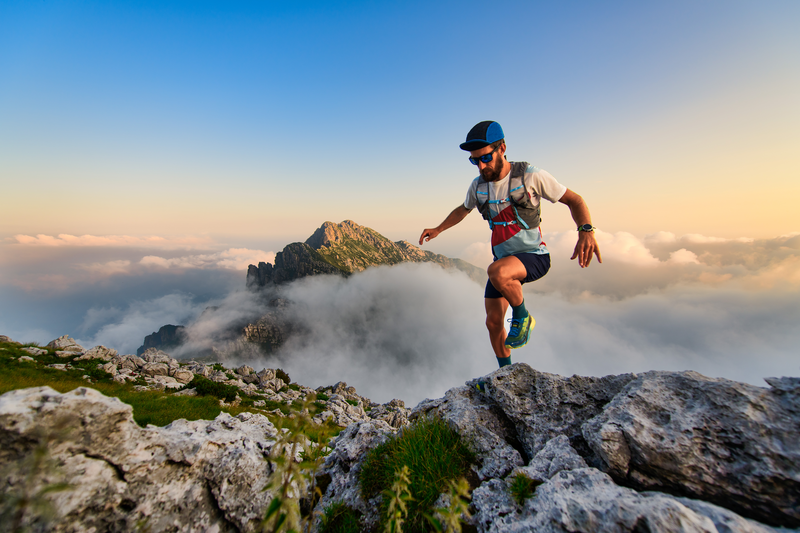
94% of researchers rate our articles as excellent or good
Learn more about the work of our research integrity team to safeguard the quality of each article we publish.
Find out more
SYSTEMATIC REVIEW article
Front. Physiol. , 01 November 2022
Sec. Renal Physiology and Pathophysiology
Volume 13 - 2022 | https://doi.org/10.3389/fphys.2022.909491
Background: The pathogenesis of idiopathic membranous nephropathy (IMN) has not yet been thoroughly clarified, and gut dysbiosis may be a contributor to IMN. However, the characterization of gut microbiota in patients with IMN remains uncertain.
Methods: Cochrane Library, PubMed, China National Knowledge Internet, Web of Science, and Embase were used to search for studies through 18 May 2022. A meta-analysis based on the standardized mean difference (SMD) with 95% confidence interval (CI) was conducted on the alpha diversity index. The between-group comparison of the relative abundance of gut microbiota taxa and the beta diversity were extracted and qualitatively analyzed.
Results: Five studies were included involving 290 patients with IMN, 100 healthy controls (HCs), and 129 patients with diabetic kidney disease (DKD). The quantitative combination of alpha diversity indices indicated that although bacterial richness was impaired [ACE, SMD = 0.12, (−0.28, 0.52), p = 0.55, I2 = 0%; Chao1, SMD = −0.34, (−0.62, −0.06), p < 0.05, I2 = 36%], overall diversity was preserved [Shannon, SMD = −0.16, (−0.64, 0.31), p = 0.50, I2 = 53%; Simpson, SMD = 0.27, (−0.08, 0.61), p = 0.13, I2 = 0%]. The beta diversity was significantly varied compared to HCs or DKD patients. Compared to HCs, the abundance of Proteobacteria increased, while that of Firmicutes decreased at the phylum level. Furthermore, the abundance of Lachnospira were depleted, while those of Streptococcus were enriched at the genus level. Proteobacteria and Streptococcus were also increased compared to DKD patients.
Conclusions: The expansion of Proteobacteria and depletion of Lachnospira may be critical features of the altered gut microbiota in patients with IMN. This condition may play an important role in the pathogenesis of IMN and could provide bacterial targets for diagnosis and therapy.
Idiopathic membranous nephropathy (IMN) is one of the most common pathological types of chronic kidney disease (CKD) and nephrotic syndrome in adults and is an immune-mediated glomerular disease characterized by the deposition of numerous immune complexes on the epithelial side of the glomerular capillary loops (Ronco et al., 2021). Currently, the pathogenesis of IMN is principally accepted to involve a combination of the target antigen of glomerular podocytes combined with autoantibodies to form immune complexes, triggering complement that causes tissue damage; but the mechanism has not been sufficiently clarified (Ronco and Debiec, 2020). The targeted antigens identified thus far include the M-type phospholipase A2 receptor (PLA2R), the thrombospondin type 1 domain-containing 7A (THSD7A), exostosin 1/exostosin 2 (EXT1/EXT2), the neural EGF-like-1 protein (NELL-1), semaphorin 3B (Sema3B), protocadherin 7 (PCDH7), and the high-temperature requirement A serine peptidase 1 (HTRA1), which all bind to antibodies of the IgG subtype (Sethi, 2021). The existing therapy for IMN primarily incorporates symptomatic treatment and etiological treatment including corticosteroids, immunosuppressive drugs and rituximab. While this treatment maintains a satisfactory prognosis for most patients (Couser, 2017), there are still almost 30% whose symptoms are not alleviated. Thus, it is urgent to explore the precise mechanisms of IMN. Recent studies have shown that gut dysbiosis may play a vital role in the pathogenesis of CKD (Zhao J. et al., 2021).
A vast ecosystem of microbes lives in the human gut, which has coevolved with humans for mutual benefit (Davenport et al., 2017), in preserving health and preventing disease (Ramezani and Raj, 2014). According to the “gut-kidney axis” theory, the disturbance of gut microbiota is closely related to CKD and interacts as both cause and effect. The gut microbes can activate immune cells via their metabolites and other components, causing inflammatory reactions and accelerating the advancement of kidney disease (Pahl and Vaziri, 2015). Antigens derived from the gut microbial community can induce conventional T cells to differentiate into a wide range of effector cells such as Th2 cells, Th17 cells, and T regulatory cells (Tregs) (Ivanov et al., 2022), which regulate the autoimmune response and immune homeostasis, thus affecting the occurrence and development of IMN (Cremoni et al., 2020; Zhao Q. et al., 2021). Systematic exposure to different microbial populations significantly stimulated B cells and induced the production of characteristic immunoglobulins such as IgG (Li et al., 2020), which may bind to IMN target antigens to mediate kidney damage. Our previous study reported that gut dysbiosis was a hallmark of patients with IMN (Dong et al., 2020) and contributed to high morbidity. Thus, we postulated that immune disorders regulated by gut microbiota dysbiosis under abnormal pathological conditions may initiate autoimmune diseases, including IMN. Secreted PLA2-IIA has been found to influence the immune system by hydrolyzing bacterial membranes and altering the composition of gut microbiota. It participates in the induction of immunophenotypes and promotes the inflammatory response (Dore et al., 2022), suggesting a potential relationship between the PLA2R antigen of IMN and gut dysbiosis. Currently, modulation of gut dysbiosis via fecal microbiota transplantation (FMT) has been shown to have promising efficacy for treating patients with IMN (Zhou et al., 2021).
