- 1College of Plant Protection, Shenyang Agricultural University, Shenyang, China
- 2State Key Laboratory for Biology of Plant Diseases and Insect Pests, Institute of Plant Protection, Chinese Academy of Agricultural Sciences, Beijing, China
- 3Henan Institute of Science and Technology, Xinxiang, China
- 4Hebi Jiaduo Industry and Trade Co., Ltd., Hebi, China
Spodoptera litura is an omnivorous pest that has spread globally. Because irradiation sterilization technology has a great potential for control of S. litura, the effect of 25–150 Gy doses of X-rays on pupal survival, flight and reproductive variables of adult moths were analyzed in this research. The X-ray irradiation with the dose of 25–150 Gy significantly affected the reproductive ability of females. Irradiating male pupae with 25–150 Gy doses of X-rays had no effect on mating, life span, or flight ability of adult moths, but significantly reduced survival and fecundity of their offspring, and the sterility rate of the F1 generation was 52.65%–99.9%. The results of logistic curve fitting showed that the sterility impact was 84% at the most appropriate irradiation dose (71.26 Gy). The sterility control was 91% in an indoor mating competition experiment when the release ratio of irradiated males (75 Gy) to nonirradiated males reached 12.6:1. The effects of X-ray irradiation doses on biological variables of S. litura and the most effective release ratio determined here provide a theoretical foundation for using radiation sterilization technology to control S. litura.
1 Introduction
Spodoptera litura (Fabricius) (Noctuidae; Lepidoptera) is a worldwide pest (Bishara, 1934; Aitkenhead et al., 1974; Tojo et al., 2013) that can feed on 389 plant species from 109 families (Qin et al., 2006), it is also widely dispersed in China, where it breeds all year in Yunnan and other tropical areas of China (Wu et al., 2016). Chemical pesticides are the most common way to control S. litura, but resistance to a range of chemical insecticides (Srivastava and Joshi, 1965; Zhou and Huang, 2002; Ahmad et al., 2008), particularly carbamates and pyrethroids, has been reported since 1965 (Zhou and Huang, 2002; Ahmad et al., 2007a; Ahmad et al., 2007b). As a result, strategies for integrated pest management (IPM) of S. litura have been widely accepted by all stakeholders (Rao et al., 1993; Klassen, 2005; Ehler, 2006).
One measure for IPM is sterilizing insect pests by irradiation with X-rays, electron beams, or γ-rays, then releasing a large number of sterile insects into the field (Knipling, 1955; LaChance et al., 1975). Since the 1950s, this eco-friendly technology has been used in wide range of IPM applications to control pests (Hendrichs et al., 2002) such as the dipterans Cochliomyia hominivorax (Coquerel) (Knipling, 1960; Wyss, 2006), Ceratitis capitata (Wiedemann) (De Longo et al.,2000; Hendrichs et al., 1983), Bactrocera cucurbitae (Coquillett) (Yosiaki et al., 2003; Koyama et al., 2004). The use of radiation sterilization has lagged for lepidopterans, however, because of their high resistance to radiation (LaChance, 1967). As a result, a method to generate inherited sterility (IS) was developed for lepidopteran insects (Proverbs and Newton, 1962; North, 1975) and has been used to control a group of lepidopteran insects such as Cydia pomonella (Bloem et al., 2007), Teia anartoides (Walker) (Suckling et al., 2007), Pectinophora gossypiella (Saunders) (Bloem et al., 2005; Tabashnik et al., 2021). However, because radioisotopes cannot be guaranteed to be safe, their usage is becoming increasingly limited. Many SIT programs, which rely on ionizing radiation from radioisotopes for insect sterilization, are severely hampered. As a result, finding a replacement for radioisotopes radiation source is critical. Because of its higher safety, lack of radioactive residue, and ease of portability, X-rays have steadily replaced highly radioactive cobalt sources that were commonly used in the past (Bakri et al., 2005; Yamada et al., 2014; Light et al., 2015).
The method of employing X-rays to irradiate insects has gradually gained traction in recent years, and considerable research has been reported. Cagnotti et al. (2012) used X-rays to sterilize Tuta absoluta pupae and found that 200 Gy was the best dose for sterility. Urquidi et al. (2015) used different wavelengths of X-rays to irradiate mosquitos, finding that long wavelengths were more effective at mosquito sterilization. Adult orangeworm moths were irradiated with X-rays by Light et al. (2015), the research showed that 125 Gy could cause sterility in both parents and F1 generations. Aedes albopictus irradiated with X-ray at a dose of 40 Gy by Du et al. (2019) also had a high sterility effect. Haff et al. (2020) discovered that X-rays and γ-rays were biologically equivalent after irradiating Amyelois transitella larvae with X-rays. With 200 Gy X-ray treatment, Ephestia elutella can be sterilized, and a 15:1 ratio of irradiated male insects to normal male insects can prevent 71.91% of the wild population from reproducing (Zhao et al., 2022). In 1974, scientists discovered that 40 Gy of 60Co radiation had a significant impact on the offspring development of male S. litura (Mochida and Miyahara, 1974). In 1993, Seth and Sehgal explored the influence of radiation on the longevity of male S. litura and its F1 generation larvae, proving that IS may be used to manage S. litura (Seth and Sehgal, 1993). However, no any study on the optimal irradiation dose of X-rays to control S.litura was reported until now. In this study, we determined the effects of X-ray irradiation doses on critical biological variables and the best release ratio of irradiated to nonirradiated males to provide a theoretical basis for the development of S. litura green control technology.
2 Materials and methods
2.1 Experimental insect
Adults of S. litura were obtained from the Langfang Experimental Station, Institute of Plant Protection, Chinese Academy of Agricultural Sciences, where field populations were collected on a regular basis for rejuvenation. After eclosion, adults were placed in a 450 ml disposable plastic bottle with a cotton ball dipped in 5% v/v honey water, and then the bottle was sealed with gauze. Daily, any eggs in the bottle were removed and placed in a self-sealed bag to await hatching, and the cotton ball was replaced with a fresh one. After eggs hatched, each larva was placed in a separate transparent plastic box (diameter: 50 mm; height: 40 mm) and an artificial feed made of soybean powder and wheat germ powder (Greene et al., 1976). On the fifth day after pupation, males and females were identified as described by Zhao et al. (2011). All insect stages were grown in an MGC intelligent program artificial climate box (MGC-450HP, Shanghai Yiheng Scientific Instrument Co., Ltd.) at 25°C ± 1°C, 70% ± 5% RH, and photoperiod of L: D = 16 h: 8 h.
2.2 Irradiation equipment
An X-ray irradiator (JYK-001 type), newly developed by Hebi Jiaduoke Industry and Trade Co., Ltd., Hebi, China, was used. The dose of X-ray was selected as 1.790 mGy/s (180 kV/10 mA) in this study. The samples were placed on an irradiation table (210 mm × 210 mm) at a distance of 400 mm from the X-ray source to receive X-rays. The temperature in the irradiation chamber was 25°C ± 1°C, and the irradiation gradient was 0 Gy (unirradiated), 25 Gy (13,416 s), 50 Gy (26,831 s), 75 Gy (40,247 s), 100 Gy (53,662 s), 125 Gy (67,078 s), and 150 Gy (80,494 s). When the cumulative dose was attained, the irradiation was halted and the sample was taken out. During irradiation, the dosage rate was monitored in real-time and ranged from 1.731 to 1.864 mGy/s.
2.3 Experimental methods
2.3.1 Effects of irradiation on S. litura pupa
Healthy male and female pupae with a pupal mass >300 mg near eclosion (8 days old) (Bushland and Hopkins, 1951; Ouye et al., 1964) were placed in different transparent boxes (length: 100 mm; width: 100 mm; height: 10 mm) according to the irradiation dose with wet gauze to maintain humidity. 30 female and 30 male pupae were irradiated for each of the six doses (25 Gy, 50 Gy, 75 Gy, 100 Gy, 125 Gy, 150 Gy), using unirradiated insects cultured in the lab at the same time as the control. The experiment was repeated six times. Each pupa was then placed in a 450 ml bottle to wait for eclosion after being irradiated. Dead and deformed pupae were used to calculate mortality and deformity rates; pupae were considered deformed if moths could not expand their wings after eclosion.
2.3.2 Effects of X-ray irradiation on mating and reproduction of the parent moths of S. litura
Pupae were irradiated as described in Section 2.3.1. After the adult emerged, they were paired with unirradiated females; the pair was placed in a 450 ml plastic bottle, eggs collected and food replaced as described in Section 2.1. The pairing methods of moths are as follows: N♀ × N♂ (control), N♀ × T♂, and T♀ × N♂ (N, nonirradiated, T, irradiation treatment); 15 pairs were treated per dose, and the experiment was done 4 times. The spermatophores within the spermathecae were counted and the mating history of the dead female moths was determined using a stereoscope with 1× objective (TS-63X, Shanghai Shangguang New Optical Technology Co., Ltd., Shanghai, China). When spermatophore was found to exist in the female moth’s spermathecae, the moth mating was identified successfully. Pre-oviposition, oviposition, and post-oviposition periods (the time between the last egg laying and the female’s death), the number of eggs deposited, eggs hatched, male adult lifespan, and mating time for each group were all recorded. Finally, the infertility rate was calculated (the probability that the eggs laid by female moth did not hatch was referred as the infertility rate).
2.3.3 Effects of different X-ray irradiation doses on flight ability of S. litura adults
Flying ability was assessed using the FXMD-24-USB insect flight information system (Henan Hebi Jiaduoke Industry and Trade Co., Ltd.) and the hoisting test based on the method of Ge et al. (2019). Male pupae were irradiated as described in Section 2.3.1. After emergence of the adults, 3-day-old adults that developed from the irradiated pupae and had intact wings were loaded into the finger tube and numbered. Twenty to thirty adults were selected from each treatment dose. The moths were removed carefully from the tubes, the wings of the moth were stretched out, the scales on the abdomen and thorax were brushed away, and the thorax was attached to the flight mill with a small droplet of cyanoacrylate glue (Deli Group Co., Ltd., Zhejiang, China). The test insect was inserted vertically at 90 degrees onto the crane arm of the flight mill and allowed to fly continuously for 24 h in the dark at 25 C ± 1°C and 70% ± 5% RH.
