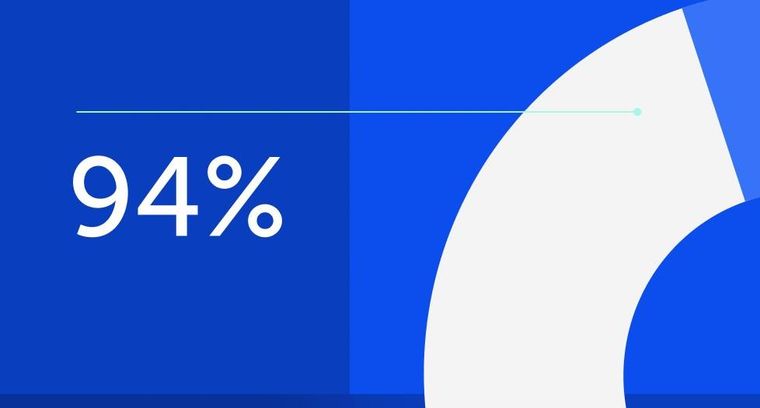
94% of researchers rate our articles as excellent or good
Learn more about the work of our research integrity team to safeguard the quality of each article we publish.
Find out more
ORIGINAL RESEARCH article
Front. Physiol., 08 July 2022
Sec. Exercise Physiology
Volume 13 - 2022 | https://doi.org/10.3389/fphys.2022.909086
A correction has been applied to this article in:
Corrigendum: Relationship between metabolites of vitamin D, free 25-(OH)D, and physical performance in indoor and outdoor athletes
The potential effects of vitamin D in athletes have received considerable attention in the literature. However, little is known about vitamin D metabolites and their association with physical performance in athletes. Therefore, the aim of our study was to determine the relationship between metabolites of vitamin D, vitamin D binding protein (VDBP), free, bioavailable 25-(OH)D, and physical fitness tests in athletes. A total of 40 indoor and outdoor players (16 judoists and 24 football players) participated in the study. Vitamin D metabolites (25-(OH)D, 24,25-(OH)2D3, 3-epi-25-(OH)D3, and 1,25-(OH)2D) were assessed using LM-MS/MS. Free 25-(OH)D concentration was evaluated by calculation using serum albumin and VDBP levels. Athletic performance was assessed using handgrip and vertical jump. Our study showed a significant correlation between vitamin D metabolites and handgrip strength and vertical jump variables in indoor players. It demonstrated a significant association between 3-epi-25-(OH)D3 and vertical jump parameters in outdoor players. The results of our study showed relationship between free, bioavailable 25-(OH)D, and vertical jump variables in indoor players. In conclusion, we provide novel information on the vitamin D metabolites and athletic performance in athletes. Based on the results of our study, we concluded that vitamin D metabolites might be involved in skeletal muscle function in relation to athletic performance.
During the last few decades, interest has increased in vitamin D research because studies frequently evidence vitamin D deficiency/insufficiency among athletes worldwide (Valtueña et al., 2014; Krzywanski et al., 2016; Lombardi et al., 2017). Findings from a meta-analysis of 23 studies, on a total of 2,313 athletes, demonstrated that 56% of those practicing both indoor and outdoor activities had insufficient vitamin D concentrations (Farrokhyar et al., 2015). Further, for athletes living at latitudes above 40°N, the risk of vitamin D inadequacy significantly increases during wintertime (Farrokhyar et al., 2015).
Current studies indicate that vitamin D plays a role in skeletal muscle function. Calcitriol [1α,25-(OH)2D] stimulates the myogenic differentiation of mesenchymal stem cells and upregulates the insulin growth factor (IGF)-II and follistatin expression (Garcia et al., 2011). It downregulates, instead, IGF-I and myostatin protein expression (Di Luigi et al., 2020). 1α,25-(OH)2D can enhance the sensitization of calcium binding sites at the sarcoplasmic reticulum level (Girgis et al., 2013) and influence muscle cell growth and differentiation, particularly of the fast-twitch type II fibers (Halfon et al., 2015). In addition, vitamin D increases insulin sensitivity, energetic substrate metabolism, and oxidative capacity by regulating mitochondrial respiration (Antinozzi et al., 2017; Ashcroft et al., 2020). 1α,25-(OH)2D exerts different non-classic biological effects that are supposed to influence health status, exercise, and sport performance in athletes (Owens et al., 2018).
Recent advances in mass spectrometry (MS)-based detection of plasma metabolites have allowed for the accurate detection of 1,25-(OH)2D, 24,25-(OH)2D, a major catabolic metabolite of 25-(OH)D, and 3-epi-25-(OH)D. Although only calcitriol has defined biological activities, there is interest in the other vitamin D metabolites. For instance, studies involving animal models demonstrated that 24,25-(OH)2D exerts a crucial role in the maintenance of bone integrity, healing, and function (Seo et al., 1997; Seo and Norman, 1997). Furthermore, 24,25-(OH)2D modulates growth plate chondrocyte physiology and parathyroid gland function (Herrmann et al., 2017). In turn, 3-epi-25-(OH)D levels are directly associated with the cardiovascular risk profile, and 3-epi-1α,25(OH)2D, a derivative of 3-epi-25-(OH)D, effectively reduces blood parathyroid hormone (PTH) without affecting calcium levels (Brown et al., 2005; Lutsey et al., 2015). To our knowledge, there are only few studies, especially intervention ones, related to the status of different vitamin D metabolites (Hassan-Smith et al., 2017; Mieszkowski et al., 2020; Mieszkowski et al., 2021).
