- 1Institut universitaire de cardiologie et de pneumologie de Québec, Université Laval, Québec, QC, Canada
- 2Centre hospitalier universitaire de Québec, Université Laval, Québec, QC, Canada
Background: Peak oxygen uptake (
Objectives: We evaluated whether peak
Methods: A retrospective analysis of patients who underwent VATS lung resection between 2002 and 2019 and in whom CPET was performed. Logistic regression models were used to determine predictors of postoperative outcomes until 30 days after surgery. The ability of peak
Results: Among the 593 patients, postoperative cardiopulmonary complications occurred in 92 (15.5%) individuals, including three deaths. Mean peak
Conclusion: Although lower peak
Introduction
Lung cancer is currently the leading cause of death by cancer (Cancer IAfRo, 2019). Although surgery still offers the best chance to cure early stage non-small-cell lung cancer (Howington et al., 2013), pre-operative assessment and stratification of lung resection candidates is a crucial step to minimize surgical risks as this population is frequently older and comorbid (Sekine et al., 2002; Kravchenko et al., 2015).
Cardiopulmonary exercise testing (CPET) has played a central role in the evaluation of fitness of lung resection candidates. This is particularly true in patients with impaired lung function in whom various peak oxygen uptake (peak
An important issue with this practice is that peak
The aim of this study was to assess if clinical and physiological variables, including peak
Methods
Subjects and study design
This is a retrospective analysis of a prospectively collected research database of all patients with a resectable lung cancer who performed CPET as part of their preoperative medical evaluation at the Institut universitaire de cardiologie et de pneumologie de Québec (IUCPQ), Québec, Canada. Patients who underwent VATS lung cancer surgery from 1 January 2002 to 31 December 2019 were included on a first occurrence of a non-small-cell lung cancer without prior thoracic surgery or radiotherapy and if they had pulmonary function testing and CPET data available. Surgeries were performed by thoracic surgeons at the IUCPQ where VATS progressively became the preferred approach. In 2005, VATS represented 25% of all lung cancer resections compared to 88% in 2019. CPET was also requested by the surgeons based on their clinical judgment that further testing was necessary to complete surgical risk assessment. When lung resection was considered as a potential therapeutic option, the following situations would generally trigger a CPET: forced expiratory volume in 1 s (FEV1) < 80% predicted, age > 65 years, the presence of one or more comorbidities, and the possibility of lung resection more extensive than a lobectomy. All study participants provided informed consent and gave written approval for the use of their clinical data in subsequent research publication (CER 21184).
The primary outcome of the study was cardiopulmonary complications occurring during the 30-day period following the surgery. Baseline characteristics, pulmonary function tests, CPET, and 30-days post-operative cardiopulmonary complications and mortality were retrieved from the research database and review of the medical chart, when necessary. Age, sex, body mass index (BMI), smoking history, and extent of resection (pneumonectomy, bilobectomy, lobectomy, sublobar resection) were noted. The following comorbid conditions were documented: coronary artery disease, peripheral artery disease, hypertension, diabetes, chronic renal failure, and chronic obstructive pulmonary disease (COPD). COPD diagnosis was based on the presence of symptoms related to COPD, spirometry showing a post-bronchodilator FEV1 to forced vital capacity (FVC) ratio < 0.70, and a smoking history of at least 10 pack-years (Vogelmeier et al., 2017). The Thoracic Revised Cardiac Risk Index (ThRCRI) was calculated for each patient (Brunelli et al., 2010). This index provides a summary score that stratifies patients into four classes, from A to D, with a progressively higher risk of post-operative cardiovascular complications.
