- State Key Laboratory of Cardiovascular Disease, Arrhythmia Center, Fuwai Hospital, National Center for Cardiovascular Diseases, Chinese Academy of Medical Sciences and Peking Union Medical College, Beijing, China
Background: Left bundle branch area pacing (LBBAP) has become a safe and effective option for heart failure (HF) patients indicated for cardiac resynchronization therapy (CRT) and/or ventricular pacing, yet the response rate was only 70%. Repolarization parameters were demonstrated to be associated with cardiac mechanics and systolic function. This study aimed to investigate the effects of LBBAP on repolarization parameters and the potential association between those parameters and echocardiographic response.
Methods and results: A total of 59 HF patients undergoing successful LBBAP were consecutively included. QTc, Tpeak-Tend (TpTe), and TpTe/QTc were measured before and after the implantation. The results turned out that the dispersion of ventricular repolarization (DVR) improved after LBBAP among the total population. Although trends of repolarization parameters varied according to different QRS configurations at baseline, the post-implant parameters showed no significant difference between groups. The association between repolarization parameters and LBBAP response was then evaluated among patients with wide QRS. Multivariate analysis demonstrated that post-implant TpTe was the independent predictor of LBBAP response (p < 0.05). Receiver operating characteristic analysis indicated an area under the curve of 0.77 (95% CI, 0.60–0.93) with a cutoff value of 81.2 ms (p < 0.01). Patients with post-implant TpTe<81.2 ms had a significantly higher rate of echocardiographic response (93.3 vs. 44.4%, p < 0.01). Further subgroup analysis indicated that the predictive value of post-implant TpTe for LBBAP response was more significant in non-left bundle branch block (LBBB) patients than in LBBB patients.
Conclusion: LBBAP improved DVR significantly in HF patients. Post-implant TpTe was associated with the echocardiographic response after LBBAP among patients with wide QRS, especially for non-LBBB patients.
Introduction
Cardiac resynchronization therapy (CRT) is an effective treatment for heart failure (HF) patients with cardiac dyssynchrony or indicated for ventricular pacing (Epstein et al., 2013). Recently, left bundle branch area pacing (LBBAP) has been reported as a safe and effective option for CRT delivery (Vijayaraman et al., 2021), which achieved better electrical synchrony and even more promising echocardiographic and clinical outcomes than traditional biventricular pacing (BiVP) (Li et al., 2020; Strocchi et al., 2020). However, a large proportion of patients still showed LBBAP non-response, up to around 30% (Vijayaraman et al., 2021). Data on predictors for LBBAP response or non-response are quite limited to date.
Ventricular repolarization was demonstrated to be significantly associated with myocardial mechanics and systolic function in the last decade (Sauer et al., 2012; Sauer et al., 2014; Prenner et al., 2016). Indices of dispersion of ventricular repolarization (DVR) were even reported to be correlated with BiVP response in HF patients. For example, Yu et al. found that baseline Tpeak-Tend (TpTe)/QTc could predict CRT non-response among BiVP patients (Yu et al., 2017). The relationship between repolarization parameters and LBBAP response has never been studied yet.
The current study aimed to evaluate the changes in repolarization parameters after LBBAP, and further determine the potential association between baseline and post-implant repolarization parameters and echocardiographic response.
Methods
Study population
HF patients undergoing successful LBBAP implantation at our center from December 2017 to February 2022 were consecutively and retrospectively enrolled in the study. The inclusion criteria were as follows: 1) New York Heart Association II-IV despite optimal guideline-based medication for at least 3 months; 2) left ventricular ejection fraction (LVEF) < 50%; 3) indications for CRT implantation and/or ventricular pacing. All patients had written informed consents for the operation and clinical data use. This study was approved by the hospital Institutional Review Board.
Procedure details
Procedure details of LBBAP were elaborated on in previous studies (Chen et al., 2019; Li et al., 2019; Lin et al., 2021). Briefly, the Select Secure pacing lead (Model 3830 69 cm, Medtronic Inc., Minneapolis, MN, United States) was screwed perpendicularly into the ventricular septum towards the left bundle branch (LBB) area under fluoroscopic RAO30° through a fixed-curve sheath (C315 HIS, Medtronic Inc., Minneapolis, MN, United States). During the whole process, unipolar-tip paced QRS configuration and pacing impedance were monitored closely along with measurement of stimulus to peak left ventricular activation time (stim-LVAT). The criteria for LBB area capture were shown below (Lin et al., 2020; Vijayaraman et al., 2021). The unipolar-tip paced QRS showed right bundle branch block (RBBB) configuration or correction of left bundle branch block (LBBB) along with at least one of the following findings:1) transition from non-selective LBB capture to selective LBB capture as the output decreased at the same site; 2) transition from non-selective LBB capture to left ventricular (LV) septal capture as the output decreased at the same site (prolongation of stim-LVAT ≥ 10 ms); 3) short and constant stim-LVAT despite the change of output (stim-LVAT ≤ 90 ms).
Measurement of electrocardiogram (ECG) parameters
ECGs under intrinsic rhythm and ventricular-paced rhythm around 24 h after the implantation were recorded, respectively, for each patient. Trained assessor masked to treatment allocation did all the ECG metrics. QRS duration (QRSd) was measured from the earliest onset throughout all leads to the latest offset. QT interval and TpTe interval were measured in V5 lead for three beats during sinus rhythm and five beats during atrial fibrillation (Af), then the average was taken (Antzelevitch et al., 2007; Tooley et al., 2019). QT interval was measured from the onset of the QRS complex to the end of the T-wave, defined as the point at which a tangent to the maximal downslope of the descending limb of the T-wave crossed the isoelectric baseline (Castro Hevia et al., 2006). QTc was the correction the of QT interval for HR and the Bazett formula was used (Lee et al., 2014; Cho et al., 2016; Vandenberk et al., 2016). TpTe was the difference between QT interval and QTpeak (distance from the onset of QRS complex to the peak of T wave). QTpeak was measured to the nadir the of T wave the if T wave was negative or biphasic (Emori and Antzelevitch, 2001; Antzelevitch et al., 2007; Itoh et al., 2013). Specifically, TpTe in the case of a negative–positive T wave was the interval between the nadir of the initial negative T wave and the end of the T wave.