2.3.4 Effects of different X-ray irradiation on F1 generations of S. litura
F1 generation larvae (the male parent was irradiated [see Section 2.3.1], the female parent was not) were provided artificial feed (see Section 2.1 for insect rearing methods), their development was observed every day, and the duration of each stage was recorded. Also, the 3-days-old pupae were weighed. Once the F1 adults had emerged, they were paired with nonirradiated heterosexual adults that emerged on the same day as F1 adults, and reproductive variables (such as the periods of pre-oviposition, oviposition, and post-oviposition; the number of eggs deposited, eggs hatched, adult lifespan, and mating rate) were recorded. Growth of at least 200 eggs and 80 first-instar larvae were observed for each treatment, and the experiment was repeated three times. Mating rate and sterility rate were calculated for the F1 generation. Finally, the life table parameters for the F1 population were calculated. The net reproductive rate (R0), generation time (T), intrinsic rate of increase (r), and finite rate of increase (λ) of the S.litura F1 populations were estimated using the formulas below (Chi and Liu, 1985; Chi 1988; Asiimwe et al., 2007):
The x is the number of days, lx is the S. litura survival probability from egg to x days old fx is the age-specific fecundity at age x, mx is the average population fecundity from egg to x days old, lxmx is the population age-specific maternity, and e is the Euler number.
2.3.5 Mating competition test between irradiated and nonirradiated males of S. litura
We set up a total of 13 groups with differing numbers of irradiated males (75 Gy X-rays in the pupal stage) to nonirradiated males to nonirradiated normal females: 0:1:1 (control), 1:1:1, 2:1:1, 3:1:1, 4:1:1, 5:1:1, 6:1:1, 7:1:1, 8:1:1, 9:1:1, 10:1:1, 11:1:1, and 12:1:1 in feeding buckets (diameter: 25 cm; height: 30 cm) with cotton balls dipped in 5% v/v honey water. The pail was sealed with gauze, eggs were collected and counted and fresh food supplied daily. The number of eggs which hatched larva was also recorded for calculating the infertility rate.
2.4 Data analyses
A two-way analysis of variance was used to examine the deformity rate and adjusted mortality of irradiated pupae, and the reproductive parameters of parental adult moths, with different radiation doses and sex as factors. If the difference was significant, Tukey’s HSD multiple comparison test was performed. The one-way ANOVA was used to analyze the effects of different irradiation doses on mean adult flight ability, and F1 insect development of S. litura in software SPSS (version 26.0; IBM, Armonk, NY, United States). Percentage data were arcsine square-root transformed before the analysis of variance. If the difference among doses was significant for a variable, multiple Tukey’s HSD comparisons were carried out, and the Log-rank test was used to analyze the survival of the F1 generation of S. litura with different dose treatments in GraphPad (version 8.0; GraphPad Software Inc., San Diego, CA, United States).
Logistic regression was used to fit the sterility rate of S. litura at different doses of X-rays, and the sterility rate of S. litura with different release ratios was fitted by logistic in Origin software (version 2019; Origin Lab Corporation, Northampton, MA, United States). The model equation was
3 Results
3.1 Effect of irradiation on pupae
The deformity rate of S. litura pupae did not differ significantly by irradiation doses and irradiation dose × sex (irradiation doses: F6, 84 = 0.539, p = 0.777; irradiation dose × sex: F6, 84 = 0.790, p = 0.581), but did significantly by sex (F1, 84 = 7.424, p = 0.008), according to two-way ANOVA (Table 1). The adjusted mortality rate of S. litura pupae did not differ significantly by irradiation doses, sex, and irradiation dose × sex (irradiation doses: F6, 72 = 0.361, p = 0.900; sex: F1, 72 = 0.181, p = 0.672; irradiation dose × sex: F6, 72 = 0.168, p = 0.984).
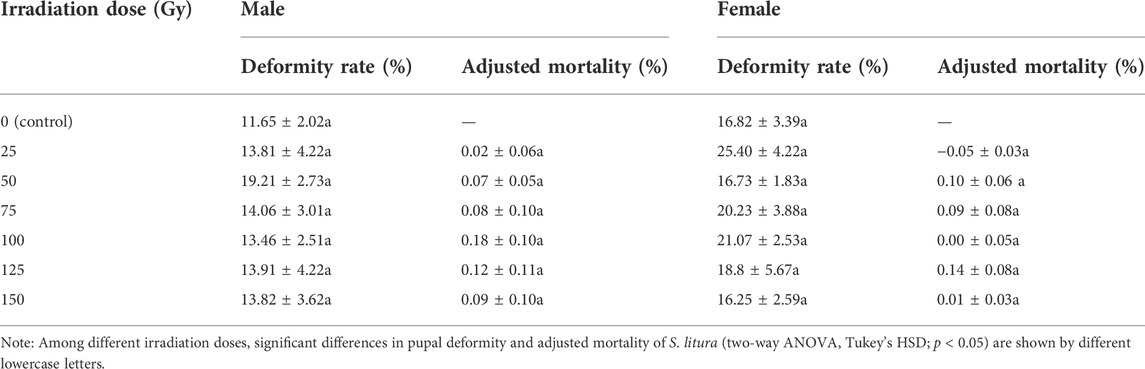
TABLE 1. Mean (±SE) deformity rates and adjusted mortality of pupae of Spodoptera litura after irradiation with different doses of X-rays.
3.2 S. litura reproductive parameters and adult longevity after various X-ray doses
The mating rate of S. litura parents was not affected by irradiation doses and irradiation dose × sex interaction, but it did significantly by sex (irradiation doses: F6, 42 = 0.241, p = 0.9602; sex: F1, 42 = 7.574, p = 0.009; irradiation dose × sex: F6, 42 = 0.459, p = 0.834; Figure 1A). The oviposition of S. litura parents was affected by irradiation dose and sex, but not by irradiation dose × sex interaction (irradiation doses: F6, 42 = 5.469, p = 0.0003; sex: F1, 42 = 10.460, p = 0.002; irradiation dose × sex: F6, 42 = 2.180, p = 0.064; Figure 1B). The irradiation dose × sex interaction had a significant influence on the pre-oviposition duration, while the irradiation dose had no effect (irradiation doses: F6, 42 = 1.292, p = 0.282; sex: F1, 42 = 45.30, p < 0.0001; irradiation dose × sex: F6, 42 = 3.106, p = 0.013; Figure 1C). The sex had a significant effect on the post-oviposition duration, while the irradiation dose and irradiation dose × sex interaction had no effect (irradiation doses: F6, 42 = 1.525, p = 0.194; sex: F1, 42 = 7.796, p = 0.008; irradiation dose × sex: F6, 42 = 0.909, p = 0.498; Figure 1D). The eggs deposited per female was affected significantly by irradiation dose and sex, but not by the interaction of irradiation dose and sex (irradiation doses: F6, 42 = 7.519, p < 0.0001; sex: F1, 42 = 23.250, p < 0.0001; irradiation dose × sex: F6, 42 = 2.113, p = 0.0718; Figure 1E). The longevity of S. litura adults was affected significantly by sex, but not by irradiation dose and irradiation dose × sex interaction (irradiation doses: F6, 42 = 0.2928, p = 0.9370; sex: F1, 42 = 8.746, p = 0.0051; irradiation dose × sex: F6, 42 = 0.2499, p = 0.9566; Figure 1F).
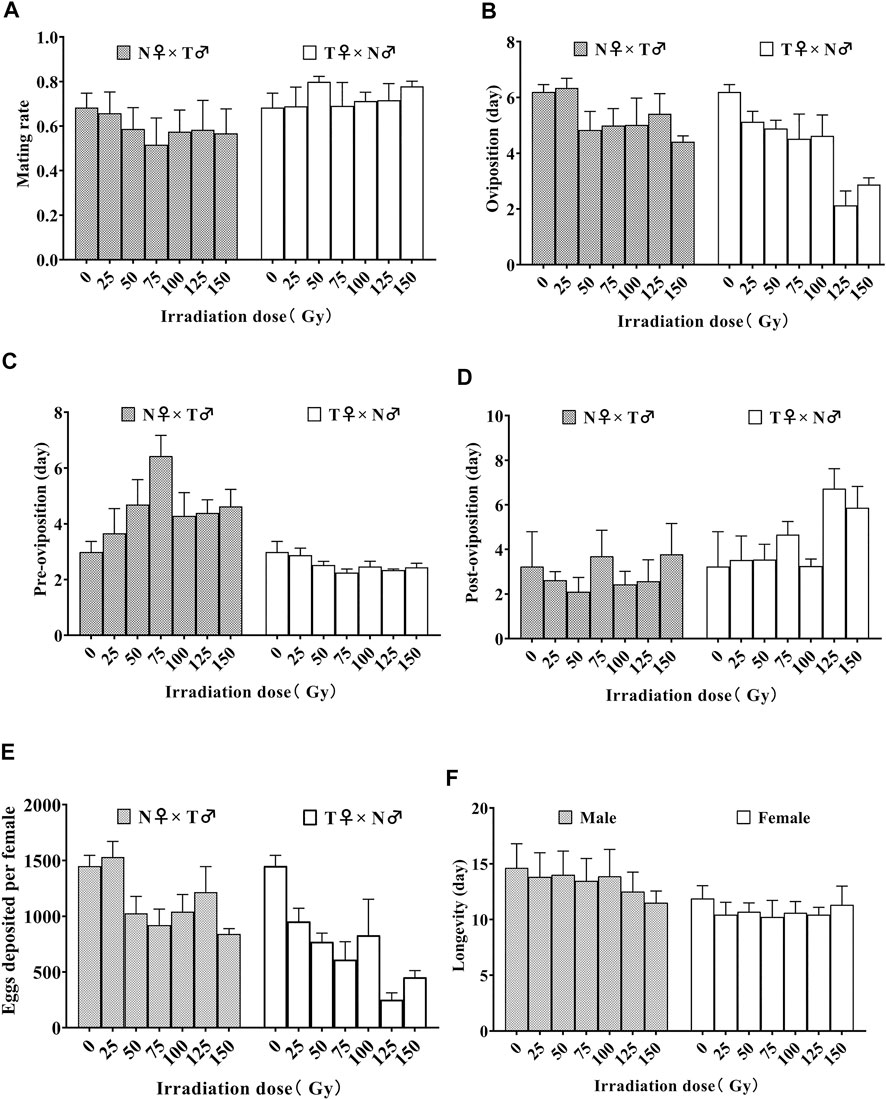
FIGURE 1. Effect of different doses of X-rays on mean (±SE) mating rate (A), -oviposition (B), pre-oviposition (C), post-oviposition (D), eggs deposited per female (E), and the longevity of irradiated moth (F) adults of patent Spodoptera litura. The pairings were N♀ × T♂ (N, no irradiation; T, irradiation treatment) while the control pairings were N♀ × N♂ (0 Gy).