The abovementioned results suggest that gut dysbiosis may be tightly correlated with IMN and is conceivably a critical target for treatment of IMN. However, the conclusions conveyed in the literature on the traits of gut microbiota in patients with IMN deviate extensively and the characteristic features are unclear. Therefore, we conducted a meta-analysis of data on the alterations in gut microbiota alpha diversity. A qualitative synthesis of the relative abundance of gut microbial taxa at different levels was also performed and beta diversity was determined. Specific metabolic characteristics of patients with IMN were assessed to identify the essential markers of gut microbiota and to provide new insights for the prevention and treatment of IMN.
We followed the guidelines of the preferred reporting project in the systematic review and meta-analysis (PRISMA), and the study was registered in the PROSPERO database (CRD42022307282). The combination of free words of medical subject terms (MeSH) or keywords, including all spellings of MN, gut, stool, and microbiota (Supplementary Table S1), were used to search for studies on gut microbiota of patients with IMN in the Cochrane Library, PubMed, China National Knowledge Internet, Web of Science, and Embase. The retrieval period was from the database creation time to 18 May 2022. To be included, a report had to be on a cross-sectional study or a case-control study and the study subjects had to be patients with IMN confirmed by clinicopathological examination. The exclusion criteria were: no control group established, unrelated confounding factors, repeated publications, inability to obtain the original data or to contact the author to obtain the original data, the abstract, the review, meta-analysis, case reports, or animal experiments. The coauthors, Zhang Y and Zhao J, screened the title, abstract, and full text of the articles to ensure consistency. The quality of the included case-control studies was assessed using the Newcastle–Ottawa scale (NOS) (Stang, 2010). The scale consisted of eight items and evaluated the three dimensions of selectivity, comparability, and exposure factors. The score, ranging from 0 to 10, indicated the quality of the publication.
The following information was extracted from each study: characteristics, analysis methods, sample collection, alpha diversity indices (the maximum, minimum, mean, M, and standard deviation, SD, of the ACE, Chao1, Shannon, and Simpson indices), beta diversity, differential taxa, and functional features of the gut microbiota. The alpha diversity indices were used to estimate the community richness (ACE and Chao1) and community diversity (Shannon and Simpson), while beta diversity was applied to measure the rate of change of the species diversity between communities. Excel was used to record and organize the data extracted from the selected studies. For parameters with quantitative descriptions including alpha diversity indices, if the original data only provided median and quartile range, a Web-based tool (http://www.math.hkbu.edu.hk/∼tongt/papers/median2mean.html) was used to convert it to mean and standard deviation. WebPlotDigitizer (Drevon et al., 2017) was used to extract digital data from figures if necessary. The two researchers (Zhang Y and Zhao J) independently extracted literature information, and any disputed content was resolved through discussion and negotiation by a third researcher (Sun S).
Meta-analysis of the alpha diversity indices reported from two or more studies was performed using RevMan5.3 software provided by the Cochrane collaboration, and the standardized mean difference (SMD) between the IMN patient group and the controls was calculated to analyze the differences in alpha diversity between the two groups. The I2 test was used to test the heterogeneity of the included studies. If I2 was ≤ 50%, the heterogeneity was small and a fixed-effect model was used for analysis; if I2 was >50%, the heterogeneity was high and a random effects model was adopted for analysis (Patrick et al., 2020). If the p values on the two sides were < 0.05, the differences were considered to be statistically significant.
The beta diversity of the microbiota and the relative abundance of its different constituents at the phylum and genus levels were qualitatively synthesized. To aid in data interpretation, we defined a parameter that the results were specific if they changed in the same direction in more than two studies and no study showed contrary results (Zhao J. et al., 2021). Results reported by only one study were excluded, because such results are considered potentially methodological- or population-specific, and thus may be false-positive results.
A total of 78 potentially eligible articles were obtained from the literature search, and five studies (Dong et al., 2020; Lang et al., 2020; Yu et al., 2020; Zhang et al., 2020; Li et al., 2022) were eventually included (Figure 1). All studies were of the case-control type, with four studies using healthy individuals as controls (HCs) and one study using patients with diabetic kidney disease (DKD) as the control group. The study researchers collected and analyzed stool samples from 519 participants from China, and16S rRNA gene sequencing was used to characterize the gut microbiota. A summary of the literature features is shown in Table 1. Additionally, all five studies mentioned freezing conditions for sample transfer or storage, while only two studies mentioned the use of special sample collection containers (Supplementary Table S2). The NOS scale was used to evaluate the quality of the reports, and four of the studies had an evaluation score of six* while the fifth was five* (Supplementary Table S3), which confirmed that the reports were of high quality.
Four studies compared the alpha diversity of gut microbiota in patients with IMN to that in HCs (Dong et al., 2020; Lang et al., 2020; Zhang et al., 2020; Li et al., 2022). In terms of richness, two studies (Dong et al., 2020; Lang et al., 2020) provided ACE index data, and three studies (Dong et al., 2020; Zhang et al., 2020; Li et al., 2022) provided Chao1 index data. In terms of diversity, three studies (Dong et al., 2020; Lang et al., 2020; Li et al., 2022) provided Shannon index data, and two studies provided Simpson index data (Dong et al., 2020; Li et al., 2022). The quantitative combination of alpha diversity indices indicated that although bacterial richness was impaired [ACE, SMD = 0.12, (−0.28, 0.52), p = 0.55, I2 = 0%; Chao1, SMD = −0.34, (−0.62, −0.06), p < 0.05, I2 = 36%], diversity was preserved overall [Shannon, SMD = −0.16, (−0.64, 0.31), p = 0.50, I2 = 53%; Simpson, SMD = 0.27, (−0.08, 0.61), p = 0.13, I2 = 0%] (Figure 2). One study (Yu et al., 2020) analyzed the alpha diversity of gut microbiota in patients with IMN or DKD, and because of the paucity of data, quantitative analysis was not possible.