Circulating levels of calcidiol [25-(OH)D] are currently used as the indicator of vitamin D status (Bikle et al., 2017; Tsuprykov et al., 2018). Recently, there has been speculation as to whether just measuring total 25-(OH)D is appropriate for the assessment of vitamin D status in different physiological and pathophysiological conditions (Bikle et al., 2017). Around 85%–90% of the total circulating 25-(OH)D and 1α,25-(OH)2D is bound to vitamin D binding protein (VDBP). About 10%–15% is bound to albumin, in contrast to free 25-(OH)D [bioavailable 25-(OH)D], which accounts <1% of total circulating vitamin D (Bikle et al., 2017; Owens et al., 2018). According to the “free hormone” hypothesis (Chun et al., 2014), current studies have shown that only the non-bound fraction of vitamin D is able to enter cells and to exert biologic effects (Bikle and Schwartz, 2019). It has been documented that the bioavailable fraction of circulating 25-(OH)D displays a stronger association with bone mineral density (BMD) than total levels, in healthy adults (Powe et al., 2011), and with intact PTH levels (iPTH) (Shieh et al., 2016).
More recently, the relationships between total 25-(OH)D concentration and performance-related factors in athletes have been observed in many studies (Seo et al., 2019; Kim et al., 2020; Most et al., 2021; Wilson-Barnes et al., 2021). Although the importance of vitamin D for athletes in regard to health and performance was emphasized, the studies found inconsistent results. A main issue is represented by the fact that those studies did not consider the metabolites of vitamin D and the amount of free or bioavailable 25-(OH)D, important to muscle function. Considering the role of vitamin D in skeletal muscle and athletic performance, there is a need to assess such factors among this population. Therefore, the primary aim of our study was to assess circulating vitamin D metabolites, VDBP, free, bioavailable 25-(OH)D concentration in athletes by considering the behavior of their training, either indoor or outdoor. A secondary aim was to determine the relationship between metabolites of vitamin D, VDBP, free, bioavailable 25-(OH)D, and physical fitness tests in athletes.
Forty injury-free male athletes (16 judoists and 24 football players) were included in the study. All participants were Caucasians with white skin, and none of them was a regular sunbed user. None of the subjects used any food supplements containing vitamin D and calcium. Participants’ characteristics are shown in Table 1. The study was carried out in Wrocław (Poland), which is situated at the latitude of 51°10’ N. It was conducted during the general preparatory period in the 2nd Polish national football team and academic judo team. Athletes within sport discipline had similar exercise loads. Data were collected during 2 weeks at the turn of February and March 2021.
Measurements were performed under the fasting state. Height was measured by an anthropometer accurate to up to 1·10–3 m (GPM Siber Hegner Machinery Ltd., Zurich, Switzerland). The body mass was measured with the use of an electronic scale accurate up to 102 g (Fawag, Lublin, Poland).
Skinfold thickness was measured at seven sites (biceps, triceps, subscapular, suprailiac, abdominal, thigh, and calf) with a Harpenden skinfold calliper (British Indicators, Burgess Hill, United Kingdom). Each measurement was taken by the same person, 3 times, and the mean value was used for calculation. Percentage body fat was assessed using the formula (Withers et al., 1987; Siri, 1993).
Athletes were instructed to visit laboratory between 7.00 and 10.00 a.m., after 10-to-12-h fasting and 24-h abstinence from training. Blood samples were collected into plain tubes, containing a clot activator (Vacutest, Kima, Italy). Blood was kept at room temperature for 1 h and then centrifuged at 1300 g, for 10 min, at 22°C. Serum was aliquoted and stored at −80°C until assayed.
iPTH in blood serum was determined by the electro-chemiluminescence assay (ECLIA) on an Elecsys analyzer (Roche, Switzerland) according to the manufacturer’s protocol and specific assay laboratory quality control procedures. The intra- and inter-assay coefficients of variation (CVs) were 4.5% and 4.8%, respectively, and the limit of detection was at 1.20 pg/mL (0.127 pmol/L).
Total calcium (Ca) was determined, in serum, by a colorimetric assay using the Konelab 60 system from bioMérieux (France).
Albumin was assayed on a Siemens Dimension Xpand Plus clinical chemistry system (Siemens, Germany).
Albumin-adjusted calcium (ACa) was calculated using the formula
The vitamin D metabolite [25-(OH)D3, 25-(OH)D2, 24,25-(OH)2D3, 3-epi-25-(OH)D3, and 1,25-(OH)2D3] levels were determined by quantitative analysis via liquid chromatography coupled with tandem mass spectrometry (LC-MS/MS; QTRAP®4,500 (Sciex) coupled with an ExionLC HPLC system), with minor changes according to a previously published method (Rola et al., 2020).
VDBP was measured by the enzyme-linked immunosorbent assay (ELISA, R and D Systems, Minneapolis, MN, United States) according to the manufacturer’s instructions. The intra-assay CV ranges between 5% and 7%, and the inter-assay CV ranges between 5% and 8%.
The levels of free 25-(OH)D were calculated from total measured 25-(OH)D, VDBP, and serum albumin concentrations by using the equations by Bikle et al. (1986) (Method 1—M1),
Moreover, free, bioavailable 25-(OH)D concentration was calculated using equations adapted from those described by Vermeulen et al. (1999) (Method 2—M2). Vermeulen equations were adapted by replacing the variables for testosterone, SHBG, and albumin and their respective binding constants with those of 25-(OH)D, VDBP, and albumin,
where Kt is the association constant of SHBG for T and N=KaCa+1. This yields a second degree equation that can be solved for either FT or SHBG (Vermeulen et al., 1999).
Hand grip strength was measured by a manual dynamometer (T.K.K.5001, Takei Scientific Inst. Co., Ltd., Niigata, Japan) at a resolution of 102 g and an accuracy of 5·102 g. Prior to testing, each subject was instructed on the correct performance of the measurements. Each subject was asked to comfortably hold the dynamometer with their fingers and palm tight on the device. They then lowered their upper limb along the trunk, while keeping a certain distance, so that neither the elbow nor the hand touched the body, and gripped the dynamometer using maximum muscle power. Throughout the test, subjects were asked to stand with their feet apart and the other upper limb freely along the body. Measurements were taken in kilograms (kg).
