- Department of Animal Biosciences, University of Guelph, Guelph, ON, Canada
Estradiol-17β (E2) has long been studied as the primary estrogen involved in sexual maturation of hens. Due to the oviparous nature of avian species, ovarian production of E2 has been indicated as the key steroid responsible for activating the formation of the eggshell and internal egg components in hens. This involves the integration and coordination between ovarian follicular development, liver metabolism and bone physiology to produce the follicle, yolk and albumen, and shell, respectively. However, the ability of E2 to be synthesized by non-gonadal tissues such as the skin, heart, muscle, liver, brain, adipose tissue, pancreas, and adrenal glands demonstrates the capability of this hormone to influence a variety of physiological processes. Thus, in this review, we intend to re-establish the role of E2 within these tissues and identify direct and indirect integration between the control of reproduction, metabolism, and bone physiology. Specifically, the sources of E2 and its activity in these tissues via the estrogen receptors (ERα, ERβ, GPR30) is described. This is followed by an update on the role of E2 during sexual differentiation of the embryo and maturation of the hen. We then also consider the implications of the recent discovery of additional E2 elevations during an extended laying cycle. Next, the specific roles of E2 in yolk formation and skeletal development are outlined. Finally, the consequences of altered E2 production in mature hens and the associated disorders are discussed. While these areas of study have been previously independently considered, this comprehensive review intends to highlight the critical roles played by E2 to alter and coordinate physiological processes in preparation for the laying cycle.
Introduction—Estrogens and Their Receptors
Estrogens play a fundamental role in controlling female reproduction, with the ovary acting as the primary source. Interestingly, in avian species, estrogen biosynthesis has also been reported in non-gonadal tissues, such as the brain and adrenal glands (Tanabe et al., 1979; Matsunaga et al., 2001). As the synthesis of estrogens in mammals has additionally been observed in the skin, heart, muscle, liver, adipose tissue, and pancreas (Hemsell et al., 1974; Cui et al., 2013; Barakat et al., 2016), there is a rationale for further investigation in the avian species. Three primary forms of estrogens can be found in circulation: estrone (E1), estradiol-17β (E2), and estriol (E3). In the hen, it has been established that E1 is a readily reversible estrogen form (MacRae et al., 1959), acting primarily as a precursor and storage form of the most prominent estrogen, E2 (Raud and Hobkirk, 1968). In avian species, E2 is primarily produced by small white follicles (SWFs) following their recruitment during sexual maturation of the ovary (Williams and Sharp, 1977; Robinson and Etches, 1986). In fact, E2 plays an instrumental role in the transition between growth and reproduction in the laying hen. Contrary to mammals, in which E2 is primarily produced by granulosa cells (Hutz, 1989), E2 synthesis in birds is localized to the theca externa layer prior to follicular selection into the hierarchy (Porter et al., 1989). In comparison to the influence of E2, E3 is a weak, minor form of estrogen (Lague et al., 1975), which is 80-fold less potent than E2 and acts as a major urinary metabolite rather than playing an active role in controlling reproduction (Mathur et al., 1966).
Three estrogen receptors (ERs) have been characterized, including two intracellular receptors, ER-alpha (ERα, also known as ESR1) and ER-beta (ERβ, also known as ESR2), and one cell surface ER, referred to as GPR30 (also known as GPER-1). E1 and E3 have shown a preference for ERα and ERβ, respectively, while E2 maintains a similar binding capacity for either intracellular receptor (Kuiper et al., 1998). Conversely, much less is known about the activity of GPR30, which was first reported to mediate the response to E2 in breast cancer cells despite the lack of ERα and ERβ (Filardo et al., 2000; Thomas et al., 2005). Since then, it has been implicated in numerous pathways regulated by E2, including germ cell proliferation (Olde and Leeb-Lundberg, 2009; Ge et al., 2012). In fact, during embryonic development, GPR30 has been implicated in the renewal of chicken primordial germ cells (PGCs) induced by E2 (Ge et al., 2012). More recent investigations in mice models have indicated that during follicle-stimulating hormone (FSH)-stimulated aromatase production, E2 binds to GPR30 to trigger ERK1/2 phosphorylation, resulting in oocyte maturation in vivo (Zhao et al., 2020). However, since the discovery of this G-coupled protein ER in chickens is more recent (Acharya and Veney, 2012; Ge et al., 2012), there is little information on its role both in reproduction and metabolic processes. In the case of the intracellular receptors, differences in expression have been identified between the subtypes. Specifically, ERα was identified within the embryonic brain of Japanese quail and chickens (Camacho-Arroyo et al., 2003; Brunström et al., 2009), as well as the hypothalamus of mature laying hens (Hansen et al., 2003), the ovary of mature hens (Hrabia et al., 2004), shell gland (uterus), kidney (Hansen et al., 2003), liver (Li et al., 2014), and bones (Ohashi et al., 1991; Ohashi and Kusuhara, 1993; Turner et al., 1993). In the case of avian ERβ, expression is less widespread, as it was detected in the embryonic brain (Brunström et al., 2009), ovary (Hrabia et al., 2008), and liver (Li et al., 2014).
While both intracellular receptors are expressed in the avian hypothalamus and pituitary, ERα is the predominant subtype (Griffin et al., 1999), and this receptor is primarily implicated in activating the hypothalamic-pituitary-gonadal (HPG) axis. However, expression of ERβ was identified in the embryonic brain region of quail associated with copulatory behaviour in mature males, suggesting a role for the β-subtype in brain differentiation (Brunström et al., 2009). In the ovary, ERα is the predominant ER (Hrabia et al., 2008). While both intracellular ERs are upregulated during follicular development and the ovulatory process (Drummond and Fuller, 2010), the α-subtype in granulosa cells is predominantly involved in triggering follicular recruitment and the maturation of the remainder of the reproductive tract at the time of activation (Kamiyoshi et al., 1986; Hrabia et al., 2004). ERα is also present within the oviduct of hens to trigger the final maturation of the reproductive tract and coordinate the egg formation processes (Hansen et al., 2003; Imamura et al., 2006). In the case of ERβ, this receptor is predominantly involved with the maturation of pre-ovulatory follicles and the ovulatory process (Emmen et al., 2005).
In the bone and liver, ERα once again plays the predominant role (Imamura et al., 2006; Li et al., 2014). While the expression of both ERs in hepatocytes elevates during sexual maturation (Tan et al., 2020), only ERα has been implicated in mediating the upregulation of yolk proteins (Li et al., 2014). GPR30 has also been detected in the liver (Liu et al., 2018), yet little is known regarding its role. Interestingly, in the presence of growth hormone (GH), a significant elevation in ERβ was reported (Hrabia, 2015), suggesting GH may upregulate ERβ in the liver to participate in nutrient partitioning and utilization during lay. ERα is the only receptor subtype reported to support eggshell formation during bone development in the laying hen. Specifically, since ERα is expressed on the surface of osteoblasts (Ohashi et al., 1991; Ohashi and Kusuhara, 1993; Turner et al., 1993), as the hen ages and transitions toward the end of the production cycle, the receptor density declines, reducing the osteogenic effect of E2 (Hansen et al., 2003). However, the recent determination that modern commercial laying hens can persistently lay up to 100 weeks of age with no detrimental impact on bone or eggshell quality (Hanlon et al., 2022) suggests that genetic selection may have impacted the response to E2. Thus, further studies are required to determine whether this results from a sustained expression of ERs in these strains.
Furthermore, we hypothesize that ER activity likely extends beyond the investigated tissues, as ERs are expressed in adipose tissue in mammals. In avian species, while the follicle-stimulating hormone receptor (FSH-R) has been identified in adipose tissue (Cui et al., 2012), the presence of ERs has yet to be determined. Therefore, it is possible that E2, via its receptors, alters adipocyte functions, diverting nutrients and energy toward egg production through the upregulation of ERs by FSH. Thus, for the remainder of this review, the focus is placed on the roles of E2 as the primary estrogen during sexual differentiation of the embryo, maturation of the hen, and extended laying cycle. Moreover, this review will emphasize the roles of E2 in yolk formation, skeletal development, and metabolism, which all impact egg production and quality (Figure 1). Lastly, the consequences and disorders associated with varying E2 concentrations are discussed to provide a comprehensive review and demonstrate that beyond the reproductive tract, E2 is key to altering metabolic processes during the life cycle of a laying hen.
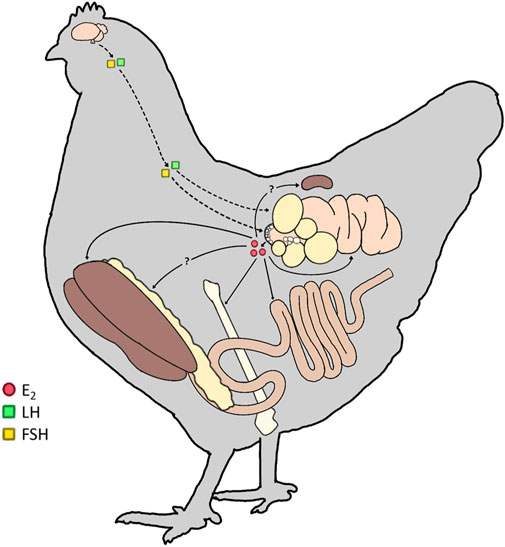
FIGURE 1. Targets of lowercase (E2) in mature hens. In the mature laying hen, the upper portion of the hypothalamic-pituitary-gonadal (HPG) axis is activated, resulting in the production of gonadotropins (Luteinizing Hormone; LH and Follicle-Stimulating Hormone; FSH). FSH and LH then act on the ovary (dashed line) to stimulate follicular development and ovulation, respectively. The small white follicle (SWF) pool synthesizes and releases E2 from the ovary (solid line). E2 targets estrogen receptors (ERs) in the liver, bone, intestine, and oviduct to participate in all levels of the egg formation process. We hypothesize additional ERs are present in the adipose tissue and kidney of hens to further integrate the physiological processes during sexual maturation and the preparation for lay.
Estradiol and Reproduction
Embryonic Sexual Differentiation
Aside from the gonadal sources of E2, studies have reported the presence of maternally derived estrogens in the yolk (Schwabl, 1993; Paitz et al., 2020), potentially implicating estrogen in all stages of embryonic development. A study in quail determined that the E2 concentration present in yolk is comparable to that of the maternal circulating concentrations (Adkins-Regan et al., 1995). In fact, chicken embryos have concentrations as high as 0.2 ng/g of body weight (Elf and Fivizzani, 2002; Hartmann et al., 2003; Wang et al., 2010), and in other avian species, this concentration is as high as 12 ng/g (Merrill et al., 2019). Since ERα expression has been reported as early as embryonic day 3.5 (E3.5; Andrews et al., 1997; Smith et al., 1997), prior to the production of aromatase (Smith et al., 1997) and E2 by the gonads (Woods and Erton, 1978), these maternally derived sources of estrogen may be the primary source acting on the ERs during this period. Additional sources of E2 are produced in the interstitial cells, irrespective of sex, between E3.5–5.5 (Woods and Erton, 1978). However, the role of estrogen in this early developmental period remains to be determined, as it was recently established that E2 is metabolized to estrone early in ovo, then conjugated to sulfates and glucuronides by E5 (Paitz et al., 2020). In female quail treated with estradiol benzoate, female offspring developed right oviducts, resulting in an atypical symmetrical reproductive tract morphology (Adkins-Regan et al., 1995). It has been speculated that the early metabolism of maternally derived E2 observed by Paitz et al. (2020) suppresses the metabolic capacity for Mullerian duct development on the right side (Zimmerman et al., 2012).
Estrogens have long been implicated in the feminization of the reproductive axis during sexual differentiation, with early exposure to estrogen critical for the normal development of the ovary (Elbrecht and Smith, 1992). Thus, female embryos of turkeys, quail, and chickens have higher circulating E2 concentrations compared to their male counterparts (Woods and Brazzill, 1981; Schumacher et al., 1988; Abdelnabi et al., 2001; Ottinger et al., 2001; Sechman et al., 2011; Rosati et al., 2021). Recent evidence demonstrates that JUN is a critical regulator of sexual differentiation, regulating DMRT1, SOX9, and FOXL2, the genes previously hypothesized to be the determinants of differentiation in avian species. In fact, overexpression of JUN results in feminization of ZZ embryos and JUN knockout of ZW embryos results in masculinization (Zhang et al., 2021). Upregulation of JUN results in the inhibition of Smad2 and the simultaneous production of E2 within the gonad (Zhang et al., 2021). These gonadal sources of E2 were detected following the activation of P45017α (CYP17A1) as early as 5–6 days into embryonic development in both the right and left ovaries, with the initiation of cytochrome P450aromatase (CYP19A1) and 3-beta-hydroxysteroid dehydrogenase (3β-HSD) by E6.5 (Yoshida et al., 1996; Andrews et al., 1997; Nakabayashi et al., 1998; Nomura et al., 1999; Nishikimi et al., 2000; Figure 2). In fact, the W chromosome has been linked to the early aromatase activation in the female embryo, leading to ovarian development during the first half of the incubation period (Ottinger, 1989; Kagami and Hanada, 1997). It has even been suggested that the presence of CYP19A1 is not only necessary but sufficient to initiate of female sexual differentiation in the embryo (Jin et al., 2020). This elevation in E2 is key to activating R-spondin-1 (RSPO1) expression during early embryonic development, thereby regulating the WNT/β-catenin signalling pathway responsible for controlling cell fate and stem cell pluripotency (Smith et al., 2008).
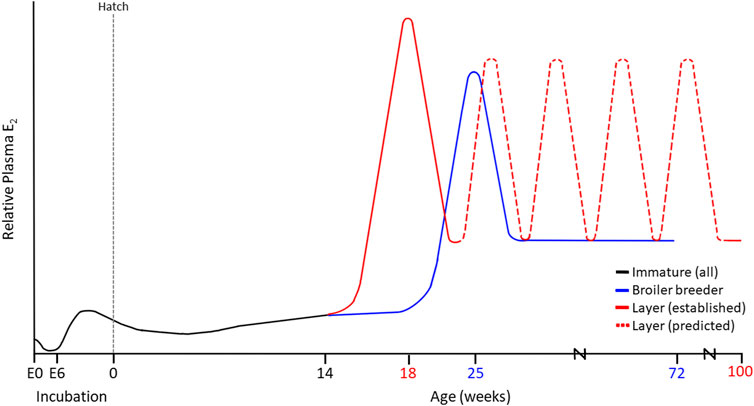
FIGURE 2. Established and Proposed Estradiol (E2) Profiles in Modern Commercial Laying Hens (100 weeks) and Broiler Breeder Hens (72 weeks). Maternal sources of E2 are present in the embryo at day 0 (E0), prior to conjugation during early incubation. At E6.5, the production of aromatase stimulates the ZW left ovarian cortex to produce E2, driving sexual differentiation. These levels decrease around the time of hatch as the chick ovary remains in a quiescent state. Maturation varies among layers and broiler breeders, yet both demonstrate an initial peak in E2. In the case of the layer, we propose that up to 5 additional recurrent E2 elevations occur throughout lay, contributing to the persistency in the cycle that is not observed in broiler breeder hens.
While the elevated synthesis of E2 will suppress anti-Mullerian hormone receptor II (AMHRII) to protect the Mullerian duct from apoptosis (Cutting et al., 2014), this protection only occurs in the left ovarian cortex of avian species. This results in an asymmetrical reproductive tract development, as the expression of ERα in the right ovarian cortex is suppressed under the influence of the pituitary homeobox 2 (PITX2) (Nakabayashi et al., 1998). E2 cannot exert its effects without its receptor present in the cortex, causing the right ovarian cortex to begin regressing as early as E6-6.5 (Ishimaru et al., 2008). Conversely, ERα is expressed in the medulla and germinal epithelium of the left ovary as early as E5.5 (Mizuno et al., 1996; Yoshida et al., 1996; Nakabayashi et al., 1998; Ishimaru et al., 2008). Interestingly, as ERα is temporarily expressed in male embryos between E7-10 (Nakabayashi et al., 1998), embryos can undergo sex reversal following exposure to supraphysiological doses of exogenous E2. This results in the development of ovotestes at hatch, despite the presence of ZZ chromosomes (Scheib, 1983). However, regardless of E2 administration, differentiation into testis was shown to eventually occur during sexual maturation (Etches and Kagami, 1997). Interestingly, supraphysiological doses of E2 are also detrimental to the development of the female reproductive tract, resulting in an intact right ovary and abnormal formation of the left Mullerian duct (Rissman et al., 1984). Therefore, an E2 threshold likely exists during embryogenesis for normal gonadal development. This may include maternal sources accumulated within the yolk, which may in turn impact hatch rate and future reproductive capacity of offspring.
Estradiol Production and Ovarian Follicular Development
In avian species, the reproductive axis is under the control of a dual stimulatory and inhibitory system, mediated through the hypothalamic neuropeptides, gonadotropin-releasing hormone-I (GnRH-I) and gonadotropin-inhibitory hormone (GnIH) (for review: Bédécarrats, 2015; Bédécarrats et al., 2016; Hanlon et al., 2020). Briefly, long-day breeders, such as the domestic chicken, respond to increasing day lengths, also referred to as photostimulation, by downregulating the expression of GnIH and activating the release of GnRH-I. As GnIH directly suppresses GnRH (Bentley et al., 2003, 2008) and gonadotropins (Tsutsui et al., 2000; Ciccone et al., 2004; Ikemoto and Park, 2005; Ubuka et al., 2006), the downregulation of GnIH allows for the full activation of the reproductive axis (Ikemoto and Park, 2005; Maddineni et al., 2008). In turn, the anterior pituitary gland releases gonadotropins, luteinizing hormone (LH) and FSH, which enter the systemic circulation.
Prior to sexual maturation, LH stimulates a gradual increase in ovarian E2 production from thousands of SWFs (Williams and Sharp, 1977; Robinson and Etches, 1986; Sechman et al., 2000; Figure 2). This E2 stimulates the final developmental stage of the oviduct by mediating epithelial cell differentiation into tubular gland cells, goblet cells, and ciliated cells (Kohler et al., 1969), thus allowing for albumen synthesis and deposition during egg formation (Palmiter and Wrenn, 1971). Activation of the reproductive axis is concurrent with follicular development via cyclic recruitment, resulting in a mature, functional ovary. Cyclic recruitment in the avian ovary is characterized by a distinct arrangement of prehierarchal and hierarchical (F1-F6) follicles (Johnson, 1993), in which the largest follicle (F1) is ovulated daily (Johnson et al., 1996). FSH supports the maintenance of prehierarchal follicles via proliferative signals (Johnson, 2003) that reduce the susceptibility of developing granulosa cells to apoptosis (Johnson et al., 1996, 1999), thus preventing follicular atresia. Furthermore, LH and FSH are both capable of initiating receptor-mediated cAMP production through their respective receptors, resulting in steroidogenesis (Porter et al., 1989; Kowalski et al., 1991). However, compared to FSH, LH has a more potent effect on steroid hormone secretion (Sharp et al., 1979; Robinson et al., 1988). LH stimulates progesterone (P4), testosterone (T), and E2 production from theca cells, with higher steroid concentrations produced by less mature follicles. However, the primary product of the theca interna layer is T, which is subsequently converted to E2 by the theca externa cells (Porter et al., 1989). In the developing granulosa cells, FSH induces the necessary steroidogenic competence (Tilly et al., 1991) for P4 synthesis and LH receptor expression in small yellow follicles and intermediate preovulatory follicles (Calvo and Bahr, 1983; Tilly et al., 1991; Johnson and Bridgham, 2001). As preovulatory follicles mature, granulosa cells lose FSH sensitivity and become exclusively LH-responsive. For instance, in the three largest follicles (F1, F2 and F3), LH increases granulosa cell cAMP production, whereas FSH has no effect (Calvo et al., 1981). In the mature preovulatory follicle (F1), LH stimulates the secretion of high concentrations of P4 (Yang et al., 1997). This P4 release exerts positive feedback on GnRH-I, which triggers the preovulatory LH surge (Johnson and van Tienhoven, 1980), inducing ovulation 4–7 h later (Furr et al., 1973). Interestingly, under the influence of the anti-estrogen tamoxifen, egg-laying ceased despite an elevation in E2 production by SWFs. This occurred concomitantly with declining P4 concentrations, leading to the inhibition of ovulation (Rzasa et al., 2009).