Pulmonary function tests, including spirometry, lung volumes, and carbon monoxide diffusion capacity were performed according to previous guidelines and related to predicted normal values (Macintyre et al., 2005; Miller et al., 2005; Wanger et al., 2005). CPET results were reviewed and then adjudicated by a second author (GC, AL, and PR). Patients with CPET judged to be non-maximal according to the American College of Sports Medicine statement on CPET (Swain et al., 2000) were excluded. Briefly, when CPET was stopped before the predicted peak
Grade II or more post-operative cardiopulmonary complications on the Clavien-Dindo (Dindo et al., 2004) classification scheme (those requiring specific interventions beyond antipyretics, antiemetic, and analgesic) were recorded and definitions were based on the Society of Thoracic Surgeons and European Society of Thoracic Surgeons joint statement (Dindo et al., 2004; Fernandez et al., 2015). The following cardiopulmonary complications were recorded: need for mechanical ventilation, pneumonia, acute respiratory distress syndrome (ARDS), atelectasis, acute coronary syndrome, arrythmia, heart failure, venous thromboembolism, stroke, and acute renal failure.
Cardiopulmonary exercise testing
CPET consisted in an incremental cycling exercise performed on a cycle ergometer, until exhaustion and were performed at the clinical exercise laboratory of our institution. After 1 minute of resting on the cycle ergometer, work rate was increased in a ramp protocol using of 10- to 20-W per minute increments, with the objective of having an exercise duration of 8–10 min based on predicted peak work rate.
Statistical analysis
Results are reported as mean ± SD for continuous variables and number or % for nominal variables. Between-group comparisons were performed using the Student’s t-test for continuous variables and Chi-Square or Fisher’s exact test for nominal variables. We conducted univariable logistic regression analyses using potential predictors of post-surgical outcomes (age, sex, comorbid conditions, ThRCRI class C and D, extent of lung resection, FEV1% predicted, FVC % predicted, FEV1/FVC, total lung capacity % predicted, residual volume % predicted, carbon monoxide diffusion capacity [DLCO] % predicted, peak values for
Results
Baseline characteristics of the study participants are summarized in Table 1. From 1 January 2002 to 31 December 2019, 2,228 patients underwent lung cancer resection by VATS at our institution. The study population included the 593 patients who performed a CPET pre-operatively, representing 27% of the entire VATS population. The study population included 264 men (44.5%), mean age was 66.8 years and BMI averaged 26.9 kg/m2. COPD, hypertension, coronary artery disease, and diabetes were the most frequent comorbid conditions. Mean peak work rate was 91.4% of predicted and mean peak
The overall distribution of postoperative cardiopulmonary complications according to the extent of lung resection is summarized in Table 2. Overall, 92 (15.5%) patients experienced one or more of the 130 cardiopulmonary complications within 30 days of the operation, including three deaths (0.5%), two from acute respiratory distress syndrome complicating a bronchopleural fistula and one from an acute exacerbation of pulmonary fibrosis. The most frequent complications were pneumonia (5.9%) and arrhythmias (6.8%). A larger proportion of patients who underwent bilobectomy and pneumonectomy experienced post-operative complications compared to those with less extensive resection.