FIGURE 2. Forest plots of alpha diversity in the gut microbiota of patients with IMN compared with HCs. (A) Ace index, SMD = 0.12, [−0.28, 0.52]; p = 0.55; I2 = 0%; (B) Chao1 index, SMD = −0.34, [−0.62, −0.06]; p < 0.05; I2 = 36%; (C) Shannon index, SMD = −0.16, [−0.64, 0.31]; p = 0.50; I2 = 53%; (D) Simpson index, SMD = 0.27, [−0.08, 0.61]; p = 0.13; I2 = 0%. IMN, idiopathic membranous nephropathy; HCs, healthy controls.
In all five studies (Dong et al., 2020; Lang et al., 2020; Yu et al., 2020; Zhang et al., 2020; Li et al., 2022), the beta diversity of the gut microbiota was measured by principal coordinate analysis (PCoA) or principal component analysis (PCA) (Supplementary Table S5). The four studies (Dong et al., 2020; Lang et al., 2020; Zhang et al., 2020; Li et al., 2022) with HCs uncovered marked dissimilarities in the beta diversity of gut microbiota between patients with IMN and HCs. One study (Yu et al., 2020) demonstrated that the beta diversity in patients with IMN was also greatly altered compared to DKD patients.
All studies (Dong et al., 2020; Lang et al., 2020; Yu et al., 2020; Zhang et al., 2020; Li et al., 2022) analyzed the distinct taxa at the phylum level. Compared with HCs, three studies reported that the abundance of Proteobacteria increased (Dong et al., 2020; Zhang et al., 2020; Li et al., 2022) while that of Firmicutes decreased (Lang et al., 2020; Zhang et al., 2020; Li et al., 2022), respectively. The abundance of Proteobacteria also increased in IMN patients compared to DKD patients (Yu et al., 2020). The enrichment of Actinobacteria was observed in patients with IMN compared with HCs (Li et al., 2022) and in one group of DKD patients (Yu et al., 2020). The variations in the abundance of Bacteroidetes were inconsistent; it was increased in one study (Lang et al., 2020), but decreased in two studies (Yu et al., 2020; Li et al., 2022) (Supplementary Table S6).
In all studies (Dong et al., 2020; Lang et al., 2020; Yu et al., 2020; Zhang et al., 2020; Li et al., 2022), the alteration of gut microbiota was evaluated at the genus level. Compared with HCs, two studies indicated that the abundance of Streptococcus was increased in IMN (Dong et al., 2020; Li et al., 2022), and three studies reported that the abundance of Lachnospira was decreased (Dong et al., 2020; Zhang et al., 2020; Li et al., 2022). One study also discovered that Streptococcus was similarly amplified when compared with DKD patients (Yu et al., 2020). The abundance of Peptostreptococcaceae_incertae_sedis was enriched in IMN in one study compared with HCs (Dong et al., 2020), and was also expanded when compared with DKD patients (Yu et al., 2020)). In addition, the abundance of Clostridium_sensu_stricto_1 (Dong et al., 2020), Veillonella (Dong et al., 2020), and Faecalibacterium (Li et al., 2022) was reduced in IMN patients compared with HCs, while it was increased compared to DKD patients (Yu et al., 2020) (Supplementary Table S7).
According to the phylogenetic profile, the decrease in abundance of the genus Lachnospira was probably the most critical reason for the depletion of the phylum Firmicutes. The increase in Streptococcus did not reverse the decline in Firmicutes, suggesting that the change in Streptococcus may not be that large or that there may be a decrease in other undetected bacteria at the genus level. In addition, the abundance of the phylum Proteobacteria significantly increased, but no bacterial genus belonging to it with the same change was found (Figure 3).
FIGURE 3. Alterations of gut microbiota at the phylum, family, and genus levels in IMN patients. (A) Alterations of gut microbiota in IMN patients compared with HCs; (B) alterations of gut microbiota in IMN patients relative to HCs and DKD. IMN, idiopathic membranous nephropathy; HCs, healthy controls; DKD, diabetic kidney disease.
Two studies (Yu et al., 2020; Li et al., 2022) analyzed the metabolic characteristics of the gut microbiota in patients with IMN (Supplementary Table S8). Wei Yu et al. (Yu et al., 2020) used LEfSe analysis to determine the crucial functional differences between DKD patients and patients with IMN according to the KEGG classifications, and found overexpression of membrane transporters involving the ABC transporters and the phosphotransferase system (PTS) in the microbiome of patients with IMN. Li et al. (Li et al., 2022) found that the KEGG pathway enrichment included alpha-linolenic acid metabolism, Staphylococcus aureus infection, and the arachidonic acid metabolic pathway in IMN patients compared to HCs. These results may represent functional differences in gut microbiota between IMN patients and healthy individuals.
To our knowledge, this is the first systematic review and meta-analysis to comprehensively analyze the profile of gut microbiota in patients with IMN and explore the characteristics of the gut microbiota that may be closely related to the occurrence and development of IMN. We observed that the results of the Chao1 index analysis, which represents community richness, showed meaningful distinctions in alpha diversity. Significant alterations in beta diversity of the gut microbiota were also observed in IMN patients. At the phylum level, the abundance of Proteobacteria increased, while that of Firmicutes decreased. At the genus level, the number of Lachnospira was depleted, while Streptococcus was enriched.
Alpha diversity is a commonly used metric in studies involving gut microbiota. Since reduced diversity is generally considered to be a manifestation of weakened health in the host or outright disease (Shade, 2017), we expected that patients with IMN would have reduced gut microbial diversity, as observed in many diseases (Scher et al., 2015). However, our study only showed reduction in the Chao1 index data for alpha diversity in patients with IMN, indicating that although the abundance of certain bacterial species was somewhat reduced, the overall diversity was preserved. Zhao H et al. (Zhao et al., 2021) analyzed the changes in gut microbiota alpha diversity in IMN rats and found that gut microbiota richness and diversity were both decreased, which is inconsistent with our results. If confounding factors such as specimen storage and testing methods are excluded, the differences in results may be related to differences in disease severity. Also, quantitative analysis may produce false-negative results due to the relatively low number of studies included. In gut microbiota, the beta diversity index is used to measure the rate of species diversity change along environmental gradients and intercommunity diversity. The significant alteration in beta diversity revealed that the gut microbial community in IMN patients was altered; therefore, we consider that gut dysbiosis was present in patients with IMN.