While E2 exerts negative and positive feedback on GnRH via kisspeptin neurons in mammals, the absence of kisspeptin in birds may explain the predominantly inhibitory feedback of E2 on gonadotropins. Removing the primary source of E2 through ovariectomy induces a substantial rise in circulating LH concentrations in pullets (Knight et al., 1981) and increases pituitary LHβ subunit mRNA levels in mature hens (Terada et al., 1997). Furthermore, E2 replacement following ovariectomy reduces plasma LH in adult hens (Wilson and Sharp, 1976; Terada et al., 1997) and downregulates pituitary LHβ subunit mRNA levels (Terada et al., 1997). Similarly, E2 injection suppresses circulating levels of LH and FSH in photostimulated birds; however, this response is age-dependent (Dunn et al., 2003). E2 may mediate the acquisition of the neuroendocrine response to photostimulation, as E2 treatment combined with exposure to long daylengths increases plasma gonadotropin concentrations in pullets (Dunn et al., 2003). Likewise, treatment with E2 or a combination of E2 and P4 reduces GnIH-R mRNA abundance in the pituitary of pullets, likely reducing the response to GnIH treatment in adult hens (Maddineni et al., 2008). Interestingly, priming with E2 is also required to develop the LH positive feedback response to P4 necessary for ovulation (Wilson and Sharp, 1976), through E2-induced P4 receptor (PR) expression in the pituitary gland (Gasc and Baulieu, 1988). At the level of the hypothalamus, continuous E2 treatment was shown to upregulate PR in immature females, with receptor levels returning to baseline following E2 withdrawal (Gasc and Baulieu, 1988). This suggests that E2 plays a stimulatory role in priming the HPG axis for maturation. However, daily tamoxifen injections in mature layers also resulted in elevated ovarian E2 production (Mao et al., 2018), which indicates that E2 feedback mechanisms in birds may be more comparable to mammals than hypothesized.
Estradiol and Oviduct Formation
During the development of the chick, implants containing estrogen diethylstilbestrol (DES) triggered cellular proliferation and prevented apoptosis in the developing oviduct (Monroe et al., 2000, 2002). Doses of up 12,000 to 25,000 IU of estradiol benzoate administered to chicks resulted in a 10-fold increase in diameter (Wolff, 1936), and doses of α-estradiol dipropionate at 4 mg per 100-g of body weight led to an 80-fold increase in oviduct weight (Munro and Kosin, 1943). Although the magnum remains an undifferentiated epithelial tube during the immature state, daily injections of estradiol benzoate beginning at 4 days of age can result in an 8-fold increase within 3-days (Palmiter and Wrenn, 1971), indicating that the chick oviduct is already prepared to respond to E2 stimulation in the days following hatch. This exogenous E2 during chick growth can also trigger an elevated expression of ovalbumin (Kohler et al., 1969; Palmiter and Wrenn, 1971). These levels will fall quickly in the absence of E2 stimulation (Schimke et al., 1975). As the hen enters maturity, E2 mediates the differentiation of tubular gland cells in the magnum, which can be inhibited by the simultaneous administration of P4 (Oka and Schimke, 1969) through the reduction of cytoplasmic, nuclear, and total ERα (Hsueh et al., 1976; Kraus and Katzenellenbogen, 1993).
The shell gland segment of the oviduct contains a large amount of calbindin 28 K (Corradino et al., 1968) and receptors for cholecalciferol (1,25(OH)2D3) (Coty, 1980; Haussler, 1986). The binding of cholecalciferol to its receptor triggers an upregulation of the calbindin gene (Theofan et al., 1986; Clemens et al., 1988; Mayel-Afshar et al., 1988). In turn, calbindin protein is responsible for binding calcium and, in the case of the shell gland, depositing this calcium as eggshell in the form of calcium carbonate (Bradfield, 1951; Corradino et al., 1968). Calbindin concentrations are also stimulated by E2 (Navickis et al., 1979; Nys et al., 1986, 1989), as calbindin is colocalized with ER within the glandular epithelial cells (Jande et al., 1981; Isola, 1990). Thus, levels of this protein increase at the time of sexual maturation (Nys et al., 1989). However, during the formation of the first egg, the expression of calbindin is highly dependent on the transfer of calcium to the shell gland rather than E2 concentrations (Nys et al., 1989; Striem and Bar, 1991).
Oviductal prolapse is the cause of up to 20% of mortality in laying hens (Shemesh et al., 1984). Evidence has shown increased incidence with declining E2 levels in the blood (Shemesh et al., 1982). Interestingly, not only has E2 treatment been shown to support recovery, but spontaneous recovery has also been associated with sudden elevations in E2 (Shemesh et al., 1984). Recovered birds had higher E2 in the theca layer of the follicles compared to healthy or prolapsed counterparts (Shemesh et al., 1984). Thus, it has been proposed that prolapse results from systemic E2 deficiency.
Estradiol and Metabolism - Yolk Formation
Activation of the Liver
The liver undergoes two metabolic states in hens: pullet growth and active laying (Gruber et al., 1976). Prior to the elevation of E2 during sexual maturation, the liver primarily contributes to pullet growth. In fact, the liver is responsible for ∼90% of fatty acid synthesis (Leveille et al., 1968; O’Hea and Leveille, 1968; Wang et al., 2014), producing a generic VLDL for the transport of dietary lipids in the blood (Chapman et al., 1977; Walzem, 1996; Walzem et al., 1999). These VLDLs are considerably large (∼70 nm diameter) due to their association with 6 identified apolipoproteins, including apoA-1, apoB, and apoC (Walzem, 1996). Thus, these lipids will only be stored in adipose or utilized by peripheral tissues (Walzem, 1996), as they are rapidly processed and energy inefficient, with only ∼40% of the substrate used (Griffin et al., 1982). At this time, lipid transport is quite similar to that in mammalian species, with the exception of dietary fat being transported as portomicrons through the portal vein in immature birds (Bensadoun and Rothfeld, 1972) rather than through the lymphatic system in mammals. Upon the initiation of egg-laying, traditional VLDLs are too large to undergo deposition into the growing follicle (Walzem et al., 1999), while intermediate-density lipoproteins (IDLs) and low-density lipoproteins (LDLs) are smaller particles but provide insufficient energy (Griffin et al., 1982). Thus, a modified lipoprotein is required to meet the demands of yolk deposition.
At the time of sexual maturation, ERα present in the liver binds E2 to elicit the expression of estrogen-dependent genes driving yolk production and enhancing the stability of genes associated with egg white proteins (Bergink and Wallace, 1974; Kirchgessner et al., 1987; Flouriot et al., 1996). Therefore, under the influence of E2, the liver produces VTG-II (Deeley et al., 1977; Evans et al., 1987), a glycophospholipoprotein that provides the oocyte with glucose, phosphorous, and fat while binding metal ions such as calcium, zinc, and iron (Montorzi et al., 1995). E2 also stimulates the liver to produce ApoVLDL-II (Chapman et al., 1977; Deeley et al., 1977; Wiskocil et al., 1980; Codina-Salada et al., 1983; Evans et al., 1987; Ratnasabapathy, 1995; Flouriot et al., 1996; Ratnasabapathy et al., 1997; Walzem et al., 1999), an apolipoprotein that inhibits lipoprotein lipase (LPL) to prevent susceptibility to the breakdown of the triglycerides (TAGs) during transport to the oocyte (Griffin et al., 1982; Schneider et al., 1990). Laying hens also have lower LPL activity in the adipose and heart and adipose tissue than their immature counterparts (Husbands, 1972), supporting the redirection of VLDLs to the ovary (Perry and Gilbert, 1979). This Apo-VLDL-II is packaged in a 23:1 ratio with ApoB, combining to form a yolk-targeted TAG-rich VLDL (VLDLy) (Walzem et al., 1999). Due to its association with only two apolipoproteins, the size of VLDLy is reduced by ∼50% (30 nm) without sacrificing energy, TAG, or phospholipid content (Griffin et al., 1982; Griffin and Perry, 1985). This allows for easier transport to the ovary, where they can be incorporated into the oocyte via receptor-mediated endocytosis and pass through the granulosa layer (Perry and Gilbert, 1979; Krumins and Roth, 1981; Griffin et al., 1982). Therefore, VLDLy is an ideal candidate for yolk deposition due to the high requirement of energy to be packaged into the follicle for embryo development (Kudzma et al., 1979; Griffin, 1981; Dashti et al., 1983; Lin and McCormick, 1986; Chan et al., 1990; Schneider et al., 1990; Speake et al., 1998; Walzem et al., 1999). These VLDLy particles make up ∼30% of the total weight of each yolk, with hens producing up to 5 g of lipids for the daily yolk accumulation (Griffin et al., 1982). Therefore, to keep up with the demand, VLDLy synthesis is significantly upregulated by E2 compared to the previous production of generic VLDL (Walzem et al., 1999). Furthermore, E2 has been implicated in the upregulation of lipolytic activities and facilitation of yolk transport during the rapid growth phase of the preovulatory follicles (Brady et al., 1976; Deeley et al., 1977; Wiskocil et al., 1980; Cochrane and Deeley, 1988; Speake et al., 1998; Walzem et al., 1999; Nikolay et al., 2013). Finally, E2 also stimulates the production of albumen, which is transported to the oviduct during egg formation (Deeley et al., 1975; Cochrane and Deeley, 1988; Evans et al., 1988; Li et al., 2014; Ratna et al., 2016), and is thus critical to the successful formation all internal egg components (Figure 3).
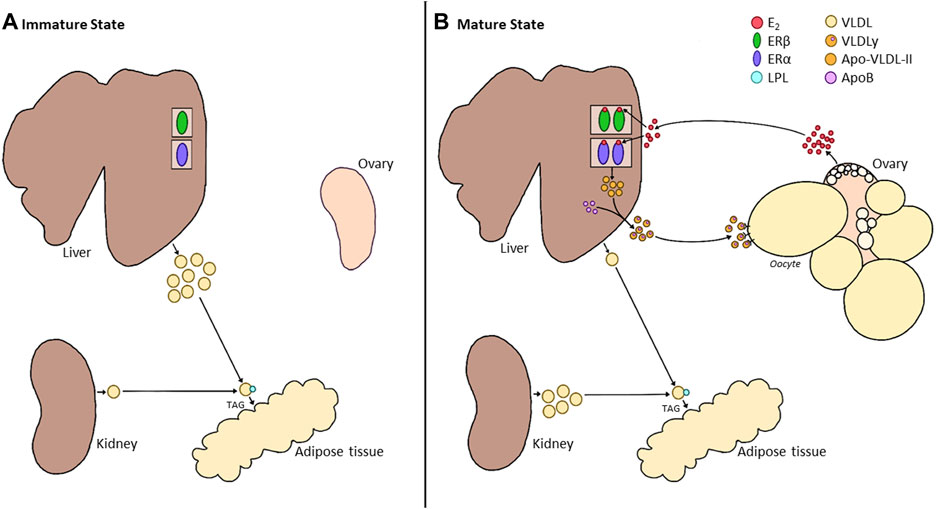
FIGURE 3. Alterations in Liver Metabolism between the Immature and Mature States of Hens. (A). During the immature state (pullet), the ovary of the bird remains in a quiescent state. While the liver already expresses estrogen receptors (ERα and ERβ), the absence of E2 results in the production of a generic VLDL. This VLDL is broken down into triglycerides (TAGs) by lipoprotein lipase (LPL) in the circulation and deposited into tissues such as the adipose. During this time, the kidney produces a small amount of generic VLDL and minor production of E2 by the immature ovary. (B). Once the ovary begins to produce E2, this hormone binds to its receptors in the liver. While little is known about the role of ERβ in the liver, binding to ERα results in the production of a smaller, more energy-efficient VLDLy, made up of ApoVLDL-II and ApoB in a 23:1 ratio. VLDLy is resistant to LPL, aiding in the deposition of this yolk protein in the rapidly growing follicle. While a small portion of generic VLDL can be produced within the liver, the primary source for peripheral tissues will now be provided by the kidney. However, the role of E2 in the switch of kidney function is still undetermined.
Studies have also investigated the impact of exogenous E2 sources prior to the activation of the HPG axis, and a similar response was observed (Balnave, 1971; Muramatsu et al., 1992; Elnager and Abd-Elhady, 2009), suggesting that ERs are expressed and responsive during pullet growth in preparation for maturation. While it has been established that the populations of both nuclear ER subtypes increase during sexual maturation (Tan et al., 2020), the precise age at which ERs are expressed has not been established. Nonetheless, VLDLy production, along with the associated yolk precursors, apo-VLDL-II and VTG-II, were found to elevate prior to reproductive maturity in response to exogenous E2 (Muramatsu et al., 1992). This was further associated with increased liver weight and plasma lipid concentrations (Balnave, 1971; Elnager and Abd-Elhady, 2009). In fact, the TAG serum content elevations are associated with a greater number of lipid droplets in the liver of E2-treated hens than the control (Ren et al., 2021), demonstrating the direct impact of E2 on liver physiology. Interestingly, while E2 is required to activate yolk precursors and E2 concentrations decline in aging hens, a recent study showed that ApoVLDL-II and VTG-II mRNA elevate with age (Cui et al., 2020). A similar result was observed in Li et al. (2020), in which Apo-VLDL-II and ApoB increased over time. This is in line with the hypothesis that the production of yolk precursors may be linked to the recurrent elevations in E2 observed in commercial strains (Hanlon et al., 2021), suggesting that E2 may play a larger role in maintaining the egg-laying cycle than previously hypothesized.
Liver Response
More recently, additional estrogen-responsive genes expressed in the liver have been under investigation (Zhang et al., 2020; Ren et al., 2021). Upon exogenous E2 administration in mature laying hens, a 23-fold elevation in peroxisome-proliferative receptor γ (PPARγ) expression was observed (Lee et al., 2010). This corresponded to increased fatty acids, TAGs, and hepatic lipids (Sato et al., 2009; Lee et al., 2010). Higher hepatic expression of PPARγ has previously been associated with a higher production rate (Chen et al., 2010) and a decline occurs with age (Cui et al., 2020). However, since the expression patterns of ERα increase in aging hens while ERβ does not change (Cui et al., 2020), circulating levels of estrogens are likely the most critical factor.
Apela, which is speculated to be involved in the hepatic lipid metabolism of hens, is activated by E2 in the liver during embryogenesis via ERα. This gene can be induced with exogenous E2, as demonstrated by the elevated expression at the peak of lay (Tan et al., 2020). Estrogen is also reported to bind to ERα to downregulate gga-miR-221-5p, increasing the expression of ELOVL6 and SQLE, responsible for stimulating lipid synthesis, consistent with the role outlined above (Zhang et al., 2020). E2 binding to ERα also directly targets miR-144 (Vivacqua et al., 2015), which is known to regulate lipid metabolism through the suppression of PPARγ-coactivator-1β (PGC-1β; Ren et al., 2021). Furthermore, PGC-1α is also influenced by ERα and ERβ (Jiang et al., 2021). However, the action of E2 on PGC-1α remains unclear. Further investigation may reveal a mechanism through which E2 can directly control lipogenic genes in the liver to influence adipose accumulation and deposition. The involvement of ERα is particularly unique and important to note, as ERβ has been the primary receptor subtype suggested to play a role in the metabolic control of E2 (Li et al., 2015, 2018). Instead, ERα may be an underappreciated potential target for treating metabolic disorders. However, that does not imply that ERβ is not involved. In fact, cathepsin E-A like gene, suggested to play a role in the cleavage and processing of VTGs and ApoB (Wang et al., 2022), acts through the β-receptor (Zhang et al., 2018), demonstrating that both receptors will play a critical role in the yolk formation process.
Activation of the Kidney
Comparable to the liver, the activity of the kidney also depends on the maturity status of the hen. Initially, it was believed that E2 did not play any role in the synthesis of ApoB in the kidney or intestine (Kirchgessner et al., 1987; Lazier et al., 1994), while it was shown to directly control the production of ApoVLDL-II in the embryonic kidney (Lazier et al., 1994). However, the hen’s kidney produces generic VLDL and HDL (Bensadoun and Rothfeld, 1972; Tarugi et al., 1998), with the generic VLDL secreted by cells of the proximal tubules. Thus, generic VLDL from the kidney during the laying cycle was proposed to provide the necessary energy to tissues evaded by VLDLy (Walzem et al., 1999). While a direct or an indirect role for E2 in the production of generic VLDL is inconclusive, low-density lipoprotein receptor-related protein-2 (LRP2) is primed by E2 in the kidney during the maturation period (Plieschnig et al., 2012). This endocytic receptor is proposed to be critical in improving the uptake of apolipoproteins to generate and supply generic VLDL to extraoocytic tissues. This is further supported by the elevation of this receptor in the mature hen compared to a mature rooster (Plieschnig et al., 2012). Additionally, ApoB content is higher in the kidney and intestine of laying hens compared to broilers, while no differences are present between breeds in the liver (Zhang et al., 2007). Therefore, it is highly likely that E2 is involved in the activity of the kidney following the maturation phase.
Fatty Liver Hemorrhagic Syndrome
Fatty liver hemorrhagic syndrome (FLHS) is a metabolic condition typically associated with high-producing laying hens (Trott et al., 2014). This disorder was first described by Couch (1956), with the terminology coined by Wolford and Polin (1972) to distinguish FLHS from non-hemorrhagic fatty liver syndrome (FLS). A high rate of lay has long been attributed to FLS or hepatic steatosis in birds, characterized by the rapid accumulation of lipoproteins in the liver. During this time, the rate of hepatic lipogenesis exceeds that of fat mobilization. FLS is typically observed in modern commercial laying hens since the production cycle requires an exceedingly high rate of lipogenesis, thus overwhelming hepatocytes (Polin and Wolford, 1973; Harms et al., 1982; Hansen and Walzem, 1993; Hermier, 1997; Scheele, 1997). The progression of this disorder eventually leads to a decline in egg production rate (Walzem et al., 1993; Shini and Bryden, 2009; Lee et al., 2010; Jiang et al., 2013; Trott et al., 2014).
While pullets and low-producing hens also have some degree of fat infiltration in the liver (Shini et al., 2020), there are fewer and smaller fenestrae in the sinusoidal endothelium of the liver compared to the mammalian species (Fraser et al., 1986; Braet and Wisse, 2002). It has been hypothesized that the size of fenestrated cells helps avoid dietary fat from overwhelming hepatocytes and prevents atherosclerosis (Fraser et al., 1986). Interestingly, E2 has also been shown to inhibit coronary atherosclerosis (Hanke et al., 1996; Beaufrère, 2013; Petzinger and Bauer, 2013). Since large particles are blocked from passage, they remain trapped in sinusoids around the central vein, resulting in FLS. This can eventually progress to FLHS with the continued accumulation of fat in the liver, overwhelming the hepatic system (Shini and Bryden, 2009).
Typically, severe cases of FLHS results in the sudden death of older laying hens during their post-peak production (Butler, 1976; Shini et al., 2012), with up to 42% of mortality observed in commercial flocks and up to 74% of caged hen mortality, making this the number one cause of non-infectious laying hen mortality (Shini et al., 2006, 2019; Shini and Bryden, 2009; Mete et al., 2013; Trott et al., 2014). While this metabolic disorder was previously believed to be a result of heat stress (Ringer and Sheppard, 1963; Wolford, 1971; Schexnailder and Griffith, 1973; Pearson and Butler, 1978a; Rozenboim et al., 2016), caged environments (Squires and Leeson, 1988), and high lipid diets (Couch, 1956; Jaussi et al., 1962; Bragg et al., 1973; Haghighi-Rad and Polin, 1982), further evidence has suggested the poorly defined pathogenesis of this disorder is much more complex. Excessive consumption of high energy, low protein (HELP) diets has been clearly established to induce FLHS (Hermier et al., 1994; Lee et al., 2010). While these diets are incredibly effective as a model for this condition, it is well-recognized that the dietary onset of FLHS is also correlated with elevations in E2 concentrations (Butler, 1976; Polin and Wolford, 1977; Pearson and Butler, 1978b). Even increasing the metabolizable energy in the diet of broiler breeder hens was shown to correspond to higher E2 concentrations (Hadinia et al., 2020). This resulted in earlier lay, higher GnRH mRNA levels and, as anticipated, higher relative lipid content in the carcass (Hadinia et al., 2020). Therefore, the administration of exogenous E2 is also highly effective at inducing FLHS independently (Butler, 1976; Polin and Wolford, 1977; Pearson and Butler, 1978b).