3.3 Effects of different doses on sterility of S. litura
According to a one-way ANOVA, the sterility rate for irradiated moths that mated with nonirradiated heterosexual moths increased significantly at higher irradiation doses (T♀ × N♂: F6, 21 = 5.514, p = 0.001; N♀ × T♂: F6, 21 = 13.611, p < 0.001) (N = nonirradiated, T = irradiation treatment), and both the growth dynamics fit the logistic curve (T♀ × N♂: R2 = 0.879, p < 0.001; N♀ × T♂: R2 = 0.981, p < 0.001, Figure 2). The equation that fits the mating sterility rate of irradiated male moths and nonirradiated female moths was
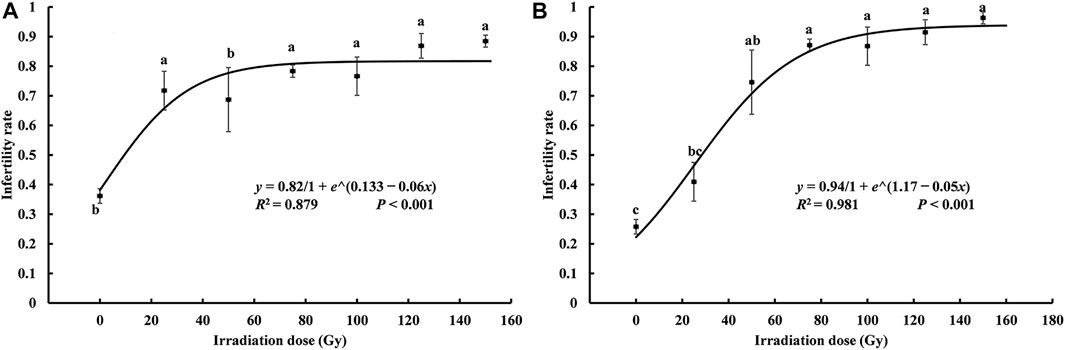
FIGURE 2. Curve of the relationship between the sterility rate of Spodoptera litura hybrids and X-ray irradiation dose. (A) the sterility rate of X-ray irradiated female paired with unirradiated male; (B) the sterility rate of unirradiated female paired with of X-ray irradiated male. Data are means ± SE; significant differences (one-way ANOVA, Tukey’s HSD; p < 0.05) among doses for a variable are indicated by different lowercase letters.
3.4 Effects of X-ray irradiation on the flight ability of S. litura
In the one-way analysis of variance, none of the flight variables differed significantly among the irradiation doses (flight mass loss: F6, 125 = 0.241, p = 0.962; flight speed: F6, 125 = 1.147, p = 0.339; flight time: F6, 125 = 0.963, p = 0.453; flight distance: F6, 125 = 0.992, p = 0.434) (Table 2).
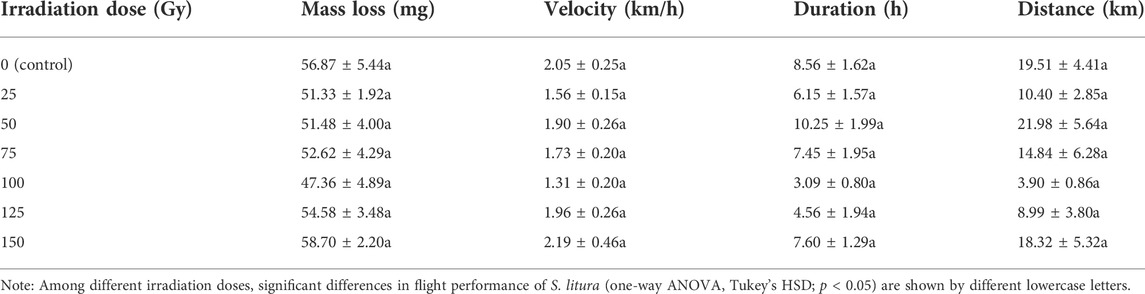
TABLE 2. Means (±SE) for flight performance variables of Spodoptera litura adults exposed to different doses of X-ray during pupal stage.
3.5 Development of S. litura F1 generations after males were irradiated with different doses of X-rays
After males that were irradiated at doses from 25 to 150 Gy at the pupal stage mated with nonirradiated females, the developmental duration of the F1 generation insects was significantly affected. As shown in the ANOVA, different doses of irradiation had significant effects on the duration of egg stage (F6, 2858 = 87.842, p < 0.001), 1st instar larva (F6, 2833 = 94.382, p < 0.001), 2nd instar larva (F6, 2803 = 29.079, p < 0.001), 3rd instar larva (F6, 2765 = 6.434, p < 0.001), 4th instar larva (F6, 2702 = 18.472, p < 0.001), 5th instar larva (F6, 2655 = 9.166, p < 0.001), 6th instar larva (F6, 2440 = 5.362, p < 0.001), larval stage (F6, 2858 = 4.255, p < 0.001), pupal stage (F6, 1278 = 5.941, p < 0.001), egg stage-pupal stage (F6, 2858 = 13.54, p = 0.03), pupal mass (F6, 1278 = 19.856, p < 0.001), adult duration (F6, 1278 = 3.283, p = 0.003), and generation period (F6, 2858 = 14.016, p < 0.001), the duration of pre-oviposition stage of females (F6, 261 = 3.921, p = 0.001), number of eggs deposited per female (F6, 261 = 4.522, p < 0.001) of insects, but had no significant effect on the duration of post-oviposition stage (F6, 261 = 1.304, p = 0.255) (Table 3). Compared with the control, the pupal mass of the F1 generation in the 25–150 Gy irradiation treatments group was significantly reduced (386.45–421.76 mg), and the sex ratio was significantly inclined to males (male: female = 1.04–2); the oviposition period (4–4.68 days) for the 50 Gy, 75 Gy, and 125 Gy treatment groups was significantly lower compared with the control (6.7 days); and the 100 Gy and 150 Gy treatment groups had significantly lower pre-oviposition (1.5–1.76 days) than the control group (3.32). Significantly fewer eggs were deposited per female (99.5–316.78) compared with the control (1028.3) when the irradiation dose was >125 Gy, and at 150 Gy, the insect generation duration (26.19 days) was significantly lower than that of the nonirradiated insects (35.01 days) (Table 3). The mating rate for the 25–150 Gy dose groups was not significantly different from that of the nonirradiated insects by different mating types (N♀ × T♂: F6, 14 = 0.166, p = 0.982; T♀ × N♂: F6, 14 = 0.479, p = 0.813; T♀ × T♂: F6, 14 = 1.311, p = 0.315) (N = normal individual whose parents were both unirradiated; T = F1 individual whose male parent was irradiated with 250 Gy dose of X-ray); however, when the irradiation dose ≥50 Gy, the sterility rate of the F1 generation with two mating types was significantly higher than that of the control (N♀ × T♂: F6, 159 = 8.973, p < 0.001; T♀ × T♂: F6, 159 = 10.238, p < 0.001). The sterility rate of the 100 Gy, 125 Gy, and 150 Gy treatment groups was significantly higher than that of the control group when the mating mode was T♀ × N♂ (F6, 159 = 5.654, p < 0.001) (Table 4).
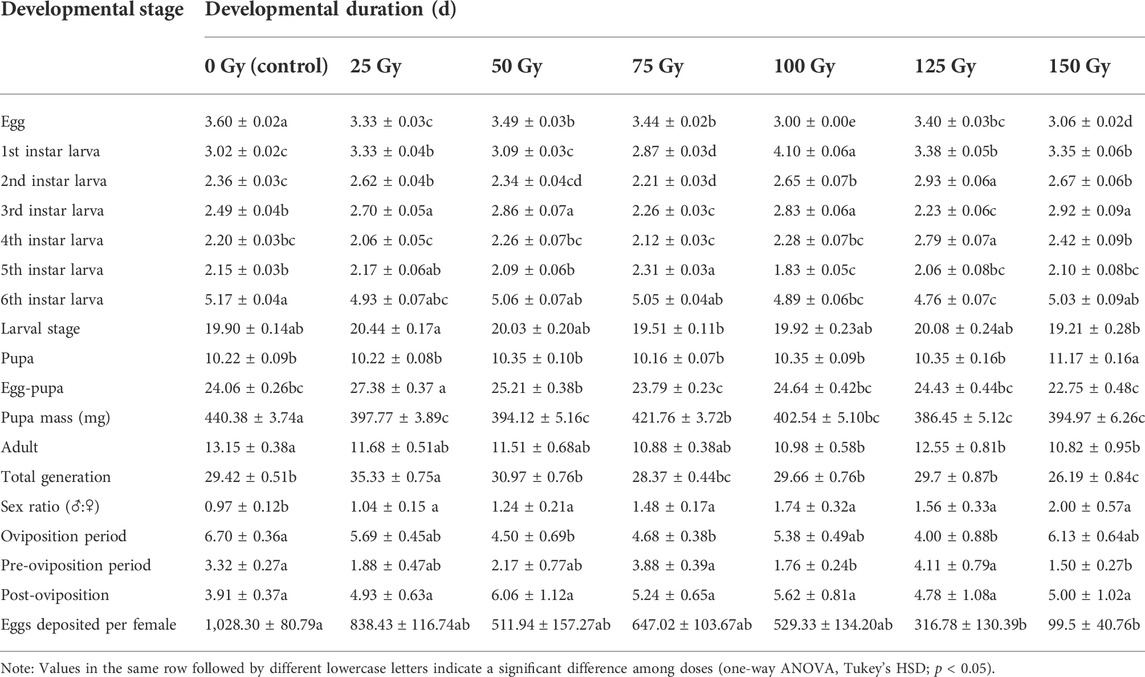
TABLE 3. Mean duration (±SE) for developmental stages of F1 generation of Spodoptera litura after male parent was irradiated with different X-ray doses during pupal stage.
