. This may be due to that air exposure over 24-h resulted in cellular hypoxia in the hepatopancreas of C. fluminum, which suppressed electron transport chain and the rate of aerobic respiration metabolism. HK and PK are the two key rate-limiting enzymes of glycolysis. The former catalyzes glucose to glucose-6-phosphate and the latter catalyzes phosphoenolpyruvate to enolpyruvate (Cota-Ruiz et al., 2015). LDH is also a vital metabolism-regulating enzyme of anaerobic respiration and can catalyze pyruvate to lactic acid (Schurr and Payne, 2007). Previous studies have shown that the activities of LDH, HK, and PK can be altered by air exposure or hypoxia stress (Bao et al., 2019; Mattioli et al., 2019; Ding et al., 2020). In the present study, we found that the activities of HK, PK, and LDH increased first and then decreased during air exposure. Therefore, the increased activity of LDH in the 24 h indicated that anaerobic respiration in the hepatopancreas enhanced during early period of air exposure to provide additional energy for resistance to stress. However, the activity of LDH recovered to normal level during 48–96 h of air exposure. One reason might be that the structure and function of hepatopancreas of C. fluminea were impaired with prolonged air exposure, leading to inhibition of anaerobic respiration.
Under air exposure, aquatic animals may suffer hypoxia or anoxia stress, which will hinder the electron transport vector and promote the generation of ROS. When ROS exceeds the scavenging capacity of the antioxidant system, the disorder of the antioxidant system will occur, resulting in oxidative damage and even cell apoptosis of tissues (Pitkanen and Robinson, 1996). The effects of air exposure on the antioxidant system have been proven by growing evidence (Bao et al., 2019; Gao et al., 2021; Lu et al., 2021). SOD is one of the key enzymes in the antioxidant system and can catalyze superoxide ions into hydrogen peroxide (H2O2), which then is converted into water and oxygen by another antioxidant enzyme CAT (Martinez-Alvarez et al., 2005). GSH is an important non-enzymatic small molecule that exerts antioxidant effects by reacting with ROS or acting as a chain-breaker of free radical reactions (Xu et al., 2021). In this study, during 6–48 h of air exposure, SOD, CAT, and GSH content were increased, but recovered to normal or even decreased during 72–96 h. Furthermore, the content of MDA, a biomarker of oxidative lipid damage (Xu et al., 2020), was increased after 96 h of air exposure. These results demonstrated that short-term air exposure enhanced the antioxidant capacity of C. fluminea, but long-term air exposure caused lipid peroxidation damage in the hepatopancreas.
Like most invertebrates, the immune system of mollusks consists mainly of non-specific immunity that is sensitive to various environmental stresses, such as salinity shift and air exposure (Li et al., 2017; Zhang et al., 2021). ACP and AKP, two important hydrolases in lysosomes, are important in fighting disease and non-specific immune responses (Zhang et al., 2021). It has been reported that the activities of the two enzymes can be affected by air exposure in shellfish, such as Pinctada fucata, Chlamys farreri, and Argopecten irradians (Chen et al., 2007; Chi et al., 2017; Wei et al., 2021). In this study, we discovered that ACP and AKP activities were increased first (6–48 h) and then kept normal or even decreased during air exposure. Thus, our results suggested that at the early stage of air exposure, the non-specific immunity of C. fluminea was enhanced to resist stress, but the immune function was inhibited with the increase of exposure time.
Growing evidence has demonstrated that air exposure can induce changes in the expression of genes related to the glycolysis and tricarboxylic acid (TCA) cycle (Cheng et al., 2020; Zhou et al., 2021). In this study, the expressions of glyceraldehyde-3-phosphate dehydrogenase (GAPDH) and citrate synthase (CS) were downregulated after air exposure. GAPDH catalyzes the sixth step of glycolysis, converting glyceraldehyde 3-phosphate to D-glycolic acid 1, 3-diphosphate (Colell et al., 2009). CS catalyzes the condensation of oxaloacetic acid and acetyl-coA to produce citric acid, which is the key regulating step of the tricarboxylic acid (TCA) cycle (Roosterman and Cottrell, 2021). The downregulated expression of GAPDH and CS genes in this study indicated that 96 h of air exposure suppressed the glycolysis and TCA cycle of C. fluminea.