Hormonal control of FLHS was first proposed by Butler (1976) since this metabolic disorder was solely accredited to hens presently in lay. Thus, the role of E2 was investigated, and studies confirmed that exogenous administration stimulates lipogenesis in immature birds, resulting in hepatic steatosis (Lorenz, 1954; Balnave, 1968, 1971; Yu and Marquardt, 1973; Balnave and Pearce, 1974; Harms et al., 1977; Tarlow et al., 1977; Pearson and Butler, 1978b; Kudzma et al., 1979; Dashti et al., 1983; Klimis-Tavantzis et al., 1983). However, this condition was more consistently induced during exogenous E2 administration in mature layers (Harms et al., 1977; Polin and Wolford, 1977; Pearson and Butler, 1978b; Stake et al., 1981).
Hepatic steatosis has been shown to have detrimental effects on E2 metabolism in both humans and birds (Adlercreutz, 1970; Haghighi-Rad and Polin, 1981). Despite elevated E2 levels during sudden FLHS outbreaks, P4 remains unchanged, and production levels decline as liver fat and FLHS scores increase (Haghighi-Rad and Polin, 1981). This is followed by the rapid regression of the ovary and oviduct (Stake et al., 1981). Reduced LPL activity associated with sexual maturation and the switch in liver metabolism at this time results in reduced clearance of fat in the adipose tissue and increased deposition in the liver (Hasegawa et al., 1980). Thus, the liver fat content of laying hens, typically ∼40% of dry weight, can reach up to 70% (Squires and Leeson, 1988). Additionally, E2 will trigger hypercholesterolemia and hypertriglyceridemia (Shini et al., 2020) as the clearance rate of E2 falls short of its production by the ovary (Hawkins et al., 1969; Johnson and van Tienhoven, 1981; Tsang and Grunder, 1984), resulting in further impaired liver function (Shini et al., 2020). This suggests E2 concentrations may become detrimental above a threshold level. Interestingly, white strains are more tolerant to higher and persistent E2 concentrations (Stake et al., 1981).
While it has been proposed that a combination of E2 and a positive energy balance via high-energy diets is required to induce FLHS (Harms et al., 1977; Polin and Wolford, 1977; Pearson and Butler, 1978b; Stake et al., 1981; Haghighi-Rad and Polin, 1982), Walzem et al. (1994) hypothesized that providing excessive energy to these hens results in decreased E2. This reduction would effectively shut down ApoVLDL-II synthesis and secretion, returning the VLDL particles to their larger, generic size (Walzem et al., 1994). However, further investigation demonstrated that E2-treated hens with low feed intake have the greatest incidence of FLHS (Stake et al., 1981), concluding that this disorder is primarily under the control of E2, and the remainder of the associated factors may be co-morbidities. Additionally, the administration of tamoxifen, an E2 inhibitor, reduced the severity of hepatic hemorrhages (Stake et al., 1981). With the updated E2 profiles of recurrent elevations linked to persistency, we hypothesize this will impact liver lipogenesis, and it is critical to determine if these elevated concentrations result in the induction of FLHS.
Estrogens and Bone Formation
Hormonal Regulation of Calcium Homeostasis
There are two phases of calcium homeostasis regulation in avian species. The first corresponds to structural bone growth occurring throughout the development of the skeletal frame. The second corresponds to the accumulation of medullary bone, which serves as an available pool of calcium for successful egg production. Thus, at the time of sexual maturation, calcium homeostasis shifts from bone growth to calcium storage (Dacke et al., 1993). Regardless of the phase, plasma calcium levels are controlled by the release of parathyroid hormone (PTH), calcitonin (CT), and 1,25-dihydroxycholicaciferol (1,25(OH)2D3), acting similarly to most mammalian species. However, in the case of chickens, once the hen enters sexual maturity, E2 has a major impact on calcium metabolism (Etches, 1987; Newman and Leeson, 1997; Deluca, 2004; Bar, 2008, 2009).
In growing pullets, the majority of calcium is stored as hydroxyapatite (Ca10(PO4)6(OH)2), requiring a tight regulation between calcium and phosphorus and adequate dietary inclusion of both minerals for successful bone development (Hurwitz and Bar, 1965; Whitehead and Fleming, 2000). While phosphorus is required to form several egg components, including yolk and albumen (Keshavarz, 1998), requirements remain constant during the laying cycle. Since calcium destined for the eggshell is deposited as calcium carbonate (CaCO3), phosphorous is not necessary during this process. Thus, the breakdown of medullary bone results in phosphorous being primarily excreted (Kebreab et al., 2009).
Under natural light, 7-dehydrocholesterol is a precursor synthesized in the liver and transported to the skin to interact with UV light and synthesize cholecalciferol, also known as Vitamin D3 (Klassing, 1998b). This form of Vitamin D is the most efficiently metabolized in chickens (Valinietse and Bauman, 1981), thus meeting the demands of the birds without requiring supplementation. However, artificial lighting programs in commercial settings lack UV light, resulting in the inability of birds to synthesize adequate Vitamin D levels (Sharp, 1996). Thus, dietary supplementation with exogenous sources is required, forming 25(OH)D3 in the liver (Lund and Deluca, 1966). This form undergoes further hydroxylation in the kidney by the rate-limiting enzyme, 1α-hydroxylase, to produce 1,25(OH)2D3 (Lund and Deluca, 1966; Gray et al., 1972; DeLuca, 1974, 1976; Haussler, 1974; Knutson and DeLuca, 1974; Rosol et al., 2000). Therefore, 1α-hydroxylase is upregulated during periods of hypocalcemia (Deluca, 1980).
Hypocalcemia also triggers the synthesis and release of PTH, a hormone produced by the chief cells of the parathyroid glands in response to signals by calcium-sensing receptors (Brown, 1991; Yarden et al., 2000) to regulate calcium within the bone, kidney, and small intestine (Laverty and Clark, 1989; Nys, 1993; Dacke et al., 2015). Specifically, PTH binds to its receptor on the cell surface of osteoblasts to alter their morphology and inactivate osteogenesis (Hurwitz, 1989). Additionally, PTH stimulation promotes calcium reabsorption in the kidney (Laverty and Clark, 1989). Simultaneously, PTH promotes the resorption of bone by stimulating osteoclasts to develop a ruffled border secreting enzymes and acid (Miller et al., 1984; Sugiyama and Kusuhara, 1994). If the hen is instead in a period of hypercalcemia, calcitonin is produced and secreted by the ultimobranchial glands (reviewed by: Dacke, 1979), acting in opposition of PTH. Calcitonin supports the development of bone by promoting osteoblasts to return to their original state, while inhibiting osteoclastic activity (Sugiyama and Kusuhara, 1993, 1996). As hens are under intensive calcium demands during a laying cycle, this hormone will primarily be responsible for downregulating the activity of PTH to support normokalemia. Calcium homeostasis is maintained throughout the life of the bird, from early embryogenesis to the end of a laying cycle. However, the production of E2 during sexual maturation promotes adaptive changes to calcium homeostasis to further support skeletal maintenance while stimulating eggshell formation.
Development of the Skeletal Frame
Since the development of the skeletal frame begins during embryogenesis, the fully formed yolk also contains all the required Vitamin D3 and phosphorous in ovo, along with 1% of the total calcium required (Klassing, 1998a; 1998b). This is sufficient for the initial formation of hyaline cartilage, which occurs during the first 7–10 days of incubation (Richards and Packard, 1996). Once this source of calcium has been depleted, the chorioallantoic membrane (CAM) will mobilize calcium carbonate from the shell membrane. Meanwhile, carbonic anhydrase dissolves calcium from the shell, which diffuses through the shell membrane, binding to calcium-binding proteins on the surface of the ectoderm by E13 (Tuan and Scott, 1977). Thus, the role of E2 in breeder hens during eggshell formation directly impacts the success of skeletal development during embryogenesis, preventing metabolic disorders that occur later in the laying cycle of the offspring.
Once hatched, bones continue to grow in width and length via intermembranous and endochondral ossification, respectively (Pechak et al., 1986a, 1986b; Whitehead, 2004). During this period of growth, the structural bone develops as two types of lamellar bone: cortical and trabecular (Whitehead and Fleming, 2000). Intramembranous ossification contributes to bone thickness, with mesenchymal stem cells differentiating into bone until the period of sexual maturation (Pechak et al., 1986a, 1986b; Whitehead, 2004). Longitudinal growth occurs at the epiphyseal growth plates, with bone formation initiated in the lower hypertrophic zone. Chondroclasts reabsorb the cartilage matrix in this region to create space and support mineralization via the formation of hydroxyapatite crystals and infiltration of bone-building cells, referred to as osteoblasts (Whitehead, 2004). Bone development and remodelling progresses through the coordination of calcium deposition by osteoblasts and calcium breakdown triggered by osteoclasts (Roodman, 1999; Suda et al., 2001; Dacke et al., 2015). Prior to sexual maturation, the skeletal frame should have reached its maximum length and optimal thickness. This emphasizes the importance of managing the pullet prior to the increase in sex steroids to prevent the development of metabolic disorders later in the cycle, such as osteoporosis and cage layer fatigue, resulting in fragile skeletal structures (Whitehead and Fleming, 2000).
Medullary Bone and the Laying Cycle
As mentioned above, the increase in circulating E2 associated with maturation leads to alterations in the control of calcium, Vitamin D, and PTH levels. The direct impact of E2 extends to the upregulation of 1,25(OH)2D3 receptors within the gut mucosa to improve the absorption of dietary sources of calcium. E2 has also been reported to increase the responsiveness of the kidney to PTH and promote the production of medullary bone (Pfeiffer and Gardner, 1938).
Particularly, upon activation of the reproductive axis, the hen terminates all further calcium deposition for the sole purpose of structural development. At this time, hens entering lay transition to the formation of the medullary bone (Bloom et al., 1941; Miller, 1992; Turner et al., 1994). This is a readily mobilizable source of calcium stored on the inner endosteal surface of the marrow cavities of long bones to provide calcium for future eggshell formation (Bloom et al., 1958; Mccoy and Reilly, 1996). This occurs primarily in the long bones, such as the femur and tibia but is also present in the pubic bone, ribs, ulna, toes, and scapula (Jacob and Pescatore, 2013). Studies have suggested medullary bone forms at the expense of structural bone since it has not been linked to direct improvements in strength (Whitehead and Wilson, 1992). However, additional findings have indicated a decline in fracture rate related to increased total content in the cavity (Fleming et al., 1998; Whitehead and Fleming, 2000), implying that medullary bone may play a beneficial role in the bone health of hens.
Medullary bone has been established to develop as early as 12–14 days preceding the first egg (Bloom et al., 1941; Miller, 1992; Turner et al., 1994), which is concomitant with the rise in E2 produced by the ovary during early follicular development (Benoit and Clavert, 1945; Common et al., 1948; Simkiss, 1961; Senior, 1974; Tanabe et al., 1981; Johnson et al., 1986; Miller, 1992). However, a recent study has demonstrated that the rise in E2 occurs at the time of age of first egg (AFE) in current commercial laying hens (Hanlon et al., 2021). Interestingly, this is further altered in broiler breeder hens, with the E2 peak occurring 2 weeks after the AFE (Takeshima, 2018; Takeshima et al., 2019). Thus, rather than require the peak concentration of E2, we hypothesize that there may be a threshold E2 concentration that must be achieved to trigger the initiation of medullary bone formation.
As ERα mRNA is expressed in osteoblasts, binding to E2 alters the morphology of these spindle-shaped cells and terminates the formation of structural bone while stimulating the initiation of medullary bone (Dacke et al., 1993, 2015). Simultaneously, osteoclasts are deactivated as they take on a cuboidal shape (Dacke et al., 2015). While PTH and 1,25(OH)2D3 levels typically regulate osteoclasts, E2 has been shown to indirectly influence the formation of this cell type (Oursler et al., 1991; Dacke et al., 1993). In mice models, E2 regulates the formation of osteoclasts by inhibiting a protein kinase pathway, resulting in indirect inhibition of the PTH-stimulated formation (Kanatani et al., 1998). However, as E2 also prevents apoptosis of osteocytes, this hormone supports the balance between the formation and resorption of bone during maturation (Tomkinson et al., 1998). Male quail treated with anti-estrogen displayed an elevation in the osteoclast population (Ohashi et al., 1987), suggesting that an inverse relationship between E2 and osteoclast activity is conserved in poultry. Unlike osteoblasts, early studies determining the presence of ERs on the surface of osteoclasts led to conflicting reports in the literature (Ohashi et al., 1991; Oursler et al., 1991). However, it is now largely accepted that osteoclasts will not express either ER subtype (Ohashi et al., 1991). Presently, most evidence suggests that E2 will play an indirect inhibitory role in bone resorption and a direct stimulatory role in bone formation (Oursler et al., 1991, 1993; Ali, 1992; Price and Russell, 1992; Figure 4).
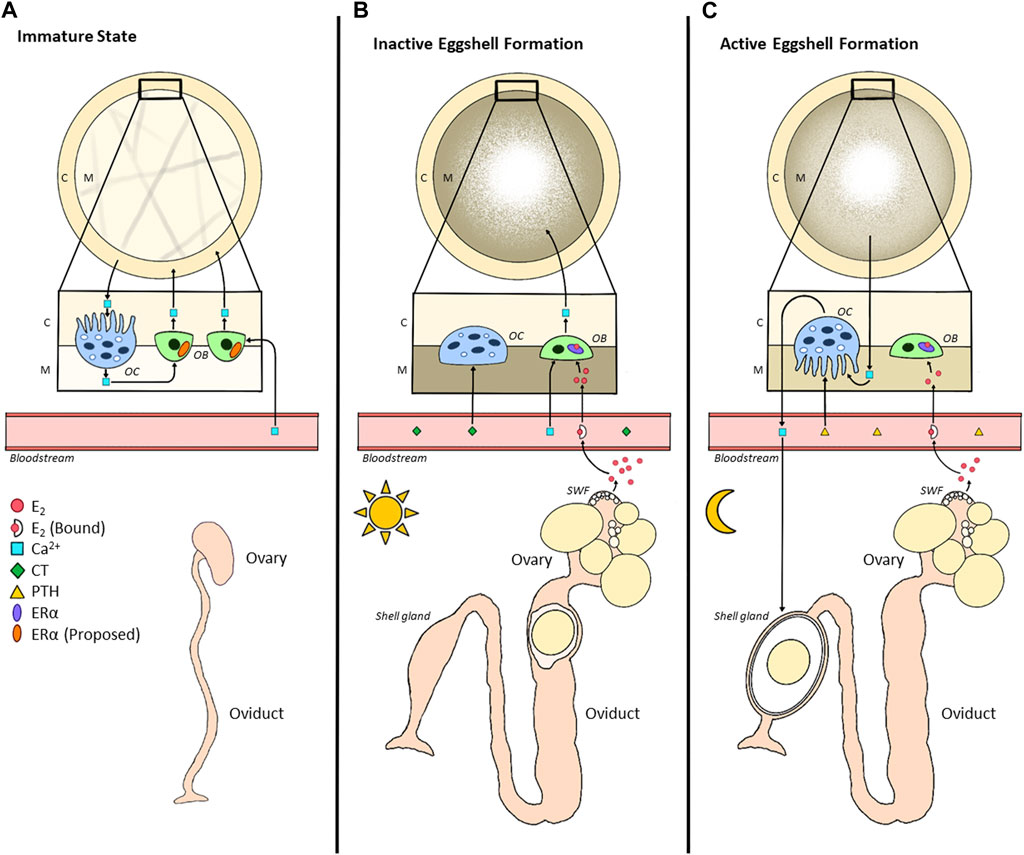
FIGURE 4. Alterations in Bone Metabolism between Immature and Mature States of Hens. (A). During the immature state (pullet), calcium (Ca2+) is deposited by osteoblasts as cortical bone (C), serving as a structural source. Continuous bone remodelling occurs due to the resorptive capacity of osteoclasts. While the immature ovary produces very little E2 during this time, we propose that osteoblasts already express ERα in the immature state. Once the hen reaches maturation (B,C), in each 24-h egg formation cycle, there will be inactive and active eggshell formation. (B). Inactive eggshell formation occurs when the egg is present in the early portion of the oviduct (infundibulum, magnum, isthmus), usually during photophase. During this time, E2 enters the circulation where it binds to its carrier protein; a portion of free E2 then enters the cavity of the bone to bind to ERα expressed in osteoblasts. This triggers a morphological alteration in the shape of the osteoblast, interrupting the formation of cortical bone, instead opting for the formation of medullary bone (M), a readily mobilizable storage site of calcium for eggshell development. Calcium homeostasis is regulated by calcitonin (CT) at this time, downregulating the activity of osteoblasts via morphological alterations. (C). Active eggshell formation begins once the egg enters the shell gland (uterus), typically during scotophase. Therefore, due to the unavailability of dietary calcium, medullary bone undergoes resorption to provide the shell gland with calcium. The morphological alterations in osteoclasts are triggered by the upregulation of parathyroid hormone (PTH). Simultaneously, although E2 binds to its receptor, the absence of additional calcium prevents the active formation of medullary bone at this time.
In commercial laying hens, 2.2-g of calcium, or 10% of total calcium stores, is required for each eggshell (Nys et al., 1999; Bouvarel et al., 2011; Bain et al., 2016). While approximately 50% of this requirement can be covered by dietary calcium absorbed within the intestine daily (Kerschnitzki et al., 2014), about 33% is directly provided by the medullary bone (Bouvarel et al., 2011). Interestingly, this bone source stores ∼12% of the total bone calcium content within the hen’s body (Nys and le Roy, 2018). Since laying consists of active and inactive eggshell formation periods (Miller, 1977), medullary bone provides a source of calcium for the active shell formation, which primarily occurs during the scotophase (Clunies and Leeson, 1995). Specifically, the ovum reaches the shell gland ∼5 h post-ovulation, with eggshell formation occurring between 7 and 20 h post-ovulation (van de Velde et al., 1984).
Osteocalcin (OC) is a major non-collagenous protein produced by osteoblasts (Ugar and Karaca, 1999) and used to evaluate bone remodelling (Christenson, 1997; Ugar and Karaca, 1999; Seibel, 2005). Studies have suggested that calcium in the bone matrix is bound by OC (Christenson, 1997). However, comparisons of brown and white laying strains unexpectedly showed that brown strains have higher OC levels while white strains have higher E2 levels (Habig et al., 2021). This suggests that brown strains would have improved bone quality, despite the E2 differences observed. This hypothesis was confirmed by several studies (Eusemann et al., 2018a; Jansen et al., 2020; Habig et al., 2021). Thus, elevated OC in brown strains (Habig et al., 2021) may explain the lower prevalence of keel bone deformities (Eusemann et al., 2018a). This has led to speculated strain differences regarding the proportion of calcium mobilized from each source to form the eggshell. Habig et al. (2021) suggested that brown laying strains mobilize a more significant proportion of calcium from the bone compared to white layers. In contrast, white strains were hypothesized to meet calcium requirements via intestinal absorption. This may partially explain the improved feed conversion rate in white versus brown strains (Jansen et al., 2020). In addition, while the cumulative production was not found to differ between strains, the total proportion of eggshell was higher in white strains (Jansen et al., 2020), indicating that these hens may require more calcium for eggshell formation. Interestingly, while E2 did not differ between strains during lay, prior to maturation at 17 weeks of age (woa), white strains were found to have significantly higher E2 concentrations than the brown (Habig et al., 2021), possibly triggering a larger recruitment of calcium. This suggests that further studies are required to identify if there are differences in E2 involvement during maturation and active lay between white and brown strains.