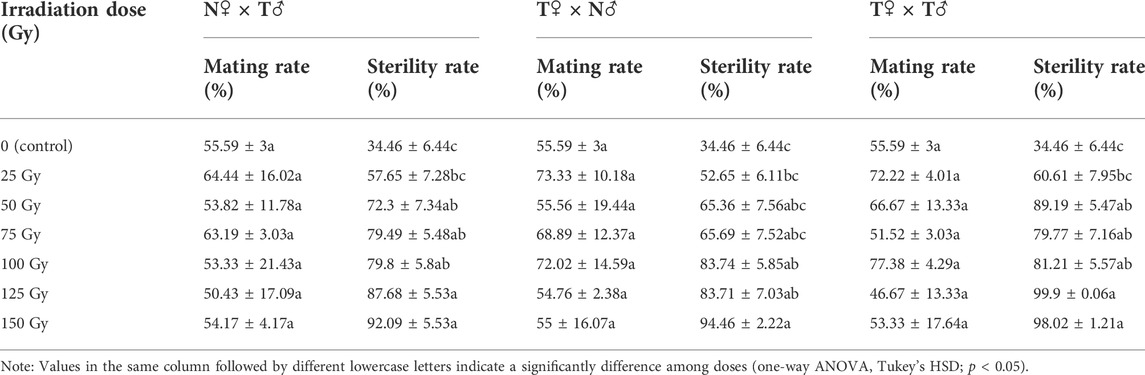
TABLE 4. Mean (±SE) mating rate and sterility rate for F1 offspring adults of Spodoptera litura by different mating types: T♀ × N♂, N♀ × T♂, and T♀ × T♂ (N = normal individual whose parents were both unirradiated; T = F1 individual whose male parent was irradiated with 250 Gy dose of X-ray).
Survival analysis showed that the age-specific survival rate curves (lx) of the population under different irradiation doses had highly significant differences (χ2 = 904.655, df = 6, p < 0.0001), and there was a significant linear trend (χ2 = 697.500, df = 1, p < 0.0001) (Figure 3). The mx curve and the lxmx curve showed that the peak reproductive age of insects in the treatment group and nonirradiated insects was similar, the maximum age-specific fecundity (mx) for the 0–150 Gy treatment group was, respectively, on the 35th, 34th, 33rd, 35th, 33rd, 33rd, 33rd day, and the age-specific maternity (lxmx) maximum was, respectively, on 32nd, 32nd, 33rd, 32nd, 33rd, 33rd, and 33rd day. For the 25–150 Gy doses, the age-specific fecundity of female adults (fx) decreased as the dose increased (highest fecundities were, respectively, 125.7, 51.6, 54.4, 67.7, 23.1, 14.3) (Figure 3). When the irradiation dose was ≥50 Gy, the net reproductive rate R0, intrinsic rate of increase r, and finite rate of increase λ of the F1 generation insects were significantly lower than those of the control group, and the F1 generation population was significantly lower (Table 5).
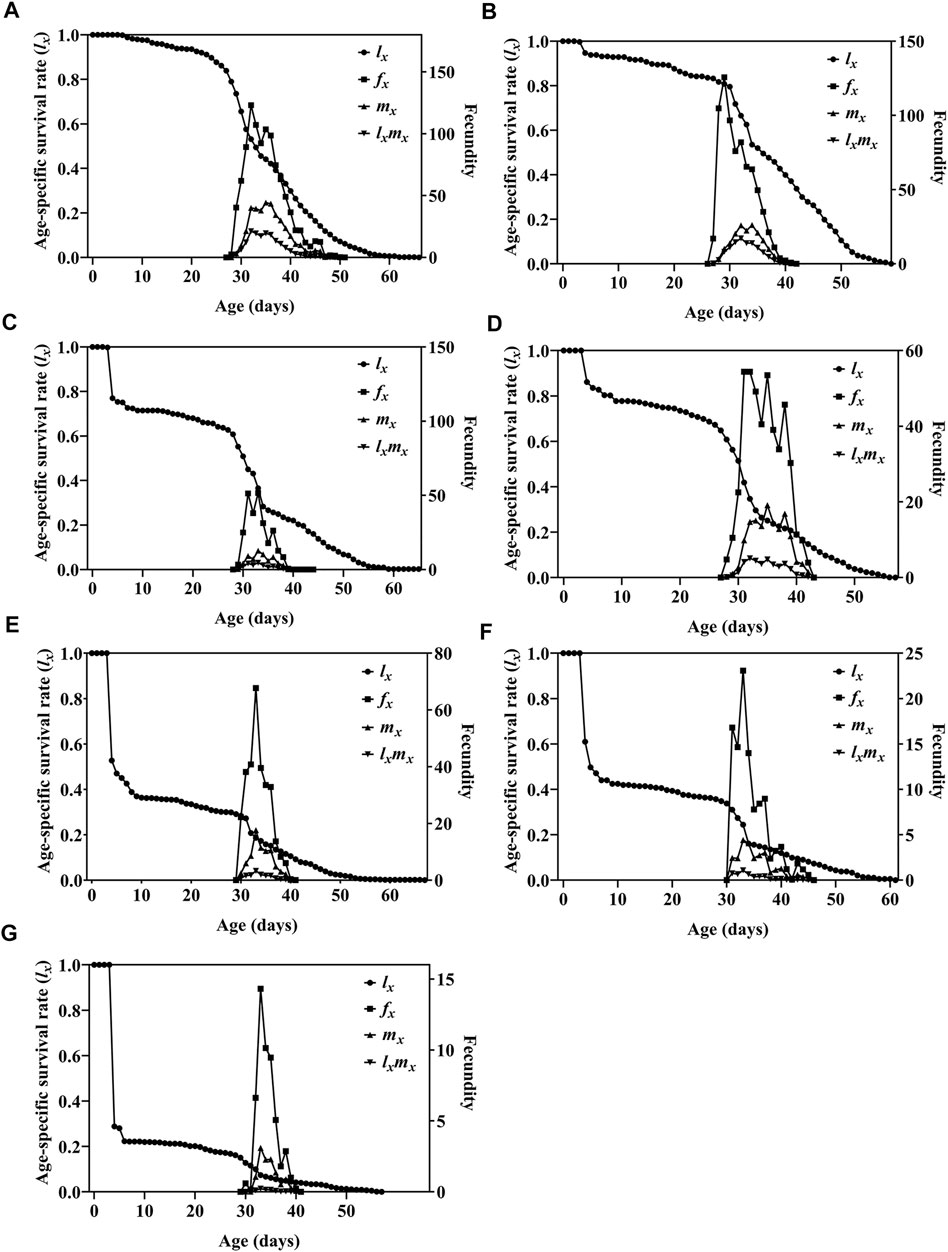
FIGURE 3. Age-specific survival rate (lx), age-specific fecundity of female adult (fx), age-specific fecundity (mx), and age-specific maternity (lxmx) of F1 generation of Spodoptera litura after male parent was irradiated with different doses of X-rays. (A) Survival rate of F1 generation of nonirradiated parents (N♀ × N♂). (B–G) F1 generation after mating with nonirradiated females and males irradiated with 25 Gy, 50 Gy, 75 Gy, 100 Gy, 125 Gy, or 150 Gy doses of X-rays during pupal stage (N♀ × T♂) (N, nonirradiated; T, irradiation treatment).
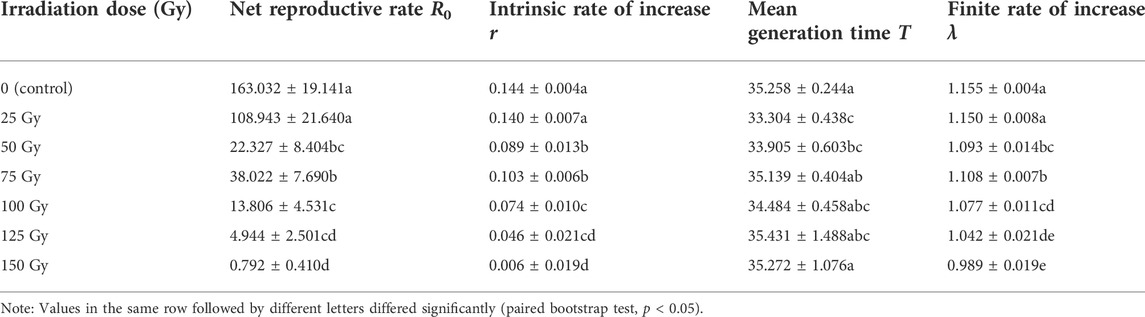
TABLE 5. Means (±SE) for life table parameters of Spodoptera litura F1 generation after male parent was irradiated with different X-ray doses during pupal stage.
