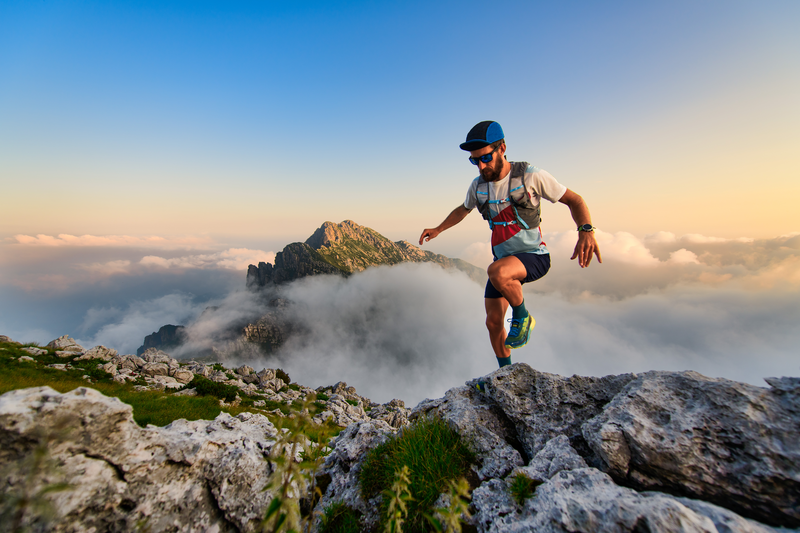
95% of researchers rate our articles as excellent or good
Learn more about the work of our research integrity team to safeguard the quality of each article we publish.
Find out more
ORIGINAL RESEARCH article
Front. Physiol. , 05 August 2022
Sec. Exercise Physiology
Volume 13 - 2022 | https://doi.org/10.3389/fphys.2022.945552
This article is part of the Research Topic Structural and Mechanistic Determinants of Endurance Performance View all 10 articles
Purpose: To compare endurance, strength and body composition indicators between cyclists of three different competition age categories.
Methods: Fifty-one male road cyclists classified as either junior (n = 13, age 16.4 ± 0.5 years), under-23 [(U23), n = 24, 19.2 ± 1.3 years] or professional (n = 14, 26.1 ± 4.8 years) were studied. Endurance (assessed through a maximal incremental test and an 8-minute time-trial), strength/power (assessed through incremental loading tests for the squat, lunge and hip thrust exercises) and body composition (assessed through dual energy X-ray absorptiometry) were determined on three different testing sessions.
Results: U23 and, particularly professional, cyclists attained significantly (p < 0.05) higher values than juniors for most of the analyzed endurance indicators [time-trial performance, maximum oxygen uptake (VO2max), peak power output (PPO), respiratory compensation point (RCP), and ventilatory threshold (VT)]. Significant differences (p < 0.05) between U23 and professionals were also found for time-trial performance, PPO and VT, but not for other markers such as VO2max or RCP. Professional cyclists also showed significantly (p < 0.05) lower relative fat mass and higher muscle mass levels than U23 and, particularly, juniors. No consistent differences between age categories were found for muscle strength/power indicators.
Conclusion: Endurance (particularly time-trial performance, PPO and VT) and body composition (fat and muscle mass) appear as factors that best differentiate between cyclists of different age categories, whereas no consistent differences are found for muscle strength/power. These findings might help in performance prediction and/or talent identification and may aid in guiding coaches in the design of training programs focused on improving those variables that appear more determinant.
The factors that contribute to top-level performance in sports remain largely unknown (Foster et al., 2022). For instance, in road cycling different indicators have been reported as determinants of performance, notably endurance-related indicators such as the capacity to produce high power outputs even in the presence of fatigue (Van Erp et al., 2021, 2021; Mateo-March et al., 2022; Valenzuela et al., 2022) or anthropometric indicators such as a low body mass (van der Zwaard et al., 2019; Leo et al., 2021b). Achieving the top level in any sport and, particularly, in road cycling is a long-term process that takes several years and requires specific characteristics that can be improved along the career trajectory, from junior to under-23 (U23), to finally reaching the professional category. However, there is scarce evidence on the actual physiological/performance differences between cyclists of different age categories that could help identify specific characteristics that should be improved in the younger categories to increase the odds of reaching top-level performance.