Calcium Homeostasis in the Aging Hen
Throughout the laying cycle, hens have been reported to undergo a progressive loss of mineralized structural bone (Webster, 2004). Previously, this was associated with declining E2 as the hen ages, causing medullary bone accumulation to cease and a breakdown of the cortical bone to supply calcium for the eggshell (Yamada et al., 2021). In fact, this decrease in E2 production coincides with the depletion of SWFs from the follicular pool, the associated decline in calcium availability for the shell formation, and slower follicular development, ultimately leading to the termination of lay. The depletion of medullary and cortical bone during the end of lay led to concerns regarding hen health and welfare, along with diminishing egg quality for the breeder and table egg industries. However, intensive genetic selection for extended laying periods resulted in commercial layer breeds adapted to meet these demands without impacting bone or eggshell quality (Hanlon et al., 2021, 2022). In fact, our latest studies suggest that modern strains of layers display recurrent elevations in E2 throughout a laying cycle. We hypothesize that this results in continuous stimulation of medullary bone formation, providing the hen with sufficient calcium storage to keep up with the demands of producing over 500 eggs in 100 weeks of life (Hanlon et al., 2021, 2022). Furthermore, these recurrent elevations in E2 were positively correlated with medullary bone mineral density (BMD) and negatively correlated with cortical BMD, yet cortical bone thickness did not change between 12 and 100 woa. Meanwhile, eggshell thickness and breaking strength did decline over time but never below an acceptable grade A quality (Hanlon et al., 2022). This is partially attributed to the selection for maintained egg size during extended lay (Bouvarel et al., 2011), since it has been determined that a constant quantity of calcium will be supplied to the shell regardless of the weight of the egg (Roland, 1979; Buss, 1988). Thus, understanding the impact of these additional E2 elevations will help understand the physiological needs associated with extended production cycles and adjust dietary calcium and Vitamin D accordingly to promote calcium uptake as the hen ages. As a matter of fact, current knowledge and guidelines, including NRC recommendations (NRC, 1994), may be outdated as it is based on reports that 1α-hydroxylase and intestinal calbindin production will decline with age contributing to the decline in Vitamin D metabolism (Abe et al., 1982; Joyner et al., 1987). However, as E2 promotes the uptake of 1,25(OH)2D3 within the digestive tract (Pfeiffer and Gardner, 1938), we propose that the unintended selection for recurrent E2 peaks has likely resulted in better Vitamin D absorption and synthesis.
Calcium Tetany and Estradiol
Calcium tetany is a condition during which muscle weakness or even paralysis results from hypocalcemia (Turner and Debeer, 2009). While this is a common issue in commercial broiler breeder production systems, this disorder has been poorly characterized within the literature. It has been established that calcium tetany has similar outcomes to the well-described cage layer fatigue in layers, yet the timing of these conditions is vastly different. In the case of laying hens, this is an osteoporotic onset occurring at the end of the reproductive cycle and is typically associated with aging (Whitehead and Fleming, 2000). However, in broiler breeders, hens have been reported to exhibit these symptoms during sexual maturation up until the peak of lay, with a consequential spike in mortality observed (Turner and Debeer, 2009). Numerous management strategies have been recommended ranging from maximizing pullet uniformity to avoiding high calcium diets during the maturation period of breeders. This opposes the dietary recommendation in the laying hen industry, as a pre-lay diet is typically provided to these hens to slowly elevate calcium for the initial formation of the medullary bone ∼10 days prior to the onset of lay. This pre-lay diet includes ∼2% calcium, increasing to ∼4% once hens reach 5% production and remaining constant through the active laying phase (Lohmann-Tierzucht, 2015). Interestingly, breeding companies like Aviagen have suggested that broiler breeder hens should not be provided with elevated dietary calcium until they reach ∼5% production (Turner and Debeer, 2009).
The current literature has alluded that calcium regulation mechanisms initiated during sexual maturation are not responsive to higher calcium inclusion until the onset of lay has commenced (Turner and Debeer, 2009). In support of this hypothesis, studies in our laboratory identified that the initial E2 peak in the laying hen occurs at the time of AFE (Hanlon et al., 2021). In contrast, this elevation in E2 typically associated with sexual maturation occurred 2 weeks after the AFE in the broiler breeder hen (Takeshima, 2018; Takeshima et al., 2019). This could potentially explain the differing responsiveness to dietary calcium between laying hens and broiler breeders. As eggshell formation is initiated, calcium levels are depleted, resulting in a decline in PTH levels. This significantly reduces calcium excretion and increases 1,25(OH)2D3 levels to stimulate calcium absorption (Pfeiffer and Gardner, 1938; Laverty and Clark, 1989). However, without an early elevation in E2 to enhance 1,25(OH)2D3 and calbindin receptors in the intestine, along with the morphological alterations to osteoblast and osteoclast activity, providing dietary calcium too early in broiler breeder hens will result in extreme calcium imbalances. Without the influences of E2, calcium retention will not be able to compensate for the excretion rate. However, further studies are required to validate this hypothesis and understand the integration between E2, AFE, and calcium supplementation to provide better management recommendations in broiler breeder hens.
Osteoporosis and Estradiol
Bone fractures and keel bone deviations have long been attributed to high rates of lay (Gregory and Wilkins, 1989; Rufener and Makagon, 2020), although the etiology of bone disorders has recently been an area of discussion (Jansen et al., 2020; Dunn et al., 2021). However, it is undisputed that keel bone fractures can occur in up to 97% of commercial laying flocks (Wilkins et al., 2011; Richards et al., 2012; Petrik et al., 2014; Heerkens et al., 2016). In laying hens, osteoporosis was previously associated with caged housing systems, hence its designation as cage layer fatigue (Grumbles, 1959). However, the onset of osteoporosis and declining bone quality is much more complex than simply being under the control of the environment. E2 has been negatively associated with cortical BMD while positively correlated with medullary BMD (Hanlon et al., 2022), indicating that the onset of lay predisposes hens to the onset of osteoporosis. This is supported by evidence suggesting that medullary bone adds no structural integrity (Wilson and Thorp, 1998). Regardless, declining E2 in the aging hen results in decreased calcium absorptive capacity, which leads to an overall negative association between E2 and osteoporosis (Abe et al., 1982; Joyner et al., 1987). Altogether, these reports suggest the relationship between this hormone and metabolic condition may be much more complex. The sustained release of the GnRH agonist, deslorelin acetate, diminishes the prevalence of keel bone damage at the expense of the laying cycle (Eusemann et al., 2018b). This supports the hypothesis that lower E2 levels are critical for maintaining skeletal structure integrity (Beck and Hansen, 2004). However, this comes at the expense of egg production and is thus impractical for commercial production. Although research from the early 1960s suggested that bone fragility was induced by E2-treatment, resulting in a thinner cortex (Urist and Deutsch, 1960), recent studies indicate that this may no longer be a primary concern (Hanlon et al., 2022).
Interestingly, osteoporosis has also been associated with obesity (Rosen and Bouxsein, 2006; Zhao et al., 2007). Specifically, visceral fat has been shown to play a detrimental role in the structural integrity of bone (Janicka et al., 2007; Gilsanz et al., 2009). This is consistent with the increased risk of developing osteoporosis under FLHS (Pardee et al., 2012; Yilmaz, 2012). Inducing FLHS via HELP diets results in upregulated bone turnover and detectable damage to skeletal integrity (Jiang et al., 2013). This suggests an additional link between the roles of E2 on fat metabolism and bone development. As a matter of fact, the inclusion of long-term dietary lipids has been detrimental to bone remodelling (Liu et al., 2003, 2004), bone formation rate (Watkins et al., 1997), and bone mineral content (Liu et al., 2004). Specifically, OC alleviated liver damage caused by this high-lipid diet (Wu et al., 2021). OC levels are highest in the pullet phase, slowly declining to reach the lowest levels at the end of a laying cycle (Jiang et al., 2013; Nys and le Roy, 2018), thus displaying an inverse relationship with E2 during maturation. Wu et al. (2021) proposed that OC can counteract the onset of FLHS in older laying hens. Taking that one step further, we hypothesize that the recurrent elevations in E2 we reported (Hanlon et al., 2021) are key to sustaining extended reproduction and balancing the activation of bone and liver metabolism to support egg formation.
Conclusion
In conclusion, the involvement of E2 in activating the reproductive axis, liver metabolism, and medullary bone formation demonstrates that this hormone contributes to and may coordinate all aspects of egg formation. However, while the success of the breeder and table egg industries is highly dependent on E2, little research has considered the underlying endocrinological alterations responsible for improvements in laying rate. These effects are primarily mediated through ERα, resulting in the production of yolk proteins in the liver and the storage of calcium in the medullary bone while also coordinating their transport to the reproductive tract. However, by diverting nutrients and energy towards egg formation, E2 can also contribute to the onset of metabolic disorders, such as FLHS and calcium tetany. Nonetheless, decades of genetic selection for egg production have impacted the patterns of E2 production, which may have helped accommodate the further demands in modern strains of commercial laying hens. Interestingly, this may not be the case in broiler breeder hens undergoing selection for rapid growth, as a delay in the initial rise in E2 is associated with calcium tetany during sexual maturation. Thus, it is apparent that rather than absolute concentrations, the timing of E2 production is key to successful reproduction, which requires tight coordination with metabolic processes to prevent liver and skeletal damage. As ERs are present in several additional metabolic organs, including adipose and kidney in mammals, future investigations should consider the impact this hormone may have on the control of energy partitioning between growth and reproductive process, particularly during the preparation for lay.
Author Contributions
CH was the primary author and performed most of the literature search. CZ contributed to the estradiol and reproduction section, created the figures, and participated in the redaction of all sections. GB participated in the redaction of all sections and supported CH throughout the project as the supervisor. All authors contributed to the article and approved the submitted version.
Funding
This review was in partly supported by funding from the Canada First Research Excellence Fund (Food from Thought ‐ Agricultural Systems for a Healthy Planet), Egg Farmers of Canada, the Ontario Ministry of Agriculture and Rural Affairs (OMAFRA) Ontario Agri-Food Innovation Alliance Program (#UofG2018-3148) and the Natural Sciences and Engineering Research Council of Canada (NSERC CRDPJ program #CRDPJ543662-19).
Conflict of Interest
The authors declare that the research was conducted in the absence of any commercial or financial relationships that could be construed as a potential conflict of interest.
Publisher’s Note
All claims expressed in this article are solely those of the authors and do not necessarily represent those of their affiliated organizations, or those of the publisher, the editors and the reviewers. Any product that may be evaluated in this article, or claim that may be made by its manufacturer, is not guaranteed or endorsed by the publisher.
References
Abdelnabi M. A., Richards M. P., Ottinger M. A. (2001). Comparison of Gonadal Hormone Levels in Turkey Embryos Incubated in Long-Term Shell-Less Culture and in Ovo. Poult. Sci. 80, 666–669. doi:10.1093/ps/80.5.666
Abe E., Horikawa H., Masumura T., Sugahara M., Kubota M., Suda T. (1982). Disorders of Cholecaiciferol Metabolism in Old Egg-Laying Hens. J. Nutr. 112, 436–446. doi:10.1093/jn/112.3.436
Acharya K. D., Veney S. L. (2012). Characterization of the G-Protein-Coupled Membrane-Bound Estrogen Receptor GPR30 in the Zebra Finch Brain Reveals a Sex Difference in Gene and Protein Expression. Devel Neurobio 72, 1433–1446. doi:10.1002/dneu.22004
Adkins-Regan E., Ottinger M. A., Park J. (1995). Maternal Transfer of Estradiol to Egg Yolks Alters Sexual Differentiation of Avian Offspring. J. Exp. Zool. 271, 466–470. doi:10.1002/jez.1402710608
Adlercreutz H. (1970). Oestrogen Metabolism in Liver Disease. J. Endorcr. 46, 129–163. doi:10.1677/joe.0.0460129
Ali S. Y. (1992). “Matrix Formation and Mineralisation in Bone,” in Bone Biology and Skeletal Disorders in Poultry. Editor C. C. Whitehead (Oxfordshire, UK: Carfax Publishing Co), 19–38.
Andrews J. E., Smith C. A., Sinclair A. H. (1997). Sites ofEstrogen ReceptorandAromataseExpression in the Chicken Embryo. General Comp. Endocrinol. 108, 182–190. doi:10.1006/gcen.1997.6978
Bain M. M., Nys Y., Dunn I. C. (2016). Increasing Persistency in Lay and Stabilising Egg Quality in Longer Laying Cycles. What Are the Challenges? Br. Poult. Sci. 57, 330–338. doi:10.1080/00071668.2016.1161727
Balnave D. (1968). Influence of Dietary Linoleic Acid on Egg Fatty Acid Composition in Hens Deficient in Essential Fatty Acids. J. Sci. Food Agric. 19, 266–269. doi:10.1002/jsfa.2740190507
Balnave D., Pearce J. (1974). Induction by Gonadal Hormones of Hepatic Lipogenic Enzyme Activity in Immature Pullets. J. Endocrinol. 61, 29–43. doi:10.1677/joe.0.0610029
Balnave D. (1971). The Influence of Exogenous Oestrogens and the Attainment of Sexual Maturity on Fatty Acid Metabolism in the Immature Pullet. Comp. Biochem. Physiology Part B Comp. Biochem. 40, 189–197. doi:10.1016/0305-0491(71)90075-7
Bar A. (2008). Calcium Homeostasis and Vitamin D Metabolism and Expression in Strongly Calcifying Laying Birds. Comp. Biochem. Physiology Part A Mol. Integr. Physiology 151, 477–490. doi:10.1016/j.cbpa.2008.07.006
Bar A. (2009). Differential Regulation of Calbindin in the Calcium-Transporting Organs of Birds with High Calcium Requirements. J. Poult. Sci. 46, 267–285. doi:10.2141/jpsa.46.267
Barakat R., Oakley O., Kim H., Jin J., Ko C. J. (2016). Extra-gonadal Sites of Estrogen Biosynthesis and Function. BMB Rep. 49, 488–496. doi:10.5483/BMBRep.2016.49.9.141
Beaufrère H. (2013). Atherosclerosis: Comparative Pathogenesis, Lipoprotein Metabolism, and Avian and Exotic Companion Mammal Models. J. Exot. Pet Med. 22, 320–335. doi:10.1053/j.jepm.2013.10.016
Beck M. M., Hansen K. K. (2004). Role of Estrogen in Avian Osteoporosis. Poult. Sci. 83, 200–206. doi:10.1093/ps/83.2.200
Bédécarrats G. Y., Baxter M., Sparling B. (2016). An Updated Model to Describe the Neuroendocrine Control of Reproduction in Chickens. General Comp. Endocrinol. 227, 58–63. doi:10.1016/j.ygcen.2015.09.023
Bédécarrats G. Y. (2015). Control of the Reproductive axis: Balancing Act between Stimulatory and Inhibitory Inputs. Poult. Sci. 94, 810–815. doi:10.3382/ps/peu042
Benoit J., Clavert J. (1945). Role indispensable des parathyroids dans la calcification de l’osfolliculinque chez le canard domestique. Cr. Seanc. Soc. Biol. 139, 743.
Bensadoun A., Rothfeld A. (1972). The Form of Absorption of Lipids in the Chicken, Gallus domesticus. Exp. Biol. Med. 141, 814–817. doi:10.3181/00379727-141-36878
Bentley G. E., Perfito N., Ukena K., Tsutsui K., Wingfield J. C. (2003). Gonadotropin-inhibitory Peptide in Song Sparrows (Melospiza Melodia) in Different Reproductive Conditions, and in House Sparrows (Passer domesticus) Relative to Chicken-Gonadotropin-Releasing Hormone. J. Neuroendocrinol. 15, 794–802. doi:10.1046/j.1365-2826.2003.01062.x
Bentley G. E., Ubuka T., McGuire N. L., Chowdhury V. S., Morita Y., Yano T., et al. (2008). Gonadotropin-inhibitory Hormone and its Receptor in the Avian Reproductive System. General Comp. Endocrinol. 156, 34–43. doi:10.1016/j.ygcen.2007.10.003
Bergink E. W., Wallace R. A. (1974). Precursor-Product Relationship between Amphibian Vitellogenin and the Yolk Proteins, Lipovitellin and Phosvitin. J. Biol. Chem. 249, 2897–2903. doi:10.1016/s0021-9258(19)42715-4
Bloom M. A., Domm L. V., Nalbandov A. V., Bloom W. (1958). Medullary Bone of Laying Chickens. Am. J. Anat. 102, 411–453. doi:10.1002/aja.1001020304
Bloom W., Bloom M. A., McLean F. C. (1941). Calcification and Ossification. Medullary Bone Changes in the Reproductive Cycle of Female Pigeons. Anat. Rec. 81, 443–475. doi:10.1002/ar.1090810404
Bouvarel I., Nys Y., Lescoat P. (2011). “Hen Nutrition for Sustained Egg Quality,” in Improving the Safety and Quality of Eggs and Egg Products, Vol 1: Egg Chemistry, Production and Consumption. Editors Y. Nys, M. Bain, and F. van Immerseel (Cambridge, UK: Woodhead Publishing Ltd), 261–299. doi:10.1533/9780857093912.3.261
Bradfield J. R. G. (1951). Radiographic Studies on the Formation of the Hen'S Egg Shell. J. Exp. Biol. 28, 125–140. doi:10.1242/jeb.28.2.125
Brady L., Romsos D. R., Leveille G. A. (1976). In Vivo Estimation of Fatty Acid Synthesis in the Chicken (Gallus Domesticus) Utilizing 3H2O. Comp. Biochem. Physiology Part B Comp. Biochem. 54, 403–407. doi:10.1016/0305-0491(76)90265-0
Braet F., Wisse E. (2002). Structural and Functional Aspects of Liver Sinusoidal Endothelial Cell Fenestrae: A Review. Comp. Hepatol. 1, 1–17. doi:10.1186/1476-5926-1-1
Bragg D. B., Sim J. S., Hodgson G. C. (1973). Influence of Dietary Energy Source on Performance and Fatty Liver Syndrome in White Leghorn Laying Hens. Poult. Sci. 52, 736–740. doi:10.3382/ps.0520736
Brown E. M. (1991). Extracellular Ca2+ Sensing, Regulation of Parathyroid Cell Function, and Role of Ca2+ and Other Ions as Extracellular (First) Messengers. Physiol. Rev. 71, 371–411. doi:10.1152/physrev.1991.71.2.371
Brunström B., Axelsson J., Mattsson A., Halldin K. (2009). Effects of Estrogens on Sex Differentiation in Japanese Quail and Chicken. General Comp. Endocrinol. 163, 97–103. doi:10.1016/j.ygcen.2009.01.006
Buss E. G. (1988). “Correlation of Egg Shell Weight with Egg Weight, Body Weight, and Percent Shell,” in Proceedings of the XVIII World’s Poultry Congress, 348–349. Available at: https://naldc.nal.usda.gov/download/CAT10414984/PDF (Accessed June 7, 2022).