3.6 Competitive release ratio between irradiated males and nonirradiated males
There were significant differences in the number of eggs laid by females after different release ratios of irradiated males (75 Gy) and nonirradiated males (F12,82 = 2.515, p = 0.007, Figure 4A). The sterility rate increased significantly with an increase in proportion of irradiated males (F12,82 = 11.914, p < 0.001; Figure 4B). When the irradiation release ratio (T♂: N♂: N♀) was 5:1:1 to 12:1:1, the sterility rate (59.85%–88.44%) was significantly higher than in the control group (24.55%) (N = nonirradiated, T = irradiation treatment). The growth dynamics in relation to the sterility rate conformed to the logistic curve
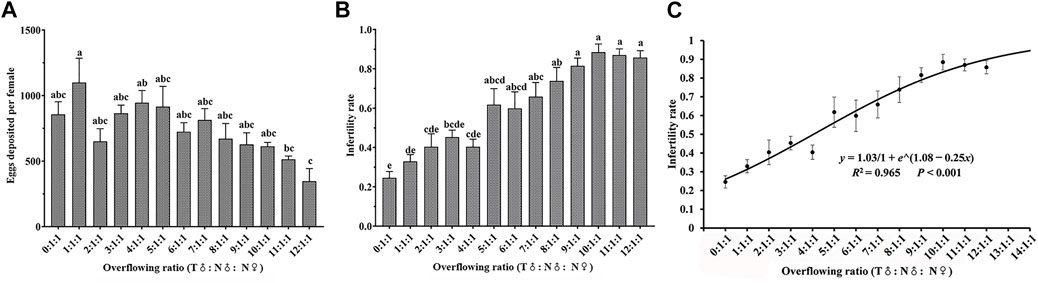
FIGURE 4. Effects of different release ratios of substerilized males (irradiated with 75 Gy dose of X-rays) and nonirradiated males on fecundity (A), sterility rate (B) and logistic curve of sterility rate of Spodoptera litura (C). Data are means ± SE; significant differences (one-way ANOVA, Tukey’s HSD; p < 0.05) among doses for a variable are shown by different lowercase letters.
4 Discussion
After S. litura pupae (8 day old) were irradiated with 25–150 Gy X-rays, our analyses of pupal survival, reproductive variables of adults, adult life span, flight ability, and offspring developmental durations showed that none of the doses altered the emergence, reproductive ability, adult life span, or flight ability of male S. litura. However, survival rate and fecundity of its offspring were reduced compared with nonirradiated controls, and the sex ratio of the F1 generation tended toward males. The X-ray of 25–150 Gy dose had no effect on the emergence rate of female pupae, but significantly affected their reproduction. However, a dose of 150 Gy still did not reach the point of female sterilization. According to the logistic curve fit, the appropriate sterilizing effect would be achieved with 71.26 Gy in theory, and the best control effect could be achieved with a release ratio of 12.6: 1 irradiated (75 Gy) males to nonirradiated males.
The type of irradiation source and the age of insects can affect the sterilization dose of insects, according to the studies on the irradiation sterility of a range of species (IDIDAS Database: https://nucleus.iaea.org/sites/naipc/ididas/Pages/Browse-IDIDAS.aspx). With a similarity, the research on S. litura also presented this point. In 1974, researchers discovered that exposing 3–5-day-old male pupae of S. litura to an 80 Gy dose of 60Co-rays sterilized all pupae (Mochida and Miyahara, 1974). When 3-day-old S. litura pupae were irradiated with a 150 Gy electron beam, the hatching rate of the eggs produced by their offspring was 0% (Yun et al., 2014). Evaluating the radiation biological characteristics of insects while using different radiation sources than in prior studies is critical. In addition, when young pupae were used, the impact of insect sterility could be achieved at a lower dose, but pupae mortality was considerable. As a result, using older pupae for irradiation would be a more cost-effective way to increase insect pupae survival after irradiation (Bushland and Hopkins, 1951; Ouye et al., 1964; Ducoff and Bosma, 1966). Among the numerous studies on irradiation-induced sterility in S. litura, few have focused on the effect of X-rays. We therefore focused here on X-ray irradiation of 8-day-old male pupae of S. litura and determined that the appropriate sub-sterilizing dose is around 75 Gy. The sub-radiation dose for males was usually equal to the sterilization dose for females of this species, because fertile radiation females were thought to cause unpredictably significant population increase in the wild (Bloem et al., 1999). In our study, we discovered that even at the dose of 150 Gy employed in the experiment, the female could not be sterilized. Seth and Sehgal (1993) found that 100 Gy and 130 Gy doses of γ-ray irradiation were good sub-sterilisation doses of S. litura, but that the γ-ray of 130 Gy did not reach the sterilization dose of females, which is similar to our findings. That was because studies have showed that after 130 Gy of γ-ray irradiation, the male sperm competitiveness of S. litura decreased (Seth et al., 2002). Similar effects have been observed in other Lepidoptera insects. In Spodoptera frugiperda, males exposed to 100 Gy γ-ray dos exhibit better sperm competitiveness than males exposed to 150 Gy dose (Carpenter and Gross, 1993). As a result, if the female sterilization dose is used as the male sub-sterility dose, the competitive ability of S. litura may be seriously impacted. As a result, populations for genetic sex separation (GSS) from released insects should be developed before SIT technology can be used on a large scale, such as C. capitata, Bombyx mori, P. gossypiella (Peloquin et al., 2000; Tamura et al., 2000; Sahara et al., 2003; Augustinos et al., 2017).
Furthermore, flying ability is an essential factor to be concerned in the SIT program (Seth et al., 2016b). According to Stephens et al. (2006), the flight ability of sterile painted apple moths was not significantly affected after being irradiated with γ-rays at 100 Gy. The flight capacity of Teia anortoides was unaffected by γ-ray radiation of 160 Gy (Suckling et al., 2002). The flying ability of S. frugiperda was not affected when the dose of X-ray was lower than 300 Gy (Jiang et al., 2022). Their findings are nearly identical to ours.
Lepidopteran insects are more resistant to radiation than insects in other orders (LaChance et al., 1975) because, after irradiation, the shattered chromosomal fragments attach to the centromere and are passed to the next generation through germ cells (Proverbs and Newton, 1962; Marec and Traut, 1993). As a result, using substerile doses to irradiate insects can not only inhibit the development of parental populations but also reduce the number of their offspring (Seth et al., 1997; 2016a). This inherited sterility has provided remarkable advancement in the control of lepidopteran pests, such as Trichoplusia ni and Helicoverpa zea (North and Holt, 1969; Carpenter and Gross, 1993). The reproductive peak of F1 females was similar to that of normal insects, and there was no significant variation in the development duration of F1 generations in different stages by irradiation with the dose range of 25 Gy–75 Gy in this study. This shows that irradiated insects’ reproduction behavior can be synchronized with that of normal insects in the wild after being released in the field. The same effects were observed in research of Helicoverpa Armigera and A. transitella F1 generation developmental stages (Kim et al., 2015; Light et al., 2015). The F1 generation of irradiated insects in this study still showed high sterility (>60%) and the mating rate was unaffected, confirming that 75 Gy was a suitable dose of sterility. Similar results were reported when S. litura was irradiated with gamma rays, the substerilizing irradiation 0–24 h old male adults of S. litura with 100 Gy and 130 Gy of γ-ray used by Seth achieved a higher sterility rate for the F1 generation than for the parents, and 130 Gy also affected larval and gonadal development of the F1 generation (Seth et al., 2000; Seth and Sharma, 2001).
The goal of SIT is to inhibit wild populations from expanding by providing large populations of irradiated individuals to mate with wild populations. As a result, determining the appropriate release ratio of irradiated males to nonirradiated wild males so that a substantial number of irradiated males compete with the wild population to suppress the pest population is essential for the highest efficacy (Bloem and Carpenter, 2001; Bloem et al., 2005). The release ratio is considered as part of the SIT program. Anopheles arabiensis exhibits better mating competitiveness when three times more irradiated males were exposed to 70 Gy doses of γ-rays than normal males, according to Helinski and Knols (2009). The hatching rate of A. albopictus females was less than 20% when the ratio of irradiated males (irradiated by γ-rays of 35 Gy dose) to normal males was 10:1. In this study, the release rate of male insects was also investigated. When the irradiated male moths (exposed to a 75 Gy X-ray during the pupal stage) to normal male release ratios were 10:1, 11:1, and 12:1, the sterility rate was greater than 85%, this is comparable to the effectiveness of pesticides such profenofos and imidacloprid in controlling S. litura (Abbas et al., 2012; Abbas et al., 2014). In order to achieve a better control effect, we used logistic fitting to confirm that the rapid increase in sterility rate ended at a release ratio of 12.6:1, and that induced sterility rate might theoretically reach 91%. S. litura population growth will be severely hampered by this release ratio. An increase in the release rate is required to limit population expansion in many sterile insect release studies. After irradiating Anastrepha fraterculus with a 40 Gy X-ray dose, the ideal release ratio of irradiated male to normal male is 50:1 (Mastrangelo et al., 2018). The induced sterility rate can be increased to more than 70% by raising the release ratio of irradiated C. capitata male to normal male to 100:1 (Rendón et al., 2004). As mentioned earlier, with X-ray doses of 75 Gy, we theoretically determined the best release ratio to be 12.6:1 irradiated to nonirradiated males. However, because this ratio was determined in the laboratory, field testing is needed to determine the appropriate release ratio (Seth and Sehgal, 1993; Hofmeyr et al., 2005).