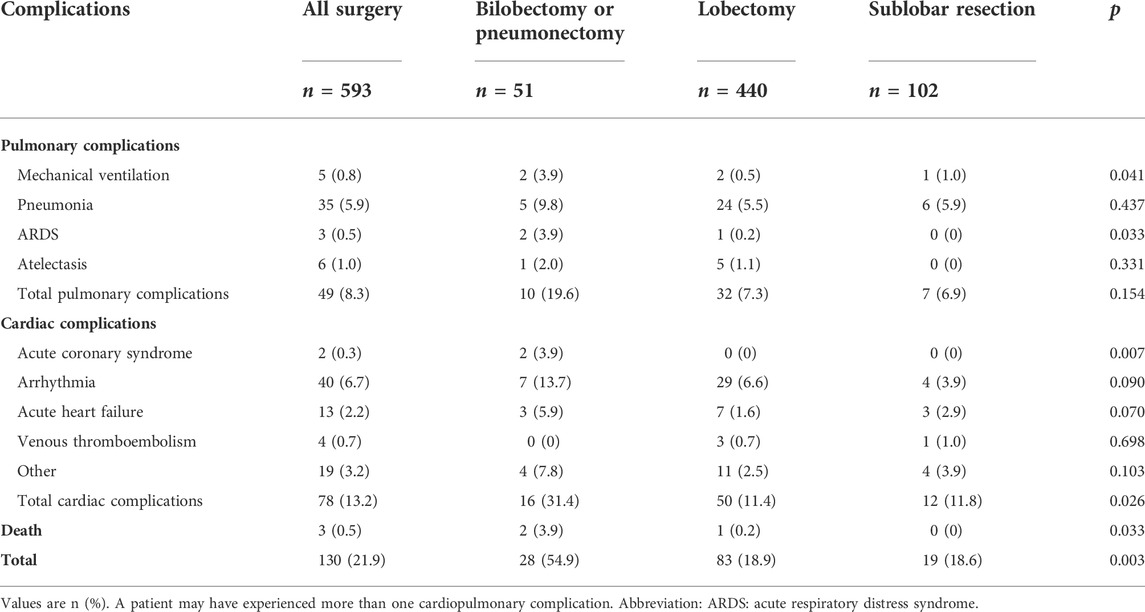
TABLE 2. Number of post-operative cardiopulmonary complications within 30 days of surgery according to the extent of lung resection (n = 593).
Comparisons between patients with at least one post-operative cardiopulmonary complications and those who experienced an uneventful surgery are provided in Table 1. Age, peripheral artery disease, hypertension, ThRCRI ≥ 2 (class C or D), and bilobectomy or pneumonectomy were associated with complications. FEV1, FVC and DLCO were lower in patients with complications. Peak
As reported in Table 3, the best predictors of post-operative mortality and morbidity in the multivariable analysis were peripheral artery disease (odds ratio [OR] = 1.90, 95% CI: 1.08–3.37), bilobectomy (OR = 3.44, 95%CI: 1.20 to 9.85 versus sublobar resection), pneumonectomy (OR = 10.32, 95%CI 3.29 to 32.35 versus sublobar resection), FEV1% predicted (OR = 0.98 per unit, 95%CI: 0.97–1.0), peak
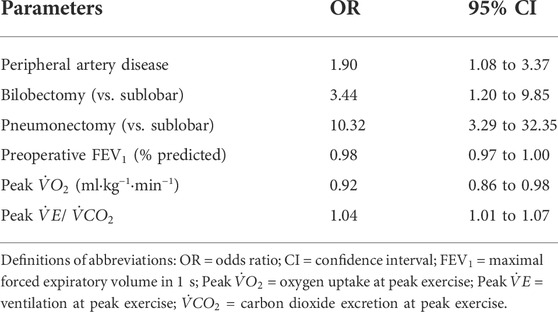
TABLE 3. Best predictors of post-operative complications based on the multivariable regression model.