Butler E. J. (1976). Fatty Liver Diseases in the Domestic Fowl - A Review. Avian Pathol. 5, 1–14. doi:10.1080/03079457608418164
Calvo F. O., Bahr J. M. (1983). Adenylyl Cyclase System of the Small Preovulatory Follicles of the Domestic Hen: Responsiveness to Follicle-Stimulating Hormone and Luteinizing Hormone 1. Biol. Reproduction 29, 542–547. doi:10.1095/biolreprod29.3.542
Calvo F. O., Wang S.-C., Bahr J. M. (1981). LH-stimulable Adenylyl Cyclase Activity during the Ovulatory Cycle in Granulosa Cells of the Three Largest Follicles and the Postovulatory Follicle of the Domestic Hen (Gallus domesticus). Biol. Reproduction 25, 805–812. doi:10.1095/biolreprod25.4.805
Camacho-Arroyo I., González-Arenas A., González-Agüero G., Guerra-Araiza C., González-Morán G. (2003). “Changes in the Content of Progesterone Receptor Isoforms and Estrogen Receptor Alpha in the Chick Brain during Embryonic Development,” in Comparative Biochemistry and Physiology - A Molecular and Integrative Physiology (Elsevier), 136, 447–452. doi:10.1016/S1095-6433(03)00204-6Comp. Biochem. Physiology Part A Mol. Integr. Physiology
Chan C. Z., Sato K., Shimada Y. (1990). Three-dimensional Electron Microscopy of the Sarcoplasmic Reticulum and T-System in Embryonic Chick Skeletal Muscle Cells In Vitro. Protoplasma 154, 112–121. doi:10.1007/bf01539838
Chapman M. J., Goldstein S., Laudat M.-H. (1977). Characterization and Comparative Aspects of the Serum Very Low and Low Density Lipoproteins and Their Apoproteins in the Chicken (Gallus domesticus). Biochemistry 16, 3006–3015. doi:10.1021/bi00632a031
Chen L.-R., Lee S.-C., Lin Y.-P., Hsieh Y.-L., Chen Y.-L., Yang J.-R., et al. (2010). Prostaglandin-D Synthetase Induces Transcription of the LH Beta Subunit in the Primary Culture of Chicken Anterior Pituitary Cells via the PPAR Signaling Pathway. Theriogenology 73, 367–382. doi:10.1016/j.theriogenology.2009.09.020
Christenson R. H. (1997). Biochemical Markers of Bone Metabolism: An Overview. Clin. Biochem. 30, 573–593. doi:10.1016/s0009-9120(97)00113-6
Ciccone N. A., Dunn I. C., Boswell T., Tsutsui K., Ubuka T., Ukena K., et al. (2004). Gonadotrophin Inhibitory Hormone Depresses Gonadotrophin Alpha and Follicle-Stimulating Hormone Beta Subunit Expression in the Pituitary of the Domestic Chicken. J. Neuroendocrinol. 16, 999–1006. doi:10.1111/j.1365-2826.2005.01260.x
Clemens T. L., Garrett K. P., Zhou X.-Y., Pike J. W., Haussler M. R., Dempster D. W. (1988). Immunocytochemical Localization of the 1,25-Dihydroxyvitamin D3Receptor in Target Cells*. Endocrinology 122, 1224–1230. doi:10.1210/endo-122-4-1224
Clunies M., Leeson S. (1995). Effect of Dietary Calcium Level on Plasma Proteins and Calcium Flux Occurring during a 24 H Ovulatory Cycle. Can. J. Anim. Sci. 75, 439–444. doi:10.4141/cjas95-064
Cochrane A. W., Deeley R. G. (1988). Estrogen-dependent Activation of the Avian Very Low Density Apolipoprotein II and Vitellogenin Genes. J. Mol. Biol. 203, 555–567. doi:10.1016/0022-2836(88)90192-1
Codina-Salada J., Moore J. P., Chan L. (1983). Kinetics of Primary and Secondary Stimulation of the mRNA for Apovldl-II, a Major Yolk Protein, in the Cockerel Liver by Estrogen. Endocrinology 113, 1158–1160. doi:10.1210/endo-113-3-1158
Common R. H., Rutledge W. A., Hale R. W. (1948). Observations on the Mineral Metabolism of Pullets: VIII. The Influence of Gonadal Hormones on Retention of Calcium and Phosphorus. J. Agric. Sci. 38, 64–80. doi:10.1017/s0021859600005153
Corradino R. A., Wasserman R. H., Pubols M. H., Chang S. I. (1968). Vitamin D3 Induction of a Calcium-Binding Protein in the Uterus of the Laying Hen. Archives Biochem. Biophysics 125, 378–380. doi:10.1016/0003-9861(68)90674-7
Coty W. A. (1980). A Specific, High Affinity Binding Protein for 1α,25-Dihydroxy Vitamin D in the Chick Oviduct Shell Gland. Biochem. Biophysical Res. Commun. 93, 285–292. doi:10.1016/s0006-291x(80)80278-6
Couch J. R. (1956). Fatty Livers in Laying Hens - a Condition Which May Occur as a Result of Increased Strain. Feedstuffs 28, 46–53.
Cui H., Zhao G., Liu R., Zheng M., Chen J., Wen J. (2012). FSH Stimulates Lipid Biosynthesis in Chicken Adipose Tissue by Upregulating the Expression of its Receptor FSHR. J. Lipid Res. 53, 909–917. doi:10.1194/jlr.M025403
Cui J., Shen Y., Li R. (2013). Estrogen Synthesis and Signaling Pathways during Aging: From Periphery to Brain. Trends Mol. Med. 19, 197–209. doi:10.1016/j.molmed.2012.12.007
Cui Z., Amevor F. K., Feng Q., Kang X., Song W., Zhu Q., et al. (2020). Sexual Maturity Promotes Yolk Precursor Synthesis and Follicle Development in Hens via Liver-Blood-Ovary Signal axis. Animals 10, 1–14. doi:10.3390/ani10122348
Cutting A. D., Ayers K., Davidson N., Oshlack A., Doran T., Sinclair A. H., et al. (2014). Identification, Expression, and Regulation of Anti-müllerian Hormone Type-II Receptor in the Embryonic Chicken Gonad. Biol. Reprod. 90, 106–112. doi:10.1095/biolreprod.113.116491
Dacke C. G., Arkle S., Cook D. J., Wormstone I. M., Jones S., Zaidi M., et al. (1993). Medullary Bone and Avian Calcium Regulation. J. Exp. Biol. 184, 63–88. doi:10.1242/jeb.184.1.63
Dacke C. G., Sugiyama T., Gay C. V. (2015). “The Role of Hormones in the Regulation of Bone Turnover and Eggshell Calcification,” in Sturkie’s Avian Physiology (London, UK: Academic Press), 549–575. doi:10.1016/b978-0-12-407160-5.00025-7
Dashti N., Kelley J. L., Thayer R. H., Ontko J. A. (1983). Concurrent Inductions of Avian Hepatic Lipogenesis, Plasma Lipids, and Plasma Apolipoprotein B by Estrogen. J. Lipid Res. 24, 368–380. doi:10.1016/s0022-2275(20)37977-3
Deeley R. G., Gordon J. I., Burns A. T., Mullinix K. P., Binastein M., Goldberg R. F. (1977). Primary Activation of the Vitellogenin Gene in the Rooster. J. Biol. Chem. 252, 8310–8319. doi:10.1016/s0021-9258(17)40972-0
Deeley R., Mullinix D., Wetekam W., Kronenberg H., Meyers M., Eldridge J., et al. (1975). Vitellogenin Synthesis in the Avian Liver. Vitellogenin Is the Precursor of the Egg Yolk Phosphoproteins. J. Biol. Chem. 250, 9060–9066. doi:10.1016/s0021-9258(19)40693-5
Deluca H. F. (2004). Overview of General Physiologic Features and Functions of Vitamin D. Am. J. Clin. Nutr. 80, 1689S–96S. doi:10.1093/ajcn/80.6.1689S
Deluca H. F. (1980). William C. Rose Lectureship in Biochemistry and Nutrition. Some New Concepts Emanating from a Study of the Metabolism and Function of Vitamin D. Nutr. Rev. 38, 169–182. doi:10.1111/j.1753-4887.1980.tb05887.x
DeLuca H. F. (1976). Metabolism of Vitamin D: Current Status. Am. J. Clin. Nutr. 29, 1258–1270. doi:10.1093/ajcn/29.11.1258
Drummond A. E., Fuller P. J. (2010). The Importance of ERβ Signalling in the Ovary. J. Endocrinol. 205, 15–23. doi:10.1677/JOE-09-0379
Dunn I. C., de Koning D.-J., McCormack H. A., Fleming R. H., Wilson P. W., Andersson B., et al. (2021). No Evidence that Selection for Egg Production Persistency Causes Loss of Bone Quality in Laying Hens. Genet. Sel. Evol. 53, 1–13. doi:10.1186/s12711-021-00603-8
Dunn I., Lewis P., Wilson P., Sharp P. (2003). Acceleration of Maturation of FSH and LH Responses to Photostimulation in Prepubertal Domestic Hens by Oestrogen. Reproduction 126, 217–225. doi:10.1530/rep.0.1260217
Elbrecht A., Smith R. G. (1992). Aromatase Enzyme Activity and Sex Determination in Chickens. Science 255, 467–470. doi:10.1126/science.1734525
Elf P. K., Fivizzani A. J. (2002). Changes in Sex Steroid Levels in Yolks of the Leghorn chicken,Gallus domesticus, during Embryonic Development. J. Exp. Zool. 293, 594–600. doi:10.1002/jez.10169
Elnager S., Abd-Elhady A. (2009). Exogenous Estradiol: Productive and Reproductive Performance and Physiological Profile of Japanese Quail Hens. Int. J. Poult. Sci. 8, 634–641. doi:10.3923/ijps.2009.634.641
Emmen J. M. A., Couse J. F., Elmore S. A., Yates M. M., Kissling G. E., Korach K. S. (2005). In Vitro Growth and Ovulation of Follicles from Ovaries of Estrogen Receptor (ER)α and ERβ Null Mice Indicate a Role for ERβ in Follicular Maturation. Endocrinology 146, 2817–2826. doi:10.1210/en.2004-1108
Etches R. J. (1987). Calcium Logistics in the Laying Hen. J. Nutr. 117, 619–628. doi:10.1093/jn/117.3.619
Etches R., Kagami H. (1997). “Genotypic and Phenotypic Sex Reversal,” in Perspectives in Avian Endocrinology. Editors S. Harvey, and R. Etches (Bristol: Journal of Endocrinology Ltd), 57–67.
Eusemann B. K., Baulain U., Schrader L., Thöne-Reineke C., Patt A., Petow S. (2018a). Radiographic Examination of Keel Bone Damage in Living Laying Hens of Different Strains Kept in Two Housing Systems. PLoS ONE 13, e0194974–17. doi:10.1371/journal.pone.0194974
Eusemann B. K., Sharifi A. R., Patt A., Reinhard A.-K., Schrader L., Thöne-Reineke C., et al. (2018b). Influence of a Sustained Release Deslorelin Acetate Implant on Reproductive Physiology and Associated Traits in Laying Hens. Front. Physiol. 9, 1–11. doi:10.3389/fphys.2018.01846
Evans M. I., O'Malley P. J., Krust A., Burch J. B. (1987). Developmental Regulation of the Estrogen Receptor and the Estrogen Responsiveness of Five Yolk Protein Genes in the Avian Liver. Proc. Natl. Acad. Sci. U.S.A. 84, 8493–8497. doi:10.1073/pnas.84.23.8493
Evans M. I., Silva R., Burch J. B. (1988). Isolation of Chicken Vitellogenin I and III cDNAs and the Developmental Regulation of Five Estrogen-Responsive Genes in the Embryonic Liver. Genes Dev. 2, 116–124. doi:10.1101/gad.2.1.116
Filardo E. J., Quinn J. A., Bland K. I., Frackelton A. R., Williams R. (2000). Estrogen-Induced Activation of Erk-1 and Erk-2 Requires the G Protein-Coupled Receptor Homolog, GPR30, and Occurs via Trans-activation of the Epidermal Growth Factor Receptor through Release of HB-EGF. Mol. Endocrinol. 14, 1649–1660. doi:10.1210/mend.14.10.0532
Fleming R. H., McCormack H. A., Mcteir L., Whitehead C. C. (1998). Medullary Bone and Humeral Breaking Strength in Laying Hens. Res. Veterinary Sci. 64, 63–67. doi:10.1016/s0034-5288(98)90117-5
Flouriot G., Pakdel F., Valotaire Y. (1996). Transcriptional and Post-transcriptional Regulation of Rainbow Trout Estrogen Receptor and Vitellogenin Gene Expression. Mol. Cell. Endocrinol. 124, 173–183. doi:10.1016/s0303-7207(96)03960-3
Fraser R., Day W. A., Fernando N. S. (1986). Review: the Liver Sinusoidal Cells. Their Role in Disorders of the Liver, Lipoprotein Metabolism and Atherogenesis. Pathology 18, 5–11. doi:10.3109/00313028609090821
Furr B. J. A., Bonney R. C., England R. J., Cunningham F. J. (1973). Luteinizing Hormone and Progesterone in Peripheral Blood during the Ovulatory Cycle of the Hen Gallus Domesticus. J. Endocrinol. 57, 159–169. doi:10.1677/joe.0.0570159
Gasc J.-M., Baulieu E.-E. (1988). Regulation by Estradiol of the Progesterone Receptor in the Hypothalamus and Pituitary: An Immunohistochemical Study in the Chicken. Endocrinology 122, 1357–1365. doi:10.1210/endo-122-4-1357
Ge C., Yu M., Zhang C. (2012). G Protein-Coupled Receptor 30 Mediates Estrogen-Induced Proliferation of Primordial Germ Cells via EGFR/Akt/β-Catenin Signaling Pathway. Endocrinology 153, 3504–3516. doi:10.1210/en.2012-1200
Gilsanz V., Chalfant J., Mo A. O., Lee D. C., Dorey F. J., Mittelman S. D. (2009). Reciprocal Relations of Subcutaneous and Visceral Fat to Bone Structure and Strength. J. Clin. Endocrinol. Metabolism 94, 3387–3393. doi:10.1210/jc.2008-2422
Gray R. W., Omdahl J. L., Ghazarian J. G., DeLuca H. F. (1972). 25-Hydroxycholecalciferol-1-hydroxylase. J. Biol. Chem. 247, 7528–7532. doi:10.1016/s0021-9258(19)44557-2
Gregory N. G., Wilkins L. J. (1989). Broken Bones in Domestic Fowl: Handling and Processing Damage in End‐of‐lay Battery Hens. Br. Poult. Sci. 30, 555–562. doi:10.1080/00071668908417179
Griffin C., Flouriot G., Sonntag-buck V., Gannon F. (1999). Two Functionally Different Protein Isoforms Are Produced from the Chicken Estrogen Receptor-α Gene. Mol. Endocrinol. 13, 1571–1587. doi:10.1210/mend.13.9.0336
Griffin H. D., Perry M. M. (1985). Exclusion of Plasma Lipoproteins of Intestinal Origin from Avian Egg Yolk Because of Their Size. Comp. Biochem. Physiology Part B Comp. Biochem. 82, 321–325. doi:10.1016/0305-0491(85)90248-2
Griffin H., Grant G., Perry M. (1982). Hydrolysis of Plasma Triacylglycerol-Rich Lipoproteins from Immature and Laying Hens (Gallus domesticus) by Lipoprotein Lipase In Vitro. Biochem. J. 206, 647–654. doi:10.1042/bj2060647
Griffin H. P. (1981). Plasma Very Low Density Lipoproteins (VLDL) in Immature and Laying Hens (Gallus domesticus). Biochem. Soc. Trans. 155, 1. doi:10.1042/bst009155p
Gruber M., Bos E. S., Ab G. (1976). Hormonal Control of Vitellogenin Synthesis in Avian Liver. Mol. Cell. Endocrinol. 5, 41–50. doi:10.1016/0303-7207(76)90069-1
Grumbles L. C. (1959). Cage Layer Fatigue (Cage Paralysis). Avian Dis. 3, 122–125. doi:10.2307/1587714
Habig C., Weigend A., Baulain U., Petow S., Weigend S. (2021). Influence of Age and Phylogenetic Background on Blood Parameters Associated with Bone Metabolism in Laying Hens. Front. Physiol. 12, 1–9. doi:10.3389/fphys.2021.678054
Hadinia S. H., Carneiro P. R. O., Fitzsimmons C. J., Bédécarrats G. Y., Zuidhof M. J. (2020). Post-photostimulation Energy Intake Accelerated Pubertal Development in Broiler Breeder Pullets. Poult. Sci. 99, 2215–2229. doi:10.1016/j.psj.2019.11.065
Haghighi-Rad F., Polin D. (1982). Lipid Alleviates Fatty Liver Hemorrhagic Syndrome. Poult. Sci. 61, 2465–2472. doi:10.3382/ps.0612465
Haghighi-Rad F., Polin D. (1981). The Relationship of Plasma Estradiol and Progesterone Levels to the Fatty Liver Hemorrhagic Syndrome in Laying Hens ,. Poult. Sci. 60, 2278–2283. doi:10.3382/ps.0602278
Hanke H., Hanke S., Bruck B., Brehme U., Gugel N., Finking G., et al. (1996). Inhibition of the Protective Effect of Estrogen by Progesterone in Experimental Atherosclerosis. Atherosclerosis 121, 129–138. doi:10.1016/0021-9150(95)05710-2
Hanlon C., Ramachandran R., Zuidhof M. J., Bédécarrats G. Y. (2020). Should I Lay or Should I Grow: Photoperiodic versus Metabolic Cues in Chickens. Front. Physiol. 11, 707–724. doi:10.3389/fphys.2020.00707
Hanlon C., Takeshima K., Bédécarrats G. Y. (2021). Changes in the Control of the Hypothalamic-Pituitary Gonadal Axis across Three Differentially Selected Strains of Laying Hens (Gallus gallus Domesticus). Front. Physiol. 12, 1–20. doi:10.3389/fphys.2021.651491
Hanlon C., Takeshima K., Kiarie E. G., Bédécarrats G. Y. (2022). Bone and Eggshell Quality throughout an Extended Laying Cycle in Three Strains of Layers Spanning 50 Years of Selection. Poult. Sci. 101, 101672. doi:10.1016/j.psj.2021.101672
Hansen K., Kittok R., Sarath G., Toombs C., Caceres N., Beck M. (2003). Estrogen Receptor-Alpha Populations Change with Age in Commercial Laying Hens. Poult. Sci. 82, 1624–1629. doi:10.1093/ps/82.10.1624
Hansen R. J., Walzem R. L. (1993). Avian Fatty Liver Hemorrhagic Syndrome: a Comparative Review. Adv. Vet. Sci. Comp. Med. 37, 451–468.
Harms R. H., Costa P. T., Miles R. D. (1982). Daily Feed Intake and Performance of Laying Hens Grouped According to Their Body Weight. Poult. Sci. 61, 1021–1024. doi:10.3382/ps.0611021
Harms R. H., Roland D. A., Simpson C. F. (1977). Experimentally Induced “Fatty Liver Syndrome” Condition in Laying Hens. Poult. Sci. 56, 517–520. doi:10.3382/ps.0560517
Hartmann C., Johansson K., Strandberg E., Rydhmer L. (2003). Genetic Correlations between the Maternal Genetic Effect on Chick Weight and the Direct Genetic Effects on Egg Composition Traits in a White Leghorn Line. Poult. Sci. 82, 1–8. doi:10.1093/ps/82.1.1
Hasegawa S., Sato K., Hikami Y., Mizuno T. (1980). Effect of Estrogen on Adipose Tissue Accumulation in Chicks, with Reference to Changes in its Chemical Composition and Lipase Activity. Nihon Chikusan Gakkaiho 51, 360–367. doi:10.2508/chikusan.51.360
Haussler M. R. (1974). Vitamin D: Mode of Action and Biomedical Applications. Nutr. Rev. 32, 257–266. doi:10.1111/j.1753-4887.1974.tb00970.x
Haussler M. R. (1986). Vitamin D Receptors: Nature and Function. Annu. Rev. Nutr. 6, 527–562. doi:10.1146/annurev.nu.06.070186.002523
Hawkins R. A., Heald P. J., Taylor P. (1969). The Uptake of (6,7-3H) 17β-Oestradiol by Tissues of the Domestic Fowl during an Ovulation Cycle. Acta Endocrinol. 60, 210–215. doi:10.1530/acta.0.0600210
Heerkens J. L. T., Delezie E., Rodenburg T. B., Kempen I., Zoons J., Ampe B., et al. (2016). Risk Factors Associated with Keel Bone and Foot Pad Disorders in Laying Hens Housed in Aviary Systems. Poult. Sci. 95, 482–488. doi:10.3382/ps/pev339
Hemsell D. L., Grodin J. M., Brenner P. F., Siiteri P. K., Macdonald P. C. (1974). Plasma Precursors of Estrogen. II. Correlation of the Extent of Conversion of Plasma Androstenedione to Estrone with Age1. J. Clin. Endocrinol. Metabolism 38, 476–479. doi:10.1210/jcem-38-3-476
Hermier D. (1997). Lipoprotein Metabolism and Fattening in Poultry. J. Nutr. 127, 805–808. doi:10.1093/jn/127.5.805s
Hermier D., Rousselot-Pailley D., Peresson R., Sellier N. (1994). Influence of Orotic Acid and Estrogen on Hepatic Lipid Storage and Secretion in the Goose Susceptible to Liver Steatosis. Biochimica Biophysica Acta (BBA) - Lipids Lipid Metabolism 1211, 97–106. doi:10.1016/0005-2760(94)90143-0
Hrabia A. (2015). Growth Hormone Production and Role in the Reproductive System of Female Chicken. General Comp. Endocrinol. 220, 112–118. doi:10.1016/j.ygcen.2014.12.022
Hrabia A., Paczoska-Eliasiewicz H., Rząsa J. (2004). Effect of Prolactin on Estradiol and Progesterone Secretion by Isolated Chicken Ovarian Follicles. folia Biol. (krakow) 52, 197–203. doi:10.3409/1734916044527494
Hrabia A., Wilk M., Rząsa J. (2008). Expression of α and β Estrogen Receptors in the Chicken Ovary. folia Biol. (krakow) 56, 187–191. doi:10.3409/fb.56_3-4.187-191
Hsueh A. J. W., Peck E. J., Clark J. H. (1976). Control of Uterine Estrogen Receptor Levels by Progesterone. Endocrinology 98, 438–444. doi:10.1210/endo-98-2-438
Hurwitz S., Bar A. (1965). Absorption of Calcium and Phosphorus along the Gastrointestinal Tract of the Laying Fowl as Influenced by Dietary Calcium and Egg Shell Formation. J. Nutr. 86, 433–438. doi:10.1093/jn/86.4.433
Hurwitz S. (1989). “Parathyroid Hormone,” in Vertebrate Endocrinology: Fundamentals and Biomedical Implications. Editors P. K. T. Pang, and M. P. Schreibman (New York, USA: Academic Press), 45–77.