As with most endurance sports, cycling performance has been reported to be mainly determined by physiological endurance indicators such as maximum oxygen uptake (VO2max), the so-called ‘lactate threshold’ [or other surrogates such as critical power or ventilatory threshold (VT)], and exercise economy (Joyner and Coyle, 2008; van der Zwaard et al., 2021). Indeed, strong evidence supports these laboratory-based indicators as predictors of cycling performance (Hawley and Noakes, 1992; Nichols et al., 1997; Bishop et al., 1998, 2000; Balmer et al., 2000; Bentley et al., 2001; Amann et al., 2006), and some studies have reported that indicators such as maximal aerobic capacity [represented by VO2max or peak power output (PPO)] might be positively associated with the probability of a junior cyclist reaching the professional category (Svendsen et al., 2018). Controversy exists, however, on whether these endurance indicators can accurately differentiate cyclists of different age categories. Thus, whereas some authors have found a higher VO2max or PPO in professional cyclists than in cyclists of lower categories [amateur (∼22 years) or junior (∼18 years)] (Pérez-Landaluce et al., 2002), have failed to find such differences (Marín-Pagán et al., 2021).
Notably, while some studies have compared the performance (Leo et al., 2022), training/competition characteristics (Leo et al., 2021a) or endurance capabilities (Pérez-Landaluce et al., 2002; Leo et al., 2021a; Marín-Pagán et al., 2021) between cyclists of different age categories, there is little or no evidence on whether other important factors such as muscle strength or body composition also differ. Accumulating evidence suggests that muscle strength/power plays a major role in cycling performance. For example, Kordi et al. recently reported that knee extension maximum voluntary torque was positively associated with both critical power and with the amount of work that can be completed above this intensity (known as W’ and considered as a marker of the so-called “anaerobic” capacity) (van der Zwaard et al., 2019; Kordi et al., 2021). Likewise, body composition has been reported to condition cycling performance. For instance, muscle mass indicators such as quadriceps volume have been identified as one of the main determinants of peak power production capacity and W’ in cyclists (Miura et al., 2002; Kordi et al., 2018, 2020a; van der Zwaard et al., 2019; Douglas et al., 2021). To the best of our knowledge, however, no previous study has determined whether strength/power or body composition indicators differ between cyclists of different age categories.
The aim of the present study was, therefore, to compare endurance, strength and body composition indicators between cyclists of three different categories (junior, U23 and professional).
Fifty-one male road cyclists classified as junior (n = 13, age 16.4 ± 0.5 years), U23 (n = 24, 19.2 ± 1.3 years) or professionals [Union Cycliste Internationale (UCI) Pro-Team] (n = 14, 26.1 ± 4.8 years) volunteered to participate in the study. U23 and professional cyclists competed actively at national or international level (including in European or World Championships with the national team of their country of birth). All cyclists were assessed at the end of the ‘pre-season’ period, after at least 2 months had elapsed since the last competition of the previous season. To participate in the study, cyclists had to be free of musculoskeletal injuries or other conditions that could hinder their participation. They were all informed of the study procedures and provided written informed consent. The study was approved by the Ethical Committee of Alcorcón University Hospital (approval number 19/86), and all procedures were conducted following the standards established by the Declaration of Helsinki and its later amendments.
The study followed a cross-sectional observational design. Participants visited the laboratory on three different days interspersed by 48 h. All tests were performed at approximately the same time of the day and under the same conditions (temperature 20 ± 3 °C, humidity 25 ± 3%). Subjects were instructed to maintain their normal dietary pattern and to refrain from doing intense exercise and consuming ergogenic aids/caffeine 48 h before each testing session. The first laboratory visit consisted of body composition assessment and a maximal incremental cycling test. During their second visit, subjects completed strength tests, and during the third visit they performed a simulated 8-minute time-trial.
Height was determined using a wall stadiometer (Seca 437). Weight and body composition [whole body fat and muscle mass, and bone mineral content (BMC)] was measured by dual energy X-ray absorptiometry (DXA, Hologic QDR series Discovery; Bedford, MA). DXA assessments were performed at least 2 days after the last exercise session. Participants were recommended to maintain a similar eating and sleeping routine the day before each testing session and were advised to be euhydrated.