To further explore possible relationships between peak
The ROC curve for peak
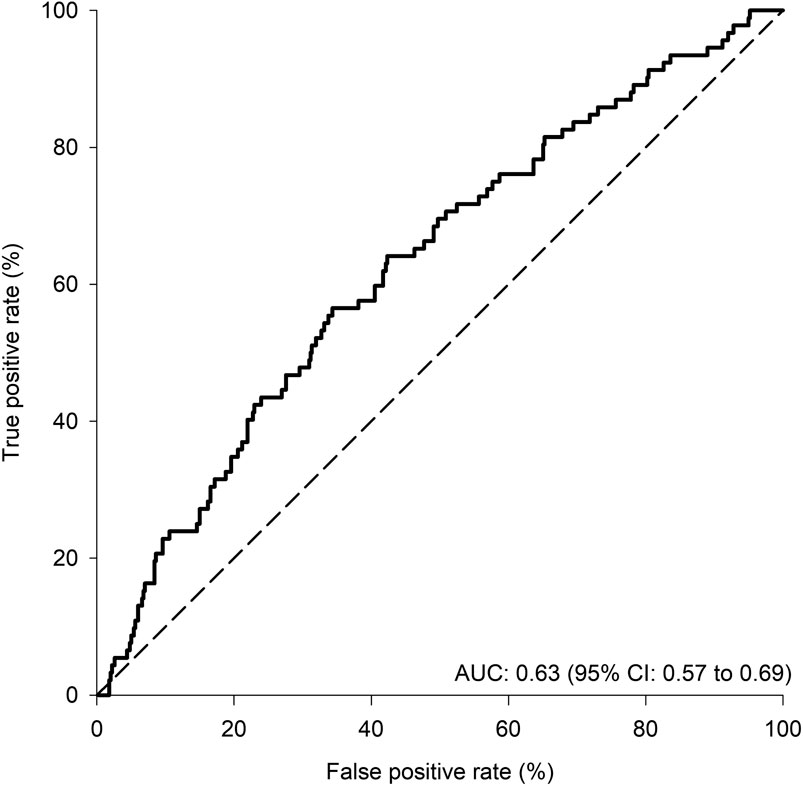
FIGURE 1. Receiver operating characteristic (ROC) curve for peak
The ability of peak
Discussion
Based on the consistent observation that peak
The past 20 years has witnessed a consistent trend in better post-operative outcome after lung cancer resection, with 30-day post-operative mortality decreasing from 3–5% to 1% (Morgant et al., 2015; Shewale et al., 2020). Improved outcome following lung resection is related to the use of minimally-invasive operative techniques that are associated with less complications than thoracotomy (Boffa et al., 2014; Laursen et al., 2016), but also to improved patient selection, anesthetic techniques, and general postoperative medical management (Morgant et al., 2015; Shewale et al., 2020). Although it is difficult to compare post-operative outcome across studies due to differences in patient populations and in how post-operative complications are documented and defined, our results are consistent with the published experience with VATS lung cancer resection (Boffa et al., 2014; Begum et al., 2016; Shewale et al., 2020).
Considering the lower complication rates that is now observed after lung resection, a lower discriminative ability could be expected from preoperative evaluating tests. This has been suggested by Berry et al who reported that pulmonary function tests lose their predictive ability for pulmonary complications in patients who underwent thoracoscopy for lung resection (Berry et al., 2010). Nonetheless, our results show that despite relatively low mortality and morbidity rates of 0.5 and 15.5%, respectively, peak
Besides peak
Strengths of the current study include its large sample size of patients who underwent VATS lung resection and in whom comprehensive CPET data were available. All exercise tests were carried out in the same clinical exercise laboratory and their validity was confirmed by three investigators. We acknowledge that our study has several limitations including its retrospective nature. To minimize the possibility of under reporting complications, we relied on a research database where clinical data is entered prospectively, and this was complemented by the review of medical charts, when necessary. Because only four patients with peak
Conclusion
Although peak
Data availability statement
The raw data supporting the conclusions of this article will be made available anonymously on request without undue reservation.
Ethics statement
The studies involving human participants were reviewed and approved by Comité d'éthique de l'Institut universitaire de cardiologie et de pneumologie de Québec. The patients/participants provided their written informed consent to participate in this study.
Author contributions
GC, PR, AL, EM, MM, YL, and FM conceived and designed the work. All authors were involved in data acquisition. GC, PR, AL, EM, YL, and FM analyzed the data and drafted the work. All authors contributed to critical reading of the drafted manuscript, to the completion of the final document, and to its approval. GC, PR, YL, and FM are accountable for all aspects of the work and to the accuracy and integrity of the work.
Funding
This work was supported by local research funds held by YL and FM.