Husbands D. R. (1972). The Distribution of Lipoprotein Lipase in Tissues of the Domestic Fowl and the Effects of Feeding and Starving. Br. Poult. Sci. 13, 85–90. doi:10.1080/00071667208415919
Hutz R. J. (1989). Disparate Effects of Estrogens on In Vitro Steroidogenesis by Mammalian and Avian Granulosa Cells1. Biol. Reproduction 40, 709–713. doi:10.1095/biolreprod40.4.709
Ikemoto T., Park M. K. (2005). Chicken RFamide-Related Peptide (GnIH) and Two Distinct Receptor Subtypes: Identification, Molecular Characterization, and Evolutionary Considerations. J. Reproduction Dev. 51, 359–377. doi:10.1262/jrd.16087
Imamura T., Sugiyama T., Kusuhara S. (2006). Expression and Localization of Estrogen Receptors Alpha and Beta mRNA in Medullary Bone of Laying Hens. Anim. Sci. J. 77, 223–229. doi:10.1111/j.1740-0929.2006.00341.x
Ishimaru Y., Komatsu T., Kasahara M., Katoh-Fukui Y., Ogawa H., Toyama Y., et al. (2008). Mechanism of Asymmetric Ovarian Development in Chick Embryos. Development 135, 677–685. doi:10.1242/dev.012856
Isola J. J. (1990). Distribution of Estrogen and Progesterone Receptors and Steroid-Regulated Gene Products in the Chick Oviduct. Mol. Cell. Endocrinol. 69, 235–243. doi:10.1016/0303-7207(90)90017-3
Jacob J., Pescatore T. (2013). Avian Skeletal System. Lexington, KY: Cooperative Extension Service University of Kentucky College of Agriculture, Food and Environment, 1–2. Available at: www.ca.uky.edu.
Jande S. S., Tolnai S., Lawson D. E. M. (1981). Immunohistochemical Localization of Vitamin D-dependent Calcium-Binding Protein in Duodenum, Kidney, Uterus and Cerebellum of Chickens. Histochemistry 71, 99–116. doi:10.1007/bf00592574
Janicka A., Wren T. A. L., Sanchez M. M., Dorey F., Kim P. S., Mittelman S. D., et al. (2007). Fat Mass Is Not Beneficial to Bone in Adolescents and Young Adults. J. Clin. Endocrinol. Metabolism 92, 143–147. doi:10.1210/jc.2006-0794
Jansen S., Baulain U., Habig C., Weigend A., Halle I., Scholz A. M., et al. (2020). Correction: Jansen, S., et al. Relationship between Bone Stability and Egg Production in Genetically Divergent Chicken Layer Lines. Animals 2020, 10, 850. Animals 10, 2355–5. doi:10.3390/ani10122355
Jaussi A. W., Newcomer W. S., Thayer R. H. (1962). Hyperlipemic Effect of ACTH Injections in the Chicken. Poult. Sci. 41, 528–532. doi:10.3382/ps.0410528
Jiang S., Cheng H. W., Cui L. Y., Zhou Z. L., Hou J. F. (2013). Changes of Blood Parameters Associated with Bone Remodeling Following Experimentally Induced Fatty Liver Disorder in Laying Hens. Poult. Sci. 92, 1443–1453. doi:10.3382/ps.2012-02800
Jiang Z., Yang Z., Zhang H., Yao Y., Ma H. (2021). Genistein Activated Adenosine 5′-Monophosphate-Activated Protein Kinase-Sirtuin1/peroxisome Proliferator-Activated Receptor γ Coactivator-1α Pathway Potentially through Adiponectin and Estrogen Receptor β Signaling to Suppress Fat Deposition in Broiler Chickens. Poult. Sci. 100, 246–255. doi:10.1016/j.psj.2020.10.013
Jin K., Zuo Q., Song J., Zhang Y., Chen G., Li B. (2020). CYP19A1 (Aromatase) Dominates Female Gonadal Differentiation in Chicken (Gallus gallus) Embryos Sexual Differentiation. Biosci. Rep. 40, 1–10. doi:10.1042/BSR20201576
Johnson A. L., Bridgham J. T., Jensen T. (1999). Bcl-Xlong Protein Expression and Phosphorylation in Granulosa Cells1. Endocrinology 140, 4521–4529. doi:10.1210/endo.140.10.7022
Johnson A. L., Bridgham J. T. (2001). Regulation of Steroidogenic Acute Regulatory Protein and Luteinizing Hormone Receptor Messenger Ribonucleic Acid in Hen Granulosa Cells*. Endocrinology 142, 3116–3124. doi:10.1210/endo.142.7.8240
Johnson A. L., Bridgham J. T., Witty J. P., Tilly J. L. (1996). Susceptibility of Avian Ovarian Granulosa Cells to Apoptosis Is Dependent upon Stage of Follicle Development and Is Related to Endogenous Levels of Bcl-Xlong Gene Expression. Endocrinology 137, 2059–2066. doi:10.1210/endo.137.5.8612548
Johnson A. L. (2003). Intracellular Mechanisms Regulating Cell Survival in Ovarian Follicles. Animal Reproduction Sci. 78, 185–201. doi:10.1016/S0378-4320(03)00090-3
Johnson A. L. (1993). Regulation of Follicle Differentiation by Gonadotropins and Growth Factors. Poult. Sci. 72, 867–873. doi:10.3382/ps.0720867
Johnson A. L., van Tienhoven A. (1981). Hypothalamo-Hypophyseal Sensitivity to Hormones in the Hen. II. Plasma Concentrations of LH, Progesterone, and Testosterone in Response to Peripheral and Central Injections of LHRH or Testosterone. Biol. Reproduction 25, 153–161. doi:10.1095/biolreprod25.1.153
Johnson A. L., van Tienhoven A. (1980). Plasma Concentrations of Six Steroids and LH during the Ovulatory Cycle of the Hen, Gallus domesticus1. Biol. Reproduction 23, 386–393. doi:10.1095/biolreprod23.2.386
Johnson P. A., Dickerman R. W., Bahr J. M. (1986). Decreased Granulosa Cell Luteinizing Hormone Sensitivity and Altered Thecal Estradiol Concentration in the Aged Hen, Gallus Domesticus1. Biol. Reproduction 35, 641–646. doi:10.1095/biolreprod35.3.641
Joyner C. J., Peddie M. J., Taylor T. G. (1987). The Effect of Age on Egg Production in the Domestic Hen. General Comp. Endocrinol. 65, 331–336. doi:10.1016/0016-6480(87)90117-1
Kagami H., Hanada H. (1997). Current Knowledge of Sexual Differentiation in Domestic Fowl. World's Poult. Sci. J. 53, 111–123. doi:10.1079/wps19970012
Kamiyoshi M., Niwa T., Tanaka K. (1986). Nuclear Estrogen Receptor Bindings in Granulosa Cells and Estradiol-17β Contents in Follicular Membranes of the Ovary of the Hen during the Ovulatory Cycle. General Comp. Endocrinol. 61, 428–435. doi:10.1016/0016-6480(86)90229-7
Kanatani M., Sugimoto T., Takahashi Y., Kaji H., Kitazawa R., Chihara K. (1998). Estrogen via the Estrogen Receptor Blocks cAMP-Mediated Parathyroid Hormone (PTH)-stimulated Osteoclast Formation. J. Bone Min. Res. 13, 854–862. doi:10.1359/jbmr.1998.13.5.854
Kebreab E., France J., Kwakkel R. P., Leeson S., Kuhi H. D., Dijkstra J. (2009). Development and Evaluation of a Dynamic Model of Calcium and Phosphorus Flows in Layers. Poult. Sci. 88, 680–689. doi:10.3382/ps.2008-00157
Kerschnitzki M., Zander T., Zaslansky P., Fratzl P., Shahar R., Wagermaier W. (2014). Rapid Alterations of Avian Medullary Bone Material during the Daily Egg-Laying Cycle. Bone 69, 109–117. doi:10.1016/j.bone.2014.08.019
Keshavarz K. (1998). Investigation on the Possibility of Reducing Protein, Phosphorus, and Calcium Requirements of Laying Hens by Manipulation of Time of Access to These Nutrients. Poult. Sci. 77, 1320–1332. doi:10.1093/ps/77.9.1320
Kirchgessner T. G., Heinzmann C., Svenson K. L., Gordon D. A., Nicosia M., Lebherz H. G., et al. (1987). Regulation of Chicken Apolipoprotein B: Cloning, Tissue Distribution, and Estrogen Induction of mRNA. Gene 59, 241–251. doi:10.1016/0378-1119(87)90332-5
Klassing K. C. (1998a). “Minerals,” in Comparative Avian Nutrition. Editor K. C. Klassing (Oxford and New York: Oxford University Press), 234–276.
Klassing K. C. (1998b). “Vitamins,” in Comparative Avian Nutrition. Editor K. Klassing (Oxford and New York: Oxford University Press), 277–329.
Klimis-Tavantzis D. J., Kris-Etherton P. M., Leach R. M. (1983). The Effect of Dietary Manganese Deficiency on Cholesterol and Lipid Metabolism in the Estrogen-Treated Chicken and the Laying Hen. J. Nutr. 113, 320–327. doi:10.1093/jn/113.2.320
Knight P. G., Gladwell R. T., Cunningham F. J. (1981). Effect of Gonadectomy on the Concentrations of Catecholamines in Discrete Areas of the Diencephalon of the Domestic Fowl. J. Endocrinol. 89, 389–397. doi:10.1677/joe.0.0890389
Knutson J. C., DeLuca H. F. (1974). 25-Hydroxyvitamin D3-24-Hydroxylase. Subcellular Location and Properties. Biochemistry 13, 1543–1548. doi:10.1021/bi00704a034
Kohler P. O., Grimley P. M., O'malley B. W. (1969). Estrogen-Induced Cytodifferentiation of the Ovalbumin-Secreting Glands of the Chick Oviduct. J. Cell Biol. 40, 8–27. doi:10.1083/jcb.40.1.8
Kowalski K. I., Tilly J. L., Johnson A. L. (1991). Cytochrome P450 Side-Chain Cleavage (P450scc) in the Hen Ovary. I. Regulation of P450scc Messenger RNA Levels and Steroidogenesis in Theca Cells of Developing Follicles1. Biol. Reproduction 45, 955–966. doi:10.1095/biolreprod45.6.955
Kraus W. L., Katzenellenbogen B. S. (1993). Regulation of Progesterone Receptor Gene Expression and Growth in the Rat Uterus: Modulation of Estrogen Actions by Progesterone and Sex Steroid Hormone Antagonists. Endocrinology 132, 2371–2379. doi:10.1210/endo.132.6.8504742
Krumins S. A., Roth T. F. (1981). High-affinity Binding of Lower-Density Lipoproteins to Chicken Oocyte Membranes. Biochem. J. 196, 481–488. doi:10.1042/bj1960481
Kudzma D. J., Swaney J. B., Ellis E. N. (1979). Effects of Estrogen Administration on the Lipoproteins and Apoproteins of the Chicken. Biochim. Biophys. Acta 572, 257–268. doi:10.1016/0005-2760(79)90041-9
Kuiper G. G. J. M., Lemmen J. G., Carlsson B., Corton J. C., Safe S. H., van der Saag P. T., et al. (1998). Interaction of Estrogenic Chemicals and Phytoestrogens with Estrogen Receptor β. Endocrinology 139, 4252–4263. doi:10.1210/endo.139.10.6216
Lague P. C., van Tienhoven A., Cunningham F. J. (1975). Concentrations of Estrogens, Progesterone and LH during the Ovulatory Cycle of the Laying Chicken [Gallus domesticus]. Biol. Reproduction 12, 590–598. doi:10.1095/biolreprod12.5.590
Laverty G., Clark N. B. (1989). “The Kidney,” in Vertebrate Endocrinology: Fundamentals and Biomedical Implications. Editors P. K. T. Pang, and M. P. Schreibman (New York, USA: Academic Press), 277–317.
Laziera C. B., Wiktorowicza M., DiMattiaa G. E., Gordonb D. A., Binderb R., Williamsb D. L. (1994). Apolipoprotein (Apo) B and apoII Gene Expression Are Both Estrogen-Responsive in Chick Embryo Liver but Only apoII Is Estrogen-Responsive in Kidney. Mol. Cell. Endocrinol. 106, 187–194. doi:10.1016/0303-7207(94)90202-x
Lee B. K., Kim J. S., Ahn H. J., Hwang J. H., Kim J. M., Lee H. T., et al. (2010). Changes in Hepatic Lipid Parameters and Hepatic Messenger Ribonucleic Acid Expression Following Estradiol Administration in Laying Hens (Gallus domesticus). Poult. Sci. 89, 2660–2667. doi:10.3382/ps.2010-00686
Leveille G. A., O'Hea E. K., Chakrabarty K. (1968). In Vivo lipogenesis in the Domestic Chicken. Exp. Biol. Med. 128, 398–401. doi:10.3181/00379727-128-33022
Li H., Li Y., Yang L., Zhang D., Liu Z., Wang Y., et al. (2020). Identification of a Novel Lipid Metabolism-Associated Hepatic Gene Family Induced by Estrogen via ERα in Chicken (Gallus gallus). Front. Genet. 11, 1–16. doi:10.3389/fgene.2020.00271
Li H., Wang T., Xu C., Wang D., Ren J., Li Y., et al. (2015). Transcriptome Profile of Liver at Different Physiological Stages Reveals Potential Mode for Lipid Metabolism in Laying Hens. BMC Genomics 16, 1–13. doi:10.1186/s12864-015-1943-0
Li J., Leghari I. H., He B., Zeng W., Mi Y., Zhang C. (2014). Estrogen Stimulates Expression of Chicken Hepatic Vitellogenin II and Very Low-Density Apolipoprotein II through ER-α. Theriogenology 82, 517–524. doi:10.1016/j.theriogenology.2014.05.003
Li Q., Zhao X., Wang S., Zhou Z. (2018). Letrozole Induced Low Estrogen Levels Affected the Expressions of Duodenal and Renal Calcium-Processing Gene in Laying Hens. General Comp. Endocrinol. 255, 49–55. doi:10.1016/j.ygcen.2017.10.005
Lin L.-Y., McCormick C. C. (1986). Quantitation of Chick Tissue Zinc-Metallothionein by Gel Electrophoresis and Silver Stain Enhancement. Comp. Biochem. Physiology Part C Comp. Pharmacol. 85, 75–84. doi:10.1016/0742-8413(86)90054-x
Liu D., Veit H. P., Denbow D. M. (2004). Effects of Long-Term Dietary Lipids on Mature Bone Mineral Content, Collagen, Crosslinks, and Prostaglandin E2 Production in Japanese Quail. Poult. Sci. 83, 1876–1883. doi:10.1093/ps/83.11.1876
Liu D., Veit H., Wilson J., Denbow D. (2003). Long-Term Supplementation of Various Dietary Lipids Alters Bone Mineral Content, Mechanical Properties and Histological Characteristics of Japanese Quail. Poult. Sci. 82, 831–839. doi:10.1093/ps/82.5.831
Liu X.-t., Lin X., Mi Y.-l., Zeng W.-d., Zhang C.-q. (2018). Age-related Changes of Yolk Precursor Formation in the Liver of Laying Hens. J. Zhejiang Univ. Sci. B 19, 390–399. doi:10.1631/jzus.B1700054
Lorenz F. W. (1954). Effects of Estrogens on Domestic Fowl and Applications in the Poultry Industry. Vitamins Hormones 12, 235–275. doi:10.1016/s0083-6729(08)61014-6
Lund J., Deluca H. F. (1966). Biologically Active Metabolite of Vitamin D3 from Bone, Liver, and Blood Serum. J. Lipid Res. 7, 739–744. doi:10.1016/s0022-2275(20)38950-1
MacRae H. F., Layne D. S., Common R. H. (1959). Formation of Estrone, Estriol and an Unidentified Steroid from Estradiol in the Laying Hen. Poult. Sci. 38, 684–687. doi:10.3382/ps.0380684
Maddineni S. R., Ocón-Grove O. M., Krzysik-Walker S. M., Hendricks G. L., Ramachandran R. (2008). Gonadotropin-inhibitory Hormone (GnIH) Receptor Gene Is Expressed in the Chicken Ovary: Potential Role of GnIH in Follicular Maturation. Reproduction 135, 267–274. doi:10.1530/rep-07-0369
Mao Y., Wu X., An L., Li X., Li Z., Zhu G. (2018). Tamoxifen Activates Hypothalamic L-Dopa Synthesis to Stimulate Ovarian Estrogen Production in Chicken. Biochem. Biophysical Res. Commun. 496, 1257–1262. doi:10.1016/j.bbrc.2018.01.182
Mathur R. S., Anastassiadis P. A., Common R. H. (1966). Urinary Excretion of Estrone and of 16-Epi-Estriol Plus 17-Epi-Estriol by the Hen ,. Poult. Sci. 45, 946–952. doi:10.3382/ps.0450946
Matsunaga M., Ukena K., Tsutsui K. (2001). Expression and Localization of Cytochrome P450 17α-Hydroxylase/c17,20-Lyase in the Avian Brain. Brain Res. 899, 112–122. doi:10.1016/s0006-8993(01)02217-x
Mayel-Afshar S., Lane S. M., Lawson D. E. (1988). Relationship between the Levels of Calbindin Synthesis and Calbindin mRNA in Chick Intestine. Quantitation of Calbindin mRNA. J. Biol. Chem. 263, 4355–4361. doi:10.1016/s0021-9258(18)68933-1
Mccoy M. A., Reilly G. A. C., Kilpatrick D. J. (1996). Density and Breaking Strength of Bones of Mortalities Among Caged Layers. Res. Veterinary Sci. 60, 185–186. doi:10.1016/s0034-5288(96)90017-x
Merrill L., Chiavacci S. J., Paitz R. T., Benson T. J. (2019). Quantification of 27 Yolk Steroid Hormones in Seven Shrubland Bird Species: Interspecific Patterns of Hormone Deposition and Links to Life History, Development, and Predation Risk. Can. J. Zool. 97, 1–12. doi:10.1139/cjz-2017-0351
Mete A., Giannitti F., Barr B., Woods L., Anderson M. (2013). Causes of Mortality in Backyard Chickens in Northern California: 2007-2011. Avian Dis. 57, 311–315. doi:10.1637/10382-092312-Case.1
Miller S. C., Bowman B. M., Myers R. L. (1984). Morphological and Ultrastructural Aspects of the Activation of Avian Medullary Bone Osteoclasts by Parathyroid Hormone. Anat. Rec. 208, 223–231. doi:10.1002/ar.1092080209
Miller S. C. (1992). “Calcium Homeostasis and Mineral Turnover in the Laying Hen,” in Bone Biology and Skeletal Disorders in Poultry. Editor C. C. Whitehead (Oxfordshire: Carfax Publishing Co.), 103–116.
Miller S. (1977). Osteoclast Cell-Surface Changes during the Egg-Laying Cycle in Japanese Quail. J. Cell Biol. 75, 104–118. doi:10.1083/jcb.75.1.104
Monroe D. G., Berger R. R., Sanders M. M. (2002). Tissue-Protective Effects of Estrogen Involve Regulation of Caspase Gene Expression. Mol. Endocrinol. 16, 1322–1331. doi:10.1210/mend.16.6.0855
Monroe D. G., Jin D. F., Sanders M. M. (2000). Estrogen Opposes the Apoptotic Effects of Bone Morphogenetic Protein 7 on Tissue Remodeling. Mol. Cell Biol. 20, 4626–4634. doi:10.1128/mcb.20.13.4626-4634.2000
Montorzi M., Falchuk K. H., Vallee B. L. (1995). Vitellogenin and Lipovitellin: Zinc Proteins of Xenopus laevis Oocytes. Biochemistry 34, 10851–10858. doi:10.1021/bi00034a018
Munro S. S., Kosin I. L. (1943). Dramatic Response of the Chick Oviduct to Estrogen. Poult. Sci. 22, 330–331. doi:10.3382/ps.0220330
Muramatsu T., Tsuchiya S., Okumura J.-i., Miyoshi S. (1992). Genetic Differences in Steroid-Induced Protein Synthesis In Vivo of the Liver and Magnum in Immature Chicks (Gallus Domesticus). Comp. Biochem. Physiology Part B Comp. Biochem. 102, 905–909. doi:10.1016/0305-0491(92)90100-6
Mizuno S., Nakabayashi O., Kikuchi H., Kikuchi T. (1996). “Expression of Genes Involved in Estrogen Synthesis and Expression of the Estrogen Receptor Gene During Early Gonadal Development of Chickens. Poultry Avian Biol. Rev.,” in Abstracts of the 6th International Symposium on Avian Endocrinology, 306.