Echocardiography
Transthoracic 2-dimensional echocardiography was performed within 1 week before CRT implantation and at least 3 months after implantation. LVEF was quantified using the biplane Simpson method, and LVEF and left ventricular end-diastolic diameter (LVEDD) were recorded. A positive echocardiographic response was defined as a ≥5% increase in LVEF between baseline and follow-up echocardiography (Vijayaraman et al., 2021). Super-response was achieved when LVEF increased by at least 20% or improved to >50% in patients with baseline LVEF ≤35% (Ellenbogen and Huizar, 2012).
Statistics
All statistical analyses were performed with SPSS software 25.0. The continuous variables, expressed as mean ± standard deviation (normal distribution) or median (interquartile range) (non-normal distribution), were analyzed with a student’s t-test or non-parametric test (Mann–Whitney test or Wilcoxon signed rank test) between two groups while with one-way ANOVA or Kruskal–Wallis test between three groups. Shapiro–Wilk was used for the normality test. Categorical variables were presented as numbers and percentages, and statistical significance was assessed by the Chi-square test or Fisher’s exact test. Stepwise multivariate logistic regression analysis was used to identify the independent predictors for LBBAP response (univariate factors presenting p < 0.15 were included). The optimal cut-off point for response predictor was obtained by receiver operating characteristic (ROC) curve analysis based on maximal Youden index (sensitivity—[1—specificity)). All tests were two-tailed, and a p value <0.05 was considered statistically significant.
Results
Baseline and procedure characteristics
A total of 59 HF patients with a mean LVEF of 33.7 ± 6.7% were included in the study. The median age of the patients was 64.0 years (67.8% men). Ischemia cardiomyopathy and Af were observed in 15.3% and 25.4% of the patients, respectively. 81.4% of the patients had QRS ≥130 ms [(54.2% had LBBB, 13.6% had intraventricular conduction defects, and 13.6% had prior right ventricular paced (RVP) rhythm], while the remaining 18.6% of patients had narrow QRS. (Table 1).
Procedure-related characteristics were recorded in Table 2. All patients achieved favorable pacing parameters during the procedure. After a median follow-up period of 4 months (range 3.0–8.0 months), R wave amplitude remained stable while pacing threshold increased slightly from 0.5 (0.5, 0.5) to 0.5 (0.5, 0.8) v/0.4 ms (p < 0.01) and pacing impedance decreased from 516.9 ± 133.8 to 456.4 ± 81.8 Ω (p < 0.001). The pacing percentage was 99.8 (97.2, 100) % among the population.
Changes in ECG parameters after the implantation
Biphasic T wave with the positive component following the negative component was observed in 12 (20.3%) patients. For the entire study population, QRSd significantly decreased after the implantation [121.3 (113.8, 127.2) vs. 168.9 (150.8, 183.0) ms, p < 0.001]. Post-implant repolarization parameters such as QTc (442.7 ± 35.8 vs. 469.8 ± 51.5 ms, p < 0.001), TpTe [78.3 (73.3, 86.0) vs. 100.0 (86.0, 113.1) ms, p < 0.001] and TpTe/QTc (0.18 ± 0.02 vs. 0.21 ± 0.03, p < 0.001) also decreased compared with baseline. (Figure 1).
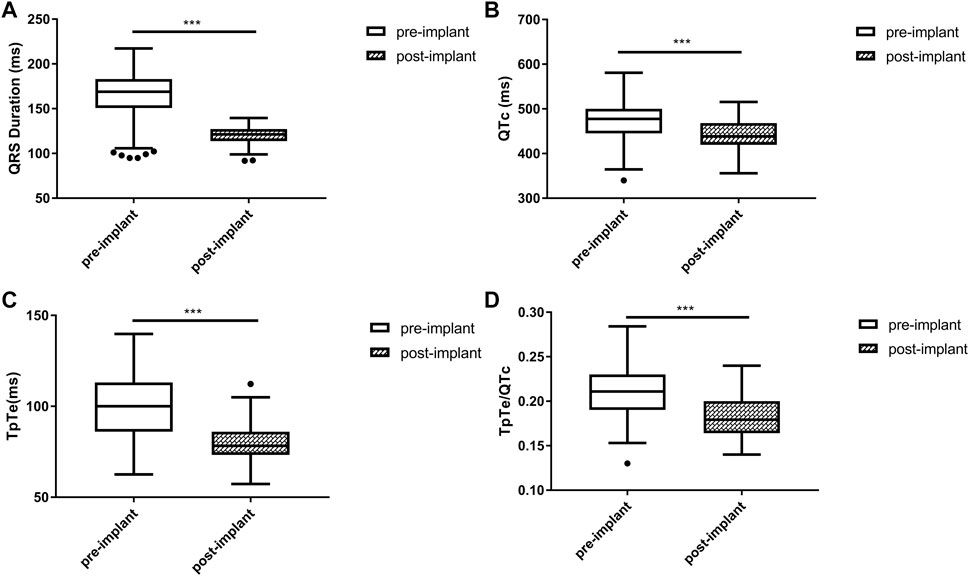
FIGURE 1. Changes in QRS duration (A), QTc (B), TpTe (C), TpTe/QTc (D) before and after LBBAP in HF patients (n = 59). (Box plots exhibited the smallest nonoutlier, first quartile, median, third quartile, and largest non-outlier of each variable from bottom to top. HF, heart failure; LBBAP, left bundle branch area pacing; QTc, QT corrected measurement; TpTe, Tpeak-end interval. *p < 0.05, **p < 0.01, ***p < 0.001).
Baseline QRSd and prior RVP history (CRT upgrade) are known to influence ventricular repolarization after traditional BiVP (Chalil et al., 2006; Lellouche et al., 2007). Therefore, we further analyzed the changes in ECG parameters after LBBAP according to baseline ECG patterns. For patients with QRSd ≥130 ms, QRSd (120.9 ± 8.7 vs. 174.5 ± 17.1 ms, p < 0.001) and the repolarization parameters [QTc, 445.5 ± 35.2 vs. 487.9 ± 43.5 ms, p < 0.001; TpTe, 78.1 (73.6, 86.3) vs. 108.3 (95.2, 116.2) ms, p < 0.001; TpTe/QTc, 0.18 ± 0.02 vs. 0.22 ± 0.03, p < 0.001] were all significantly decreased after LBBAP (Figure 2). For patients with QRSd <130 ms, QRSd, TpTe, and TpTe/QTc were similar before and after the implantation, only QTc (430.0 ± 25.9 vs. 404.4 ± 29.9 ms, p < 0.05) was significantly increased after the implantation (Figure 3). For upgrade patients, ECGs recorded during baseline RVP and post-implant LBBAP were compared. The results turned out that post-implant QRSd (124.7 ± 7.9 vs. 169.0 ± 17.4 ms, p < 0.001) and TpTe/QTc (0.19 ± 0.02 vs. 0.22 ± 0.02, p < 0.05) significantly decreased; while no significant changes in QTc and TpTe were observed (Figure 4). In addition, although baseline ECG data were statistically different between the three groups, repolarization parameters after the implantation showed no significant difference (Table 3).