The maximum power, force, velocity, reactive strength index—modified (RSImod), and propulsive force/power of the lower limbs were determined by the vertical jump test using a dual single-axis force platform system (Pasco PS 2141 plates, Pasco Scientific Inc., Roseville, CA, United States) with a sampling frequency of 1,000 Hz, unfiltered (Owen et al., 2014). Participants were instructed to stand with one foot on each platform with their hands on their hips. Athletes were asked to stand still for a period of 3 s to allow the system to ascertain body weight and the onset of movement threshold (Owen et al., 2014; McMahon et al., 2018). Once the system had recorded body weight, the participant was instructed to jump as high as possible while keeping their hands on their hips. For each jump, the athlete was required to return to the “quiet standing” position with the hands remaining on the hips. Three attempts were given for each athlete.
Aerobic fitness was determined using standardized 30–15 intermittent fitness test (30-15IFT). The test consisted of 30 s of shuttle runs with 15 s of passive recovery (Buchheit, 2008). The test starting velocity was set at 8 km/h, and the speed was increased by 0.5 km/h every 30 s. Participants run back and forth between two lines (40 m apart) at a pace directed by a pre-recorded audible signal. Subjects ran until voluntary exhaustion or when subjects could no longer maintain the required running speed were asked to stop the test. The distance covered at that point was recorded as the test result.
Descriptive statistics were presented using mean standard deviations. The normality of data was assessed using the Shapiro–Wilk test and homogeneity of variance using the Levene test. The difference in athletes’ characteristic and physical performance tests between both groups of athletes was analyzed using the Welch two sample t-test.
In addition, the effect size was determined by Cohen’s d with 95% confidence interval. The effect size was defined as small if < 0.2, medium if between 0.2 and 0.5, large if between 0.5 and 0.8, and very large if > 0.8.
Multiple regression was performed to analyze association between iPTH, Ca, ACa, and vitamin D metabolites levels. Moreover, we used this analysis to check relationship between vitamin D metabolites levels and athletic performance. Statistically significant models were adjusted for age, body mass, and body fat.
All analyses were performed with R for Windows, version 4.1 (R Foundation for Statistical Computing, Vienna, Austria). p < 0.05 was selected as the significance threshold.
We included forty participants in this study. Athletes competed in two sport’s discipline: judo (classified as indoor) and football (classified as outdoor) (Table 1). Indoor athletes’ weekly training hours were significantly higher than outdoor (15.1 ± 1.4 vs. 11.0 ± 1.1 h, p < 0.001). VO2max was significantly higher in outdoor than in indoor players (53.4 ± 3.5 vs. 50.9 ± 2.1 ml/kg/min, p < 0.02).
Assuming serum total 25-(OH)D levels in the range of 30–50 ng/mL to be the physiological norm 17.5% (n = 7) of athletes achieved this level. We found that 42.5% (n = 17) of the participants had a total 25-(OH)D concentration of below 20 ng/ml, which is defined as vitamin D deficiency (Table 1) (Pludowski et al., 2018).
There was no statistical difference in vitamin D metabolites [total 25-OH)D, 25-(OH)D3, 25-(OH)D2, 24,25-(OH)2D3, 3-epi-25-(OH)D3, 1,25-(OH)2D3], VDBP, free 25-(OH)D (M1, M2), and bioavailable 25-(OH)D between the indoor and outdoor player group (Table 1).
Handgrip strength (L) was higher in judoists (p = 0.018) than in football players. There were no changes observed between groups for handgrip strength (R), vertical jump parameters, and VO2max (Table 1).
Multiple regression analyses demonstrated association between iPTH, Ca, ACa, and vitamin D metabolites, VDBP, free (M1, M2), and bioavailable 25-(OH)D in both groups with no intergroup differences.
We performed multiple regression analyses to explore possible association between vitamin D metabolites and the athletic performance parameters in indoor (Table 2) and outdoor players (Table 3). Furthermore, we performed multiple regression analysis also to determine relationship between VDBP, free (M1, M2), bioavailable 25-(OH)D, and the physical fitness tests for both groups (Table 4).
TABLE 2. Association of athletic performance with vitamin D metabolites by multiple regression analyses* in indoor players (n = 16).
TABLE 3. Association of athletic performance with vitamin D metabolites by multiple regression analyses* in outdoor players (n = 24).
TABLE 4. Association of athletic performance with VDBP, free, and bioavailable 25-(OH)D concentration by multiple regression analyses* in indoor players (n = 16).
Table 2 presents the association of vitamin D metabolites with athletic performance by multiple regression analyses in indoor players. Multiple regression analysis demonstrated that total 25-(OH)D, 24,25-(OH)2D3, and 3-epi-25-(OH)D3 were significantly associated with jump height, peak velocity, peak power, RSImod, propulsive force, and power. There was also significant correlation between 3-epi-25(OH)D3 and handgrip strength (L, R). Multiple regression analysis indicated that 1,25(OH)2D significantly associated with handgrip strength (R), peak power, and propulsive power.
There was significant correlation between free 25-(OH)D (M1, M2) and handgrip strength (L) in indoor players. Analysis showed that free 25-(OH)D (M1,M2) and bioavailable 25-(OH)D significantly associated with jump height, peak velocity, power, RSImod, propulsive force, and power (Table 4).
In the group of outdoor players, multiple regression analysis showed that peak power was significantly associated with serum total 25-(OH)D and 3-epi-25-(OH)D3. The association between peak force, propulsive force and power, and 3-epi-25-(OH)D3 was observed (Table 3).
Table 5 presents the association of VDBP, free (M1, M2), and bioavailable 25-(OH)D with athletic performance by multiple regression analyses in outdoor players. According to our results, there was no significant correlation between VDBP, free (M1, M2), bioavailable 25-(OH)D, and physical performance parameters in outdoor athletes.
TABLE 5. Association of athletic performance with VDBP, free, and bioavailable 25-(OH)D concentration by multiple regression analyses* in outdoor players (n = 24).