Nakabayashi O., Kikuchi H., Kikuchi T., Mizuno S. (1998). Differential Expression of Genes for Aromatase and Estrogen Receptor during the Gonadal Development in Chicken Embryos. J. Endocrinol. 20, 193–202. doi:10.1677/jme.0.0200193
Navickis R. J., Katzenellenbogen B. S., Nalbandov A. V. (1979). Effects of the Sex Steroid Hormones and Vitamin D3 on Calcium-Binding Proteins in the Chick Shell Gland1. Biol. Reproduction 21, 1153–1162. doi:10.1095/biolreprod21.5.1153
Newman S., Leeson S. (1997). Skeletal Integrity in Layers at the Completion of Egg Production. World's Poult. Sci. J. 53, 265–277. doi:10.1079/wps19970021
Nikolay B., Plieschnig J. A., Šubik D., Schneider J. D., Schneider W. J., Hermann M. (2013). A Novel Estrogen-Regulated Avian Apolipoprotein. Biochimie 95, 2445–2453. doi:10.1016/j.biochi.2013.09.005
Nishikimi H., Kansaku N., Saito N., Usami M., Ohno Y., Shimada K. (2000). Sex Differentiation and mRNA Expression of P450c17, P450arom and AMH in Gonads of the Chicken. Mol. Reprod. Dev. 55, 20–30. doi:10.1002/(sici)1098-2795(200001)55:1<20::aid-mrd4>3.0.co;2-e
Nomura O., Nakabayashi O., Nishimori K., Yasue H., Mizuno S. (1999). Expression of Five Steroidogenic Genes Including Aromatase Gene at Early Developmental Stages of Chicken Male and Female Embryos. J. Steroid Biochem. Mol. Biol. 71, 103–109. doi:10.1016/s0960-0760(99)00127-2
NRC (1994). National Research Council. Nutrient Requirements of Poultry. Washington, DC: National Academy Press. 9th rev. e.
Nys Y., Hincke M. T., Garcia-ruiz J. M., Solomon S. (1999). Avian Eggshell Mineralization. Poult. Avian Biol. Rev. 10, 143–166.
Nys Y., le Roy N. (2018). “Calcium Homeostasis and Eggshell Biomineralization in Female Chicken,” in Vitamin D. Fourth Edition(Elsevier), 361–382. doi:10.1016/B978-0-12-809965-0.00022-7
Nys Y., Mayel-Afshar S., Bouillon R., Van Baelen H., Lawson D. E. M. (1989). Increases in Calbindin D 28K mRNA in the Uterus of the Domestic Fowl Induced by Sexual Maturity and Shell Formation. General Comp. Endocrinol. 76, 322–329. doi:10.1016/0016-6480(89)90164-0
Nys Y., N'Guyen T. M., Williams J., Etches R. J. (1986). Blood Levels of Ionized Calcium, Inorganic Phosphorus, 1,25-dihydroxycholecalciferol and Gonadal Hormones in Hens Laying Hard-Shelled or Shell-Less Eggs. J. Endocrinol. 111, 151–157. doi:10.1677/joe.0.1110151
Nys Y. (1993). “Regulation of Plasma 1,25-(OH)2D3, of Osteocalcin and of Intestinal and Uterine Calbindin in Hens,” in Avian Endocrinology. Editor P. J. Sharp (Bristol, UK: Journal of Endocrinology Ltd.), 345–357.
Ohashi T., Kusuhara S. (1993). Immunoelectron Microscopic Detection of Estrogen Target Cells in the Bone Marrow of Estrogen-Treated Male Japanese Quail. Bone Mineral 20, 31–39. doi:10.1016/s0169-6009(08)80035-9
Ohashi T., Kusuhara S., Ishida K. (1987). Effects of Oestrogen and Anti‐oestrogen on the Cells of the Endosteal Surface of Male Japanese Quail. Br. Poult. Sci. 28, 727–732. doi:10.1080/00071668708417008
Ohashi T., Kusuhara S., Ishida K. (1991). Estrogen Target Cells during the Early Stage of Medullary Bone Osteogenesis: Immunohistochemical Detection of Estrogen Receptors in Osteogenic Cells of Estrogen-Treated Male Japanese Quail. Calcif. Tissue Int. 49, 124–127. doi:10.1007/bf02565134
O’Hea E. K., Leveille G. A. (1968). Lipogenesis in Isolated Adipose Tissue of the Domestic Chick (Gallus Domesticus). Comp. Biochem. Physiology 26, 111–120.
Oka T., Schimke R. T. (1969). Interaction of Estrogen and Progesterone in Chick Oviduct Development. J. Cell Biol. 43, 123–137. doi:10.1083/jcb.43.1.123
Olde B., Leeb-Lundberg L. M. F. (2009). GPR30/GPER1: Searching for a Role in Estrogen Physiology. Trends Endocrinol. Metabolism 20, 409–416. doi:10.1016/j.tem.2009.04.006
Ottinger M. A., Pitts S., Abdelnabi M. A. (2001). Steroid Hormones during Embryonic Development in Japanese Quail: Plasma, Gonadal, and Adrenal Levels. Poult. Sci. 80, 795–799. doi:10.1093/ps/80.6.795
Ottinger M. A. (1989). Sexual Differentiation of Neuroendocrine Systems and Behavior ,. Poult. Sci. 68, 979–989. doi:10.3382/ps.0680979
Oursler M. J., Landers J. P., Riggs B. L., Spelsberg T. C. (1993). Oestrogen Effects on Osteoblasts and Osteoclasts. Ann. Med. 25, 361–371. doi:10.3109/07853899309147298
Oursler M. J., Osdoby P., Pyfferoen J., Riggs B. L., Spelsberg T. C. (1991). Avian Osteoclasts as Estrogen Target Cells. Proc. Natl. Acad. Sci. U.S.A. 88, 6613–6617. doi:10.1073/pnas.88.15.6613
Paitz R. T., Angles R., Cagney E. (2020). In Ovo Metabolism of Estradiol to Estrone Sulfate in Chicken Eggs: Implications for How Yolk Estradiol Influences Embryonic Development. General Comp. Endocrinol. 287, 113320–113326. doi:10.1016/j.ygcen.2019.113320
Palmiter R. D., Wrenn J. T. (1971). Interaction of Estrogen and Progesterone in Chick Oviduct Development. J. Cell Biol. 50, 598–615. doi:10.1083/jcb.50.3.598
Pardee P. E., Dunn W., Schwimmer J. B. (2012). Non-alcoholic Fatty Liver Disease Is Associated with Low Bone Mineral Density in Obese Children. Alimentary Pharmacol. Ther. 35, 248–254. doi:10.1111/j.1365-2036.2011.04924.x
Pearson A. W., Butler E. J. (1978a). Environmental Temperature as a Factor in the Aetiology of Fatty Liver-Haemorrhagic Syndrome in the Fowl. Res. Veterinary Sci. 25, 133–138. doi:10.1016/s0034-5288(18)32967-9
Pearson A. W., Butler E. J. (1978b). The Oestrogenised Chick as an Experimental Model for Fatty Liver-Haemorrhagic Syndrome in the Fowl. Res. Veterinary Sci. 24, 82–86. doi:10.1016/s0034-5288(18)33103-5
Pechak D. G., Kujawa M. J., Caplan A. I. (1986a). Morphological and Histochemical Events during First Bone Formation in Embryonic Chick Limbs. Bone 7, 441–458. doi:10.1016/8756-3282(86)90004-9
Pechak D. G., Kujawa M. J., Caplan A. I. (1986b). Morphology of Bone Development and Bone Remodeling in Embryonic Chick Limbs. Bone 7, 459–472. doi:10.1016/8756-3282(86)90005-0
Perry M. M., Gilbert A. B. (1979). Yolk Transport in the Ovarian Follicle of the Hen (Gallus Domesticus): Lipoprotein-like Particles at the Periphery of the Oocyte in the Rapid Growth Phase. J. Cell Sci. 39, 257–272. doi:10.1242/jcs.39.1.257
Petrik M. T., Guerin M. T., Widowski T. M. (2015). On-farm Comparison of Keel Fracture Prevalence and Other Welfare Indicators in Conventional Cage and Floor-Housed Laying Hens in Ontario, Canada. Poult. Sci. 94, 579–585. doi:10.3382/ps/pev039
Petzinger C., Bauer J. E. (2013). Dietary Considerations for Atherosclerosis in Common Companion Avian Species. J. Exot. Pet Med. 22, 358–365. doi:10.1053/j.jepm.2013.10.013
Pfeiffer C. A., Gardner W. U. (1938). Skeletal Changes and Blood Serum Calcium Level in Pigeons Receiving Estrogens. Endocrinology 23, 485–491. doi:10.1210/endo-23-4-485
Plieschnig J. A., Gensberger E. T., Bajari T. M., Schneider W. J., Hermann M. (2012). Renal LRP2 Expression in Man and Chicken Is Estrogen-Responsive. Gene 508, 49–59. doi:10.1016/j.gene.2012.07.041
Polin D., Wolford J. H. (1973). Factors Influencing Food Intake and Caloric Balance in Chickens. Fed. Proc. 32, 1720–1726.
Polin D., Wolford J. H. (1977). Role of Estrogen as a Cause of Fatty Liver Hemorrhagic Syndrome. J. Nutr. 107, 873–886. doi:10.1093/jn/107.5.873
Porter T. E., Hargis B. M., Silsby J. L., Halawani M. E. E. (1989). Differential Steroid Production between Theca Interna and Theca Externa Cells: A Three-Cell Model for Follicular Steroidogenesis in Avian Species*. Endocrinology 125, 109–116. doi:10.1210/endo-125-1-109
Price J. S., Russell R. G. G. (1992). “Bone Remodeling: Regulation by Systemic and Local Factors,” in Bone Biology and Skeletal Disorders in Poultry. Editor C. C. Whitehead (Oxfordshire, UK: Carfax Publishing Co.), 39–60.
Ratna W. N., Bhatt V. D., Chaudhary K., Ariff A. B., Bavadekar S. A., Ratna H. N. (2016). Estrogen-responsive Genes Encoding Egg Yolk Proteins Vitellogenin and Apolipoprotein II in Chicken Are Differentially Regulated by Selective Estrogen Receptor Modulators. Theriogenology 85, 376–383. doi:10.1016/j.theriogenology.2015.08.015
Ratnasabapathy R. (1995). In Vitro characterization of an Estrogen-Regulated mRNA Stabilizing Activity in the Avian Liver. Cell Mol. Biol. Res. 41, 583–594.
Ratnasabapathy R., Tom M., Post C. (1997). Modulation of the Hepatic Expression of the Estrogen-Regulated mRNA Stabilizing Factor by Estrogenic and Antiestrogenic Nonsteroidal Xenobiotics. Biochem. Pharmacol. 53, 1425–1434. doi:10.1016/s0006-2952(97)00084-1
Raud H. R., Hobkirk R. (1968). In Vitro metabolism of estrone-4-14C and Estrone-6,7-3h-3-Sulfate by Laying Hen Liver Homogenates. Can. J. Biochem. 46, 759–764. doi:10.1139/o68-116
Ren J., Tian W., Jiang K., Wang Z., Wang D., Li Z., et al. (2021). Global Investigation of Estrogen-Responsive Genes Regulating Lipid Metabolism in the Liver of Laying Hens. BMC Genomics 22, 1–14. doi:10.1186/s12864-021-07679-y
Richards G. J., Wilkins L. J., Knowles T. G., Booth F., Toscano M. J., Nicol C. J., et al. (2012). Pop Hole Use by Hens with Different Keel Fracture Status Monitored throughout the Laying Period. Veterinary Rec. 170, 494. doi:10.1136/vr.100489
Richards M., Packard M. (1996). Mineral Metabolism in Avian Embryos. Avian Poult. Biol. Rev. 7, 143–161.
Ringer R., Sheppard C. (1963). Report of Fatty-Liver Syndrome in a Michigan Caged Layer Operation. Q. Bull. Mich. State Univ. Agric. Exp. Stn. 45, 426–427.
Rissman E. F., Ascenzi M., Johnson P., Adkins-Regan E. (1984). Effect of Embryonic Treatment with Oestradiol Benzoate on Reproductive Morphology, Ovulation and Oviposition and Plasma LH Concentrations in Female Quail (Coturnix coturnix Japonica). Reproduction 71, 411–417. doi:10.1530/jrf.0.0710411
Robinson F. E., Etches R. J., Anderson-Langmuir C. E., Burke W. H., Cheng K.-W., Cunningham F. J., et al. (1988). Steroidogenic Relationships of Gonadotrophin Hormones in the Ovary of the Hen (Gallus domesticus). General Comp. Endocrinol. 69, 455–466. doi:10.1016/0016-6480(88)90038-x
Robinson F. E., Etches R. J. (1986). Ovarian Steroidogenesis during Foillicular Maturation in the Domestic Fowl (Gallus Domesticus)1. Biol. Reprod. 35, 1096–1105. doi:10.1095/biolreprod35.5.1096
Roland D. A. (1979). Factors Influencing Shell Quality of Aging Hens. Poult. Sci. 58, 774–777. doi:10.3382/ps.0580774
Roodman G. D. (1999). Cell Biology of the Osteoclast. Exp. Hematol. 27, 1229–1241. doi:10.1016/s0301-472x(99)00061-2
Rosati L., Falvo S., Chieffi Baccari G., Santillo A., di Fiore M. M. (2021). The Aromatase-Estrogen System in the Testes of Non-mammalian Vertebrates. Animals 11, 1–12. doi:10.3390/ani11061763
Rosen C. J., Bouxsein M. L. (2006). Mechanisms of Disease: Is Osteoporosis the Obesity of Bone? Nat. Rev. Rheumatol. 2, 35–43. doi:10.1038/ncprheum0070
Rosol T. J., Chew D. J., Nagode L. A. (2000). “Disorders of Calcium: Hypercalcemia and Hypocalcaemia,” in Fluid Therapy in Small Animal Clinical Practice. Editor S. P. DiBartola (Philadelphia, USA: WB Saunders), 108–161.
Rozenboim I., Mahato J., Cohen N. A., Tirosh O. (2016). Low Protein and High-Energy Diet: A Possible Natural Cause of Fatty Liver Hemorrhagic Syndrome in Caged White Leghorn Laying Hens. Poult. Sci. 95, 612–621. doi:10.3382/ps/pev367
Rufener C., Makagon M. M. (2020). Keel Bone Fractures in Laying Hens: A Systematic Review of Prevalence across Age, Housing Systems, and Strains. J. Animal Sci. 98, S36–S51. doi:10.1093/JAS/SKAA145
Rząsa J., Sechman A., Paczoska-Eliasiewicz H., Hrabia A. (2009). Effect of Tamoxifen on Sex Steroid Concentrations in Chicken Ovarian Follicles. Acta Veterinaria Hung. 57, 85–97. doi:10.1556/AVet.57.2009.1.9
Sato K., Abe H., Kono T., Yamazaki M., Nakashima K., Kamada T., et al. (2009). Changes in Peroxisome Proliferator-Activated Receptor Gamma Gene Expression of Chicken Abdominal Adipose Tissue with Different Age, Sex and Genotype. Animal Sci. J. 80, 322–327. doi:10.1111/j.1740-0929.2009.00639.x
Scheele C. W. (1997). Pathological Changes in Metabolism of Poultry Related to Increasing Production Levels. Veterinary Q. 19, 127–130. doi:10.1080/01652176.1997.9694756
Scheib D. (1983). Effects and Role of Estrogens in Avian Gonadal Differentiation. Differentiation 1, 87–92. doi:10.1007/978-3-642-69150-8_15
Schexnailder R., Griffith M. (1973). Liver Fat and Egg Production of Laying Hens as Influenced by Choline and Other Nutrients. Poult. Sci. 52, 1188–1194. doi:10.3382/ps.0521188
Schimke R. T., McKnight G. S., Shapiro D. J., Sullivan D., Palacios R. (1975). Hormonal Regulation of Ovalbumin Synthesis in the Chick Oviduct. Proc. 1974 Laurent. Hormone Conf. 1, 175–211. doi:10.1016/b978-0-12-571131-9.50009-8
Schneider W., Carroll R., Severson D., Nimpf J. (1990). Apolipoprotein VLDL-II Inhibits Lipolysis of Triglyceride-Rich Lipoproteins in the Laying Hen. J. Lipid Res. 31, 507–513. doi:10.1016/s0022-2275(20)43172-4
Schumacher M., Sulon J., Balthazart J. (1988). Changes in Serum Concentrations of Steroids during Embryonic and Post-hatching Development of Male and Female Japanese Quail (Coturnix coturnix Japonica). J. Endocr. 118, 127–134. doi:10.1677/joe.0.1180127
Schwabl H. (1993). Yolk Is a Source of Maternal Testosterone for Developing Birds. Proc. Natl. Acad. Sci. U.S.A. 90, 11446–11450. doi:10.1073/pnas.90.24.11446
Sechman A., Paczoska-Eliasiewicz H., Rzasa J., Hrabia A. (2000). Simultaneous Determination of Plasma Ovarian and Thyroid Hormones during Sexual Maturation of the Hen (Gallus domesticus). Folia Biol. (Krakow) 48, 7–12.
Sechman A., Hrabia A., Lis M. W., Niedziółka J. (2011). Effect of 2,3,7,8-Tetrachlorodibenzo-P-Dioxin (TCDD) on Steroid Concentrations in Blood and Gonads of Chicken Embryo. Toxicol. Lett. 205, 190–195. doi:10.1016/j.toxlet.2011.06.004
Seibel M. J. (2005). Biochemical Markers of Bone Turnover: Part I: Biochemistry and Variability. Clin. Biochem. Rev. 26, 97–122.
Senior B. E. (1974). Oestradiol Concentration in the Peripheral Plasma of the Domestic Hen from 7 Weeks of Age until the Time of Sexual Maturity. Reproduction 41, 107–112. doi:10.1530/jrf.0.0410107
Sharp P. J., Scanes C. G., Williams J. B., Harvey S., Chadwick A. (1979). Variations in Concentrations of Prolactin, Luteinizing Hormone, Growth Hormone and Progesterone in the Plasma of Broody Bantams (Gallus domesticus). J. Endocrinol. 80, 51–57. doi:10.1677/joe.0.0800051
Sharp P. J. (1996). Strategies in Avian Breeding Cycles. Animal Reproduction Sci. 42, 505–513. doi:10.1016/0378-4320(96)01556-4
Shemesh M., Shore L., Bendheim U., Lavi S., Weisman Y. (1982). Correlation between Serum Estrogen Concentrations, Light and Prolapsed Uterus in Laying Hens. Adv. Pathology 1, 273–276.
Shemesh M., Shore L., Lavi S., Ailenberg M., Bendheim U., Totach A., et al. (1984). The Role of 17β-Estradiol in the Recovery from Oviductal Prolapse in Layers. Poult. Sci. 63, 1638–1643. doi:10.3382/ps.0631638
Shini A., Shini S., Bryden W. L. (2019). Fatty Liver Haemorrhagic Syndrome Occurrence in Laying Hens: Impact of Production System. Avian Pathol. 48, 25–34. doi:10.1080/03079457.2018.1538550
Shini A., Shini S., Filippich L., Anderson S., Bryden W. (2012). Role of Inflammation in the Pathogenesis of Fatty Liver Haemorrhagic Syndrome in Laying Hens. University of Sydney Poultry Foundation: Australian Poultry Science Symposium. 193.
Shini S., Bryden W. (2009). Occurrence and Control of Fatty Liver Haemorrhagic Syndrome (FLHS) in Caged Hens. Sydney: Austrian Egg Corporation, 44–48.
