- 1School of Kinesiology, Shanghai University of Sport, Shanghai, China
- 2Department of Rehabilitation, The People’s Hospital of Liaoning Province, Shenyang, China
- 3Department of Orthopaedic, The First Affiliated Hospital, Guangzhou University of Chinese Medicine, Guangzhou, China
- 4School of Biomedical Sciences, University of Western Australia, Perth, WA, Australia
- 5School of Sports Science, Wenzhou Medical University, Wenzhou, China
Fibrillin is the major constituent of extracellular microfibrils, which are distributed throughout connective tissues. Asprosin is derived from the C-terminal region of the FBN1 gene, which encodes profibrillin that undergoes cleavage by furin protein. In response to fasting with low dietary glucose, asprosin is released as a secreted factor from white adipose tissue, and is transported to the liver for the mediation of glucose release into the blood circulation. Through binding to OLFR734, an olfactory G-protein-coupled receptor in liver cells, asprosin induces a glucogenic effect to regulate glucose homeostasis. Bioinformatics analyses revealed that the FBN1 gene is abundantly expressed in human skeletal muscle-derived mesoangioblasts, osteoblast-like cells, and mesenchymal stem cells, indicating that the musculoskeletal system might play a role in the regulation of asprosin expression. Interestingly, recent studies suggest that asprosin is regulated by exercise. This timely review discusses the role of asprosin in metabolism, its receptor signalling, as well as the exercise regulation of asprosin. Collectively, asprosin may have a vital regulatory effect on the improvement of metabolic disorders such as diabetes mellitus and obesity via exercise.
Introduction
Asprosin was initially identified as a new class of hormone, or a centrally acting orexigenic hormone that regulates the liver to release hepatic glucose and increase plasma glucose levels (Greenhill, 2016; Romere et al., 2016). It was subsequently revealed that asprosin acts as a central appetite stimulator, or a fasting-induced glucogenic protein hormone (Duerrschmid et al., 2017), via a signalling pathway similar to ghrelin, also known as lenomorelin or “hunger hormone” (Donma and Donma, 2018). Asprosin deficiency is found in patients with neonatal progeroid syndrome (NPS), while excess production of asprosin is detected in the condition of insulin resistance and obesity (Greenhill, 2016; Romere et al., 2016). It is proposed that asprosin in cooperation with ghrelin is beneficial to cachexia-anorexia, a complex metabolic syndrome occurring in severe burns victims (Donma and Donma, 2018). These findings imply that asprosin plays an essential role in a range of metabolic-related diseases. Exercise improves the outcomes of metabolic disorders, such as promoting energy substrate redistribution, losing fat mass, and reducing inflammation (Gonzalez-Gil and Elizondo-Montemayor, 2020). Notably, exercise affects the release of asprosin, which may regulate the corresponding metabolism (Ko et al., 2019). In this review, we summarize the expression profile of asprosin and the effects of exercise on asprosin to mediate metabolic diseases, such as diabetes mellitus (DM), obesity and polycystic ovary syndrome (PCOS).
Molecular Structure, Expression and Function of Asprosin
Asprosin is encoded by FBN1 gene and belongs to a post-translationally modified product of fibrillin. Asprosin is released as the C-terminal propeptide from profibrillin-1, and is cleaved by pro proteinase furin (Jensen et al., 2014). It is well conserved between human and mouse based on multiple sequence alignment (Figure 1). Molecular structure analysis of human asprosin protein shows that it contains Asprosin (aa2732-2871) at the C-terminal region of prefibrillin-1 which contains a signal peptide (aa1-24), propeptide (aa25-44), a furin cleavage motif after RAKR (aa44), fibrillin unique N-terminal (FUN) domain (aa45-81), microfibrillar-associated protein 4 (MFAP4) interacting domain (aa119-329), proline rich domain (aa402-446), RGD motif (aa1541-1543), a furin cleavage motif after RKRR (aa2731), and fribrillin-1 (aa25-2731) (Figure 2A). Further, Asprosin is predicted to consist of two alpha helixes and several beta strands based on the analysis of the Phyre2 web portal (Figure 2B). Tertiary structure analysis shows that human asprosin protein mimics the crystal structure of cadherin8 ec1 domain using the Phyre2 web portal (Figure 2C). FBN1 mRNA expression was detected widely in human tissues and cell lines (Figure 3). Gene expression analyses by Genevisible®- based bioinformatics (Hruz et al., 2008) reveal that FBN1 mRNA was most abundantly expressed in human tissues of skeletal muscle-derived mesoangioblasts, meniscal cells, liver artery endothelium cells, liver vein endothelium cells and osteoblast-like cells (Figure 3A). It was most highly expressed in human fibroblast cell lines of GM05659, tendon stem/progenitor cell, adult stem cells, mesenchymal stem cell, and mesoangioblast (Figure 3B). These results indicate that asprosin is likely to be released from these tissues and cell sources. The expression of asprosin varies between different tissues in pathological conditions. For instance, the asprosin level is elevated in gastric and testicular tissues but decreased in liver, kidney, and heart tissues in diabetic rats compared with normal controls (Kocaman and Kuloglu, 2020). Asprosin can circulate to the liver to induce a glucogenic effect through OLFR734, an olfactory G-protein-coupled receptor in liver cells to regulate glucose homeostasis (Li et al., 2019). It also crosses the blood-brain barrier to induce an orexigenic effect via regulating appetite-modulating neurons in the arcuate nucleus of the hypothalamus (Duerrschmid et al., 2017).