This study represents a picture of the entire set of vitamin D metabolites in athletes. In this study, the enrolled athletes were classified into two groups, train indoor and outdoor. The aim of our study was to determine the relationship between metabolites of vitamin D, VDBP, free, bioavailable 25-(OH)D, related blood parameters (iPTH, Ca, and ACa), and physical fitness tests in athletes.
Physical activity can affect vitamin D metabolism and, hence, the final level of 25-(OH)D, main indicator of vitamin D status, and the other metabolites, all having important physiological roles. Further, it is possible that vitamin D metabolism is differently affected by different kinds of physical activity (in terms of muscle action and sun exposure). Thereby, it is interesting to compare these two situations.
Skeletal muscles express, other than the VDR, CYP27B1 (the gene encoding for 1alpha-hydroxylase, the enzyme that hydroxylates 25-(OH)D into 1,25-(OH)2D. Therefore, skeletal muscles are able to activate vitamin D (Latham et al., 2021). Although the biological relevance of the expression of this enzymatic activity is not well defined, it has been hypothesized that it is related to mitochondrial function and muscle regeneration, at least in rodents (Latham et al., 2021). Further, 1alpha-hydroxylase expression is decreased in denervation, in rats (Mori et al., 2020), while it is increased in muscle biopsies from amyotrophic lateral sclerosis patients (Si et al., 2020). Therefore, although the unclear mechanisms underlying its regulation, evidences suggest a direct connection between the muscle activity and vitamin D metabolism.
We showed that in the group of indoor judoists there was a significant correlation between total 25-(OH)D and vertical jump parameters (jump height, peak velocity, peak power, RSImod, and propulsive force and power). The relationship between total 25-(OH)D and physical performance levels of male athletes has been described in several studies (Fitzgerald et al., 2015; Kim et al., 2020; Most et al., 2021). Our study also showed the relationship between 24,25-(OH)2D3, 3-epi-25-(OH)D3, 1,25-(OH)2D, and vertical jump variables in indoor players. A small number of studies have assessed correlations between serum concentrations of 1,25-(OH)2D. Other vitamin D metabolites such as 24,25-(OH)2D3 and 3-epi-25-(OH)D3 have not been previously studied in relation to physical performance in athletes. Hassan-Smith et al. (2017) observed a statistically significant association between 1,25-(OH)2D and jump height-peak power in a group of 116 healthy human volunteers (79 women and 37 men; aged 20 ± 74 years). The authors demonstrated that 25-(OH)D3 and 24,25-(OH)2D3 were associated with efficiency (a measure of the relationship between maximum jump force and power, with the less force required to generate the same power, the more efficient the jump) in the studied group (Hassan-Smith et al., 2017). 24,25-(OH)2D3 is not considered to be physiologically active although studies involving animal models demonstrated that it plays an important role in normal bone integrity, function, and healing (Seo et al., 1997; Nemere et al., 2006).
We showed that in the group of outdoor players there was a significant association between serum total 25-(OH)D and peak power. Other studies demonstrated a positive association between 25-(OH)D levels and jump height in male soccer players (Koundourakis et al., 2014) and university-level outdoor athletes (Wilson-Barnes et al., 2020). We also showed the association between 3-epi-25-(OH)D3 and peak power, force, propulsive force, and power in outdoor athletes. Mieszkowski et al. (2020) demonstrated that serum 25-(OH)D3, 24,25-(OH)2D3, and 3-epi-25-(OH)D3 levels significantly increased after the ultra-marathon in control and supplemented group. C-3 epimerization is a common metabolic pathway of major metabolites of vitamin D3. 25-(OH)D3 undergoes epimerization, and 3-epi-25-(OH)D3 is the most prevalent form (Singh et al., 2006). The biological function of 3-epi-25-(OH)D3 is not well understood. To the best of our knowledge, this is the first paper reporting relationship between physical performance indicators and 24,25-(OH)2D3, 3-epi-25-(OH)D3 levels in athletes. Therefore, it is pivotal to study the effects of vitamin D metabolites’ action on skeletal muscle function in relation to athletic performance.
The free hormone hypothesis postulates that only the non-bound fraction (the free fraction) of hormones, which otherwise circulate in blood bound to their carrier proteins, is able to enter the cells and to exert their biologic effects (Bikle and Schwartz, 2019). Studies suggest that some functions of vitamin D may be more closely related to the free or bioavailable fraction of vitamin D than to total serum 25-(OH)D concentrations (Bikle et al., 2017; Owens et al., 2018).
Therefore, we considered whether the free fraction of 25-(OH)D correlates with musculoskeletal functions in relation to physical performance in athletes. To the best of our knowledge, there are no studies designed to assess VDBP, free, and bioavailable vitamin D status in athletes and its correlation to athletic performance. The impact of vitamin D on muscle strength, especially lower muscle strength in athletes, was evident in most studies (Hamilton et al., 2014; Koundourakis et al., 2014; Książek et al., 2016; Książek et al., 2018) and was physiologically explained by different vitamin D receptor expressions in various muscle groups (Bischoff et al., 2001; Hassan-Smith et al., 2017). Vitamin D affects the number and diameter of type II muscle fibers, which mainly regulate the ability to perform short high-power exercises (Dzik and Kaczor, 2019). Therefore, we used a vertical jump to check athletic performance in studied groups. A vertical jump is one of the essential motor skills that requires complex motor coordination, and it has been identified as one of the fundamental movement skills. Therefore, vertical jump test is used to evaluate simple lower limb muscular strength and complex tasks, such as sprint deceleration, sprint acceleration, throwing, and change of direction (Petrigna et al., 2019), which reflect physical effort during judo and football match.