Cyclists performed a graded exercise test on their own bikes, which were placed on a validated indoor trainer (Hammer, CycleOps, Madison, WI) (Lillo-Bevia and Pallarés, 2018). They started with a standardized 10-minute warm-up at 75 W, and they subsequently accomplished a maximal incremental cycling test with an initial workload of 75 W, increasing by 5 W every 12 s (ramp-like protocol) until volitional exhaustion or when pedaling cadence could not be maintained above 60 rpm. Gas exchange data were collected breath-by-breath (Ultima Series Medgraphics, Cardiorespiratory Diagnostics, Saint Paul, MN). The VT was determined as the workload at which an increase in both the ventilatory equivalent for oxygen (VE∙VO−1) and end-tidal partial pressure of carbon dioxide (PetCO2) occurred with no concomitant increase in the ventilatory equivalent for carbon dioxide (VE∙VCO−1), whereas the respiratory compensation point (RCP, also termed “second ventilatory threshold”) corresponded to the work rate at which both VE∙VO−1 and VE∙VCO−1 increased together with a decrease in PetCO2 (Lucía et al., 2000). PPO was defined as the highest power output value reached during the test, and VO2max was defined as the highest VO2 value (mean of 10 s) attained during the test.
Participants performed an incremental loading test on a Smith machine to assess muscle strength and power-related outcomes in the squat, lunge and hip-thrust exercises, as explained elsewhere (Gil-Cabrera et al., 2021; Valenzuela et al., 2021). Bar mean propulsive power (MPP) during the concentric phase was measured with a validated linear position transducer (T-Force System; Ergotech, Murcia, Spain) (Martínez-Cava et al., 2020). The initial weight was 20 kg (i.e., only the bar), and the load was increased by 10 kg until a decrease in MPP was observed in two consecutive loads. Participants performed three consecutive repetitions with each load, and a 2-minute rest was allowed between loads. We analyzed the highest MPP registered for each exercise and the one-repetition maximum (1RM) was estimated as explained elsewhere (Gil-Cabrera et al., 2021; Valenzuela et al., 2021).
During the third visit, cyclists performed a simulated 8-minute time-trial after a standardized 10-minute warm-up at 60% of their PPO. Participants received no instructions regarding pacing and were blinded to power output values during the trial, but they were instructed to attain the highest mean power output possible, and they were allowed to adjust resistance by changing the gears of the bicycle. The mean power output during an 8-minute time trial has been reported as a valid indicator of performance in cyclists, being also correlated with laboratory-based performance indicators (e.g., lactate threshold) (Klika et al., 2007; Gavin et al., 2012; Sanders et al., 2020).
Data are presented as mean ± SD. Normality (Kolmogorov–Smirnov test) and homoscedasticity (Levene’s test) of the data were checked prior to any statistical treatment. Differences between age categories were performed by one-way analysis of variance. In order to reduce the risk of type I error, Bonferroni post-hoc tests were only conducted when a significant group effect was found. Effect sizes (Cohen’s d) were computed to examine the magnitude of the differences and considered small, moderate, large, very large and extremely large (d > 0.1, > 0.3, > 0.5, > 0.7 or > 0.9, respectively) (Hopkins et al., 2009) (Hopkins et al., 2009). Statistical analyses were performed with specific statistical software (SPSS 26.0, Inc., Chicago, IL, United States), setting the alpha for significance at 0.05.
Differences between age categories for endurance, strength and body composition indicators are shown in Table 1, Table 2 and Table 3, respectively.
A significant group effect was observed for all endurance indicators, with a linear increase from juniors to professionals in most analyzed variables (Table 1). Accordingly, U23 and, particularly, professionals attained significantly higher values than juniors for most of the analyzed endurance indicators (i.e., 8-minute time-trial performance, VO2max, PPO, RCP, and VT). Differences between U23 and professionals were found only for the 8-minute time-trial, PPO and VT (all of them expressed in both absolute and relative units) and for the relative workload (%VO2max) at which the VT occurred. No differences were found for VO2max or RCP. Of note, no consistent linear association (i.e., no increase from juniors to professionals) was observed for the relative workload (%VO2max) at which the VT and the RCP occurred.