Further analysis revealed significant expansion of Proteobacteria in patients with IMN. A previous study found that Proteobacteria was also increased in patients with DKD (He et al., 2022). Thus, we believe that, as with DKD, the increase in Proteobacteria in IMN patients was meaningful and may indicate an intestinal inflammatory response and intestinal epithelial cell dysfunction (Litvak et al., 2017). The impaired intestinal epithelial function could cause further systemic inflammation, which would accelerate the development of kidney disease and result in more damage (Pahl and Vaziri, 2015). For example, lipopolysaccharide (LPS) derived from Escherichia coli (E. coli), which belongs to the phylum Proteobacteria, can induce the production of the cytokines tumor necrosis factor α (TNF-α), interleukin-1β (IL-1β), and interleukin-6 (IL-6) through the TLR4–NF-κB and TLR4–p38MAPK pathways, resulting in an inflammatory response (Qiu et al., 2021). Polymorphisms in the Toll-like receptor 4 (TLR4) gene and the IL-6 gene were associated with IMN morbidity (Liu et al., 2019). Moreover, genome-wide association studies (GWAS) in IMN patients revealed that a site on the NF-κB1 gene was associated with disease risk (Xie et al., 2020). Therefore, we speculated that Proteobacteria might be involved in the pathogenesis of IMN by upregulating expression of the TLR4, IL-6 and NF-κB1 genes. Secretory immunoglobulin A (sIgA), found in the intestinal mucosal immune barrier, is secreted by B cells induced by symbiotic microorganisms penetrating Peyer’s patches. This induction can produce a potent mucosal immune response including neutralizing endotoxins and enveloping bacteria to prevent pathogen invasion and maintain gut microbial homeostasis through the NF-κB pathway to reduce the inflammatory response (Pietrzak et al., 2020). By analyzing the correlation between gut microbiota and fecal sIgA in patients with early IMN, the change in E. coli may be linked abnormalities in sIgA, thus leading to an increase in intestinal permeability (Wang, 2017). Destruction of the intestinal mucosal barrier can result in the systemic circulation of bacteria and bacterial products and promote the accumulation of a large number of metabolic wastes in the body, especially indoxyl sulfate (IS), p-cresol sulfate (PCS) and trimethylamine oxide (TMAO) (Vaziri et al., 2016). IS can increase the secretion of TNF-α, IL-6 and other cytokines, thus stimulating B cells to produce IgG antibodies that participate in the pathogenesis of IMN (Liu et al., 2018). Moreover, IS, PCS and TMAO induced the expression of transforming growth factor β1 (TGF-β1) (Simona et al., 2017) and promoted the epithelial-mesenchymal transition (EMT) (Geng et al., 2020), leading to renal injury (Figure 4). Multiple cytokines, including TNF-α and IL-1β were also found to be elevated in untreated celiac patients compared with HCs and treated patients (Boonpheng et al., 2018). Celiac disease is an inflammatory disease of the small intestine, and renal insufficiency, including membranous nephropathy, is one of its known manifestations (Hamla and Ubels, 2007). Tacrolimus is a commonly used drug for the treatment of IMN. It interferes with T cell-induced activation of TNF-α, IL-1β and IL-6 by inhibiting calcineurin, thereby blocking the immune response and delaying the progression of renal injury (Tanaka et al., 2012). As a macrolide antibiotic, tacrolimus can also alter the gut microbiota, which is one of the possible mechanisms of its immunosuppressive effect. Further studies may focus on the prognosis of IMN by observing the effect of different treatment regimens on changes in gut dysbiosis, for monitoring efficacy and adjusting medication. Salmonella also belongs to the phylum Proteobacteria and can reduce the number of IgG-secreting plasma cells in the bone marrow, resulting in a decrease in serum IgG titer and immune complex formation (Manne et al., 2019). In summary, Proteobacteria is closely associated with IMN, but the mechanism remains unclear. As screening for Proteobacteria in patients with IMN at the genus level has not been reported thus far, more studies are needed to identify changes in Proteobacteria at the species level.
FIGURE 4. Possible mechanisms associated with gut dysbiosis and effects of host immunity on gut microbiota in IMN patients. Alterations in the specific microbiome may be involved in the pathogenesis and development of IMN through depletion of SCFAs and sIgA, increased cytokines (TNF-α, IL-1β), uremic toxins (PCS, IS, TMAO), and common antigens (SPeB) with the glomerular basement membrane. The host immune system can regulate the gut microbiota homeostasis through the production of sIgA and the induction of Ang. IMN, idiopathic membranous nephropathy; sIgA, secretory immunoglobulin A; TMAO, trimethylamine N-oxide; PCS, p-cresol sulfate; IS, indoxyl sulfate; IL, interleukin; TNF-α, tumor necrosis factor-α; TGF-β, transforming growth factor-β; Treg, regulatory T cells; EMT, epithelial-mesenchymal transition; SPeB, streptococcal pyrogenic exotoxin B; PLA2R, phospholipase A2 receptor; THSD7A, thrombospondin type 1 domain-containing 7A; EXT1/EXT2, exostosin 1/exostosin 2; NELL-1, neural EGF-like-1 protein; Sema3B, semaphorin 3B; PCDH7, protocadherin 7; HTRA1, high-temperature requirement A serine peptidase 1; Ang, angiogenin.
The abundance of the phylum Firmicutes and genus Lachnospira was significantly decreased in patients with IMN. A previous study with high-throughput sequencing of the fecal microbiome of CKD patients and HCs found that Lachnospira was significantly reduced in CKD patients, which is a good microbial marker (Lun et al., 2019). Firmicutes are bacteria that produce a large amount of butyric acid (Jandhyala et al., 2015), and Lachnospira is an obligate anaerobic bacterium that decomposes carbohydrates to produce short-chain fatty acids (SCFAs) (Vacca et al., 2020). Jun Zhang et al. (Zhang et al., 2020) analyzed fecal samples from IMN and control groups for organic acids and found that the SCFAs, propionic acid and butyric acid, were significantly lower in patients with IMN than in healthy controls. In addition, in a study analyzing the fecal flora of IMN rats, it was discovered that the numbers of bacteria producing butyric acid in the intestinal tract were lower than in control rats (Wang et al., 2022). These findings suggested that the decreased abundance of Lachnospira may result in lower production of SCFAs. Alteration of the Th17/Treg ratio is a possible mechanism for the pathogenesis of IMN (Motavalli et al., 2021), because SCFAs derived from microbial metabolites regulate Treg cell homeostasis, which has been proven to be critical for the health of gut microbiota and maintaining the integrity of the intestinal epithelial cells that inhibit invasion of gut microbiota (Furusawa et al., 2013). Treg cells were also found to be essential for autoantigen tolerance and the prevention of autoimmune diseases (Takahashi et al., 2020) Administration of SCFAs to mice can increase the differentiation of thymus-derived T-cells into peripheral Treg cells (Arpaia et al., 2013). Thus, lower production of SCFAs resulted in reduced production and differentiation of Treg cells, leading to lower production of the anti-inflammatory cytokine interleukin-10 (IL-10) and impaired anti-inflammatory effects (Proto et al., 2018) (Figure 4). The abovementioned results may be part of the mechanism by which Lachnospira is involved in the progression of IMN, and the intervention of Lachnospira and SCFAs may be a promising new direction in the treatment of IMN.