We demonstrated associations between free and bioavailable 25-(OH)D levels and aspects of physical performance (handgrip strength L, vertical jump variables); this was, however, only observed within the indoor group. The differences in the obtained result between indoor and outdoor athletes may be due to the baseline individual training profile, different kinds of physical effort related to sport discipline, and hence activation of different muscle groups. This might explain the lack of significant association between VDBP, free, bioavailable 25-(OH)D, and physical performance variables in our outdoor players.
As a homeostatic perturbation, exercise and training affects calcium metabolism. Thereby, the measurement of PTH and calcium is relevant to assess the athlete’s health status as well as to contextualize the change observed in vitamin D metabolites (Lombardi et al., 2020). The results of our study showed that iPTH, Ca, and ACa were associated with and vitamin D metabolites, VDBP, free and bioavailable 25-(OH)D in both groups. However, we did not find differences in these associations between indoor and outdoor athletes. However, studies in this field are limited. In a recent study conducted at the British university athletes, we compared the seasonal effects (fall vs spring) of discipline, specific training, either indoors or outdoors on bone indices, and vitamin D status. Seasonal variation of serum 25-(OH)D was found independent from training modality (indoor vs outdoor) and PTH resulted negatively associated with the vitamin D status in the combined groups, indoor group, and more significant in the outdoor group during the spring term (Wilson-Barnes et al., 2020). In military recruits, a 32-week training program reduced vitamin D status (25-(OH)D < 50 nmol/L) and enhanced concentrations of PTH associated with the occurrence of stress fractures (Davey et al., 2016). Similarly to our study, instead, PTH resulted unaffected by an 8-week repeated sprint training regimen in young active men (Sansoni et al., 2018) and by 13 weeks of military training (O'Leary et al., 2019), despite the changes in bone indexes.
Some limitations should be considered when evaluating the result of this analysis: first, the limited sample size, so further research on a larger group of athletes is needed, and second, there was a lack of a non-sportive control group. Furthermore, our data are associational and no causal links between the vitamin D metabolites and physical performance can be drawn from this study. A notable strength of our investigation is, instead, the homogeneity within each cohort of experienced male athletes sharing the same age and anthropometry. We performed blood drawing and physical performance test in the same period (within two weeks) for both cohorts and strong pre-analytical phase.
Our study showed a significant correlation between vitamin D metabolites [total 25-(OH)D, 24,25-(OH)2D3, 3-epi-25-(OH)D3, and 1,25-(OH)2D] and handgrip strength and vertical jump variables in indoor players. It also showed a significant association between 3-epi-25-(OH)D3 and vertical jump parameters in outdoor players. The results of our study demonstrated the relationship between free, bioavailable 25-(OH)D, and vertical jump variables only in the group of indoor players.
In conclusion, this is the first study demonstrating the relationship between vitamin D metabolites, free and bioavailable 25-(OH)D, and physical performance in athletes. These observations imply that vitamin D metabolites might be involved in skeletal muscle function. However, more work is needed to explore the role of vitamin D metabolites in relation to athletic performance.
The raw data supporting the conclusion of this article will be made available by the authors, without undue reservation.
The study was approved by the Bioethics Committee of the University School of Physical Education, Wrocław, Poland (resolution number 18/2013). Informed consent was obtained from all participants included in the study. All related procedures were conducted in compliance with the standard of ethics outlined in the Declaration of Helsinki (Harriss et al., 2019).
AK participated in the conception and design of the study, contributed to the investigation, data curation, analysis, and interpretation of data for the work, and prepared the original draft. AZ participated in the conception and design of the study and contributed to the investigation and data curation. MS-L participated in the design of the study and reviewed the manuscript. GL participated in the conception and design of the study, contributed to the interpretation of results, and reviewed and edited the manuscript. All authors read and approved the final manuscript.
This research was supported financially by the University School of Physical Education, Wrocław.
The authors declare that the research was conducted in the absence of any commercial or financial relationships that could be construed as a potential conflict of interest.
All claims expressed in this article are solely those of the authors and do not necessarily represent those of their affiliated organizations, or those of the publisher, the editors and the reviewers. Any product that may be evaluated in this article, or claim that may be made by its manufacturer, is not guaranteed or endorsed by the publisher.