Contrary to endurance indicators, no significant group effects were found for the analyzed strength indicators with the exception of the squat absolute MMP, which was higher in professionals than in juniors (Table 2). Likewise, no significant group effect was found for anthropometric variables such as body mass, BMI, BMC or absolute fat mass (Table 3). However, a significant group effect was found for relative fat mass—with professionals showing a significantly lower relative fat mass than both juniors and U23—as well as for absolute and relative muscle mass, both of which were higher among professionals than in U23 and, particularly, juniors.
The main finding of the present study is that U23 and, particularly, professional cyclists differed from junior cyclists for most of the laboratory-based endurance indicators (e.g., VO2max, PPO, RCP, VT and simulated 8-minute time-trial performance). Similarly, there were evident anthropometric differences between professional cyclists and the other two groups, including lower relative fat mass and higher muscle mass levels. Finally, professional cyclists also had higher values for many endurance indicators compared with U23 cyclists, although no differences were found for major markers such as VO2max. Likewise, no consistent differences were found between age categories for strength/power indicators. Although longitudinal studies are warranted to confirm the influence of the abovementioned endurance and anthropometric indicators on performance, these findings might serve for performance prediction and talent identification, as well as to aid coaches in the design of training programs aimed at improving those variables that appear more determinant.
The utility of laboratory-based endurance indicators as determinants of cycling performance has been widely reported. In addition to the 8-minute time-trial performance, which can be considered an indicator of ‘actual’ performance—and indeed differed between all three age categories in the present study—most of the analyzed endurance indicators showed different results between junior cyclists and the remaining age categories, but only some indicators such as PPO (but not VO2max) differed between professionals and U23 cyclists. PPO, therefore, appears as a more sensitive marker of performance than VO2max. In line with this finding, Marín-Pagán et al. (2021) found that relative PPO was greater in U23 cyclists than in junior cyclists, with no differences in VO2max. Moreover, PPO has been reported to be more strongly correlated with cycling performance than VO2max (Bishop et al., 1998, 2000; Bentley et al., 2001). In this line, Menaspà et al. (2012) reported that while VO2max can discern between junior cyclists of different levels, its accuracy to predict future success (becoming professional) is limited. On the other hand, VT was also different between all three age categories, which is also in accordance with previous studies reporting stronger correlations between cycling performance and threshold-related parameters than for other indicators such as VO2max (Nichols et al., 1997; Bishop et al., 1998, 2000; Bentley et al., 2001; Michalik et al., 2019). Thus, PPO and VT appear as more sensitive markers of performance (and a more sensitive differentiating factor between age categories) than VO2max in well-trained or elite cyclists.
It is noteworthy that no linear association (i.e., no consistent increase from juniors to professionals) was observed for the relative workload (%VO2max) at which VT and RCP occurred. The fraction of VO2max that can be sustained for a given period of time has been traditionally proposed as a marker of endurance performance (Joyner and Coyle, 2008), and it has been reported that trained subjects might have their lactate threshold (overall equivalent to ventilatory thresholds) at a higher %VO2max than untrained peers (Joyner and Coyle, 2008), although this is controversial. In the present study U23 cyclists attained the RCP at a higher %VO2max than juniors, but no differences were found between U23 and professionals. Similarly, professional cyclists attained the VT at a higher %VO2max than U23 cyclists, but no differences were found between professionals and juniors, or between U23 and juniors. In line with these findings, a recent study reported that the fraction of %VO2max at which the lactate threshold occurs was a poor individual predictor of performance (running velocity) and did not differentiate between elite, national and recreational runners (Støa et al., 2020). Therefore, the fraction of VO2max at which thresholds occur should not be used individually as a predictor of endurance performance, unless it is used in combination with other parameters such as maximal aerobic speed or PPO (Støa et al., 2020).
Another major finding of the present study was that, although no differences in body mass were found between age categories, professional cyclists had a lower relative fat mass and higher levels of muscle mass than U23 and juniors. Previous studies have suggested that muscle mass indicators (e.g., quadriceps or thigh muscle cross-sectional area or volume) are positively associated with cycling performance, at least for short-duration efforts (Miura et al., 2002; Kordi et al., 2018, 2020a). In turn, a higher body mass can be negatively associated with performance (i.e., riding speed) owing to the influence of gravity, particularly on the steepest slopes. Thus, it seems that professional cyclists present with higher levels of muscle mass, which can be potentially associated with a greater power production capacity, but in turn a lower fat mass, which enables them to maintain an optimal body mass. Body composition appears, therefore, as a major differentiating factor between cyclists of different age categories.