Shini S., Shini A., Bryden W. L. (2020). Unravelling Fatty Liver Haemorrhagic Syndrome: 1. Oestrogen and Inflammation. Avian Pathol. 49, 87–98. doi:10.1080/03079457.2019.1674444
Shini S., Stewart G., Shini A., Bryden W. (2006). “Mortality Rates and Causes of Death in Laying Hens Kept in Cage and Alternative Housing Systems,” in 12th European Poultry Conference. Editor D. Martin (Verona: World Poultry Science Association), 601.
Simkiss K. (1961). Calcium Metabolism and Avian Reproduction. Biol. Rev. 36, 321–359. doi:10.1111/j.1469-185x.1961.tb01292.x
Smith C. A., Andrews J. E., Sinclair A. H. (1997). Gonadal Sex Differentiation in Chicken Embryos: Expression of Estrogen Receptor and Aromatase Genes. J. Steroid Biochem. Mol. Biol. 60, 295–302. doi:10.1016/s0960-0760(96)00196-3
Smith C. A., Roeszler K. N., Bowles J., Koopman P., Sinclair A. H. (2008). Onset of Meiosis in the Chicken Embryo; Evidence of a Role for Retinoic Acid. BMC Dev. Biol. 8, 85–19. doi:10.1186/1471-213X-8-85
Speake B. K., Murray A. M. B., C. Noble R. (1998). Transport and Transformations of Yolk Lipids during Development of the Avian Embryo. Prog. Lipid Res. 37, 1–32. doi:10.1016/s0163-7827(97)00012-x
Squires E. J., Leeson S. (1988). Aetiology of Fatty Liver Syndrome in Laying Hens. Br. Veterinary J. 144, 602–609. doi:10.1016/0007-1935(88)90031-0
Stake P. E., Fredrickson T. N., Bourdeau C. A. (1981). Induction of Fatty Liver-Hemorrhagic Syndrome in Laying Hens by Exogenous B-Estradiol. Avian Dis. 25, 410–422. doi:10.2307/1589933
Striem S., Bar A. (1991). Modulation of Quail Intestinal and Egg Shell Gland Calbindin (Mr 28,000) Gene Expression by Vitamin D3, 1,25-dihydroxyvitamin D3 and Egg Laying. Mol. Cell. Endocrinol. 75, 169–177. doi:10.1016/0303-7207(91)90232-H
Suda T., Kobayashi K., Jimi E., Udagawa N., Takahashi N. (2001). The Molecular Basis of Osteoclast Differentiation and Activation. Novartis Found. Symp. 232, 235–250. doi:10.1002/0470846658.ch16
Sugiyama T., Kusuhara S. (1994). Efeect of Parathyroid Hormone on Osteoclasts in Organ-Cultured Medullary Bone. Jpn. Poult. Sci. 31, 392–399. doi:10.2141/jpsa.31.392
Sugiyama T., Kusuhara S. (1996). “Morphological Changes of Osteoclasts on Hen Medullary Bone during the Egg-Laying Cycle and Their Regulation,” in Comparative Endocrinology of Calcium Regulating Hormones. Editors C. G. Dacke, J. Danks, G. Flik, and I. Caple (Bristol, UK: Journal of Endocrinology Ltd), 149–160.
Sugiyama T., Kusuhara S. (1993). Ultrastructural Changes of Osteoclasts on Hen Medullary Bone during the Egg‐laying Cycle. Br. Poult. Sci. 34, 471–477. doi:10.1080/00071669308417602
Takeshima K., Zuidhof M., Hanlon C., Bédécarrats G. (2019). “Differential Effects of Constant Supplemental Light Wavelength on Plasma Estradiol and Melatonin Levels, Body Weight, and Egg Production in Female Broiler Breeders,” in Poult. Sci. (Montreal, Canada, 261. 98.E-suppl. 1
Tan W., Zheng H., Wang D., Tian F., Li H., Liu X. (2020). Expression Characteristics and Regulatory Mechanism of Apela Gene in Liver of Chicken (Gallus gallus). PLoS ONE 15, e0238784–16. doi:10.1371/journal.pone.0238784
Tanabe Y., Nakamura T., Fujioka K., Doi O. (1979). Production and Secretion of Sex Steroid Hormones by the Testes, the Ovary, and the Adrenal Glands of Embryonic and Young Chickens (Gallus domesticus). General Comp. Endocrinol. 39, 26–33. doi:10.1016/0016-6480(79)90189-8
Tanabe Y., Nakamura T., Tanase H., Doi O. (1981). Comparisons of Plasma LH, Progesterone, Testosterone and Estradiol Concentrations in Male and Female Chickens (Gallus domesticus) from 28 to 1141 Days of Age. Endocrinol. Jpn. 28, 605–613. doi:10.1507/endocrj1954.28.605
Tarlow D. M., Watkins P. A., Reed R. E., Miller R. S., Zwergel E. E., Lane M. D. (1977). Lipogenesis and the Synthesis and Secretion of Very Low Density Lipoprotein by Avian Liver Cells in Nonproliferating Monolayer Culture. Hormonal Effects. J. Cell Biol. 73, 332–353. doi:10.1083/jcb.73.2.332
Tarugi P., Ballarini G., Pinotti B., Franchini A., Ottaviani E., Calandra S. (1998). Secretion of apoB- and apoA-I-Containing Lipoproteins by Chick Kidney. J. Lipid Res. 39, 731–743. doi:10.1016/s0022-2275(20)32562-1
Terada O., Shimada K., Saito N. (1997). Effect of Oestradiol Replacement in Ovariectomized Chickens on Pituitary LH Concentrations and Concentrations of mRNAs Encoding LH and Subunits. Reproduction 111, 59–64. doi:10.1530/jrf.0.1110059
Theofan G., Nguyen A. P., Norman A. W. (1986). Regulation of Calbindin-D28k Gene Expression by 1,25-dihydroxyvitamin D3 Is Correlated to Receptor Occupancy. J. Biol. Chem. 261, 16943–16947. doi:10.1016/s0021-9258(19)75981-x
Thomas P., Pang Y., Filardo E. J., Dong J. (2005). Identity of an Estrogen Membrane Receptor Coupled to a G Protein in Human Breast Cancer Cells. Endocrinology 146, 624–632. doi:10.1210/en.2004-1064
Tilly J. L., Kowalski K. I., Johnson A. L. (1991). Cytochrome P450 Side-Chain Cleavage (P450scc) in the Hen Ovary. II. P450scc Messenger RNA, Immunoreactive Protein, and Enzyme Activity in Developing Granulosa Cells1. Biol. Reprod. 45, 967–974. doi:10.1095/biolreprod45.6.967
Tomkinson A., Gevers E. F., Wit J. M., Reeve J., Noble B. S. (1998). The Role of Estrogen in the Control of Rat Osteocyte Apoptosis. J. Bone Min. Res. 13, 1243–1250. doi:10.1359/jbmr.1998.13.8.1243
Trott K. A., Giannitti F., Rimoldi G., Hill A., Woods L., Barr B., et al. (2014). Fatty Liver Hemorrhagic Syndrome in the Backyard Chicken. Vet. Pathol. 51, 787–795. doi:10.1177/0300985813503569
Tsang C. P. W., Grunder A. A. (1984). Production, Clearance Rates and Metabolic Fate of Estradiol-17β in the Plasma of the Laying Hen. Steroids 43, 71–84. doi:10.1016/0039-128X(84)90059-X
Tsutsui K., Saigoh E., Ukena K., Teranishi H., Fujisawa Y., Kikuchi M., et al. (2000). A Novel Avian Hypothalamic Peptide Inhibiting Gonadotropin Release. Biochem. Biophysical Res. Commun. 275, 661–667. doi:10.1006/bbrc.2000.3350
Tuan R. S., Scott W. A. (1977). Calcium-binding Protein of Chorioallantoic Membrane: Identification and Development Expression. Proc. Natl. Acad. Sci. U.S.A. 74, 1946–1949. doi:10.1073/pnas.74.5.1946
Turner B. J., Debeer M. (2009). Calcium Tetany in Broiler Breeder Hens: Update. Aviagen Brief. 1, 1–5.
Turner R. T., Riggs B. L., Spelsberg T. C. (1994). Skeletal Effects of Estrogen. Endocr. Rev. 15, 275–300. doi:10.1210/edrv-15-3-275
Turner R. T., Bell N. H., Gay C. V. (1993). Evidence that Estrogen Binding Sites Are Present in Bone Cells and Mediate Medullary Bone Formation in Japanese Quail. Poult. Sci. 72, 728–740. doi:10.3382/ps.0720728
Ubuka T., Ukena K., Sharp P. J., Bentley G. E., Tsutsui K. (2006). Gonadotropin-Inhibitory Hormone Inhibits Gonadal Development and Maintenance by Decreasing Gonadotropin Synthesis and Release in Male Quail. Endocrinology 147, 1187–1194. doi:10.1210/en.2005-1178
Ugar D., Karaca I. (1999). Serum Osteocalcin as a Biochemical Bone Formation Marker. Biochem. Arch. 15, 379–382.
Urist M. R., Deutsch N. M. (1960). Osteoporosis in the Laying Hen1. Endocrinology 66, 377–391. doi:10.1210/endo-66-3-377
Valinietse M. I. U., Bauman V. K. (1981). Comparative Antirachitic Activity of Vitamins D2 and D3 in the Body of Chicks. Prikl. Biokhim Mikrobiol. 17, 712–719.
van de Velde J. P., Vermeiden J. P. W., Touw J. J. A., Veldhuijzen J. P. (1984). Changes in Activity of Chicken Medullary Bone Cell Populations in Relation to the Egg-Laying Cycle. Metabolic Bone Dis. Relat. Res. 5, 191–193. doi:10.1016/0221-8747(84)90029-8
Vivacqua A., de Marco P., Santolla M. F., Cirillo F., Pellegrino M., Panno M. L. (2015). Estrogenic Gper Signaling Regulates Mir144 Expression in Cancer Cells and Cancer-Associated Fibroblasts (Cafs). Oncotarget 6, 16573–16587. doi:10.18632/oncotarget.4117
Walzem R. L. (1996). Lipoproteins and the Laying Hen: Form Follows Function. Poultry Avian Biol. Rev. 7, 31–64.
Walzem R. L., Hansen R. J., Williams D. L., Hamilton R. L. (1999). Estrogen Induction of VLDLy Assembly in Egg-Laying Hens. J. Nutr. 129, 467S–472S. doi:10.1093/jn/129.2.467S
Walzem R. L., Davis P. A., Hansen R. J. (1994). Overfeeding Increases Very Low Density Lipoprotein Diameter and Causes the Appearance of a Unique Lipoprotein Particle in Association with Failed Yolk Deposition. J. Lipid Res. 35, 1354–1366. doi:10.1016/s0022-2275(20)40077-x
Walzem R. L., Simon C., Morishita T., Lowenstine L., Hansen R. J. (1993). Fatty Liver Hemorrhagic Syndrome in Hens Overfed a Purified Diet. Selected Enzyme Activities and Liver Histology in Relation to Liver Hemorrhage and Reproductive Performance ,. Poult. Sci. 72, 1479–1491. doi:10.3382/ps.0721479
Wang Q.-l., Zhang A.-z., Pan X., Chen L.-r. (2010). Simultaneous Determination of Sex Hormones in Egg Products by ZnCl2 Depositing Lipid, Solid-phase Extraction and Ultra Performance Liquid Chromatography/electrospray Ionization Tandem Mass Spectrometry. Anal. Chim. Acta 678, 108–116. doi:10.1016/j.aca.2010.08.014
Wang X., Yang L., Wang H., Shao F., Yu J., Jiang H., et al. (2014). Growth Hormone-Regulated mRNAs and miRNAs in Chicken Hepatocytes. PLoS ONE 9, e112896–15. doi:10.1371/journal.pone.0112896
Wang Y., Wang J., Shi Y., Ye H., Luo W., Geng F. (2022). Quantitative Proteomic Analyses during Formation of Chicken Egg Yolk. Food Chem. 374, 1–11. doi:10.1016/j.foodchem.2021.131828
Watkins B. A., Shen C.-L., Mcmurtry J. P., Xu H., Bain S. D., Allen K. G. D., et al. (1997). Dietary Lipids Modulate Bone Prostaglandin E2 Production, Insulin-like Growth Factor-I Concentration and Formation Rate in Chicks. Biochem. Mol. Roles Nutrients Diet. Lipids Modul. Bone Prostaglandin E 2 Prod. Nutr 127, 1084–1091. doi:10.1093/jn/127.6.1084
Webster A. B. (2004). Welfare Implications of Avian Osteoporosis. Poult. Sci. 83, 184–192. doi:10.1093/ps/83.2.184
Whitehead C. C., Fleming R. H. (2000). Osteoporosis in Cage Layers. Poult. Sci. 79, 1033–1041. doi:10.1093/ps/79.7.1033
Whitehead C. C. (2004). Overview of Bone Biology in the Egg-Laying Hen. Poult. Sci. 83, 193–199. doi:10.1093/ps/83.2.193
Whitehead C. C., Wilson S. (1992). “Characteristics of Osteopenia in Hens,” in Bone Biology and Skeletal Disorders in Poultry. Editor C. C. Whitehead (Oxfordshire, UK: Carfax Publishing Co.), 265–280.
Wilkins L. J., McKinstry J. L., Avery N. C., Knowles T. G., Brown S. N., Tarlton J., et al. (2011). Influence of Housing System and Design on Bone Strength and Keel Bone Fractures in Laying Hens. Veterinary Rec. 169, 414. doi:10.1136/vr.d4831
Williams J. B., Sharp P. J. (1977). A Comparison of Plasma Progesterone and Luteinizing Hormone in Growing Hens from Eight Weeks of Age to Sexual Maturity. J. Endocrinol. 75, 447–448. doi:10.1677/joe.0.0750447
Wilson S. C., Sharp P. J. (1976). Induction of Luteinizing Hormone Release by Gonadal Steroids in the Ovariectomized Domestic Hen. J. Endocrinol. 71, 87–98. doi:10.1677/joe.0.0710087
Wilson S., Thorp B. H. (1998). Estrogen and Cancellous Bone Loss in the Fowl. Calcif. Tissue Int. 62, 506–511. doi:10.1007/s002239900470
Wiskocil R., Bensky P., Dower W., Goldberger R. F., Gordon J. I., Deeley R. G. (1980). Coordinate Regulation of Two Estrogen-dependent Genes in Avian Liver. Proc. Natl. Acad. Sci. U.S.A. 77, 4474–4478. doi:10.1073/pnas.77.8.4474
Wolff E. (1936). L’action de l’oestrone sur 1’oviducte et la cloaque des poussins femelles, intersexues et males. Compt. Rend. Soc. De. Biol. 123, 235–236.
Wolford J. H., Polin D. (1972). Lipid Accumulation and Hemorrhage in Livers of Laying Chickens. Poult. Sci. 51, 1707–1713. doi:10.3382/ps.0511707
Wolford J. H. (1971). The Effect of Temperature and Iodinated Casein on Liver Lipids of Laying Chickens. Poult. Sci. 50, 1331–1335. doi:10.3382/ps.0501331
Woods J. E., Brazzill D. M. (1981). Plasma 17β-Estradiol Levels in the Chick Embryo. General Comp. Endocrinol. 44, 37–43. doi:10.1016/0016-6480(81)90353-1
Woods J. E., Erton L. H. (1978). The Synthesis of Estrogens in the Gonads of the Chick Embryo. General Comp. Endocrinol. 36, 360–370. doi:10.1016/0016-6480(78)90117-x
Wu X. L., Zou X. Y., Zhang M., Hu H. Q., Wei X. L., Jin M. L., et al. (2021). Osteocalcin Prevents Insulin Resistance, Hepatic Inflammation, and Activates Autophagy Associated with High-Fat Diet-Induced Fatty Liver Hemorrhagic Syndrome in Aged Laying Hens. Poult. Sci. 100, 73–83. doi:10.1016/j.psj.2020.10.022
Yamada M., Chen C., Sugiyama T., Kim W. K. (2021). Effect of Age on Bone Structure Parameters in Laying Hens. Animals 11, 570–578. doi:10.3390/ani11020570
Yang J., Long D. W., Bacon W. L. (1997). Changes in Plasma Concentrations of Luteinizing Hormone, Progesterone, and Testosterone in turkey Hens during the Ovulatory Cycle. General Comp. Endocrinol. 106, 281–292. doi:10.1006/gcen.1997.6884
Yarden N., Lavelin I., Genina O., Hurwitz S., Diaz R., Brown E. M., et al. (2000). Expression of Calcium-Sensing Receptor Gene by Avian Parathyroid Gland In Vivo: Relationship to Plasma Calcium. General Comp. Endocrinol. 117, 173–181. doi:10.1006/gcen.1999.7405
Yilmaz Y. (2012). Review Article: Non-alcoholic Fatty Liver Disease and Osteoporosis - Clinical and Molecular Crosstalk. Aliment. Pharmacol. Ther. 36, 345–352. doi:10.1111/j.1365-2036.2012.05196.x
Yoshida K., Shimada K., Saito N. (1996). Expression of P45017αHydroxylase and P450 Aromatase Genes in the Chicken Gonad before and after Sexual Differentiation. General Comp. Endocrinol. 102, 233–240. doi:10.1006/gcen.1996.0064
Yu J. Y.-L., Marquardt R. R. (1973). Effects of Estradiol and Testosterone on the Immature Female Chicken (Gallus domesticus)-II. Immunodiffusional Patterns of Yolk Lipoproteins in the Liver and Plasma. Comp. Biochem. Physiology Part B Comp. Biochem. 46, 759–767. doi:10.1016/0305-0491(73)90120-x
Zhang D.-D., Wang D.-D., Wang Z., Wang Y.-B., Li G.-X., Sun G.-R., et al. (2020). Estrogen Abolishes the Repression Role of Gga-miR-221-5p Targeting ELOVL6 and SQLE to Promote Lipid Synthesis in Chicken Liver. Ijms 21, 1–18. doi:10.3390/ijms21051624
Zhang M., Xu P., Sun X., Zhang C., Shi X., Li J., et al. (2021). JUN Promotes Chicken Female Differentiation by Inhibiting Smad2. Cytotechnology 73, 101–113. doi:10.1007/s10616-020-00447-y
Zhang S., Shi H., Li H. (2007). Cloning and Tissue Expression Characterization of the ChickenAPOBGene. Anim. Biotechnol. 18, 243–250. doi:10.1080/10495390701574887
Zhang X. Y., Wu M. Q., Wang S. Z., Zhang H., Du Z. Q., Li Y. M., et al. (2018). Genetic Selection on Abdominal Fat Content Alters the Reproductive Performance of Broilers. Animal 12, 1232–1241. doi:10.1017/s1751731117002658
Zhao H., Ge J., Wei J., Liu J., Liu C., Ma C., et al. (2020). Effect of FSH on E2/GPR30-Mediated Mouse Oocyte Maturation In Vitro. Cell. Signal. 66, 1–13. doi:10.1016/j.cellsig.2019.109464
Zhao L.-J., Liu Y.-J., Liu P.-Y., Hamilton J., Recker R. R., Deng H.-W. (2007). Relationship of Obesity with Osteoporosis. J. Clin. Endocrinol. Metabolism 92, 1640–1646. doi:10.1210/jc.2006-0572
Keywords: estradiol (17ß-estradiol), reproduction, egg formation, sexual maturation, laying persistency, medullary bone, yolk deposition
Citation: Hanlon C, Ziezold CJ and Bédécarrats GY (2022) The Diverse Roles of 17β-Estradiol in Non-Gonadal Tissues and Its Consequential Impact on Reproduction in Laying and Broiler Breeder Hens. Front. Physiol. 13:942790. doi: 10.3389/fphys.2022.942790
Received: 13 May 2022; Accepted: 13 June 2022;
Published: 01 July 2022.
Edited by:
Sandra G. Velleman, The Ohio State University, United StatesReviewed by:
Monika Proszkowiec-Weglarz, United States Department of Agriculture, United StatesAndrzej Sechman, University of Agriculture in Krakow, Poland
Copyright © 2022 Hanlon, Ziezold and Bédécarrats. This is an open-access article distributed under the terms of the Creative Commons Attribution License (CC BY). The use, distribution or reproduction in other forums is permitted, provided the original author(s) and the copyright owner(s) are credited and that the original publication in this journal is cited, in accordance with accepted academic practice. No use, distribution or reproduction is permitted which does not comply with these terms.
*Correspondence: Charlene Hanlon, chanlon@uoguelph.ca