Heat shock proteins (HSPs) are a group of molecular chaperones and play important roles in various enverinemental stress responses (Junprung et al., 2017). Previous studies have shown that air exposure can influence the expressions of multiple subtypes of HSP genes, including HSP70, HSP90, and HSP21, in aquatic animals (Nie et al., 2020; Lu et al., 2021). In the current study, we found that the expressions of several HSP90 genes (HSP90aa1 and HSP90ab1) were downregulated after air exposure, revealing that HSP90 is closely related to the response of C. fluminea to air exposure. Glutathione peroxidase (Gpx) is a vital component in the antioxidant system and can reduce toxic peroxides to non-toxic hydroxyl compounds and promote the decomposition of H2O2 (Ighodaro and Akinloye, 2017). The expression of the GPx gene was upregulated by a short-term (8 h) air exposure (Lu et al., 2021). However, in this study, the expression of GPx-1 was decreased after 96 h of air exposure, suggesting inhibited the antioxidant capacity of C. fluminea. Ras-related C3 botulinum toxin substrate 1 protein (Rac1) is Rho family small GTPase and exerts crucial roles in phagocytosis, inflammation, and immune response to extracellular stimuli (Feng et al., 2019). Calreticulin (CARL) is a calcium-binding chaperone that takes part in many immune processes, including phagocytosis and antigen presentation (Raghavan et al., 2013). In this work, the downregulated expressions of Rac1 and CARL genes revealed suppressed immune function of C. fluminea by air exposure.
Conclusion
Overall, this study clarified the effects of air exposure on the histology, physiology as well as transcriptome of the hepatopancreas of C. fluminea for the first time. Detection of physiological indicators revealed that the respiratory metabolism, antioxidant capacity, and immune function of C. fluminea were enhanced to resist adversity under a short time (0–48 h) of air exposure, while these physiological indices were inhibited under a longer time (72–96 h) of air exposure. RNA-seq identified 8 DEGs related to glycolysis, TCA cycle, immune, and antioxidant, and all of the 8 DEGs were downregulated after air exposure. These findings will contribute to explaining the coping mechanism of C. fluminea and other freshwater shellfish to air exposure.
Data availability statement
The datasets presented in this study can be found in online repositories. The names of the repository/repositories and accession number(s) can be found below: https://www.ncbi.nlm.nih.gov/, PRJNA817020.
Ethics statement
The animal study was reviewed and approved by Animal Experiments Ethics Committee of Freshwater Fisheries Research Center, Chinese Academy of Fishery Sciences.
Author contributions
TZ, DX, and HW contributed to the conception of this study. TZ, DX, GL, and AW performed the experiment, collected samples, and completed index detection. TZ, DX, and GL contributed to data analysis. TZ wrote the manuscript. HW and AW reviewed and edited the manuscript.
Funding
This study was supported by the National Key R&D Program of China (Grant Number 2020YFD0900500) and the Central Public-interest Scientific Institution Basal Research Fund, CAFS (Grant Numbers 2020XT13, 2020TD61, and 2021JBFM17).
Conflict of interest
The authors declare that the research was conducted in the absence of any commercial or financial relationships that could be construed as a potential conflict of interest.
Publisher’s note
All claims expressed in this article are solely those of the authors and do not necessarily represent those of their affiliated organizations, or those of the publisher, the editors and the reviewers. Any product that may be evaluated in this article, or claim that may be made by its manufacturer, is not guaranteed or endorsed by the publisher.