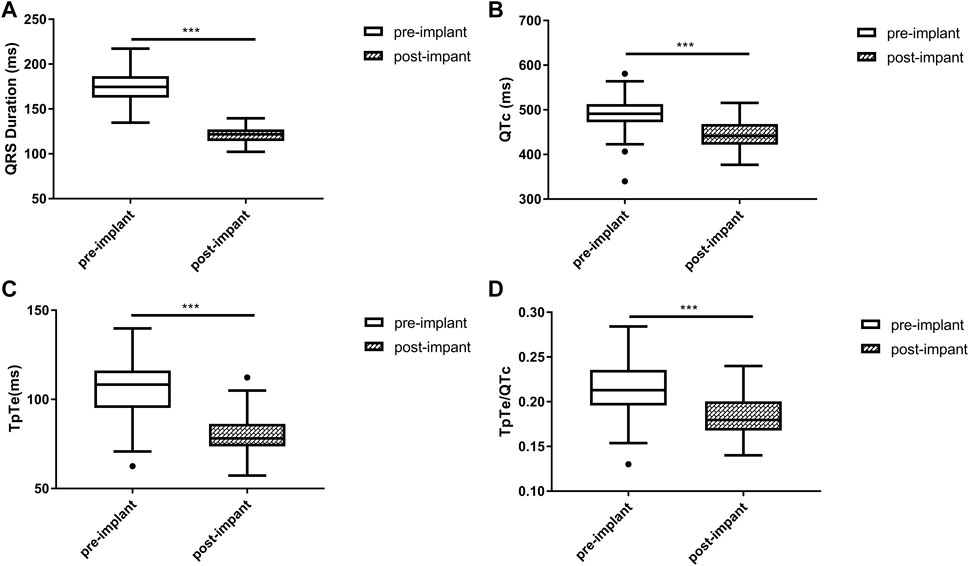
FIGURE 2. Changes in QRS duration (A), QTc (B), TpTe (C), TpTe/QTc (D) before and after LBBAP in patients with wide QRS but not paced rhythm (n = 40). (Box plots exhibited the smallest nonoutlier, first quartile, median, third quartile, and largest non-outlier of each variable from bottom to top. LBBAP left bundle branch area pacing; QTc, QT corrected measurement; TpTe, Tpeak-Tend interval. *p < 0.05, **p < 0.01, ***p < 0.001).
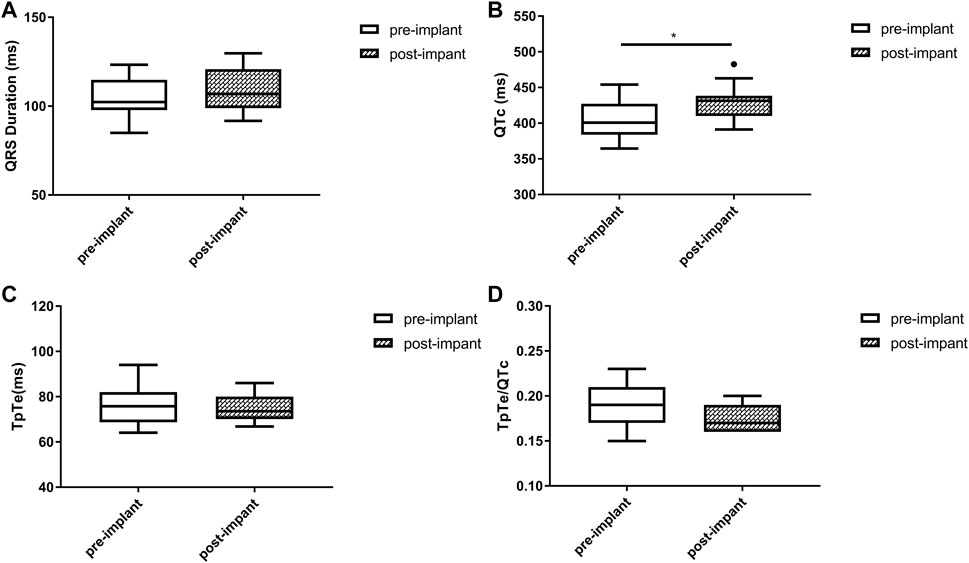
FIGURE 3. Changes in QRS duration (A), QTc (B), TpTe (C), TpTe/QTc (D) before and after LBBAP in patients with baseline narrow QRS (n = 11). (Box plots exhibited the smallest nonoutlier, first quartile, median, third quartile, and largest non-outlier of each variable from bottom to top. LBBAP left bundle branch area pacing; QTc, QT corrected measurement; TpTe, Tpeak-Tend interval. *p < 0.05, **p < 0.01, ***p < 0.001).
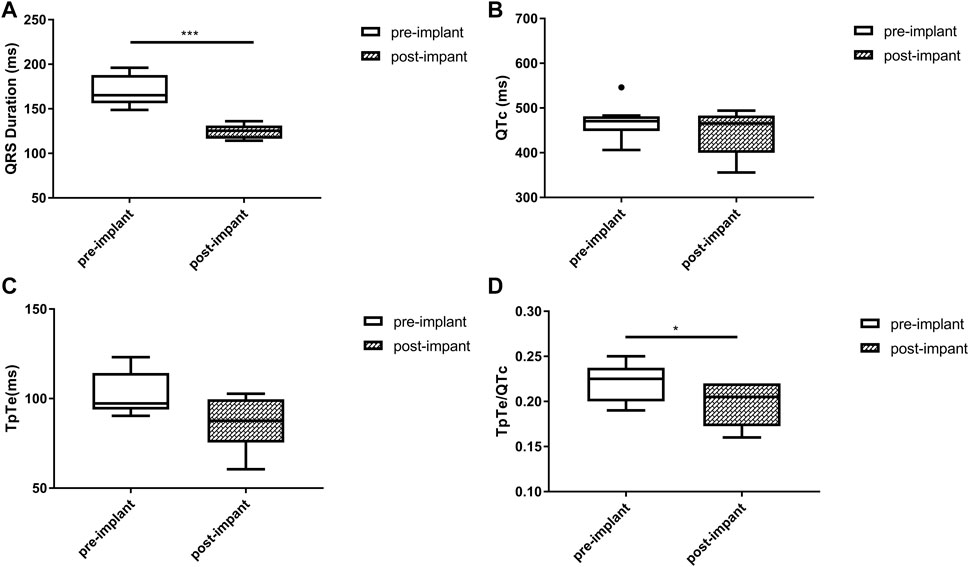
FIGURE 4. Changes in QRS duration (A), QTc (B), TpTe (C), TpTe/QTc (D) before and after LBBAP in patients with prior right ventricular paced rhythm (n = 8). (Box plots exhibited the smallest nonoutlier, first quartile, median, third quartile, and largest non-outlier of each variable from bottom to top. LBBAP left bundle branch area pacing; QTc, QT corrected measurement; TpTe, Tpeak-Tend interval. *p < 0.05, **p < 0.01, ***p < 0.001).