The induction of antimicrobial peptides (AMPs) is one of the mechanisms by which the host immune system establishes intestinal homeostasis of symbiotic microbes (Mami et al., 2016). As an AMP, angiogenin (Ang), secreted by Paneth cells, directly inhibits α -Proteobacteria strains by destroying the integrity of the bacterial membrane to balance the numbers of α-Proteobacteria and Lachnospiraceae and maintain homeostasis of the gut microbiota. Ang1 deficiency in mice resulted in expansion of α-Proteobacteria and reduction of protective intestinal symbiotic strains such as Lachnospiraceae (Sun et al., 2021). Ang1 is also an endothelial factor produced by podocytes and is involved in the regulation and maintenance of glomerular basement membrane (GBM) permeability (Satchell et al., 2004). Therefore, we hypothesized that damage to podocytes in IMN patients may reduce production of Ang1, resulting in characteristic disturbances of gut microbiota. Administration of Ang1 may be a promising intervention to treat IMN, although the mechanism of its action has not yet been reported, and further experiments are needed to test this hypothesis.
Streptococcus was observed to be elevated in patients with IMN but not HCs or DKD patients. Interestingly, an increased abundance of Streptococcus was also found in the salivary microbiota of IMN patients (Luan et al., 2022). LPS from Streptococcus can enter the blood through the damaged intestinal where it activates the NF-κB pathway and stabilizes hypoxia-inducible factor-1α, which triggers the release of inflammatory factors such as IL-6 and IL-1β, thus promoting the occurrence of inflammatory disease (Tannahill et al., 2013; Ramezani and Raj, 2014; Manfredo Vieira et al., 2018). IL-1β can promote Th17 cell responses (Park et al., 2017), which are involved in autoimmune diseases such as inflammatory bowel disease (IBD). It has been reported that the diversity and abundance of gut microbiota in IBD patients is highly consistent with the changes in gut microbiota in IMN patients (Nishida et al., 2018). Furthermore, streptococcal pyrogenic exotoxin B (SPeB) is a common antigen of the GBM, and antibodies generated by the body against Streptococcus may react with the GBM, resulting in deposition of immune complexes in the GBM and damage to the basement membrane (Balasubramanian and Marks, 2017). Streptococcus has also been significantly associated with a number of other kidney diseases. For example, Streptococcus was increased in Henoch-Schonlein purpura nephritis and disease severity was proportional to its abundance (Tan et al., 2022). Intravenous administration of Streptococcus mutans to rats can cause the transient induction of lesions similar to those from IgA nephropathy (Naka et al., 2020). Consequently, the amplification of Streptococcus may be involved in the progression of kidney injury through these possible mechanisms.
ABC transporters participate in the biogenesis of the outer membrane of gram-negative bacteria and mediate the separation of LPS from the inner membrane (Narita, 2011; Alexander et al., 2018). The stimulation of TLR4 by LPS derived from gram-negative bacteria induces the production of crucial pro-inflammatory cytokines and then activates the host immune response (Cochet and Peri, 2017). It was demonstrated that gut dysbiosis may be involved in IMN pathogenesis by inducing TLR4 gene expression. In another way, the expansion of ABC membrane transporters may mean an increase in bacteria such as E. coli that respond to LPS-mediated inflammation. More studies are needed to explore the metabolic function of gut bacteria in patients with IMN.
IMN is one of the most common pathological types of CKD, and its incidence has been increasing in recent years (Tang et al., 2017). In patients with CKD, membrane nephropathy is correlated with consistent changes in specific bacteria at the phylum and genus level, including an increase in Proteobacteria and Streptococcus and a reduction in Firmicutes and Lachnospira (Lun et al., 2019; Zhao J. et al., 2021). The abovementioned studies examined the changes in gut microbiota in patients with IMN, which may provide a more accurate basis for further studies to discover or verify specific bacterial taxa at the genus and species levels that are closely related to IMN. The identical results of the alteration in patients with CKD and IMN may be reasonable but the correlation is unclear. Further studies are needed to explore the changes in gut microbiota in patients with different pathological types of CKD to better determine the characteristic alterations of gut microbiota at the species level.
Microbiome-based precision medicine targeting specific alterations in gut microbiota in patients with IMN, including fecal microbial transplantation (FMT), prebiotics, probiotics, antibiotics, vaccines, and dietary immune stimulation products, may be a more selective and safer therapy for inhibiting the aberrant immune response and reducing kidney injury in patients with IMN. FMT has been applied to treat IMN patients and has shown promising efficacy, with alleviation of symptoms and improvement of renal function (Zhou et al., 2021). High-quality clinical studies are needed to validate the efficacy and potential mechanisms of microbial therapy in the future. In addition, whether gut dysbiosis involving specific strains are a cause or result of IMN remains undetermined, which warrants further investigation in well-designed interventional clinical trials or animal experiments.
This systematic review and meta-analysis still has some limitations. First, we did not evaluate publication bias due to the small number of included studies. Second, the selected studies did not distinguish the disease severity of patients, which needs to be addressed in future research. Third, the original studies were all performed in China, and caution must be exercised in applying these findings to non-Chinese populations. Therefore, high quality and multiregional, multicenter clinical studies are still needed to verify our findings.
This review reveals that the beta diversity is changed and the richness is reduced in the gut microbiota of IMN patients. The alternation of gut microbiota structure in patients with IMN was characterized by augmentation of the phylum Proteobacteria, while depletion of butyric acid-producing bacteria mainly included the phylum Firmicutes and the genus Lachnospira. Notably, the genus Lachnospira was markedly depleted and, thus, may be closely related to the occurrence of IMN, which needs further exploration.
All data obtained or scrutinized in this study is contained in this review and in Supplementary Material. Further queries can be addressed to the corresponding author.
Study design: YZ and SS. Data acquisition and analysis: YZ, JZ, YQ, YW, and ZY. Manuscript modification: SS and XN. Each author played a critical role in preparing the manuscript, providing accountability, and guaranteeing that the correctness or thoroughness of the overall work is sufficient.