Acknowledgments
The authors would like to thank the team at the IUCPQ site of the Quebec Respiratory Health Network Tissue Bank for their valuable assistance and Serge Simard for the statistical analyses.
Conflict of interest
The authors declare that the research was conducted in the absence of any commercial or financial relationships that could be construed as a potential conflict of interest.
Publisher’s note
All claims expressed in this article are solely those of the authors and do not necessarily represent those of their affiliated organizations, or those of the publisher, the editors and the reviewers. Any product that may be evaluated in this article, or claim that may be made by its manufacturer, is not guaranteed or endorsed by the publisher.
References
Begum S. S., Papagiannopoulos K., Falcoz P. E., Decaluwe H., Salati M., Brunelli A. (2016). Outcome after video-assisted thoracoscopic surgery and open pulmonary lobectomy in patients with low VO2 max: A case-matched analysis from the ests database. Eur. J. Cardiothorac. Surg. 49, 1054–1058. discussion 1058. doi:10.1093/ejcts/ezv378
Benzo R., Kelley G. A., Recchi L., Hofman A., Sciurba F. (2007). Complications of lung resection and exercise capacity: A meta-analysis. Respir. Med. 101, 1790–1797. doi:10.1016/j.rmed.2007.02.012
Berry M. F., Villamizar-Ortiz N. R., Tong B. C., Burfeind W. R., Harpole D. H., D'Amico T. A., et al. (2010). Pulmonary function tests do not predict pulmonary complications after thoracoscopic lobectomy. Ann. Thorac. Surg. 89, 1044–1051. discussion 1051-1042. doi:10.1016/j.athoracsur.2009.12.065
Boffa D. J., Dhamija A., Kosinski A. S., Kim A. W., Detterbeck F. C., Mitchell J. D., et al. (2014). Fewer complications result from a video-assisted approach to anatomic resection of clinical stage I lung cancer. J. Thorac. Cardiovasc. Surg. 148, 637–643. doi:10.1016/j.jtcvs.2013.12.045
Bolliger C. T., Jordan P., Soler M., Stulz P., Gradel E., Skarvan K., et al. (1995). Exercise capacity as a predictor of postoperative complications in lung resection candidates. Am. J. Respir. Crit. Care Med. 151, 1472–1480. doi:10.1164/ajrccm.151.5.7735602
Brunelli A., Belardinelli R., Pompili C., Xiume F., Refai M., Salati M., et al. (2012). Minute ventilation-to-carbon dioxide output (VE/VCO2) slope is the strongest predictor of respiratory complications and death after pulmonary resection. Ann. Thorac. Surg. 93, 1802–1806. doi:10.1016/j.athoracsur.2012.03.022
Brunelli A., Belardinelli R., Refai M., Salati M., Socci L., Pompili C., et al. (2009). Peak oxygen consumption during cardiopulmonary exercise test improves risk stratification in candidates to major lung resection. Chest 135, 1260–1267. doi:10.1378/chest.08-2059
Brunelli A., Charloux A., Bolliger C. T., Rocco G., Sculier J. P., Varela G., et al. (2009). ERS/ESTS clinical guidelines on fitness for radical therapy in lung cancer patients (surgery and chemo-radiotherapy). Eur. Respir. J. 34, 17–41. doi:10.1183/09031936.00184308
Brunelli A., Kim A. W., Berger K. I., Addrizzo-Harris D. J. (2013). Physiologic evaluation of the patient with lung cancer being considered for resectional surgery: Diagnosis and management of lung cancer, 3rd ed: American College of Chest Physicians evidence-based clinical practice guidelines. Chest 143, e166S–e190S. doi:10.1378/chest.12-2395