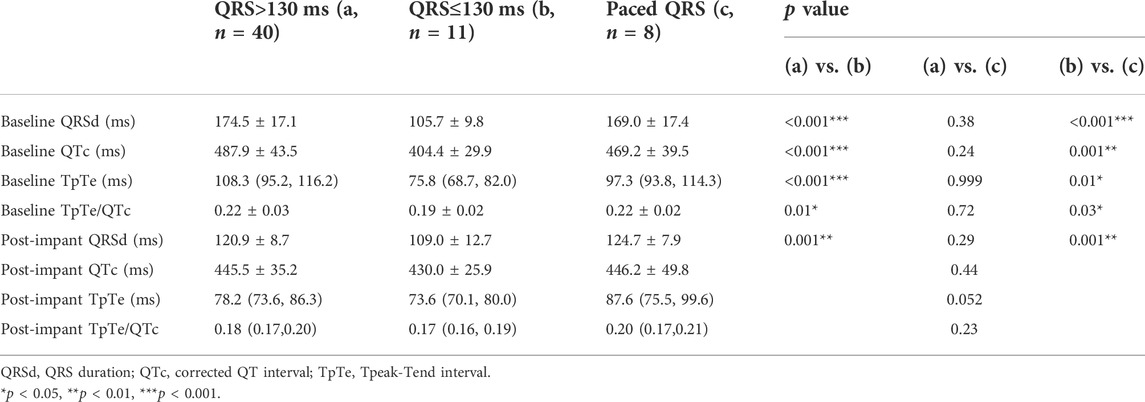
TABLE 3. Comparison of electrocardiographic data between patients with different QRS configuration at baseline.
Echocardiographic improvement after LBBAP
After a median follow-up period of 4 months, overall LVEF improved significantly from 33.7 ± 6.7% to 46.6 ± 11.0%, while LVEDD decreased from 63.0 (57.0, 71.0) mm to 55.0 (53.0, 63.0) mm (p < 0.001). Echocardiographic response was noted in 44 (74.6%) patients for the whole population. Specifically, the response rate was 72.7% (8/11) for patients with QRS<130 ms while 75% (36/48) for patients with wide QRS. Baseline LVEF and LVEDD were similar between responders and non-responders, while follow-up LVEF [52.5 (43.5, 56.8) vs. 36.0 (28.0, 44.0) %, p < 0.001] was significantly higher and LVEDD [54.0 (50.3, 58.8) vs. 63.0 (55.0, 69.0) mm, p < 0.01] was significantly smaller in responders. The absolute improvement in LVEF was also significantly higher in responders than non-responders [16.0 (8.0, 23.0) vs. 2.0 (-2.0, 4.0) %, p < 0.001]. Super-response was noted in 18 (30.5%) patients, and the improvement in LVEF in this subgroup was even higher (26.4 ± 5.8 vs. 7.0 ± 6.0%,p < 0.001).
The association between ECG parameters and CRT response
The association between ECG parameters and CRT response in patients with wide QRS (QRSd ≥130 ms with paced rhythm or not) was evaluated. A comparison of baseline characteristics between responders and non-responders was illustrated in Table 4. Stepwise multivariable analysis was performed including factors presenting p < 0.15 in univariate analysis (amiodarone, post-implant QRSd, post-implant TpTe, and post-implant QTc) and previously identified correlation factors (LBBB and LVEDD), and the result turned out that post-implant TpTe (odds ratios: 0.887, 95% confidence interval: 0.802 to 0.982, p < 0.05), amiodarone, and baseline LVEDD were independently associated with LBBAP response (Table 5). To be noted, post-implant TpTe/QTc with p = 0.08 was excluded from the multivariate model due to the collinearity problem.