Supplementary material
The Supplementary Material for this article can be found online at: https://www.frontiersin.org/articles/10.3389/fphys.2022.952744/full#supplementary-material
References
Abaychi J. K., Mustafa Y. Z. (1988). The asiatic clam, Corbicula fluminea: An indicator of trace metal pollution in the Shatt al-Arab River, Iraq. Environ. Pollut. 54, 109–122. doi:10.1016/0269-7491(88)90141-8
Ali F., Nakamura K. (2000). Metabolic characteristics of the Japanese clam Ruditapes philippinarum (Adams & Reeve) during aerial exposure. Aquac. Res. 31, 157–165. doi:10.1046/j.1365-2109.2000.00402.x
Arlinghaus R., Hallermann J. (2007). Effects of air exposure on mortality and growth of undersized pikeperch, Sander lucioperca, at low water temperatures with implications for catch-and-release fishing. Fish. Manag. Ecol. 14, 155–160. doi:10.1111/j.1365-2400.2007.00536.x
Bao J., Li X., Xing Y., Feng C., Jiang H. (2019). Respiratory metabolism and antioxidant response in Chinese mitten crab eriocheir sinensis during air exposure and subsequent reimmersion. Front. Physiol. 10, 907. doi:10.3389/fphys.2019.00907
Byrne R. A., Gnaiger E., McMahon R. F., Dietz T. H. (1990). Behavioral and metabolic responses to emersion and subsequent reimmersion in the freshwater bivalve, Corbicula fluminea. Biol. Bull. 178, 251–259. doi:10.2307/1541826
Chen M.-y., Yang H.-s., Delaporte M., Zhao S.-j., Xing K. (2007). Immune responses of the scallop Chlamys farreri after air exposure to different temperatures. J. Exp. Mar. Biol. Ecol. 345, 52–60. doi:10.1016/j.jembe.2007.01.007
Cheng C.-H., Ma H.-L., Deng Y.-Q., Feng J., Chen X.-L., Guo Z.-X. (2020). Transcriptome analysis and histopathology of the mud crab (Scylla paramamosain) after air exposure. Comp. Biochem. Physiol. C. Toxicol. Pharmacol. 228, 108652. doi:10.1016/j.cbpc.2019.108652
Chi C., Giri S. S., Jun J. W., Kim H. J., Kim S. W., Yun S., et al. (2017). Effects of algal toxin okadaic acid on the non-specific immune and antioxidant response of bay scallop (Argopecten irradians). Fish. Shellfish Immunol. 65, 111–117. doi:10.1016/j.fsi.2017.03.031
Colell A., Green D. R., Ricci J. E. (2009). Novel roles for GAPDH in cell death and carcinogenesis. Cell Death Differ. 16, 1573–1581. doi:10.1038/cdd.2009.137
Cota-Ruiz K., Peregrino-Uriarte A. B., Felix-Portillo M., Martínez-Quintana J. A., Yepiz-Plascencia G. (2015). Expression of fructose 1, 6-bisphosphatase and phosphofructokinase is induced in hepatopancreas of the white shrimp Litopenaeus vannamei by hypoxia. Mar. Environ. Res. 106, 1–9. doi:10.1016/j.marenvres.2015.02.003
Ding J., Liu C., Luo S., Zhang Y., Gao X., Wu X., et al. (2020). Transcriptome and physiology analysis identify key metabolic changes in the liver of the large yellow croaker (Larimichthys crocea) in response to acute hypoxia. Ecotoxicol. Environ. Saf. 189, 109957. doi:10.1016/j.ecoenv.2019.109957
Feng Y., Ma M., Zhang X., Liu D., Wang L., Qian C., et al. (2019). Characterization of small GTPase Rac1 and its interaction with PAK1 in crayfish Procambarus clarkii. Fish. Shellfish Immunol. 87, 178–183. doi:10.1016/j.fsi.2019.01.013
Gao J., Xu G., Xu P. (2021). Effect of addition of salt on oxidant activity and apoptosis of Coilia nasus juveniles under air exposure stress. Aquac. Rep. 20, 100696. doi:10.1016/j.aqrep.2021.100696
Gu W.-B., Zhou Z.-K., Tu D.-D., Zhou Y.-L., Shu M.-A. (2017). Molecular cloning and characterization of an inhibitor of apoptosis protein in the mud crab Scylla paramamosain: Its potential anti-apoptosis role under air exposure stress. Fish. Sci. 83, 715–723. doi:10.1007/s12562-017-1103-3