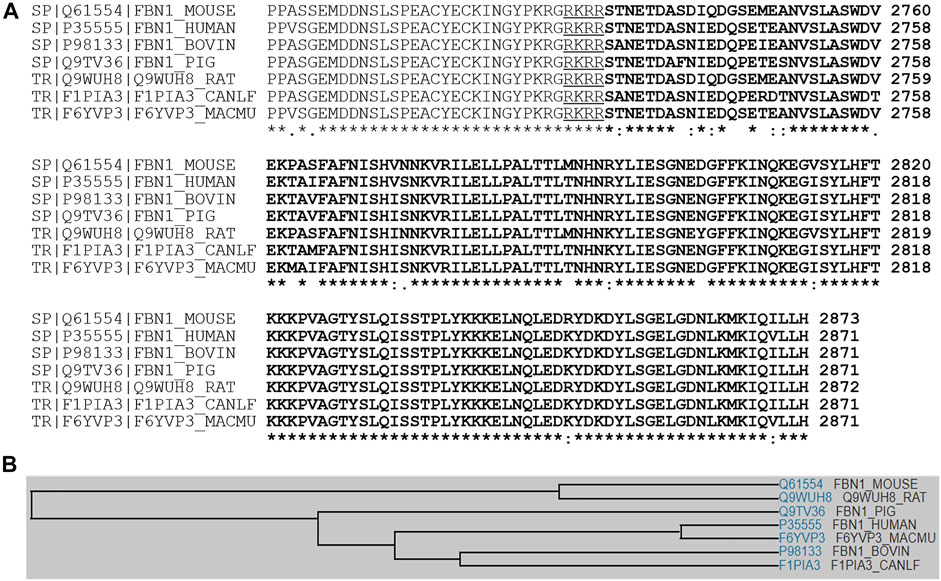
FIGURE 1. (A) Multiple sequence alignment analyses signifying amino acid sequence identity and similarity among asprosin in various species of human, mouse, rat, pig, dog, bovine, and rhesus macaque. (B) A family tree of asprosin proteins is presented.
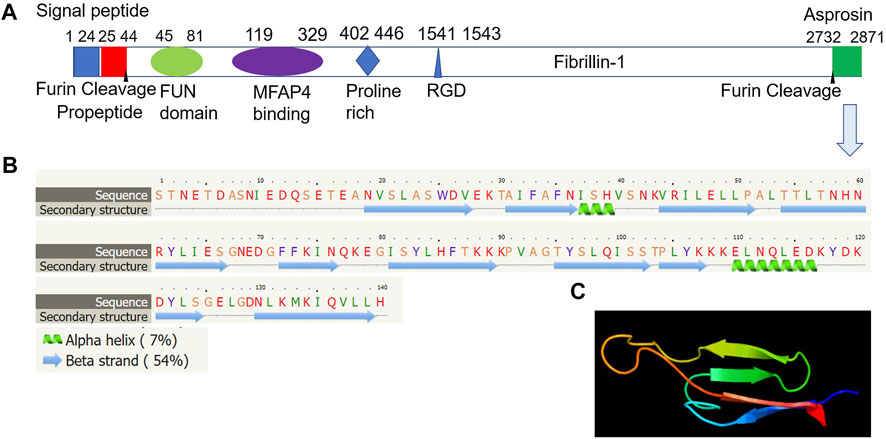
FIGURE 2. Molecular structure of human asprosin protein. (A) Asprosin (aa2732-2871) is derived from the C-terminal region of prefibrillin-1 which contains a signal peptide (aa1-24), propeptide (aa25-44), a furin cleavage motif after RAKR (aa44), fibrillin unique N-terminal (FUN) domain (aa45-81), microfibrillar-associated protein 4 (MFAP4) interacting domain (aa119-329), proline rich domain (aa402-446), RGD motif (aa1541-1543), a furin cleavage motif after RKRR (aa2731), and fribrillin-1 (aa25-2731). (B) Asprosin is predicted to consist of two alpha helixes and several beta strands based on the analysis by Phyre2 web portal (http://www.sbg.bio.ic.ac.uk/phyre2/). (C) Tertiary structure analysis showing human asprosin protein that mimics crystal structure of cadherin8 ec1 domain based on template c1zxkB, which has 46 residues (33% of asprosin sequence) have been modelled with 95.2% confidence by the single highest scoring template using Phyre2 web portal analysis (http://www.sbg.bio.ic.ac.uk/phyre2/).