We gratefully acknowledge all the athletes for their interest and participation in this study.
Antinozzi C., Corinaldesi C., Giordano C., Pisano A., Cerbelli B., Migliaccio S., et al. (2017). Potential Role for the VDR Agonist Elocalcitol in Metabolic Control: Evidences in Human Skeletal Muscle Cells. J. Steroid Biochem. Mol. Biol. 167, 169–181. doi:10.1016/j.jsbmb.2016.12.010
Ashcroft S. P., Bass J. J., Kazi A. A., Atherton P. J., Philp A. (2020). The Vitamin D Receptor Regulates Mitochondrial Function in C2C12 Myoblasts. Am. J. Physiology-Cell Physiology 318 (3), C536–c541. doi:10.1152/ajpcell.00568.2019
Bikle D. D., Gee E., Halloran B., Kowalski M. A., Ryzen E., Haddad J. G. (1986). Assessment of the Free Fraction of 25-Hydroxyvitamin D in Serum and its Regulation by Albumin and the Vitamin D-Binding Protein *. J. Clin. Endocrinol. Metabolism 63 (4), 954–959. doi:10.1210/jcem-63-4-954
Bikle D. D., Malmstroem S., Schwartz J. (2017). Current Controversies. Endocrinol. Metabolism Clin. N. Am. 46 (4), 901–918. doi:10.1016/j.ecl.2017.07.013
Bikle D. D., Schwartz J. (2019). Vitamin D Binding Protein, Total and Free Vitamin D Levels in Different Physiological and Pathophysiological Conditions. Front. Endocrinol. 10, 317. doi:10.3389/fendo.2019.00317
Bischoff H. A., Borchers M., Gudat F., Duermueller U., Theiler R., Stähelin H. B., et al. (2001). In Situ detection of 1,25-dihydroxyvitamin D3 Receptor in Human Skeletal Muscle Tissue. Histochem J. 33 (1), 19–24. doi:10.1023/a:1017535728844
Brown A. J., Ritter C. S., Weiskopf A. S., Vouros P., Sasso G. J., Uskokovic M. R., et al. (2005). Isolation and Identification of 1α-Hydroxy-3-Epi-Vitamin D3, a Potent Suppressor of Parathyroid Hormone Secretion. J. Cell. Biochem. 96 (3), 569–578. doi:10.1002/jcb.20553
Buchheit M. (2008). The 30-15 Intermittent Fitness Test: Accuracy for Individualizing Interval Training of Young Intermittent Sport Players. J. Strength Cond. Res. 22 (2), 365–374. doi:10.1519/JSC.0b013e3181635b2e
Chun R. F., Peercy B. E., Orwoll E. S., Nielson C. M., Adams J. S., Hewison M. (2014). Vitamin D and DBP: the Free Hormone Hypothesis Revisited. J. Steroid Biochem. Mol. Biol. 144, 132–137. doi:10.1016/j.jsbmb.2013.09.012
Davey T., Lanham-New S. A., Shaw A. M., Hale B., Cobley R., Berry J. L., et al. (2016). Low Serum 25-hydroxyvitamin D Is Associated with Increased Risk of Stress Fracture during Royal Marine Recruit Training. Osteoporos. Int. 27 (1), 171–179. doi:10.1007/s00198-015-3228-5
Di Luigi L., Antinozzi C., Piantanida E., Sgrò P. (2020). Vitamin D, Sport and Health: a Still Unresolved Clinical Issue. J. Endocrinol. Invest. 43 (12), 1689–1702. doi:10.1007/s40618-020-01347-w
Dzik K. P., Kaczor J. J. (2019). Mechanisms of Vitamin D on Skeletal Muscle Function: Oxidative Stress, Energy Metabolism and Anabolic State. Eur. J. Appl. Physiol. 119 (4), 825–839. doi:10.1007/s00421-019-04104-x
Farrokhyar F., Tabasinejad R., Dao D., Peterson D., Ayeni O. R., Hadioonzadeh R., et al. (2015). Prevalence of Vitamin D Inadequacy in Athletes: a Systematic-Review and Meta-Analysis. Sports Med. 45 (3), 365–378. doi:10.1007/s40279-014-0267-6
Fitzgerald J. S., Peterson B. J., Warpeha J. M., Johnson S. C., Ingraham S. J. (2015). Association between Vitamin D Status and Maximal-Intensity Exercise Performance in Junior and Collegiate Hockey Players. J. Strength Cond. Res. 29 (9), 2513–2521. doi:10.1519/jsc.0000000000000887
Garcia L. A., King K. K., Ferrini M. G., Norris K. C., Artaza J. N. (2011). 1,25(OH)2vitamin D3 Stimulates Myogenic Differentiation by Inhibiting Cell Proliferation and Modulating the Expression of Promyogenic Growth Factors and Myostatin in C2C12 Skeletal Muscle Cells. Endocrinology 152 (8), 2976–2986. doi:10.1210/en.2011-0159
Girgis C. M., Clifton-Bligh R. J., Hamrick M. W., Holick M. F., Gunton J. E. (2013). The Roles of Vitamin D in Skeletal Muscle: Form, Function, and Metabolism. Endocr. Rev. 34 (1), 33–83. doi:10.1210/er.2012-1012
Halfon M., Phan O., Teta D. (2015). Vitamin D: a Review on its Effects on Muscle Strength, the Risk of Fall, and Frailty. BioMed Res. Int. 2015, 953241. doi:10.1155/2015/953241
Hamilton B., Whiteley R., Farooq A., Chalabi H. (2014). Vitamin D Concentration in 342 Professional Football Players and Association with Lower Limb Isokinetic Function. J. Sci. Med. Sport 17 (1), 139–143. doi:10.1016/j.jsams.2013.03.006
Harriss D. J., MacSween A., Atkinson G. (2019). Ethical Standards in Sport and Exercise Science Research: 2020 Update. Int. J. Sports Med. 40 (13), 813–817. doi:10.1055/a-1015-3123
Hassan-Smith Z. K., Jenkinson C., Smith D. J., Hernandez I., Morgan S. A., Crabtree N. J., et al. (2017). 25-hydroxyvitamin D3 and 1,25-dihydroxyvitamin D3 Exert Distinct Effects on Human Skeletal Muscle Function and Gene Expression. PLoS One 12 (2), e0170665. doi:10.1371/journal.pone.0170665
Herrmann M., Farrell C.-J. L., Pusceddu I., Fabregat-Cabello N., Cavalier E. (2017). Assessment of Vitamin D Status - a Changing Landscape. Clin. Chem. Lab. Med. 55 (1), 3–26. doi:10.1515/cclm-2016-0264