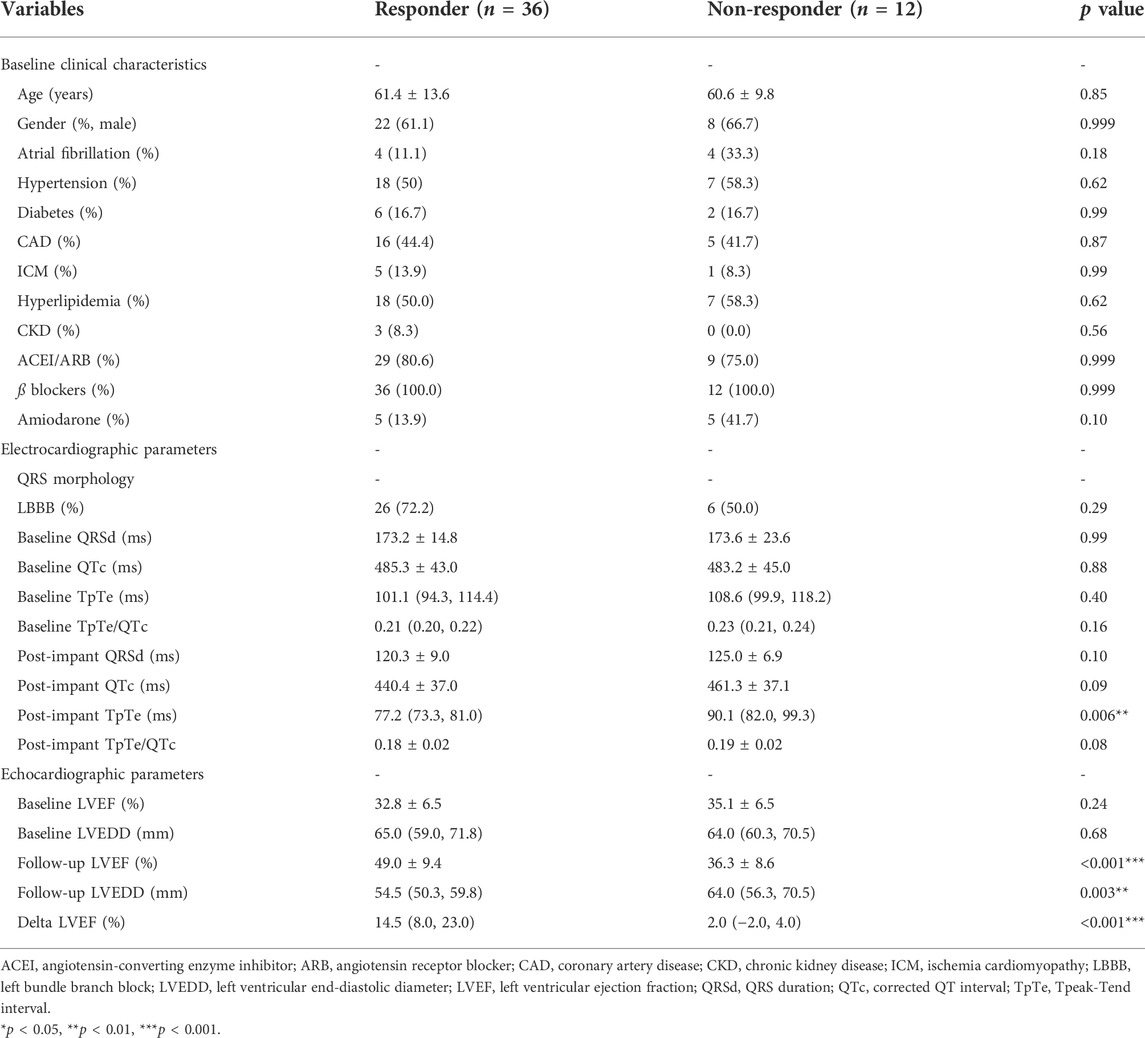
TABLE 4. Comparison of clinical, electro-cardiographic and echocardiographic data between responders and non-responders with QRS >130 ms.
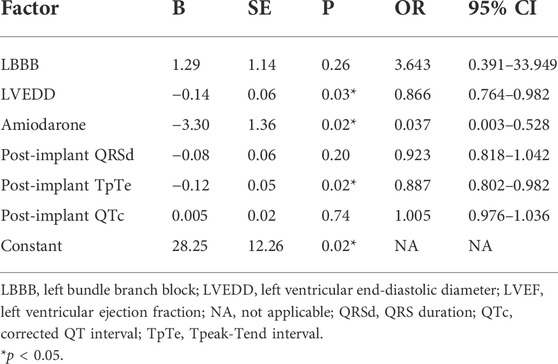
TABLE 5. Multivariable logistic regression analysis of factors associated with echocardiographic response among patients with QRS >130 ms (n = 48).
ROC curve analysis was then performed. The result showed an area under the curve of 0.77 with 95% confidential interval of 0.60–0.93 (p < 0.01) (Figure 5). Sensitivity and specificity were 83.3% and 77.8% respectively with a cutoff value of 81.2 ms for post-implant TpTe predicting LBBAP echocardiographic response. The positive and negative predictive values of post-implant TpTe were 93.3% and 55.6%, respectively. Patients with post-implant TpTe shorter than 81.2 ms had more significant increase in LVEF [12.5 (7.0, 23.0) vs. 4.0 (1.0, 14.0) %, p < 0.05] than others. The rate of echocardiographic response was also significantly higher in this subgroup of patients (93.3 vs. 44.4%, p < 0.01).
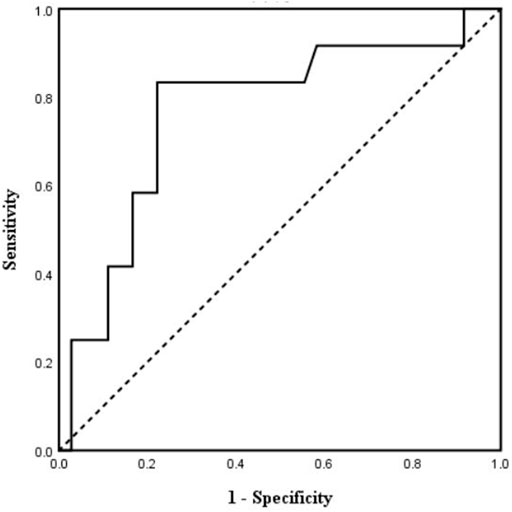
FIGURE 5. Receiver operating characteristic curve analysis of post-implant TpTe according to echocardiographic response (n = 48). TpTe: Tpeak-Tend interval.
Subgroup analysis was then performed among patients with or without LBBB at baseline. Among the 16 patients with non-LBBB, post-implant TpTe <81.2 ms showed a significantly higher rate of LBBAP response (100% vs. 14.3%, p < 0.01). However, of the remaining 32 patients with LBBB at baseline, the response rate seemed higher in patients with shorter TpTe but without statistical significance (90.5 vs. 63.6%, p = 0.07). Of note, no significant difference was observed in post-implant TpTe between responders and non-responders among patients with narrow QRS at baseline (74.6 ± 9.9 vs. 75.2 ± 5.6 ms, p > 0.05).
Discussion
The study found that DVR improved after LBBAP among the total HF population. Although trends of repolarization parameters varied according to different QRS configurations at baseline, the post-implant parameters were similar between groups. In addition, LBBAP caused significant echocardiographic improvement after a median follow-up period of 4 months, and post-implant TpTe was significantly associated with the echocardiographic response after LBBAP among patients with wide QRS.
Effects of LBBAP on repolarization parameters in HF patients
QTc, TpTe, and TpTe/QTc are all simple and accurate ECG markers of DVR (Shimizu and Antzelevitch, 1998; Prenner et al., 2016). These parameters are not only predictors of ventricular arrhythmias (VAs) and sudden cardiac death after CRT implantation but also related to myocardial mechanics (myocardial contraction and dilation) (Chalil et al., 2006; Sauer et al., 2014; Cvijic et al., 2018). Recently, Ponnusamy and Vijayaraman (2021) found that QTc, TpTe, and TpTe/QTc decreased immediately after LBBAP in 13 patients with LBBB-induced cardiomyopathy, preliminarily indicating the improvement of DVR after LBBAP. Our study analyzed the changes in repolarization parameters after LBBAP in the largest HF population to date, and the results turned out that QTc, TpTe, and TpTe/QTc significantly decreased, consistent with Ponnusamy’s study. Those results possibly suggested lower risks of VAs and better myocardial mechanics after LBBAP in HF patients and further studies were in need to prove this.