Using SIT alone cannot adequately control insects that migrate long distances, but the release of substerilized pests is compatible with other pest control methods (Knipling, 1964), and can be part of synergistic management that includes, for example, the release of substerilized pest insects with natural enemies of the pests (Seth et al., 2009; Cagnotti et al., 2016). Area-wide integrated pest management (AW-IPM), in conjunction with SIT, has also provided remarkable control of lepidopteran pests, using Bacillus thuringiensis to control T. anartoides (Suckling et al., 2007) and using Bt cotton to eradicate P. gossypiella (Saunders) in the continental United States and northern Mexico (Tabashnik et al., 2021). Such strategy also is good for the control of S. litura, which survives in winter season only in the tropical area of China (such as Hainan, Guangxi, Yunnan) (Fu et al., 2015; Bei et al., 2001). As a result, the activity range of S. litura is quite limited and isolated in the winter season, it should be a good option to manage the pest using the SIT strategy. The Bt corn planting is now legal in China (http://www.moa.gov.cn/ztzl/zjyqwgz/spxx/201912/t20191230_6334015.htm). By planting Bt corn in areas where S. litura overwinters and releasing sterile males in the winter in those areas, S. litura damage can be reduced there and nationwide as the moths migrate in the spring.
Although the radiation biology of S. litura was investigated in this study, there are still a number of issues to be resolved before the insect is released on a broad scale. Low-energy X-ray irradiators had a lower dose rate than γ-ray irradiators, and the relative efficiency of 30 kV–280 kV X-rays is about 8.9% lower than 60Co (Hjørringgaard, et al., 2020). This implies that X-rays might take a longer time to irradiate than 60Co. Current research indicated that the role of low-energy X-rays on insects was quite comparable to that of γ-rays, which could achieve high sterility (Yamada et al., 2014; Kumano et al., 2018). Recently, the X-ray irradiator had been upgraded, tweaked, and improved on a routine basis (Mastrangelo et al., 2010; Fan and Niemira, 2020). Furthermore, although there is a feasible feed for large-scale rearing of S. litura (Gupta et al., 2005), compared to easier rearing of dipteran insects, rearing S. litura on a large scale is expensive, so efficient, cheaper rearing methods need to be developed. Overall, X-ray irradiation technology to substerilize S. litura is safe and “green,” may play an important and special role compared to existing control methods to the pest. Therefore, there is a great necessary to take a field trial for establishing a mature method for deployment in a large-scale in southern China.
Data availability statement
The original contributions presented in the study are included in the article/supplementary material, further inquiries can be directed to the corresponding author.
Author contributions
KW and SJ designed the project. SJ and S-SJ did the indoor experiment, and H-YZ irradiated the experimental insects. SJ, X-WF, X-MY, and KW analyzed the data. SJ and KW wrote and edited the manuscript. All authors contributed to the article and approved the submitted version.
Funding
This research was funded by the Research projects from the Ministry of Industry and Information Technology of China and China National Tobacco Corporation Science and Technology (grant no. 110201901039).
Conflict of interest
The Author H-YZ was employed by the Hebi Jiaduo Industry and Trade Co., Ltd.
The remaining authors declare that the research was conducted in the absence of any commercial or financial relationships that could be construed as a potential conflict of interest.
Publisher’s note
All claims expressed in this article are solely those of the authors and do not necessarily represent those of their affiliated organizations, or those of the publisher, the editors and the reviewers. Any product that may be evaluated in this article, or claim that may be made by its manufacturer, is not guaranteed or endorsed by the publisher.
Acknowledgments
We thank Aili Li, Juan Li, and Feng-Qin Li for their contributions to larvae cultivation and interns Lei Xu, Si-Jia Wang, Meng-Qi Liu, and Wan-Wan Sun for their contributions to this research.
References
Abbas N., Samiullah S. A., Shad S. A., Razaq M., Waheed A., Aslam M. (2014). Resistance of Spodoptera litura (Lepidoptera: Noctuidae) to profenofos: Relative fitness and cross resistance. Crop Prot. 58, 49–54. doi:10.1016/j.cropro.2014.01.002
Abbas N., Shad S. A., Razaq M. (2012). Fitness cost, cross resistance and realized heritability of resistance to imidacloprid in Spodoptera litura (Lepidoptera: Noctuidae). Pesticide Biochem. Physiology 103, 181–188. doi:10.1016/j.pestbp.2012.05.001
Ahmad M., Iqbal Arif M., Ahmad M. (2007a). Occurrence of insecticide resistance in field populations of Spodoptera litura (Lepidoptera: Noctuidae) in Pakistan. Crop Prot. 26, 809–817. doi:10.1016/j.cropro.2006.07.006
Ahmad M., Sayyed A. H., Crickmore N., Saleem M. A. (2007b). Genetics and mechanism of resistance to deltamethrin in a field population of Spodoptera litura (Lepidoptera: Noctuidae). Pest. Manag. Sci. 63, 1002–1010. doi:10.1002/ps.1430
Ahmad M., Sayyed A. H., Saleem M. A., Ahmad M. (2008). Evidence for field evolved resistance to newer insecticides in Spodoptera litura (Lepidoptera: Noctuidae) from pakistan. Crop Prot. 27, 1367–1372. doi:10.1002/ps.143010.1016/j.cropro.2008.05.003
Aitkenhead R., Baker C. R. B., Chickera G. W. D. (1974). New or common plant diseases and pests. Plant Pathol. 23, 117–118. doi:10.1111/j.1365-3059.1974.tb02921.x
Akca I., Ayvaz T., Yazici E., Smith C. L., Chi H. (2015). Demography and population projection ofAphis fabae(Hemiptera: Aphididae): With additional comments on life table research criteria. J. Econ. Entomol. 108, 1466–1478. doi:10.1093/jee/tov187
Asano S.-i., Bando H., Yasukochi Y., Sahara K., Yoshido A., Kawamura N., et al. (2003). W-derived BAC probes as a new tool for identification of the w chromosome and its aberrations in Bombyx mori. Chromosoma 112, 48–55. doi:10.1007/s00412-003-0245-5
Asiimwe P., Ecaat J. S., Otim M., Gerling D., Kyamanywa S., Legg J. P. (2007). Life-table analysis of mortality factors affecting populations of Bemisia tabaci on cassava in Uganda. Entomol. Exper Applic 122, 37–44. doi:10.1111/j.1570-8703.2006.00487.x
Augustinos A. A., Targovska A., Cancio-Martinez E., Schorn E., Franz G., Cáceres C., et al. (2017). Ceratitis capitata genetic sexing strains: laboratory evaluation of strains from mass-rearing facilities worldwide. Entomol. Exp. Appl. 164, 305–317. doi:10.1111/eea.12612
Bakri A., Mehta K., Lance D. R. (2005). “Sterilizing insects with ionizing radiation,” in Sterile insect technique: Principles and practice in area-wide integrated pest management. Editors V. A. D. J. Hendrichs,, and A. S. Robinson (Dordrecht: Springer), 233–268. doi:10.1007/1-4020-4051-2_9
Bei W. Y., Ru S. J., Chen X. Y., Chen H. P., Wang H. R., Gu X. H., et al. (2001). The effect of temperature on the development and survival of Spodoptera litura (Fabricius). J. Zhejiang Agric. Univ. 13, 197–200. doi:10.3969/j.issn.1004-1524.2001.04.004
Bloem K. A., Bloem S., Carpenter J. E. (2005). “Impact of moth suppression/eradication programmes using the sterile insect technique or inherited sterility,” in Sterile insect technique: principles and practice in area-wide integrated pest management. Editors V. A. D. J. Hendrichs,, and A. S. Robinson (Dordrecht: Springer), 677–700. doi:10.1007/1-4020-4051-2_26
Bloem S., Bloem K. A., Carpenter J. E., Calkins C. O. (1999). Inherited sterility in codling moth (Lepidoptera: Tortricidae): Effect of substerilizing doses of radiation on insect fecundity, fertility, and control. Ann. Entomol. Soc. Am. 92, 222–229. doi:10.1093/aesa/92.2.222
Bloem S., Carpenter J. E. (2001). Evaluation of population suppression by irradiated Lepidoptera and their progeny. Fla. Entomologist 84, 165–171. doi:10.2307/3496163
Bloem S., Carpenter J., McCluskey A., Fugger R., Arthur S., Wood S. (2007). “Suppression of the codling moth Cydia pomonella in British columbia, canada using an area-wide integrated approach with an SIT components,” in Area-wide control of insect pests from research to field implementation. Editors M. J. B. Vreysen, A. S. Robinson, and J. Hendrichs (Dordrecht: Springer), 591–601. doi:10.1007/978-1-4020-6059-5_55
Bushland R. C., Hopkins D. E. (1951). Experiments with screw-worm flies sterilized by X-rays1. J. Econ. Entomol. 44, 725–731. doi:10.1093/jee/44.5.725
Cagnotti C. L., Andorno A. V., Hernández C. M., Paladino L. C., Botto E. N., López S. N. (2016). Inherited sterility in tuta absoluta(Lepidoptera: Gelechiidae): pest population suppression and potential for combined use with a generalist predator. Fla. Entomol. 99, 87–94. doi:10.1653/024.099.sp112
Cagnotti C. L., Viscarret M. M., Riquelme M. B., Botto E. N., Carabajal L. Z., Segura D. F., et al. (2012). Effects of X-rays on Tuta absoluta for use in inherited sterility programmes. J. Pest Sci. 85, 413–421. doi:10.1007/s10340-012-0455-9
Cagnotti C. L., Viscarret M. M., Riquelme M. B., Botto E. N., Carabajal L. Z., Segura D. F., et al. (2012). Effects of X-rays on Tuta absoluta for use in inherited sterility programmes. J. Pest Sci. 85, 413–421. doi:10.1007/s10340-012-0455-9
Carpenter J. E., Gross H. R. (1993). Suppression of feral Helicoverpa zea (Lepidoptera: Noctuidae) populations following the infusion of inherited sterility from released substerile males. Environ. Entomol. 22, 1084–1091. doi:10.1093/ee/22.5.1084
Chi H. (1988). Life-table analysis incorporating both sexes and variable development rates among individuals. Environ. Entomol. 17, 26–34. doi:10.1093/ee/17.1.26
Chi H. S. I. N., Liu H. S. I. (1985). Two new methods for the study of insect population ecology. Bull. Inst. Zool. Acad. Sin. 24, 225
Chi H. (2009). TWOSEX-MSChart: a computer program for age-stage, two sex life table analysis. Available at: http://140.120.197.173/Ecology/prod02.htm (Accessed October 12, 2012).