Contrary to the findings for endurance indicators and body composition, no consistent differences between age categories were found for muscle strength/power indicators. A positive association between maximal strength and cycling performance has been previously reported, at least for short-duration efforts (Kordi et al., 2018, 2021). However, the association between strength capabilities and performance on longer efforts remains unclear. For instance, no associations have been found between critical power and knee extensor torque or back squat 1RM (Kordi et al., 2018; Byrd et al., 2021). Thus, although strength training has proven beneficial for cycling performance (Rønnestad et al., 2015, 2017; Kordi et al., 2020b), strength/power levels measured through an incremental loading test on a Smith machine in three different exercises seem not to be a major determinant of performance.
Some limitations of the present study should be noted. Despite the observed differences between groups, the cross-sectional design of the study precludes from drawing conclusions on performance prediction. Therefore, longitudinal studies are warranted to determine whether these variables could be used to accurately predict performance in young cyclists. Moreover, given that a large number of variables was assessed, the presence of type I error should not be disregarded. However, it must be noted that most variables would remain significant even when applying a stringent threshold p-value (i.e., p < 0.001). Finally, some methodological considerations should be also taken in mind. We used an incremental ramp protocol with short steps, which might influence the absolute and relative (%VO2max) position of ventilatory thresholds compared to longer steps (Bentley et al., 2007; Michalik et al., 2019). Moreover, the inclusion of a longer (e.g., 20 or 40 km) time trial, of other strength/power measures such as isometric strength tests (maximal torque and rate of force development) or Wingate anaerobic test, or other body composition indicators such as quadriceps’ cross-sectional area, could have provided additional information.
In summary, endurance indicators (e.g., VO2max, PPO, RCP, VT, simulated 8-minute time-trial performance) seem to be the main differentiating factor between junior cyclists and higher categories. Smaller, yet significant, differences in endurance indicators were also found between U23 and professional cyclists, with the latter showing higher time-trial performance, PPO and VT, but without differences in VO2max and RCP. Some differences were also found in anthropometric parameters, with professional cyclists showing a lower relative fat mass and higher muscle mass compared with the remaining age categories. No consistent differences were found between age categories for strength/power indicators. Although longitudinal studies are needed to confirm these findings, the present results might help in performance prediction and/or talent identification and could also aid coaches in the design of training programs focused on improving those variables that appear more determinant.
The raw data supporting the conclusion of this article will be made available by the authors, without undue reservation.
The studies involving human participants were reviewed and approved by The study was approved by the Ethical Committee of Alcorcón University Hospital (approval number 19/86). Written informed consent to participate in this study was provided by the participants’ legal guardian/next of kin.
Conception and design of the experiments: LA, AM-P, PV, DB-G, CR, LO, VC, and MM-M. Experimental preparation and data collection: LA, AM-P, CR, DB-G, LO, VC, and AS. Analysis and interpretation: DB-G, PV, and LA. Drafting of the manuscript: AL, AS, PV, DB-G, LA, and MM-M. Revision of the manuscript for important intellectual content: AL, AS, PV, DB-G, and LA. All authors read and approved the final version of the manuscript.
Research by PV is funded by a postdoctoral contract from the Instituto de Salud Carlos III (Sara Borrell, CD21/00138). Research by AL is funded by the Spanish Ministry of Economy and Competitiveness and Fondos FEDER (PI18/00139).
We sincerely thank the Caja Rural professional team, Caja Rural development team, MMR Academy U18 team and all the participants for their help and cooperation during the study. The resistance training equipment used in this study was kindly provided by Life Fitness (Barcelona, Spain).
The authors declare that the research was conducted in the absence of any commercial or financial relationships that could be construed as a potential conflict of interest.
All claims expressed in this article are solely those of the authors and do not necessarily represent those of their affiliated organizations, or those of the publisher, the editors and the reviewers. Any product that may be evaluated in this article, or claim that may be made by its manufacturer, is not guaranteed or endorsed by the publisher.