Brunelli A., Varela G., Salati M., Jimenez M. F., Pompili C., Novoa N., et al. (2010). Recalibration of the revised cardiac risk index in lung resection candidates. Ann. Thorac. Surg. 90, 199–203. doi:10.1016/j.athoracsur.2010.03.042
Brutsche M. H., Spiliopoulos A., Bolliger C. T., Licker M., Frey J. G., Tschopp J. M. (2000). Exercise capacity and extent of resection as predictors of surgical risk in lung cancer. Eur. Respir. J. 15, 828–832. doi:10.1034/j.1399-3003.2000.15e03.x
Dindo D., Demartines N., Clavien P. A. (2004). Classification of surgical complications: A new proposal with evaluation in a cohort of 6336 patients and results of a survey. Ann. Surg. 240, 205–213. doi:10.1097/01.sla.0000133083.54934.ae
Fang Y., Ma G., Lou N., Liao W., Wang D. (2014). Preoperative maximal oxygen uptake and exercise-induced changes in pulse oximetry predict early postoperative respiratory complications in lung cancer patients. Scand. J. Surg. 103, 201–208. doi:10.1177/1457496913509235
Fernandez F. G., Falcoz P. E., Kozower B. D., Salati M., Wright C. D., Brunelli A. (2015). The Society of Thoracic Surgeons and the European Society of Thoracic Surgeons general thoracic surgery databases: Joint standardization of variable definitions and terminology. Ann. Thorac. Surg. 99, 368–376. doi:10.1016/j.athoracsur.2014.05.104
Howington J. A., Blum M. G., Chang A. C., Balekian A. A., Murthy S. C. (2013). Treatment of stage i and ii non-small cell lung cancer: Diagnosis and management of lung cancer, 3rd ed: American College of Chest Physicians evidence-based clinical practice guidelines. Chest 143, e278S–e313S. doi:10.1378/chest.12-2359
Kravchenko J., Berry M., Arbeev K., Lyerly H. K., Yashin A., Akushevich I. (2015). Cardiovascular comorbidities and survival of lung cancer patients: Medicare data based analysis. Lung Cancer 88, 85–93. doi:10.1016/j.lungcan.2015.01.006
Laursen L. O., Petersen R. H., Hansen H. J., Jensen T. K., Ravn J., Konge L. (2016). Video-assisted thoracoscopic surgery lobectomy for lung cancer is associated with a lower 30-day morbidity compared with lobectomy by thoracotomy. Eur. J. Cardiothorac. Surg. 49, 870–875. doi:10.1093/ejcts/ezv205
Loewen G. M., Watson D., Kohman L., Herndon J. E. 2nd, Shennib H., Kernstine K., et al. (2007). Preoperative exercise VO2 measurement for lung resection candidates: Results of cancer and leukemia group b protocol 9238. J. Thorac. Oncol. 2, 619–625. doi:10.1097/JTO.0b013e318074bba7
Macintyre N., Crapo R. O., Viegi G., Johnson D. C., van der Grinten C. P., Brusasco V., et al. (2005). Standardisation of the single-breath determination of carbon monoxide uptake in the lung. Eur. Respir. J. 26, 720–735. doi:10.1183/09031936.05.00034905
Mandrekar J. N. (2010). Receiver operating characteristic curve in diagnostic test assessment. J. Thorac. Oncol. 5, 1315–1316. doi:10.1097/JTO.0b013e3181ec173d
Miller M. R., Hankinson J., Brusasco V., Burgos F., Casaburi R., Coates A., et al. (2005). Standardisation of spirometry. Eur. Respir. J. 26, 319–338. doi:10.1183/09031936.05.00034805
Morgant M. C., Pages P. B., Orsini B., Falcoz P. E., Thomas P. A., Barthes Fle P., et al. (2015). Time trends in surgery for lung cancer in France from 2005 to 2012: A nationwide study. Eur. Respir. J. 46, 1131–1139. doi:10.1183/13993003.00354-2015