Chi H., You M., Atlıhan R., Smith C. L., Kavousi A., Özgökçe M. S., et al. (2020). Age-stage, two-sex life table: an introduction to theory, data analysis, and application. entomologia 40, 103–124. doi:10.1127/entomologia/2020/0936
Du W., Hu C., Yu C., Tong J., Qiu J., Zhang S., et al. (2019). Comparison between pupal and adult X-ray radiation, designed for the sterile insect technique for Aedes albopictus control. Acta Trop. 199, 105110–110. doi:10.1016/j.actatropica.2019.105110
Ducoff H. S., Bosma G. C. (1966). The influence of pupal age on sensitivity to radiation. Biol. Bull. 130, 151–156. doi:10.2307/1539693
Ehler L. E. (2006). Integrated pest management (IPM): definition, historical development and implementation, and the other IPM. Pest. Manag. Sci. 62, 787–789. doi:10.1002/ps.1247
Fan X., Niemira B. A. (2020). Gamma ray, electron beam, and X-ray irradiation. Food Saf. Eng., 471–492. doi:10.1007/978-3-030-42660-6_18
Fu X., Zhao X., Xie B., Ali A., Wu K. (2015). Seasonal pattern of Spodoptera litura (Lepidoptera: Noctuidae) migration across the bohai strait in northern China. J. Econ. Entomology 108, 525–538. doi:10.1093/jee/tov019
Ge S. S., He L. M., He W., Xu R. B., Sun X. T., Wu K. M. (2019). Determination on moth flight capacity of Spodoptera frugiperda. Plant Prot. 45, 28–33. doi:10.16688/j.zwbh.2019322
Greene G. L., Leppla N. C., Dickerson W. A. (1976). Velvetbean caterpillar: a rearing procedure and artificial medium123. J. Econ. Entomol. 69, 487–488. doi:10.1093/jee/69.4.487
Gupta G. P., Rani S., Birah A., Raghuraman M. (2005). Improved artificial diet for mass rearing of the tobacco caterpillar, Spodoptera litura (Lepidoptera: Noctuidae). Jti 25, 55–58. doi:10.1079/ijt200551
Haff R., Ovchinnikova I., Liang P., Mahoney N., Gee W., Gomez J., et al. (2020). X-ray-based irradiation of larvae and pupae of the navel orangeworm (Lepidoptera: Pyralidae). J. Econ. Entomol. 113, 1685–1693. doi:10.1093/jee/toaa111
Helinski M. E. H., Knols B. G. J. (2009). The influence of late-stage pupal irradiation and increased irradiated: un-irradiated male ratio on mating competitiveness of the malaria mosquito Anopheles arabiensis patton. Bull. Entomol. Res. 99, 317–322. doi:10.1017/s0007485308006354
Hendrichs J., Ortiz G., Liedo P., Schwarz A. (1983). “Six years of successful medfly program in Mexico and Guatemala,” in fruit flies of economic importance. Editor R. Cavallaro (Rotterdam, Netherlands: Commission of the European Communities), 353
Hendrichs J., Robinson A. S., Cayol J. P., Enkerlin W. (2002). Medfly areawide sterile insect technique programmes for prevention, suppression or eradication: The importance of mating behavior studies. Fla. Entomol. 85, 1–13. doi:10.1653/0015-4040(2002)085[0001:masitp]2.0.co;2
Hjørringgaard J. G., Ankjærgaard C., Bailey M., Miller A. (2020). Alanine pellet dosimeter efficiency in a 40 kV x-ray beam relative to cobalt-60. Radiat. Meas. 136, 106374. doi:10.1016/j.radmeas.2020.106374
Hofmeyr J. H., Carpenter J. E., Bloem S. (2005). Developing the sterile insect technique for Cryptophlebia leucotreta (Lepidoptera: Tortricidae): Influence of radiation dose and release ratio on fruit damage and population growth in field cages. J. Econ. Entomol. 98, 1924–1929. doi:10.1093/jee/98.6.1924
Jessup A. J., Dominiak B., Woods B., De Lima C. P. F., Tomkins A., Smallridge C. J. (2007). “The use of massive SIT for the control of the medfly, Ceratitis capitata (wied.), strain SEIB 6–96, in mendoza, Argentina,” in area-wide control of fruit flies and other insect pests. Editors K. H. Tan Penang (Penerbit Universiti Sains Malaysia), 685–697. doi:10.1007/978-1-4020-6059-5_63
Jiang S., He L. M., He W., Zhao H. Y., Yang X. M., Yang X. Q., et al. (2022). Effects of X-ray irradiation on the fitness of the established invasive pest fall armyworm Spodoptera frugiperda. Pest Manag. Sci. 78, 2806–2815. doi:10.1002/ps.6903
Kim J., Jung S.-O., Jang S. A., Kim J., Park C. G. (2015). X-ray radiation and development inhibition of helicoverpa armigera hübner (Lepidoptera: Noctuidae). Radiat. Phys. Chem. 115, 148–152. doi:10.1016/j.radphyschem.2015.06.021
Klassen W., Vreysen M. J. B. (2021). “Area-wide integrated pest management and the sterile insect technique,” in sterile insect technique: Principles and practice in area-wide integrated pest management. Editors V. A. D. J. Hendrichs,, and A. S. Robinson (Dordrecht: Springer), 75–112. doi:10.1201/9781003035572-3
Knipling E. F. (1955). Possibilities of insect control or eradication through the use of sexually sterile males1. J. Econ. Entomol. 48, 459–462. doi:10.1093/jee/48.4.459
Knipling E. F. (1960). The eradication of the screw-worm fly. Sci. Am. 203, 54–61. doi:10.1038/scientificamerican1060-54
Knipling E. F. (1964). “Trends of an insect population subjected to an integrated program of sterile 546 insect releases and conventional methods of control,” in The potential role of the sterility method for insect population control with special reference to combining this method with conventional methods, Editor E. F. Knipling, (Washington DC: US Department of Agriculture, Agricultural Research Service), 8. doi:10.5962/bhl.title.67214
Koyama J., Kakinohana H., Miyatake T. (2004). Eradication of the melon fly, Bactrocera cucurbitae, in Japan: importance of behavior, ecology, genetics, and evolution. Annu. Rev. Entomol. 49, 331–349. doi:10.1146/annurev.ento.49.061802.123224
Kumano N., Haraguchi D., Tsurui-Sato K. (2018). Effects of X-ray irradiation on male sperm transfer ability and fertility in the sweetpotato weevils Euscepes postfasciatus (Coleoptera: Curculionidae) and Cylas formicarius (Coleoptera: Brentidae). Appl. Entomol. Zool. 53, 485–492. doi:10.1007/s13355-018-0578-4
LaChance L. E. (1967). “The induction of dominant lethal mutations in insects by ionizing radiation and chemicals as related to the sterile male technique of insect control,” in Genetics of insect vectors of disease. Editors J. W. Wright,, and R. Pal (Amsterdam: Elsevier), 617
LaChance L. E., Richard R. D., Proshold F. I. (1975). Radiation response in the pink bollworm: 1 a comparative study of sperm bundle production, sperm transfer, and oviposition response elicited by native and laboratory-reared males 3. Environ. Entomol. 4, 321–324. doi:10.1093/ee/4.2.321
Light D. M., Ovchinnikova I., Jackson E. S., Haff R. P. (2015). Effects of x-ray irradiation on male navel orangeworm moths (Lepidoptera: Pyralidae) on mating, fecundity, fertility, and inherited sterility. J. Econ. Entomol. 108, 2200–2212. doi:10.1093/jee/tov201
Marec F., Traut W. (1993). Synaptonemal complexes in female and male meiotic prophase of Ephestia kuehniella (Lepidoptera). Heredity 71, 394–404. doi:10.1038/hdy.1993.154
Mastrangelo T., Kovaleski A., Botteon V., Scopel W., Costa M. D. L. Z. (2018). Optimization of the sterilizing doses and overflooding ratios for the South American fruit fly. PLoS One 13, e0201026. doi:10.1371/journal.pone.0201026
Mastrangelo T., Parker A. G., Jessup A., Pereira R., Orozco-Dávila D., Islam A., et al. (2010). A new generation of X ray irradiators for insect sterilization. J. Econ. Entomol. 103, 85–94. doi:10.1603/ec09139
Mochida O., Miyahara Y. (1974). Effect of gamma radiation on male pupae and adults of Spodoptera litura (F.) (Lepidoptera : Noctuidae) following pupal exposure. Appl. Entomol. Zool. 9, 41–47. doi:10.1303/aez.9.41
North D. T., Holt G. G. (1969). Population suppression by transmission of inherited sterility to progeny of irradiated cabbage loopers, Trichoplusia ni. Can. Entomol. 101, 513–520. doi:10.4039/ent101513-5
North D. T. (1975). Inherited sterility in Lepidoptera. Annu. Rev. Entomol. 20, 167–182. doi:10.1146/annurev.en.20.010175.001123
Ouye M. T., Garcla R. S., Martin D. F. (1964). Determination of the optimum sterilizing dosage for pink bollworms treated as pupae with gamma Radiation2. J. Econ. Entomol. 57, 387–390. doi:10.1093/jee/57.3.387
Peloquin J. J., Thibault S. T., Staten R., Miller T. A. (2000). Germ-line transformation of pink bollworm (Lepidoptera: Gelechiidae) mediated by the piggyBac transposable element. Insect Mol. Biol. 9, 323–333. doi:10.1046/j.1365-2583.2000.00194.x