Hägerhäll C. (1997). Succinate: Quinone oxidoreductases. Variations on a conserved theme. Biochim. Biophys. Acta 1320, 107–141. doi:10.1016/s0005-2728(97)00019-4
Harianto E., Supriyono E., Budiardi T., Affandi R., Hadiroseyani Y. (2022). Gill and skin oxygen uptake, biochemical, hematological, and histological responses of eel (Anguilla bicolor bicolor) exposed to air. Turkish J. Fish. Aquatic Sci. 22, TRJFAS19989. doi:10.4194/TRJFAS19989
Ighodaro O. M., Akinloye O. A. (2017). First line defence antioxidants-superoxide dismutase (SOD), catalase (CAT) and glutathione peroxidase (GPX): Their fundamental role in the entire antioxidant defence grid. Alexandria J. Med. 54, 287–293. doi:10.1016/j.ajme.2017.09.001
Junprung W., Supungul P., Tassanakajon A. (2017). HSP70 and HSP90 are involved in shrimp Penaeus vannamei tolerance to AHPND-causing strain of Vibrio parahaemolyticus after non-lethal heat shock. Fish. Shellfish Immunol. 60, 237–246. doi:10.1016/j.fsi.2016.11.049
Li Y. Y., Chen X. X., Lin F. Y., Chen Q. F., Ma X. Y., Liu H. P. (2017). CqToll participates in antiviral response against white spot syndrome virus via induction of anti-lipopolysaccharide factor in red claw crayfish Cherax quadricarinatus. Dev. Comp. Immunol. 74, 217–226. doi:10.1016/j.dci.2017.04.020
Liao N., Chen S., Ye X., Zhong J., Wu N., Dong S., et al. (2013). Antioxidant and anti-tumor activity of a polysaccharide from freshwater clam, Corbicula fluminea. Food Funct. 4, 539–548. doi:10.1039/c2fo30178d
Lu Y.-P., Zhang X.-X., Zheng P.-H., Zhang Z.-L., Li J.-T., Wang D.-M., et al. (2021). Effects of air exposure on survival, histological structure, non-specific immunity and gene expression of red claw crayfish (Cherax quadricarinatus). Aquac. Rep. 21, 100898. doi:10.1016/j.aqrep.2021.100898
Martinez-Alvarez R. M., Morales A. E., Sanz A. (2005). Antioxidant defenses in fish: Biotic and abiotic factors. Rev. Fish. Biol. Fish. 15, 75–88. doi:10.1007/s11160-005-7846-4
Mattioli C. C., Takata R., de Oliveira Paes Leme F., Costa D. C., Luz R. K. (2019). Physiological and metabolic responses of juvenile Lophiosilurus alexandri catfish to air exposure. Fish. Physiol. Biochem. 45, 455–467. doi:10.1007/s10695-018-0576-z
Meng J., Wang T., Li L., Zhang G. F. (2018). Inducible variation in anaerobic energy metabolism reflects hypoxia tolerance across the intertidal and subtidal distribution of the Pacific oyster (Crassostrea gigas). Mar. Environ. Res. 138, 135–143. doi:10.1016/j.marenvres.2018.04.002
Mi K. H., Chen X., Lu K. Y., Zhu Y. J., Zhang M., Yang H., et al. (2021). Bisphenol A induces hepatic triglyceride level in adult male rare minnow Gobiocypris rarus. Ecotoxicol. Environ. Saf. 213, 112050. doi:10.1016/j.ecoenv.2021.112050
Nie H., Jiang K., Li N., Li D., Yan X. (2020). Transcriptomic analysis of Ruditapes philippinarum under aerial exposure and reimmersion reveals genes involved in stress response and recovery capacity of the Manila clam. Aquaculture 524, 735271. doi:10.1016/j.aquaculture.2020.735271
Pihl L., Baden S. P., Diaz R. J. (1991). Effects of periodic hypoxia on distribution of demersal fish and crustaceans. Mar. Biol. 108, 349–360. doi:10.1007/bf01313644
Pitkanen S., Robinson B. H. (1996). Mitochondrial complex I deficiency leads to increased production of superoxide radicals and induction of superoxide dismutase. J. Clin. Invest. 98, 345–351. doi:10.1172/JCI118798