To be noted, baseline QRS configurations of the population were heterogeneous in our study. QRS configuration was known to influence repolarization parameters (Chalil et al., 2006; Lellouche et al., 2007), thus the study further analyzed those parameters in terms of baseline QRS patterns. In patients with QRS ≥130 ms, all three repolarization parameters decreased significantly after LBBAP, indicating the definite improvement of DVR in this subgroup of patients. In the other two groups, the changes of QTc were slightly discordant with the changes of TpTe and TpTe/QTc. Since TpTe reflected more accurately DVR than QTc (Yan and Antzelevitch, 1998), it was thus concluded that DVR improved in patients with prior RV-paced rhythm while barely deteriorated in patients with narrow QRS after LBBAP. Those results suggested the protective effects of LBBAP on repolarization stability in HF patients despite baseline QRS morphology. A similar study on BiVP found that DVR increased significantly in patients with LBBB or narrow QRS (Lellouche et al., 2007). Therefore, LBBAP might show prominent edges over BiVP concerning repolarization stability in HF patients indicated for CRT.
The mechanisms underlying the changes in DVR after LBBAP remain unclear. Mechano-electrical feedback, an established mechanism whereby myocardial strain causes changes in electrophysiological parameters, may partly account for this (Orini et al., 2017). Specifically, the reduction of ventricular wall stress and the improvement of ventricular synchrony could reverse the remodeling of repolarization-related potassium and calcium channels (Aiba and Tomaselli, 2012), which may be the molecular basis for the improvement of DVR after LBBAP in patients with wide QRS. Since LBBAP could achieve quite narrow QRSd and near-physiological ventricular synchrony (Strocchi et al., 2020), DVR barely deteriorated in patients with narrow QRSd at baseline.
Post-implant TpTe was associated with the echocardiographic response after LBBAP
LV reverse remodeling of HF patients after LBBAP has been demonstrated by several studies (Huang W. et al., 2020; Li et al., 2020; Vijayaraman et al., 2021). The population in this study achieved an echocardiographic response rate of 74.6% after a median follow-up period of 4 months. However, two recent studies reported a much higher response rate of 88.9% (Huang W. et al., 2020)and 92% (Li et al., 2020), respectively. Baseline QRS configuration and definition of echocardiographic response may account for this discrepancy. For example, both studies mentioned above merely included patients with LBBB at baseline, which has been demonstrated as an independent predictor of LBBAP response (Vijayaraman et al., 2021). Yet baseline QRS pattern was heterogeneous in our study, only 54.2% were LBBB. Consistent with our results, Vijayaraman et al. reported a response rate of 73% in a population with various baseline QRS configurations (Vijayaraman et al., 2021).
Data on predictors for LBBAP response or non-response are quite limited to date. Recently, LBBB and smaller LVEDD were reported as independent predictors of echocardiographic response after LBBAP (Vijayaraman et al., 2021). Yet even in an LBBB population, around 10% of cases showed LBBAP was non-responsive (Huang W. et al., 2020; Li et al., 2020). More favorable predictors remain a matter of investigation. Ventricular repolarization parameters were related to cardiac mechanics and systolic function. Sauer et al. reported an independent relationship between TpTe and radial SD-tPkS (measuring contraction duration heterogeneity on echocardiography) (Sauer et al., 2014). In addition, DVR was found to be significantly correlated with impaired LVEF (Huang H. C. et al., 2020). However, whether repolarization parameters were associated with LBBAP response remains unclear. In the current cohort, post-implant TpTe was proved to be the independent predictor of echocardiographic response in addition to previously identified LVEDD among patients with wide QRS. Further ROC analysis determined a cut-off value of 81.2 ms in predicting echocardiographic response (sensitivity 83.3%, specificity77.8%, p < 0.01). Post-implant TpTe <81.2 ms had a high positive predictive value (93.3%) in LBBAP response, although an ECG not meeting the aforementioned criteria did not guarantee LBBAP non-response (negative predictive value 55.6%). These results indicated the significant association between post-implant TpTe and echocardiographic response after LBBAP in HF patients. Of note, amiodarone was also significant in the multivariate model with an OR of 0.037, consistent with the previous finding that amiodarone was related to adverse outcomes in patients upgraded to CRT-defibrillators (Adelstein et al., 2019). These two new predictors may further optimize LBBAP response via post-implant management.
A previous study has reported baseline LBBB as an independent predictor of LBBAP response (Vijayaraman et al., 2021). Consistently, there were more LBBB patients in responders than non-responders in our study, although without statistical significance (responders vs. non-responders: 72.2% vs. 50%). Since non-LBBB tends to result in a higher rate of non-response and worse prognosis, it is of vital clinical significance to improve response in this subgroup of patients. In the current study, post-implant TpTe <81.2 ms showed a significantly higher rate of LBBAP response among patients with non-LBBB (100% vs. 14.3%, p < 0.01). The result implied that a high percentage of non-LBBB patients may respond to LBBAP if post-implant TpTe criteria were met through post-operative ECG management. For LBBB patients, the TpTe criteria seemed to exert similar influence although without statistical significance (90.5 vs. 63.6%, p = 0.07). Thus, the effects of post-implant TpTe in predicting echocardiographic response may be more prominent in non-LBBB patients than LBBB patients although larger-sample trials were warranted to verify this. In addition, no association between post-implant TpTe and LBBAP response among patients with narrow QRSd was observed, thus the predictive value of this ECG marker could not be applied to these patients.
This was the first study to demonstrate the potential value of repolarization parameters in predicting LBBAP echocardiographic response, although the underlying mechanisms remained to be further studied. Electromechanical coupling and calcium handling may play a role (Prenner et al., 2016). If our data are to be confirmed by larger prospective studies, post-implant TpTe should be considered for future management of CRT recipients in addition to paced QRSd and QRS morphology to further improve response.
However, the study still had several limitations. First, this was a retrospective, single-center study with small sample size, thus our results needed to be confirmed by a larger, prospective study. Second, echocardiogram measurements were not performed by two independent investigators due to the retrospective nature of the study. Finally, only echocardiographic response was assessed in the study, and it would be more desirable to assess clinical response markers such as heart failure hospitalizations and mortality as well.