Amann M., Subudhi A. W., Foster C. (2006). Predictive validity of ventilatory and lactate thresholds for cycling time trial performance. Scand. J. Med. Sci. Sports 16, 27–34. doi:10.1111/j.1600-0838.2004.00424.x
Balmer J., Davison R. C., Bird S. R. (2000). Peak power predicts performance power during an outdoor 16.1-km cycling time trial. Med. Sci. Sports Exerc. 32, 1485–1490. doi:10.1097/00005768-200008000-00018
Bentley D. J., McNaughton L. R., Thompson D., Vleck V. E., Batterham A. M. (2001). Peak power output, the lactate threshold, and time trial performance in cyclists. Med. Sci. Sports Exerc. 33, 2077–2081. doi:10.1097/00005768-200112000-00016
Bentley D. J., Newell J., Bishop D. (2007). Incremental exercise test design and analysis: Implications for performance diagnostics in endurance athletes. Sports Med. 37, 575–586. doi:10.2165/00007256-200737070-00002
Bishop D., Jenkins D. G., Mackinnon L. T. (1998). The relationship between plasma lactate parameters, Wpeak and 1-h cycling performance in women. Med. Sci. Sports Exerc. 30, 1270–1275. doi:10.1097/00005768-199808000-00014
Bishop D., Jenkins D. G., McEniery M., Carey M. F. (2000). Relationship between plasma lactate parameters and muscle characteristics in female cyclists. Med. Sci. Sports Exerc. 32, 1088–1093. doi:10.1097/00005768-200006000-00008
Byrd M. T., Wallace B. J., Clasey J. L., Bergstrom H. C. (2021). Contributions of lower-body strength parameters to critical power and anaerobic work capacity. J. Strength Cond. Res. 35, 97–101. doi:10.1519/JSC.0000000000002555
Douglas J., Ross A., Martin J. C. (2021). Maximal muscular power: Lessons from sprint cycling. Sports Med. Open 7, 48. doi:10.1186/s40798-021-00341-7
Foster C., Barroso R., Beneke R., Bok D., Boullosa D., Casado A., et al. (2022). How to succeed as an athlete: What we know, what we need to know. Int. J. Sports Physiol. Perform. 17, 333–334. doi:10.1123/ijspp.2021-0541
Gavin T. P., Van Meter J. B., Brophy P. M., Dubis G. S., Potts K. N., Hickner R. C., et al. (2012). Comparison of a field-based test to estimate functional threshold power and power output at lactate threshold. J. Strength Cond. Res. 26, 416–421. doi:10.1519/JSC.0b013e318220b4eb
Gil-Cabrera J., Valenzuela P. L., Alejo L. B., Talavera E., Montalvo-Pérez A., Lucia A., et al. (2021). Traditional versus optimum power load training in professional cyclists: A randomized controlled trial. Int. J. Sports Physiol. Perform. 16, 496–503. doi:10.1123/ijspp.2020-0130
Hawley J. A., Noakes T. D. (1992). Peak power output predicts maximal oxygen uptake and performance time in trained cyclists. Eur. J. Appl. Physiol. Occup. Physiol. 65, 79–83. doi:10.1007/BF01466278
Hopkins W. G., Marshall S. W., Batterham A. M., Hanin J. (2009). Progressive statistics for studies in sports medicine and exercise science. Med. Sci. Sports Exerc. 41, 3–13. doi:10.1249/MSS.0b013e31818cb278
Joyner M. J., Coyle E. F. (2008). Endurance exercise performance: The physiology of champions. J. Physiol. 586, 35–44. doi:10.1113/jphysiol.2007.143834
Klika R. J., Alderdice M. S., Kvale J. J., Kearney J. T. (2007). Efficacy of cycling training based on a power field test. J. Strength Cond. Res. 21, 265–269. doi:10.1519/00124278-200702000-00047
Kordi M., Folland J., Goodall S., Haralabidis N., Maden-Wilkinson T., Sarika Patel T., et al. (2020a). Mechanical and morphological determinants of peak power output in elite cyclists. Scand. J. Med. Sci. Sports 30, 227–237. doi:10.1111/sms.13570
Kordi M., Folland J. P., Goodall S., Menzies C., Patel T. S., Evans M., et al. (2020b). Cycling-specific isometric resistance training improves peak power output in elite sprint cyclists. Scand. J. Med. Sci. Sports 30, 1594–1604. doi:10.1111/sms.13742