National Natural Science Foundation of China grants (Reference number: 82170722 and 81870470), Key project of Shaanxi province (Reference number: 2017ZDXM-SF-045), and Clinical research project of Air Force Military Medical University (Reference number: 202ILC2205) supported this study.
The authors sincerely thank all the scholars who participated in this study for their support and contributions.
The authors declare that the research was conducted in the absence of any commercial or financial relationships that could be construed as a potential conflict of interest.
All claims expressed in this article are solely those of the authors and do not necessarily represent those of their affiliated organizations, or those of the publisher, the editors and the reviewers. Any product that may be evaluated in this article, or claim that may be made by its manufacturer, is not guaranteed or endorsed by the publisher.
The Supplementary Material for this article can be found online at: https://www.frontiersin.org/articles/10.3389/fphys.2022.909491/full#supplementary-material
Alexander M., Miu A., Oh A., Reichelt M., Ho H., Chalouni C. (2018). Disrupting gram-negative bacterial outer membrane biosynthesis through inhibition of the lipopolysaccharide transporter MsbA. Antimicrob. Agents Chemother. 62 (11), e01142–e01118. doi:10.1128/AAC.01142-18
Arpaia N., Campbell C., Fan X., Dikiy S., Veeken J., deRoos P. (2013). Metabolites produced by commensal bacteria promote peripheral regulatory T-cell generation. Nature 504 (7480), 451–455. doi:10.1038/nature12726
Balasubramanian R., Marks S. (2017). Post-infectious glomerulonephritis. Paediatr. Int. Child. Health 37 (4), 240–247. doi:10.1080/20469047.2017.1369642
Boonpheng B., Cheungpasitporn W., Wijarnpreecha K. (2018). Renal disease in patients with celiac disease. Minerva Med. 109 (2), 126–140. doi:10.23736/S0026-4806.17.05403-9
Cochet F., Peri F. (2017). The role of carbohydrates in the lipopolysaccharide (LPS)/Toll-Like receptor 4 (TLR4) signalling. Int. J. Mol. Sci. 18 (11), E2318. doi:10.3390/ijms18112318
Couser W. G. (2017). Primary membranous nephropathy. Clin. J. Am. Soc. Nephrol. 12 (6), 983–997. doi:10.2215/CJN.11761116
Cremoni M., Brglez V., Perez S., Decoupigny F., Zorzi K., Andreani M., et al. (2020). Th17-Immune response in patients with membranous nephropathy is associated with thrombosis and relapses. Front. Immunol. 11, 574997. doi:10.3389/fimmu.2020.574997
Davenport E., R., Sanders J., G., Song S. J., Amato K., R., Clark A., G., Knight R. (2017). The human microbiome in evolution. BMC Biol. 15 (1), 127. doi:10.1186/s12915-017-0454-7
Dong R., Bai M., Zhao J., Wang D., Ning X., Sun S. (2020). A comparative study of the gut microbiota associated with immunoglobulin a nephropathy and membranous nephropathy. Front. Cell. Infect. Microbiol. 10, 557368. doi:10.3389/fcimb.2020.557368
Dore E., Joly-Beauparlant C., Morozumi S., Mathieu A., Levesque T., Allaeys I., et al. (2022). The interaction of secreted phospholipase A2-IIA with the microbiota alters its lipidome and promotes inflammation. JCI Insight 7 (2), e152638. doi:10.1172/jci.insight.152638
Drevon D., Fursa S. R., Malcolm A. L. (2017). Intercoder reliability and validity of WebPlotDigitizer in extracting graphed data. Behav. Modif. 41 (2), 323–339. doi:10.1177/0145445516673998
Furusawa Y., Obata Y., Fukuda S., Endo T. A., Nakato G., Takahashi D., et al. (2013). Commensal microbe-derived butyrate induces the differentiation of colonic regulatory T cells. Nature 504 (7480), 446–450. doi:10.1038/nature12721
Geng X. Q., Ma A., He J. Z., Wang L., Jia Y. L., Shao G. Y., et al. (2020). Ganoderic acid hinders renal fibrosis via suppressing the TGF-β/Smad and MAPK signaling pathways. Acta Pharmacol. Sin. 41 (5), 670–677. doi:10.1038/s41401-019-0324-7
Hamla C., Ubels F. (2007). Celiac sprue-associated membranous nephropathy. Clin. Nephrol. 68 (3), 197. doi:10.5414/cnp68197
He X., Sun J., Liu C., Yu X., Li H., Zhang W., et al. (2022). Compositional alterations of gut microbiota in patients with diabetic kidney disease and type 2 diabetes mellitus. Diabetes Metab. Syndr. Obes. 15, 755–765. doi:10.2147/DMSO.S347805
Ivanov I. I., Tuganbaev T., Skelly A. N., Honda K. (2022). T cell responses to the microbiota. Annu. Rev. Immunol. 40 (1), 559–587. doi:10.1146/annurev-immunol-101320-011829
Jandhyala S. M., Talukdar R., Subramanyam C., Vuyyuru H., Sasikala M., Nageshwar Reddy D. (2015). Role of the normal gut microbiota. World J. Gastroenterol. 21 (29), 8787–8803. doi:10.3748/wjg.v21.i29.8787
Lang R., Wang X. H., Li A. F., Liang Y., Zhu B. C., Shi B., et al. (2020). Effects of jian pi qu shi formula on intestinal bacterial flora in patients with idiopathic membranous nephropathy: A prospective randomized controlled trial. Chronic Dis. Transl. Med. 6 (2), 124–133. doi:10.1016/j.cdtm.2020.04.004
Li H., Limenitakis J. P., Greiff V., Yilmaz B., Scharen O., Urbaniak C., et al. (2020). Mucosal or systemic microbiota exposures shape the B cell repertoire. Nature 584 (7820), 274–278. doi:10.1038/s41586-020-2564-6
Li M., Wei L., Sun J., Zhu Q., Yang H., Zhang Y., et al. (2022). Association of gut microbiota with idiopathic membranous nephropathy. BMC Nephrol. 23 (1), 164. doi:10.1186/s12882-022-02797-5
Litvak Y., Byndloss M. X., Tsolis R. M., Bäumler A. J. (2017). Dysbiotic proteobacteria expansion: A microbial signature of epithelial dysfunction. Curr. Opin. Microbiol. 39, 1–6. doi:10.1016/j.mib.2017.07.003