Conclusion
LBBAP improved DVR significantly in HF patients. Post-implant TpTe was associated with the echocardiographic response after LBBAP among patients with wide QRS, especially for non-LBBB patients.
Data availability statement
The raw data supporting the conclusions of this article will be made available by the authors, without undue reservation.
Ethics statement
The studies involving human participants were reviewed and approved by Fuwai Hospital Institutional Review Board. The patients/participants provided their written informed consent to participate in this study.
Author contributions
YL and WL provided the design of the study and wrote the drafting of the manuscript; QH and CC were involved in the literature search and statistical analysis of the data; Data acquisition was completed by JL, YZ, and RC. SZ participated in the manuscript review and revision. KC and YD were responsible for the integrity of the work from inception to the published article. All authors read and met the criteria for authorship and agree with the results and conclusions of the report.
Funding
The study was funded by the National Natural Science Foundation of China (Grant No. 81870260) and the Central Public-Interest Scientific Institution Basal Research Fund: Chinese Academy of Medical Sciences (Internal Grant Number 2018-F01).
Conflict of interest
The authors declare that the research was conducted in the absence of any commercial or financial relationships that could be construed as a potential conflict of interest.
Publisher’s note
All claims expressed in this article are solely those of the authors and do not necessarily represent those of their affiliated organizations, or those of the publisher, the editors, and the reviewers. Any product that may be evaluated in this article, or claim that may be made by its manufacturer, is not guaranteed or endorsed by the publisher.
References
Adelstein E. C., Althouse A. D., Davis L., Schwartzman D., Bazaz R., Jain S., et al. (2019). Amiodarone is associated with adverse outcomes in patients with sustained ventricular arrhythmias upgraded to cardiac resynchronization therapy-defibrillators. J. Cardiovasc. Electrophysiol. 30 (3), 348–356. doi:10.1111/jce.13828
Aiba T., Tomaselli G. (2012). Electrical remodeling in dyssynchrony and resynchronization. J. Cardiovasc. Transl. Res. 5 (2), 170–179. doi:10.1007/s12265-012-9348-9
Antzelevitch C., Sicouri S., Di Diego J. M., Burashnikov A., Viskin S., Shimizu W., et al. (2007). Does Tpeak-Tend provide an index of transmural dispersion of repolarization? Heart rhythm. 4 (8), 1114–1116. doi:10.1016/j.hrthm.2007.05.028
Castro Hevia J., Antzelevitch C., Tornés Bárzaga F., Dorantes Sánchez M., Dorticós Balea F., Zayas Molina R., et al. (2006). Tpeak-Tend and Tpeak-Tend dispersion as risk factors for ventricular tachycardia/ventricular fibrillation in patients with the Brugada syndrome. J. Am. Coll. Cardiol. 47 (9), 1828–1834. doi:10.1016/j.jacc.2005.12.049
Chalil S., Yousef Z. R., Muyhaldeen S. A., Smith R. E., Jordan P., Gibbs C. R., et al. (2006). Pacing-induced increase in QT dispersion predicts sudden cardiac death following cardiac resynchronization therapy. J. Am. Coll. Cardiol. 47 (12), 2486–2492. doi:10.1016/j.jacc.2005.12.074
Chen K., Li Y., Dai Y., Sun Q., Luo B., Li C., et al. (2019). Comparison of electrocardiogram characteristics and pacing parameters between left bundle branch pacing and right ventricular pacing in patients receiving pacemaker therapy. Europace 21 (4), 673–680. doi:10.1093/europace/euy252
Cho E. J., Park S. J., Park K. M., On Y. K., Kim J. S. (2016). Paced QT interval as a risk factor for new-onset left ventricular systolic dysfunction and cardiac death after permanent pacemaker implantation. Int. J. Cardiol. 203, 158–163. doi:10.1016/j.ijcard.2015.10.128
Cvijic M., Antolic B., Klemen L., Zupan I. (2018). Repolarization heterogeneity in patients with cardiac resynchronization therapy and its relation to ventricular tachyarrhythmias. Heart rhythm. 15 (12), 1784–1790. doi:10.1016/j.hrthm.2018.06.023
Ellenbogen K. A., Huizar J. F. (2012). Foreseeing super-response to cardiac resynchronization therapy: A perspective for clinicians. J. Am. Coll. Cardiol. 59 (25), 2374–2377. doi:10.1016/j.jacc.2011.11.074
Emori T., Antzelevitch C. (2001). Cellular basis for complex T waves and arrhythmic activity following combined I(Kr) and I(Ks) block. J. Cardiovasc. Electrophysiol. 12 (12), 1369–1378. doi:10.1046/j.1540-8167.2001.01369.x
Epstein A. E., DiMarco J. P., Ellenbogen K. A., Estes N. A., Freedman R. A., Gettes L. S., et al. (2013). 2012 ACCF/AHA/HRS focused update incorporated into the ACCF/AHA/HRS 2008 guidelines for device-based therapy of cardiac rhythm abnormalities: A report of the American College of cardiology foundation/American heart association task force on practice guidelines and the heart rhythm society. J. Am. Coll. Cardiol. 61 (3), e6–75. doi:10.1016/j.jacc.2012.11.007
Huang H. C., Chien K. L., Chang Y. C., Lin L. Y., Wang J., Liu Y. B., et al. (2020a). Increases in repolarization heterogeneity predict left ventricular systolic dysfunction and response to cardiac resynchronization therapy in patients with left bundle branch block. J. Cardiovasc. Electrophysiol. 31 (7), 1770–1778. doi:10.1111/jce.14488
Huang W., Wu S., Vijayaraman P., Su L., Chen X., Cai B., et al. (2020b). Cardiac resynchronization therapy in patients with nonischemic cardiomyopathy using left bundle branch pacing. JACC. Clin. Electrophysiol. 6 (7), 849–858. doi:10.1016/j.jacep.2020.04.011
Itoh M., Yoshida A., Fukuzawa K., Kiuchi K., Imamura K., Fujiwara R., et al. (2013). Time-dependent effect of cardiac resynchronization therapy on ventricular repolarization and ventricular arrhythmias. Europace 15 (12), 1798–1804. doi:10.1093/europace/eut145