Kim D. K., Park G., Kuo L.-T., Park W.-H. (2020). Association of Vitamin D Status with Lower Limb Muscle Strength in Professional Basketball Players: A Cross-Sectional Study. Nutrients 12 (9), 2715. doi:10.3390/nu12092715
Koundourakis N. E., Androulakis N. E., Malliaraki N., Margioris A. N. (2014). Vitamin D and Exercise Performance in Professional Soccer Players. PLoS One 9 (7), e101659. doi:10.1371/journal.pone.0101659
Krzywanski J., Mikulski T., Krysztofiak H., Mlynczak M., Gaczynska E., Ziemba A. (2016). Seasonal Vitamin D Status in Polish Elite Athletes in Relation to Sun Exposure and Oral Supplementation. PLoS One 11 (10), e0164395. doi:10.1371/journal.pone.0164395
Książek A., Dziubek W., Pietraszewska J., Słowińska-Lisowska M. (2018). Relationship between 25(OH)D Levels and Athletic Performance in Elite Polish Judoists. bs 35 (2), 191–196. doi:10.5114/biolsport.2018.74195
Książek A., Zagrodna A., Dziubek W., Pietraszewski B., Ochmann B., Słowińska Lisowska M. (2016). 25(OH)D3 Levels Relative to Muscle Strength and Maximum Oxygen Uptake in Athletes. J. Hum. Kinet. 50, 71–77. doi:10.1515/hukin-2015-0144
Latham C. M., Brightwell C. R., Keeble A. R., Munson B. D., Thomas N. T., Zagzoog A. M., et al. (2021). Vitamin D Promotes Skeletal Muscle Regeneration and Mitochondrial Health. Front. Physiol. 12, 660498. doi:10.3389/fphys.2021.660498
Lombardi G., Vitale J. A., Logoluso S., Logoluso G., Cocco N., Cocco G., et al. (2017). Circannual Rhythm of Plasmatic Vitamin D Levels and the Association with Markers of Psychophysical Stress in a Cohort of Italian Professional Soccer Players. Chronobiology Int. 34 (4), 471–479. doi:10.1080/07420528.2017.1297820
Lombardi G., Ziemann E., Banfi G., Corbetta S. (2020). Physical Activity-dependent Regulation of Parathyroid Hormone and Calcium-Phosphorous Metabolism. Ijms 21 (15), 5388. doi:10.3390/ijms21155388
Lutsey P. L., Eckfeldt J. H., Ogagarue E. R., Folsom A. R., Michos E. D., Gross M. (2015). The 25-hydroxyvitamin D3 C-3 Epimer: Distribution, Correlates, and Reclassification of 25-hydroxyvitamin D Status in the Population-Based Atherosclerosis Risk in Communities Study (ARIC). Clin. Chim. Acta 442, 75–81. doi:10.1016/j.cca.2014.12.036
McMahon J. J., Suchomel T. J., Lake J. P., Comfort P. (2018). Understanding the Key Phases of the Countermovement Jump Force-Time Curve. Strength & Cond. J. 40 (4), 96–106. doi:10.1519/ssc.0000000000000375
Mieszkowski J., Kochanowicz A., Piskorska E., Niespodziński B., Siódmiak J., Buśko K., et al. (2021). Serum Levels of Bone Formation and Resorption Markers in Relation to Vitamin D Status in Professional Gymnastics and Physically Active Men during Upper and Lower Body High-Intensity Exercise. J. Int. Soc. Sports Nutr. 18 (1), 29. doi:10.1186/s12970-021-00430-8
Mieszkowski J., Stankiewicz B., Kochanowicz A., Niespodziński B., Kowalik T., Żmijewski M. A., et al. (2020). Ultra-Marathon-Induced Increase in Serum Levels of Vitamin D Metabolites: A Double-Blind Randomized Controlled Trial. Nutrients 12 (12), 3629. doi:10.3390/nu12123629
Mori R., Yokokawa T., Fujita S. (2020). Modified Expression of Vitamin D Receptor and CYP27B1 in Denervation-Induced Muscle Atrophy. Biochem. Biophysical Res. Commun. 529 (3), 733–739. doi:10.1016/j.bbrc.2020.05.205
Most A., Dörr O., Nef H., Hamm C., Bauer T., Bauer P. (2021). Influence of 25-Hydroxy-Vitamin D Insufficiency on Maximal Aerobic Power in Elite Indoor Athletes: A Cross-Sectional Study. Sports Med. Open 7 (1), 74. doi:10.1186/s40798-021-00363-1
Nemere I., Wilson C., Jensen W., Steinbeck M., Rohe B., Farach-Carson M. C. (2006). Mechanism of 24,25-dihydroxyvitamin D3-Mediated Inhibition of Rapid, 1,25-dihydroxyvitamin D3-Induced Responses: Role of Reactive Oxygen Species. J. Cell. Biochem. 99 (6), 1572–1581. doi:10.1002/jcb.21008
O'Leary T. J., Izard R. M., Walsh N. P., Tang J. C. Y., Fraser W. D., Greeves J. P. (2019). Skeletal Macro- and Microstructure Adaptations in Men Undergoing Arduous Military Training. Bone 125, 54–60. doi:10.1016/j.bone.2019.05.009
Owen N. J., Watkins J., Kilduff L. P., Bevan H. R., Bennett M. A. (2014). Development of a Criterion Method to Determine Peak Mechanical Power Output in a Countermovement Jump. J. Strength Cond. Res. 28 (6), 1552–1558. doi:10.1519/jsc.0000000000000311
Owens D. J., Allison R., Close G. L. (2018). Vitamin D and the Athlete: Current Perspectives and New Challenges. Sports Med. 48 (Suppl. 1), 3–16. doi:10.1007/s40279-017-0841-9
Petrigna L., Karsten B., Marcolin G., Paoli A., D’Antona G., Palma A., et al. (2019). A Review of Countermovement and Squat Jump Testing Methods in the Context of Public Health Examination in Adolescence: Reliability and Feasibility of Current Testing Procedures. Front. Physiol. 10, 1384. doi:10.3389/fphys.2019.01384
Pludowski P., Holick M. F., Grant W. B., Konstantynowicz J., Mascarenhas M. R., Haq A., et al. (2018). Vitamin D Supplementation Guidelines. J. Steroid Biochem. Mol. Biol. 175, 125–135. doi:10.1016/j.jsbmb.2017.01.021
Powe C. E., Ricciardi C., Berg A. H., Erdenesanaa D., Collerone G., Ankers E., et al. (2011). Vitamin D-Binding Protein Modifies the Vitamin D-Bone Mineral Density Relationship. J. Bone Min. Res. 26 (7), 1609–1616. doi:10.1002/jbmr.387