Kordi M., Menzies C., Parker Simpson L. (2018). Relationship between power–duration parameters and mechanical and anthropometric properties of the thigh in elite cyclists. Eur. J. Appl. Physiol. 118, 637–645. doi:10.1007/s00421-018-3807-1
Kordi M., Parker Simpson L., Thomas K., Goodall S., Maden-Wilkinson T., Menzies C., et al. (2021). The relationship between neuromuscular function and the W’ in elite cyclists. Int. J. Sports Physiol. Perform. 16, 1656–1662. doi:10.1123/ijspp.2020-0861
Leo P., Spragg J., Mujika I., Giorgi A., Lorang D., Simon D., et al. (2021a). Power profiling, workload characteristics, and race performance of U23 and professional cyclists during the multistage race tour of the alps. Int. J. Sports Physiol. Perform. 16, 1089–1095. doi:10.1123/ijspp.2020-0381
Leo P., Spragg J., Mujika I., Menz V., Lawley J. S. (2021b). Power profiling in U23 professional cyclists during a competitive season. Int. J. Sports Physiol. Perform. 16, 881–889. doi:10.1123/ijspp.2020-0200
Leo P., Spragg J., Podlogar T., Lawley J. S., Mujika I. (2022). Power profiling and the power-duration relationship in cycling: A narrative review. Eur. J. Appl. Physiol. 122, 301–316. doi:10.1007/s00421-021-04833-y
Lillo-Bevia J. R., Pallarés J. G. (2018). Validity and reliability of the cycleops hammer cycle ergometer. Int. J. Sports Physiol. Perform. 13, 853–859. doi:10.1123/ijspp.2017-0403
Lucía A., Hoyos J., Pérez M., Chicharro J. L. (2000). Heart rate and performance parameters in elite cyclists: A longitudinal study. Med. Sci. Sports Exerc. 32, 1777–1782. doi:10.1097/00005768-200010000-00018
Marín-Pagán C., Dufour S., Freitas T. T., Alcaraz P. E. (2021). Performance profile among age categories in young cyclists. Biol. (Basel) 10, 1196. doi:10.3390/biology10111196
Martínez-Cava A., Hernández-Belmonte A., Courel-Ibáñez J., Morán-Navarro R., González-Badillo J. J., Pallarés J. G., et al. (2020). Reliability of technologies to measure the barbell velocity: Implications for monitoring resistance training. PLoS ONE 15, e0232465. doi:10.1371/journal.pone.0232465
Mateo-March M., Valenzuela P. L., Muriel X., Gandia-Soriano A., Zabala M., Lucia A., et al. (2022). The record power profile of male professional cyclists: Fatigue matters. Int. J. Sports Physiol. Perform. 17, 926–931. doi:10.1123/ijspp.2021-0403
Menaspà P., Rampinini E., Bosio A., Carlomagno D., Riggio M., Sassi A. (2012). Physiological and anthropometric characteristics of junior cyclists of different specialties and performance levels. Scand. J. Med. Sci. Sports 22, 392–398. doi:10.1111/j.1600-0838.2010.01168.x
Michalik K., Danek N., Zatoń M. (2019). Assessment of the physical fitness of road cyclists in the step and ramp protocols of the incremental test. J. Sports Med. Phys. Fit. 59, 1285–1291. doi:10.23736/S0022-4707.19.09126-6
Miura A., Endo M., Sato H., Sato H., Barstow T. J., Fukuba Y., et al. (2002). Relationship between the curvature constant parameter of the power-duration curve and muscle cross-sectional area of the thigh for cycle ergometry in humans. Eur. J. Appl. Physiol. 87, 238–244. doi:10.1007/s00421-002-0623-3
Nichols J. F., Phares S. L., Buono M. J. (1997). Relationship between blood lactate response to exercise and endurance performance in competitive female master cyclists. Int. J. Sports Med. 18, 458–463. doi:10.1055/s-2007-972664
Pérez-Landaluce J., Fernández-García B., Rodríguez-Alonso M., García-Herrero F., García-Zapico P., Patterson A. M., et al. (2002). Physiological differences and rating of perceived exertion (RPE) in professional, amateur and young cyclists. J. Sports Med. Phys. Fit. 42, 389–395.