Lee J. M., Janardhan A. H., Kang K. W., Joung B., Pak H. N., Sundaram S., et al. (2014). Paced QT interval is a better predictor of mortality than the intrinsic QT interval: Long-term follow-up study. Heart rhythm. 11 (7), 1184–1189. doi:10.1016/j.hrthm.2014.04.006
Lellouche N., De Diego C., Akopyan G., Boyle N. G., Mahajan A., Cesario D. A., et al. (2007). Changes and predictive value of dispersion of repolarization parameters for appropriate therapy in patients with biventricular implantable cardioverter-defibrillators. Heart rhythm. 4 (10), 1274–1283. doi:10.1016/j.hrthm.2007.06.012
Li X., Qiu C., Xie R., Ma W., Wang Z., Li H., et al. (2020). Left bundle branch area pacing delivery of cardiac resynchronization therapy and comparison with biventricular pacing. Esc. Heart Fail. 7 (4), 1711–1722. doi:10.1002/ehf2.12731
Li Y., Chen K., Dai Y., Li C., Sun Q., Chen R., et al. (2019). Left bundle branch pacing for symptomatic bradycardia: Implant success rate, safety, and pacing characteristics. Heart rhythm. 16 (12), 1758–1765. doi:10.1016/j.hrthm.2019.05.014
Lin J., Chen K., Dai Y., Sun Q., Li Y., Jiang Y., et al. (2020). Bilateral bundle branch area pacing to achieve physiological conduction system Activation. Circ. Arrhythm. Electrophysiol. 13 (8), e008267. doi:10.1161/circep.119.008267
Lin J., Hu Q., Chen K., Dai Y., Chen R., Sun Q., et al. (2021). Relationship of paced left bundle branch pacing morphology with anatomic location and physiological outcomes. Heart rhythm. 18 (6), 946–953. doi:10.1016/j.hrthm.2021.03.034
Orini M., Nanda A., Yates M., Di Salvo C., Roberts N., Lambiase P. D., et al. (2017). Mechano-electrical feedback in the clinical setting: Current perspectives. Prog. Biophys. Mol. Biol. 130 (Pt B), 365–375. doi:10.1016/j.pbiomolbio.2017.06.001
Ponnusamy S. S., Vijayaraman P. (2021). Left bundle branch block-induced cardiomyopathy: Insights from left bundle branch pacing. JACC. Clin. Electrophysiol. 7, 1155–1165. doi:10.1016/j.jacep.2021.02.004
Prenner S. B., Shah S. J., Goldberger J. J., Sauer A. J. (2016). Repolarization heterogeneity: Beyond the QT interval. J. Am. Heart Assoc. 5 (5), e003607. doi:10.1161/jaha.116.003607
Sauer A. J., Selvaraj S., Aguilar F. G., Martinez E. E., Wilcox J. E., Passman R., et al. (2014). Relationship between repolarization heterogeneity and abnormal myocardial mechanics. Int. J. Cardiol. 172 (1), 289–291. doi:10.1016/j.ijcard.2013.12.232
Sauer A., Wilcox J. E., Andrei A. C., Passman R., Goldberger J. J., Shah S. J., et al. (2012). Diastolic electromechanical coupling: Association of the ECG T-peak to T-end interval with echocardiographic markers of diastolic dysfunction. Circ. Arrhythm. Electrophysiol. 5 (3), 537–543. doi:10.1161/circep.111.969717
Shimizu W., Antzelevitch C. (1998). Cellular basis for the ECG features of the LQT1 form of the long-QT syndrome: Effects of beta-adrenergic agonists and antagonists and sodium channel blockers on transmural dispersion of repolarization and torsade de pointes. Circulation 98 (21), 2314–2322. doi:10.1161/01.cir.98.21.2314
Strocchi M., Lee A. W. C., Neic A., Bouyssier J., Gillette K., Plank G., et al. (2020). His-bundle and left bundle pacing with optimized atrioventricular delay achieve superior electrical synchrony over endocardial and epicardial pacing in left bundle branch block patients. Heart rhythm. 17 (11), 1922–1929. doi:10.1016/j.hrthm.2020.06.028
Tooley J., Ouyang D., Hadley D., Turakhia M., Wang P., Ashley E., et al. (2019). Comparison of QT interval measurement methods and correction formulas in atrial fibrillation. Am. J. Cardiol. 123 (11), 1822–1827. doi:10.1016/j.amjcard.2019.02.057
Vandenberk B., Vandael E., Robyns T., Vandenberghe J., Garweg C., Foulon V., et al. (2016). Which QT correction formulae to use for QT monitoring? J. Am. Heart Assoc. 5 (6), e003264. doi:10.1161/jaha.116.003264
Vijayaraman P., Ponnusamy S., Cano Ó., Sharma P. S., Naperkowski A., Subsposh F. A., et al. (2021). Left bundle branch area pacing for cardiac resynchronization therapy: Results from the international LBBAP collaborative study group. JACC. Clin. Electrophysiol. 7 (2), 135–147. doi:10.1016/j.jacep.2020.08.015
Yan G. X., Antzelevitch C. (1998). Cellular basis for the normal T wave and the electrocardiographic manifestations of the long-QT syndrome. Circulation 98 (18), 1928–1936. doi:10.1161/01.cir.98.18.1928
Keywords: left bundle branch area pacing, repolarization, Tpeak—Tend, echocardiographic response, heart failure
Citation: Li Y, Lu W, Hu Q, Cheng C, Lin J, Zhou Y, Chen R, Dai Y, Chen K and Zhang S (2022) Changes of repolarization parameters after left bundle branch area pacing and the association with echocardiographic response in heart failure patients. Front. Physiol. 13:912126. doi: 10.3389/fphys.2022.912126
Received: 04 April 2022; Accepted: 04 July 2022;
Published: 04 August 2022.
Edited by:
Aldo Rinaldi, King’s College London, United KingdomReviewed by:
Viktoria Szuts, University of Szeged, HungaryJan E. Azarov, Institute of Physiology, Komi Scientific Center (RAS), Russia
Copyright © 2022 Li, Lu, Hu, Cheng, Lin, Zhou, Chen, Dai, Chen and Zhang. This is an open-access article distributed under the terms of the Creative Commons Attribution License (CC BY). The use, distribution or reproduction in other forums is permitted, provided the original author(s) and the copyright owner(s) are credited and that the original publication in this journal is cited, in accordance with accepted academic practice. No use, distribution or reproduction is permitted which does not comply with these terms.
*Correspondence: Yan Dai, ZGFpeTc1MTZAc2luYS5jb20=; Keping Chen, Y2hlbmtlcGluZ0AyNjMubmV0
†These authors have contributed equally to this work and share the first authorship