Raghavan M., Wijeyesakere S. J., Peters L. R., Del Cid N. (2013). Calreticulin in the immune system: Ins and outs. Trends Immunol. 34, 13–21. doi:10.1016/j.it.2012.08.002
Romero M. C., Ansaldo M., Lovrich G. A. (2007). Effect of aerial exposure on the antioxidant status in the subantarctic stone crab Paralomis granulosa (Decapoda: Anomura). Comp. Biochem. Physiol. C. Toxicol. Pharmacol. 146, 54–59. doi:10.1016/j.cbpc.2006.06.009
Roosterman D., Cottrell G. S. (2021). Rethinking the citric acid cycle: Connecting pyruvate carboxylase and citrate synthase to the flow of energy and material. Int. J. Mol. Sci. 22, 604. doi:10.3390/ijms22020604
Rosa I. C., Pereira J. L., Gomes J., Saraiva P. M., Goncalves F., Costa R. (2011). The asian clam Corbicula fluminea in the European freshwater-dependent industry: A latent threat or a friendly enemy? Ecol. Econ. 70, 1805–1813. doi:10.1016/j.ecolecon.2011.05.006
Schurr A., Payne R. S. (2007). Lactate, not pyruvate, is neuronal aerobic glycolysis end product: An in vitro electrophysiological study. Neuroscience 147, 613–619. doi:10.1016/j.neuroscience.2007.05.002
Wei H., Deng Z., Zhao W., Liao M., Li Y., Yu G., et al. (2021). Effects of air exposure at different temperatures in selected breeding lines of Pinctada fucata with different shell color. Aquac. Rep. 21, 100879. doi:10.1016/j.aqrep.2021.100879
Xu Y.-H., Hogstrand C., Xu Y.-C., Zhao T., Zheng H., Luo Z. (2021). Environmentally relevant concentrations of oxytetracycline and copper increased liver lipid deposition through inducing oxidative stress and mitochondria dysfunction in grass carp Ctenopharyngodon idella. Environ. Pollut. 283, 117079. doi:10.1016/j.envpol.2021.117079
Xu Y.-H., Xu Y.-C., Hogstrand C., Zhao T., Wu L.-X., Zhuo M.-Q., et al. (2020). Waterborne copper exposure up-regulated lipid deposition through the methylation of GRP78 and PGC1α of grass carp Ctenopharyngodon idella. Ecotoxicol. Environ. Saf. 205, 111089. doi:10.1016/j.ecoenv.2020.111089
Yin X., Chen P., Chen H., Jin W., Yan X. (2017). Physiological performance of the intertidal Manila clam (Ruditapes philippinarum) to long-term daily rhythms of air exposure. Sci. Rep. 7, 41648. doi:10.1038/srep41648
Zhang T., Yao J., Xu D., Ma X., Jin W., Lv G., et al. (2021). Gill physiological and transcriptomic response of the threatened freshwater mussel Solenaia oleivora to salinity shift. Comp. Biochem. Physiol. Part D. Genomics Proteomics 40, 100913. doi:10.1016/j.cbd.2021.100913
Keywords: C. fluminea, air exposure, histology, physiology, transcriptome
Citation: Zhang T, Xu D, Lv G, Wang A and Wen H (2022) Histological, physiological, and transcriptomic responses of hepatopancreas to air exposure in asian freshwater clam Corbicula fluminea. Front. Physiol. 13:952744. doi: 10.3389/fphys.2022.952744
Received: 25 May 2022; Accepted: 18 July 2022;
Published: 11 August 2022.
Edited by:
Alaa El-Din Hamid Sayed, Assiut University, EgyptReviewed by:
Adriana Rios Lopes, Butantan Institute, BrazilAli R. Bandani, University of Tehran, Iran
Copyright © 2022 Zhang, Xu, Lv, Wang and Wen. This is an open-access article distributed under the terms of the Creative Commons Attribution License (CC BY). The use, distribution or reproduction in other forums is permitted, provided the original author(s) and the copyright owner(s) are credited and that the original publication in this journal is cited, in accordance with accepted academic practice. No use, distribution or reproduction is permitted which does not comply with these terms.
*Correspondence: Haibo Wen, wenhb@ffrc.cn