Paul S., Sedrakyan A., Chiu Y. L., Nasar A., Port J. L., Lee P. C., et al. (2013). Outcomes after lobectomy using thoracoscopy vs thoracotomy: A comparative effectiveness analysis utilizing the nationwide inpatient sample database. Eur. J. Cardiothorac. Surg. 43, 813–817. doi:10.1093/ejcts/ezs428
Rodrigues F., Grafino M., Faria I., Pontes da Mata J., Papoila A. L., Felix F. (2016). Surgical risk evaluation of lung cancer in COPD patients - a cohort observational study. Rev. Port. Pneumol. 22, 266–272. doi:10.1016/j.rppnen.2016.02.010
Sekine Y., Behnia M., Fujisawa T. (2002). Impact of COPD on pulmonary complications and on long-term survival of patients undergoing surgery for NSCLC. Lung Cancer 37, 95–101. doi:10.1016/s0169-5002(02)00014-4
Shewale J. B., Correa A. M., Brown E. L., Leon-Novelo L. G., Nyitray A. G., Antonoff M. B., et al. (2020). Time trends of perioperative outcomes in early stage non-small cell lung cancer resection patients. Ann. Thorac. Surg. 109, 404–411. doi:10.1016/j.athoracsur.2019.08.018
Swain D. P., Brawne. C. A., American College of Sports M., Williams & Wilkins L. (2000). ACSM's guidelines for exercise testing and prescription. Philadelphia.
Torchio R., Guglielmo M., Giardino R., Ardissone F., Ciacco C., Gulotta C., et al. (2010). Exercise ventilatory inefficiency and mortality in patients with chronic obstructive pulmonary disease undergoing surgery for non-small-cell lung cancer. Eur. J. Cardiothorac. Surg. 38, 14–19. doi:10.1016/j.ejcts.2010.01.032
Vogelmeier C. F., Criner G. J., Martinez F. J., Anzueto A., Barnes P. J., Bourbeau J., et al. (2017). Global strategy for the diagnosis, management, and prevention of chronic obstructive lung disease 2017 report. GOLD Executive Summary. Am. J. Respir. Crit. Care Med. 195, 557–582. doi:10.1164/rccm.201701-0218PP
Wanger J., Clausen J. L., Coates A., Pedersen O. F., Brusasco V., Burgos F., et al. (2005). Standardisation of the measurement of lung volumes. Eur. Respir. J. 26, 511–522. doi:10.1183/09031936.05.00035005
Keywords: post-operative outcomes, cardiopulmonary exercise, peak oxygen consumption (peak VO2), thoracoscopy (VATS), lung cancer, lung resection
Citation: Chouinard G, Roy P, Blais M-C, Lippens A, Pelletier É, Roy E, Marcoux M, Ugalde PA, Rheault J, Pigeon M-A, Nicodème F, Lacasse Y and Maltais F (2022) Exercise testing and postoperative complications after minimally invasive lung resection: A cohort study. Front. Physiol. 13:951460. doi: 10.3389/fphys.2022.951460
Received: 24 May 2022; Accepted: 24 August 2022;
Published: 23 September 2022.
Edited by:
Leonardo Alexandre Peyré-Tartaruga, Federal University of Rio Grande do Sul, BrazilReviewed by:
Marcelo Coertjens, Federal University of Piauí, BrazilDanilo C Berton, Federal University of Rio Grande do Sul, Brazil
Copyright © 2022 Chouinard, Roy, Blais, Lippens, Pelletier, Roy, Marcoux, Ugalde, Rheault, Pigeon, Nicodème, Lacasse and Maltais. This is an open-access article distributed under the terms of the Creative Commons Attribution License (CC BY). The use, distribution or reproduction in other forums is permitted, provided the original author(s) and the copyright owner(s) are credited and that the original publication in this journal is cited, in accordance with accepted academic practice. No use, distribution or reproduction is permitted which does not comply with these terms.
*Correspondence: François Maltais, ZnJhbmNvaXMubWFsdGFpc0BtZWQudWxhdmFsLmNh
†These authors have contributed equally to this work