Proverbs M. D., Newton J. R. (1962). Influence of gamma radiation on the development and fertility of the codling moth, Carpocapsa pomonella (L.) (Lepidoptera: Olethreutidae). Can. J. Zool. 40, 401–420. doi:10.1139/z62-038
Qin H. G., Wang D. D., Ding J., Huang R. H., Ye Z. X. (2006). Host plants of Spodoptera litura. Acta. Agr. jiangxi. 18, 51–58. doi:10.3969/j.issn.1001-8581.2006.05.017
Ranga Rao G. V., Wightman J. A., Ranga Rao D. V. (1993). World review of the natural enemies and diseases of Spodoptera litura (F.) (Lepidoptera: Noctuidae). Int. J. Trop. Insect Sci. 14, 273–284. doi:10.1017/s1742758400014764
Rendón P., McInnis D., Lance D., Stewart J. (2004). Medfly (Diptera:tephritidae) genetic sexing: large-scale field comparison of males-only and bisexual sterile fly releases in Guatemala. ec 97, 1547–1553. doi:10.1603/0022-0493-97.5.1547
Seth R. K., Barik T. K., Chauhan S. (2009). Interaction of entomopathogenic nematodes, Steinernema glaseri (Rhabditida: Steinernematidae), cultured in irradiated hosts, with ‘F1 sterility’: towards management of a tropical pest, Spodoptera litura (Fabr.) (Lepidoptera: Noctuidae). Biocontrol Sci. Technol. 19, 139–155. doi:10.1080/09583150902814515
Seth R. K., Kaur J. J., Rao D. K., Reynolds S. E. (2002). Sperm transfer during mating, movement of sperm in the female reproductive tract, and sperm precedence in the common cutworm Spodoptera litura. Physiol. Entomol. 27, 1–14. doi:10.1046/j.1365-3032.2002.00260.x
Seth R. K., Khan Z., Rao D. K., Zarin M. (2016a). Appraisal of sperm dynamics as a crucial trait of radiosterilized Spodoptera litura (Lepidoptera: Noctuidae) and its F1 progeny for evaluation of the ‘inherited sterility technique’for pest suppression. Fla. Entomol. 99, 105–118. doi:10.1653/024.099.sp114
Seth R. K., Khan Z., Rao D. K., Zarin M. (2016b). Flight activity and mating behavior of irradiated Spodoptera litura (Lepidoptera: Noctuidae) males and their F1 progeny for use of inherited sterility in pest management approaches. Fla. Entomol. 99, 119–130. doi:10.1653/024.099.sp115
Seth R. K., Rao D. K., Kaur J. J. (2000). Developmental pattern of testes in F1 progeny of gamma irradiated Spodoptera litura (Fabr.). J. Nucl. Agric. Biol. 29, 129
Seth R. K., Sehgal S. S. (1993). “Partial sterilizing radiation dose effect on the F1 progeny of Spodoptera litura (Fabr.): growth, bioenergetics and reproductive competence,” in Management of insect pests: Nuclear and related molecular and genetic techniques (Vienna: International Atomic Energy Agency), 427
Seth R. K., Sharma V. P. (2001). Inherited sterility by substerilizing radiation in Spodoptera litura (Lepidoptera: Noctuidae): bioefficacy and potential for pest suppression. Fla. Entomologist 84, 183–193. doi:10.2307/3496165
Seth R. K., Sharma V. P., Rao D. K., Kaur J. J. (1997). Pupa as a physiological and morphogenetic indicator for assessing F-1 sterility technique for management of Spodoptera litura (Fabricius). J. Nucl. Agric. Biol. 26, 247
Srivastava B. K., Joshi H. C. (1965). Occurrence of resistance to BHC in Prodenia litura fab. (Lepidoptera: Noctuidae). Indian J. Entomol. 27, 102–104.
Stephens A. E. A., Barrington A. M., Fletcher N. M., Suckling D. M. (2006). Irradiation conditions affect the quality of irradiated painted apple moth. Nzpp 59, 119–124. doi:10.30843/nzpp.2006.59.4443
Suckling D. M., Barrington A. M., Chhagan A., Stephens A. E. A., Burnip G. M., Charles J. G., et al. (2007). “Eradication of the Australian painted apple moth Teia anartoides in New Zealand: trapping, inherited sterility, and male competitiveness,” in Area-wide control of insect pests from research to field implementation. Editors M. J. B. Vreysen, A. S. Robinson, and J. Hendrichs (Dordrecht: Springer), 603–615. doi:10.1007/978-1-4020-6059-5_56
Suckling D. M., Hackett J. K., Barrington A. M., Daly J. M. (2002). Sterilisation of painted apple moth teia anortoides (Lepidoptera Lymantriidae) by irradiation. Nzpp 55, 7–11. doi:10.30843/nzpp.2002.55.3905
Tabashnik B. E., Liesner L. R., Ellsworth P. C., Unnithan G. C., Fabrick J. A., Naranjo S. E., et al. (2021). Transgenic cotton and sterile insect releases synergize eradication of pink bollworm a century after it invaded the united states. Proc. Natl. Acad. Sci. U.S.A. 118, 1–5. doi:10.1073/pnas.2019115118
Tamura T., Thibert C., Royer C., Kanda T., Eappen A., Kamba M., et al. (2000). Germline transformation of the silkworm Bombyx mori L. using a piggyBac transposon-derived vector. Nat. Biotechnol. 18, 81–84. doi:10.1038/7544610.1038/71978
Tojo S., Ryuda M., Fukuda T., Matsunaga T., Choi D.-R., Otuka A. (2013). Overseas migration of the common cutworm, Spodoptera litura (Lepidoptera: Noctuidae), from may to mid-july in east asia. Appl. Entomol. Zool. 48, 131–140. doi:10.1007/s13355-013-0162-x
Urquidi J., Brar R. K., Rodriguez S., Hansen I. (2015). “The development of new radiation protocols for insect sterilization using long wavelength X-rays,” in AIP conference proceedings. Editors E. Guillermo, V. L. Carlos, A. L. Jorge, and C. Ciudad Juárez (México: AIP Publishing LLC), 1671, 1–7. doi:10.1063/1.4927187
Wei M., Chi H., Guo Y., Li X., Zhao L., Ma R. (2020). Demography of Cacopsylla chinensis (Hemiptera: Psyllidae) reared on four cultivars of Pyrus bretschneideri (Rosales: Rosaceae) and P. communis pears with estimations of confidence intervals of specific life table statistics. J. Econ. Entomol. 113, 2343–2353. doi:10.1093/jee/toaa149
Wu H. R., Huang M. S., Lei C. L., Wan P. (2016). The spatial-temporal distribution of Spodoptera litura in China. J. Anhui Agric. Sci. 44, 142–144. doi:10.3969/j.issn.0517-6611.2016.09.049
Wyss J. H. (2006). Screwworm eradication in the americas. Ann. N. Y. Acad. Sci. 916, 186–193. doi:10.1111/j.1749-6632.2000.tb05289.x
Yamada H., Parker A. G., Oliva C. F., Balestrino F., Gilles J. R. L. (2014). X-Ray-induced sterility inAedes albopictus(Diptera: Culicidae) and male longevity following irradiation. J. Med. Entomol. 51, 811–816. doi:10.1603/me13223
Yosiaki I. T. O., Kakinohana H., Yamagishi M., Kohama T. (2003). Eradication of the melon fly, Bactrocera cucurbitae, from okinawa, japan, by means of the sterile insect technique, with special emphasis on the role of basic studies. J. Asia-Pac. Entomol. 6, 119–129. doi:10.1016/s1226-8615(08)60177-6
Yun S.-H., Lee S.-W., Koo H.-N., Kim G.-H. (2014). Assessment of electron beam-induced abnormal development and DNA damage in Spodoptera litura (F.) (Lepidoptera: Noctuidae). Radiat. Phys. Chem. 96, 44–49. doi:10.1016/j.radphyschem.2013.08.008
Zhang L. X., Liu X. Z. (1992). The analysis and application of the two important characteristic points on logistic curve. Hebei J. Fore. Orch. Res. 7, 154
Zhao J., Li S., Xu L., Li C., Li Q., Dewer Y., et al. (2022). Effects of X-ray irradiation on biological parameters and induced sterility of Ephestia elutella: establishing the optimum irradiation dose and stage. Front. Physiol. 13, 895882. doi:10.3389/fphys.2022.895882
Zhao Q., Zhang Y. H., Liu H., Cheng D. F. (2011). A method used for distinguishing between the sexes of Scotogramma trifolii. Chin. J. Appl. Entomol. 48, 1879–1881. doi:10.7679/j.issn.2095-1353.2011.289
Keywords: Spodoptera litura, sterile insect technology, X-ray, radiation biology, release ratios
Citation: Jiang S, Fu X-W, Jiang S-S, Yang X-M, Zhao H-Y and Wu K (2022) Effect of X-ray irradiation on development, flight, and reproduction of Spodoptera litura. Front. Physiol. 13:947848. doi: 10.3389/fphys.2022.947848
Received: 19 May 2022; Accepted: 27 June 2022;
Published: 18 July 2022.
Edited by:
Peng He, Guizhou University, ChinaReviewed by:
Maofa Yang, Guizhou University, ChinaHamzeh Izadi, Vali-E-Asr University of Rafsanjan, Iran
Daguang Lu, Joint FAO/IAEA Programme of Nuclear Techniques in Food and Agriculture, Austria
Copyright © 2022 Jiang, Fu, Jiang, Yang, Zhao and Wu. This is an open-access article distributed under the terms of the Creative Commons Attribution License (CC BY). The use, distribution or reproduction in other forums is permitted, provided the original author(s) and the copyright owner(s) are credited and that the original publication in this journal is cited, in accordance with accepted academic practice. No use, distribution or reproduction is permitted which does not comply with these terms.
*Correspondence: Kongming Wu, d3Vrb25nbWluZ0BjYWFzLmNu