Liu D., Zhang J., Shi Y., Liu Z. (2019). Gene polymorphism and risk of idiopathic membranous nephropathy. Life Sci. 229, 124–131. doi:10.1016/j.lfs.2019.05.010
Liu W. C., Tomino Y., Lu K. C. (2018). Impacts of indoxyl sulfate and p-cresol sulfate on chronic kidney disease and mitigating effects of AST-120. Toxins (Basel) 10 (9), 367. doi:10.3390/toxins10090367
Luan S., Zhang S., Pan L., Hu W., Cui H., Wei X., et al. (2022). Salivary microbiota analysis of patients with membranous nephropathy. Mol. Med. Rep. 25 (5), 190. doi:10.3892/mmr.2022.12706
Lun H., Yang W., Zhao S., Jiang M., Xu M., Liu F., et al. (2019). Altered gut microbiota and microbial biomarkers associated with chronic kidney disease. Microbiologyopen 8 (4), e00678. doi:10.1002/mbo3.678
Mami I., Bouvier N., El Karoui K., Gallazzini M., Rabant M., Laurent-Puig P., et al. (2016). Angiogenin mediates cell-autonomous translational control under endoplasmic reticulum stress and attenuates kidney injury. J. Am. Soc. Nephrol. 27 (3), 863–876. doi:10.1681/ASN.2015020196
Manfredo Vieira S., Hiltensperger M., Kumar V., Zegarra-Ruiz D., Dehner C., Khan N., et al. (2018). Translocation of a gut pathobiont drives autoimmunity in mice and humans. Science 359 (6380), 1156–1161. doi:10.1126/science.aar7201
Manne C., Takaya A., Yamasaki Y., Mursell M., Hojyo S., Wu T. Y., et al. (2019). Salmonella SiiE prevents an efficient humoral immune memory by interfering with IgG(+) plasma cell persistence in the bone marrow. Proc. Natl. Acad. Sci. U. S. A. 116 (15), 7425–7430. doi:10.1073/pnas.1818242116
Motavalli R., Etemadi J., Soltani-Zangbar M. S., Ardalan M. R., Kahroba H., Roshangar L., et al. (2021). Altered Th17/Treg ratio as a possible mechanism in pathogenesis of idiopathic membranous nephropathy. Cytokine 141, 155452. doi:10.1016/j.cyto.2021.155452
Naka S., Wato K., Misaki T., Ito S., Nagasawa Y., Nomura R., et al. (2020). Intravenous administration of Streptococcus mutans induces IgA nephropathy-like lesions. Clin. Exp. Nephrol. 24 (12), 1122–1131. doi:10.1007/s10157-020-01961-1
Narita S. (2011). ABC transporters involved in the biogenesis of the outer membrane in gram-negative bacteria. Biosci. Biotechnol. Biochem. 75 (6), 1044–1054. doi:10.1271/bbb.110115
Nishida A., Inoue R., Inatomi O., Bamba S., Naito Y., Andoh A. (2018). Gut microbiota in the pathogenesis of inflammatory bowel disease. Clin. J. Gastroenterol. 11 (1), 1–10. doi:10.1007/s12328-017-0813-5
Pahl M. V., Vaziri N. D. (2015). The chronic kidney disease - colonic Axis. Semin. Dial. 28 (5), 459–463. doi:10.1111/sdi.12381
Park S. H., Kim M. S., Lim H. X., Cho D., Kim T. S. (2017). IL-33-matured dendritic cells promote Th17 cell responses via IL-1β and IL-6. Cytokine 99, 106–113. doi:10.1016/j.cyto.2017.07.022
Patrick H. S., Mitra A., Rosen T., Ananth C. V., Schuster M. (2020). Pharmacologic intervention for the management of retained placenta: A systematic review and meta-analysis of randomized trials. Am. J. Obstet. Gynecol. 223 (3), e419–e441. doi:10.1016/j.ajog.2020.06.044
Pietrzak B., Tomela K., Olejnik-Schmidt A., Mackiewicz A., Schmidt M. (2020). Secretory IgA in intestinal mucosal secretions as an adaptive barrier against microbial cells. Int. J. Mol. Sci. 21 (23), E9254. doi:10.3390/ijms21239254
Proto J. D., Doran A. C., Gusarova G., Yurdagul A., Sozen E., Subramanian M., et al. (2018). Regulatory T cells promote macrophage efferocytosis during inflammation resolution. Immunity 49 (4), 666–677. doi:10.1016/j.immuni.2018.07.015
Qiu C., Yuan Z., He Z., Chen H., Liao Y., Li S., et al. (2021). Lipopolysaccharide preparation derived from Porphyromonas gingivalis induces a weaker immuno-inflammatory response in BV-2 microglial cells than Escherichia coli by differentially activating TLR2/4-mediated NF-κB/STAT3 signaling pathways. Front. Cell. Infect. Microbiol. 11, 606986. doi:10.3389/fcimb.2021.606986
Ramezani A., Raj D. S. (2014). The gut microbiome, kidney disease, and targeted interventions. J. Am. Soc. Nephrol. 25 (4), 657–670. doi:10.1681/ASN.2013080905
Ronco P., Beck L., Debiec H., Fervenza F. C., Hou F. F., Jha V., et al. (2021). Membranous nephropathy. Nat. Rev. Dis. Prim. 7 (1), 69. doi:10.1038/s41572-021-00303-z
Ronco P., Debiec H. (2020). Molecular pathogenesis of membranous nephropathy. Annu. Rev. Pathol. 15, 287–313. doi:10.1146/annurev-pathol-020117-043811
Satchell S. C., Anderson K. L., Mathieson P. W. (2004). Angiopoietin 1 and vascular endothelial growth factor modulate human glomerular endothelial cell barrier properties. J. Am. Soc. Nephrol. 15 (3), 566–574. doi:10.1097/01.asn.0000115397.22519.03
Scher J. U., Ubeda C., Artacho A., Attur M., Isaac S., Reddy S. M., et al. (2015). Decreased bacterial diversity characterizes the altered gut microbiota in patients with psoriatic arthritis, resembling dysbiosis in inflammatory bowel disease. Arthritis Rheumatol. 67 (1), 128–139. doi:10.1002/art.38892
Sethi S. (2021). New 'antigens' in membranous nephropathy. J. Am. Soc. Nephrol. 32 (2), 268–278. doi:10.1681/ASN.2020071082
Shade A. (2017). Diversity is the question, not the answer. ISME J. 11 (1), 1–6. doi:10.1038/ismej.2016.118
Simona A., Tim M., Salvatore C., Michela C., Björn R., Orlando P., et al. (2017). Indoxyl sulfate affects glial function increasing oxidative stress and neuroinflammation in chronic kidney disease: Interaction between astrocytes and microglia. Front. Pharmacol. 8, 370. doi:10.3389/fphar.2017.00370
Stang A. (2010). Critical evaluation of the Newcastle-Ottawa scale for the assessment of the quality of nonrandomized studies in meta-analyses. Eur. J. Epidemiol. 25 (9), 603–605. doi:10.1007/s10654-010-9491-z