Rola R., Kowalski K., Bieńkowski T., Studzińska S. (2020). Improved Sample Preparation Method for Fast LC-MS/MS Analysis of Vitamin D Metabolites in Serum. J. Pharm. Biomed. Analysis 190, 113529. doi:10.1016/j.jpba.2020.113529
Sansoni V., Perego S., Vernillo G., Barbuti A., Merati G., La Torre A., et al. (2018). Effects of Repeated Sprints Training on Fracture Risk-Associated miRNA. Oncotarget 9 (26), 18029–18040. doi:10.18632/oncotarget.24707
Seo E.-G., Einhorn T. A., Norman A. W. (1997). 24R,25-Dihydroxyvitamin D3: An Essential Vitamin D3 Metabolite for Both Normal Bone Integrity and Healing of Tibial Fracture in Chicks*. Endocrinology 138 (9), 3864–3872. doi:10.1210/endo.138.9.5398
Seo E.-G., Norman A. W. (1997). Three-fold Induction of Renal 25-hydroxyvitamin D3-24-Hydroxylase Activity and Increased Serum 24,25-dihydroxyvitamin D3 Levels Are Correlated with the Healing Process after Chick Tibial Fracture. J. Bone Min. Res. 12 (4), 598–606. doi:10.1359/jbmr.1997.12.4.598
Seo M.-W., Song J. K., Jung H. C., Kim S.-W., Kim J.-H., Lee J.-M. (2019). The Associations of Vitamin D Status with Athletic Performance and Blood-Borne Markers in Adolescent Athletes: A Cross-Sectional Study. Ijerph 16 (18), 3422. doi:10.3390/ijerph16183422
Shieh A., Chun R. F., Ma C., Witzel S., Meyer B., Rafison B., et al. (2016). Effects of High-Dose Vitamin D2 versus D3 on Total and Free 25-Hydroxyvitamin D and Markers of Calcium Balance. J. Clin. Endocrinol. Metabolism 101 (8), 3070–3078. doi:10.1210/jc.2016-1871
Si Y., Kazamel M., Kwon Y., Lee I., Anderson T., Zhou S., et al. (2020). The Vitamin D Activator CYP27B1 Is Upregulated in Muscle Fibers in Denervating Disease and Can Track Progression in Amyotrophic Lateral Sclerosis. J. Steroid Biochem. Mol. Biol. 200, 105650. doi:10.1016/j.jsbmb.2020.105650
Singh R. J., Taylor R. L., Reddy G. S., Grebe S. K. G. (2006). C-3 Epimers Can Account for a Significant Proportion of Total Circulating 25-hydroxyvitamin D in Infants, Complicating Accurate Measurement and Interpretation of Vitamin D Status. J. Clin. Endocrinol. Metab. 91 (8), 3055–3061. doi:10.1210/jc.2006-0710
Siri W. E. (1993). Body Composition from Fluid Spaces and Density: Analysis of Methods 1961. Nutrition 9 (5), 480–492.
Tsuprykov O., Chen X., Hocher C.-F., Skoblo R., Lianghong Y., Hocher B. (2018). Why Should We Measure Free 25(OH) Vitamin D? J. Steroid Biochem. Mol. Biol. 180, 87–104. doi:10.1016/j.jsbmb.2017.11.014
Valtueña J., Dominguez D., Til L., González-Gross M., Drobnic F. (2014). High Prevalence of Vitamin D Insufficiency Among Elite Spanish Athletes the Importance of Outdoor Training Adaptation. Nutr. Hosp. 30 (1), 124–131. doi:10.3305/nh.2014.30.1.7539
Vermeulen A., Verdonck L., Kaufman J. M. (1999). A Critical Evaluation of Simple Methods for the Estimation of Free Testosterone in Serum. J. Clin. Endocrinol. Metab. 84 (10), 3666–3672. doi:10.1210/jcem.84.10.6079
Wilson-Barnes S. L., Hunt J. E. A., Mendis J., Williams E. L., King D., Roberts H., et al. (2021). The Relationship between Vitamin D Status, Intake and Exercise Performance in UK University-Level Athletes and Healthy Inactive Controls. PLoS One 16 (4), e0249671. doi:10.1371/journal.pone.0249671
Wilson-Barnes S. L., Hunt J. E. A., Williams E. L., Allison S. J., Wild J. J., Wainwright J., et al. (2020). Seasonal Variation in Vitamin D Status, Bone Health and Athletic Performance in Competitive University Student Athletes: a Longitudinal Study. J. Nutr. Sci. 9, e8. doi:10.1017/jns.2020.1
Keywords: vitamin D, 24,25-(OH)2D3, 3-epi-25-(OH)D, 1,25(OH)2D, VDBP, vertical jump, physical performance
Citation: Książek A, Zagrodna A, Słowińska-Lisowska M and Lombardi G (2022) Relationship Between Metabolites of Vitamin D, Free 25-(OH)D, and Physical Performance in Indoor and Outdoor Athletes. Front. Physiol. 13:909086. doi: 10.3389/fphys.2022.909086
Received: 01 April 2022; Accepted: 30 May 2022;
Published: 08 July 2022.
Edited by:
Hassane Zouhal, University of Rennes 2—Upper Brittany, FranceReviewed by:
Jedrzej Antosiewicz, Medical University of Gdansk, PolandCopyright © 2022 Książek, Zagrodna, Słowińska-Lisowska and Lombardi. This is an open-access article distributed under the terms of the Creative Commons Attribution License (CC BY). The use, distribution or reproduction in other forums is permitted, provided the original author(s) and the copyright owner(s) are credited and that the original publication in this journal is cited, in accordance with accepted academic practice. No use, distribution or reproduction is permitted which does not comply with these terms.
*Correspondence: Anna Książek, YW5uYS5rc2lhemVrQGF3Zi53cm9jLnBs
Disclaimer: All claims expressed in this article are solely those of the authors and do not necessarily represent those of their affiliated organizations, or those of the publisher, the editors and the reviewers. Any product that may be evaluated in this article or claim that may be made by its manufacturer is not guaranteed or endorsed by the publisher.
Research integrity at Frontiers
Learn more about the work of our research integrity team to safeguard the quality of each article we publish.