Rønnestad B. R., Hansen J., Hollan I., Ellefsen S. (2015). Strength training improves performance and pedaling characteristics in elite cyclists. Scand. J. Med. Sci. Sports 25, e89–98. doi:10.1111/sms.12257
Rønnestad B. R., Hansen J., Nygaard H. (2017). 10 weeks of heavy strength training improves performance-related measurements in elite cyclists. J. Sports Sci. 35, 1435–1441. doi:10.1080/02640414.2016.1215499
Sanders D., Taylor R. J., Myers T., Akubat I. (2020). A field-based cycling test to assess predictors of endurance performance and establishing training zones. J. Strength Cond. Res. 34, 3482–3488. doi:10.1519/JSC.0000000000001910
Støa E. M., Helgerud J., Rønnestad B. R., Hansen J., Ellefsen S., Støren Ø. (2020). Factors influencing running velocity at lactate threshold in male and female runners at different levels of performance. Front. Physiology 11. 585267, Available at: https://www.frontiersin.org/articles/10.3389/fphys.2020.585267 (Accessed July 4, 2022).
Svendsen I. S., Tønnesen E., Tjelta L. I., Ørn S. (2018). Training, performance, and physiological predictors of a successful elite senior career in junior competitive road cyclists. Int. J. Sports Physiol. Perform. 1, 1287–1292. doi:10.1123/ijspp.2017-0824
Valenzuela P. L., Gil-Cabrera J., Talavera E., Alejo L. B., Montalvo-Pérez A., Rincón-Castanedo C., et al. (2021). On- versus off-bike power training in professional cyclists: A randomized controlled trial. Int. J. Sports Physiol. Perform. 16, 674–681. doi:10.1123/ijspp.2020-0305
Valenzuela P. L., Muriel X., van Erp T., Mateo-March M., Gandia-Soriano A., Zabala M., et al. (2022). The record power profile of male professional cyclists: Normative values obtained from a large database. Int. J. Sports Physiol. Perform. 17, 701–710. doi:10.1123/ijspp.2021-0263
van der Zwaard S., Brocherie F., Jaspers R. T. (2021). Under the hood: Skeletal muscle determinants of endurance performance. Front. Sports Act. Living 3, 719434. doi:10.3389/fspor.2021.719434Available at: https://www.frontiersin.org/articles/10.3389/fspor.2021.719434 (Accessed July 4, 2022)
van der Zwaard S., de Ruiter C. J., Jaspers R. T., de Koning J. J. (2019). Anthropometric clusters of competitive cyclists and their sprint and endurance performance. Front. Physiology 10, 1276. doi:10.3389/fphys.2019.01276Available at: https://www.frontiersin.org/articles/10.3389/fphys.2019.01276 (Accessed July 4, 2022)
Keywords: predictors, performance, determinants, laboratory, testing, cycling
Citation: Alejo LB, Montalvo-Pérez A, Valenzuela PL, Revuelta C, Ozcoidi LM, de la Calle V, Mateo-March M, Lucia A, Santalla A and Barranco-Gil D (2022) Comparative analysis of endurance, strength and body composition indicators in professional, under-23 and junior cyclists. Front. Physiol. 13:945552. doi: 10.3389/fphys.2022.945552
Received: 16 May 2022; Accepted: 07 July 2022;
Published: 05 August 2022.
Edited by:
Fábio Juner Lanferdini, Universidade Federal de Santa Maria, BrazilReviewed by:
Stephan van der Zwaard, VU Amsterdam, NetherlandsCopyright © 2022 Alejo, Montalvo-Pérez, Valenzuela, Revuelta, Ozcoidi, de la Calle, Mateo-March, Lucia, Santalla and Barranco-Gil. This is an open-access article distributed under the terms of the Creative Commons Attribution License (CC BY). The use, distribution or reproduction in other forums is permitted, provided the original author(s) and the copyright owner(s) are credited and that the original publication in this journal is cited, in accordance with accepted academic practice. No use, distribution or reproduction is permitted which does not comply with these terms.
*Correspondence: Almudena Montalvo-Pérez, YWxtdWRlbmEubW9udGFsdm9AdW5pdmVyc2lkYWRldXJvcGVhLmVz
Disclaimer: All claims expressed in this article are solely those of the authors and do not necessarily represent those of their affiliated organizations, or those of the publisher, the editors and the reviewers. Any product that may be evaluated in this article or claim that may be made by its manufacturer is not guaranteed or endorsed by the publisher.
Research integrity at Frontiers
Learn more about the work of our research integrity team to safeguard the quality of each article we publish.