Sun D., Bai R., Zhou W., Yao Z., Liu Y., Tang S., et al. (2021). Angiogenin maintains gut microbe homeostasis by balancing α-Proteobacteria and Lachnospiraceae. Gut 70 (4), 666–676. doi:10.1136/gutjnl-2019-320135
Takahashi D., Hoshina N., Kabumoto Y., Maeda Y., Suzuki A., Tanabe H., et al. (2020). Microbiota-derived butyrate limits the autoimmune response by promoting the differentiation of follicular regulatory T cells. EBioMedicine 58, 102913. doi:10.1016/j.ebiom.2020.102913
Tan J., Zhong Z., Tang Y., Qin W. (2022). Intestinal dysbiosis featuring abundance of Streptococcus associates with Henoch-Schonlein purpura nephritis (IgA vasculitis with nephritis) in adult. BMC Nephrol. 23 (1), 10. doi:10.1186/s12882-021-02638-x
Tanaka H., Tsuruga K., Aizawa-Yashiro T., Watanabe S., Imaizumi T. (2012). Treatment of young patients with lupus nephritis using calcineurin inhibitors. World J. Nephrol. 1 (6), 177–183. doi:10.5527/wjn.v1.i6.177
Tang L., Yao J., Kong X., Sun Q., Wang Z., Zhang Y., et al. (2017). Increasing prevalence of membranous nephropathy in patients with primary glomerular diseases: A cross-sectional study in China. Nephrol. Carlt. 22 (2), 168–173. doi:10.1111/nep.12739
Tannahill G. M., Curtis A. M., Adamik J., Palsson-McDermott E. M., McGettrick A. F., Goel G., et al. (2013). Succinate is an inflammatory signal that induces IL-1β through HIF-1α. Nature 496 (7444), 238–242. doi:10.1038/nature11986
Vacca M., Celano G., Calabrese F. M., Portincasa P., Gobbetti M., De Angelis M. (2020). The controversial role of human gut Lachnospiraceae. Microorganisms 8 (4), 573–597. doi:10.3390/microorganisms8040573
Vaziri N. D., Zhao Y. Y., Pahl M. V. (2016). Altered intestinal microbial flora and impaired epithelial barrier structure and function in CKD: The nature, mechanisms, consequences and potential treatment. Nephrol. Dial. Transpl. 31 (5), 737–746. doi:10.1093/ndt/gfv095
Wang J. L. (2017). The investigation of intestinal flora and SIgA in the initial IMN patients and its significance. Master (Shijiazhuang, China: Hebei Medical University).
Wang X. H., Yang Y. N., Liang Y., Lang R., Zeng Q., Yan L., et al. (2022). Structural modulation of gut microbiota during alleviation of experimental passive Heymann nephritis in rats by a traditional Chinese herbal formula. Biomed. Pharmacother. 145, 112475. doi:10.1016/j.biopha.2021.112475
Xie J., Liu L., Mladkova N., Li Y., Ren H., Wang W., et al. (2020). The genetic architecture of membranous nephropathy and its potential to improve non-invasive diagnosis. Nat. Commun. 11 (1), 1600. doi:10.1038/s41467-020-15383-w
Yu W., Shang J., Guo R., Zhang F., Zhang W., Zhang Y., et al. (2020). The gut microbiome in differential diagnosis of diabetic kidney disease and membranous nephropathy. Ren. Fail. 42 (1), 1100–1110. doi:10.1080/0886022X.2020.1837869
Zhang J., Luo D., Lin Z., Zhou W., Rao J., Li Y., et al. (2020). Dysbiosis of gut microbiota in adult idiopathic membranous nephropathy with nephrotic syndrome. Microb. Pathog. 147, 104359. doi:10.1016/j.micpath.2020.104359
Zhao H., Chen C., Zhao Y., Tang W., Gao Q., Kong L., et al. (2021). Effect of polysaccharides from plantaginis semen on renal injury and gut microbiota in rats with membranous nephropathy. Chin. J. Exp. Traditional Med. Formulae 27 (22), 92–99. doi:10.13422/j.cnki.syfjx.20212101
Zhao J., Ning X., Liu B., Dong R., Bai M., Sun S. (2021a). Specific alterations in gut microbiota in patients with chronic kidney disease: An updated systematic review. Ren. Fail. 43 (1), 102–112. doi:10.1080/0886022X.2020.1864404
Zhao Q., Dai H., Liu X., Jiang H., Liu W., Feng Z., et al. (2021b). Helper T cells in idiopathic membranous nephropathy. Front. Immunol. 12, 665629. doi:10.3389/fimmu.2021.665629
Keywords: gut microbiota, membranous nephropathy, kidney disease, meta-analysis, systematic review
Citation: Zhang Y, Zhao J, Qin Y, Wang Y, Yu Z, Ning X and Sun S (2022) Specific alterations of gut microbiota in patients with membranous nephropathy: A systematic review and meta-analysis. Front. Physiol. 13:909491. doi: 10.3389/fphys.2022.909491
Received: 12 April 2022; Accepted: 03 October 2022;
Published: 01 November 2022.
Edited by:
Eugenia Bezirtzoglou, Democritus University of Thrace, GreeceReviewed by:
Mingsong Kang, Canadian Food Inspection Agency (CFIA), CanadaCopyright © 2022 Zhang, Zhao, Qin, Wang, Yu, Ning and Sun. This is an open-access article distributed under the terms of the Creative Commons Attribution License (CC BY). The use, distribution or reproduction in other forums is permitted, provided the original author(s) and the copyright owner(s) are credited and that the original publication in this journal is cited, in accordance with accepted academic practice. No use, distribution or reproduction is permitted which does not comply with these terms.
*Correspondence: Shiren Sun, c3Vuc2hpcmVuQG1lZG1haWwuY29tLmNu
†These authors have contributed equally to this work and share first authorship
Disclaimer: All claims expressed in this article are solely those of the authors and do not necessarily represent those of their affiliated organizations, or those of the publisher, the editors and the reviewers. Any product that may be evaluated in this article or claim that may be made by its manufacturer is not guaranteed or endorsed by the publisher.
Research integrity at Frontiers
Learn more about the work of our research integrity team to safeguard the quality of each article we publish.