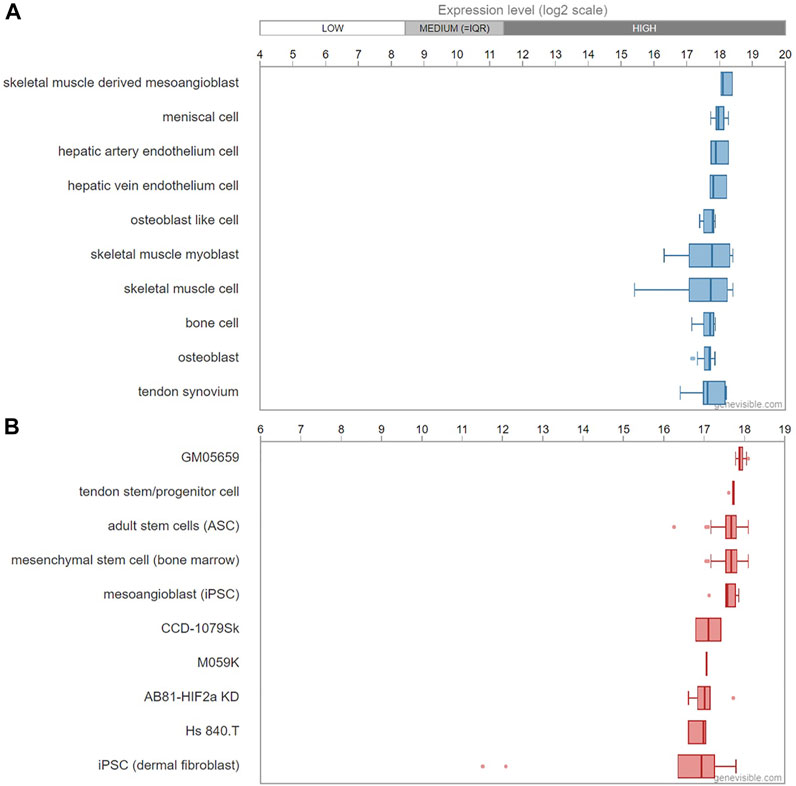
FIGURE 3. mRNA expression profiling of FBN1 gene in human tissues (A) and cell lines (B) predicted by Genevisible® bioinformatics analyses (http://genevisible.com). The ten most highly ranking tissues and cell lines that express FBN1 mRNA are shown.
During diverse progressions of cell metabolism, asprosin appears to play varying roles. For example, it is shown that asprosin inhibits apoptosis and reactive oxygen species (ROS) generation, through the activation of the ERK1/2-SOD2 pathway, which increases the antioxidant level of mesenchymal stromal cells (MSCs) in an ischemic heart disease model. But there is no influence on cell migration and proliferation by asprosin (Zhang Z. et al., 2019).
Asprosin in Metabolic Diseases
The endocrine system acts through hormones in the regulation of carbohydrate, protein, and fat metabolism, and maintains the development and functional activities of our body. The level of circulating asprosin is positively correlated with risk factors of abnormal metabolism, such as fasting glucose and atherogenesis index in menopausal women (Wiecek et al., 2019). Asprosin is shown to mediate lipid metabolism, which negatively regulates fat browning by reducing the expression of the browning marker, uncoupling protein 1 (UCP1), and by promoting lipid deposition by suppressing nuclear factor E2-related factor 2 (Nrf2) activation, in both a cold-stimulated and high-fat diet murine model (Miao et al., 2021).
DM
Type 1 diabetes mellitus (T1DM), Type 2 diabetes mellitus (T2DM), and gestational diabetes mellitus (GDM) are three subtypes of DM. Serum asprosin is reported to increase in all these types (Baykus et al., 2019; Ko et al., 2019; Zhang L. et al., 2019). Oral glucose tolerance test (OGTT) is used to assess dynamic changes in insulin secretion following oral glucose load. In normal glucose tolerance (NGT) participants, after a 75 g OGTT, asprosin is negatively correlated with blood glucose (BG) and C-peptide, after adjusting for age, gender, and BMI (Zhang X. et al., 2020). Conversely, in T2DM patients, plasma asprosin level is positively correlated with fasting blood glucose (FBG), hemoglobin A1c (HbA1c), post-challenge plasma glucose (2hPG), homeostasis model assessment for insulin resistance (HOMA-IR), triglyceride (TG), triacylglycerol (TAG) and waist circumference (Wc). Among them, FBG and HOMA-IR are independently correlated with asprosin in T2DM, which was obtained by multiple stepwise regression analysis (Li et al., 2018; Naiemian et al., 2020; Wang et al., 2018). Similarly, plasma asprosin is elevated in GDM patients, as related factors of insulin resistance are increased in the early stages of gestation (Zhong et al., 2020).
The prophase of T2DM, also known as impaired glucose regulation (IGR), including impaired glucose tolerance (IGT) and impaired fasting glycaemia (IFG) (Alberti and Zimmet, 1998), is associated with insulin resistance and β-cell dysfunction (Williamson, 2018), and has a high risk of DM (Tabak et al., 2012). Individuals with IGR show relatively higher plasma asprosin levels than newly T2DM patients, and both are higher than non-T2DM subjects. Subsequent correlation analysis elucidates that plasma asprosin is negatively correlated with a homeostasis model assessment for β-cell function (HOMA-β), acute insulin response (AIR), and glucose disposition index (GDI). Specifically, circulation asprosin level is correlated with IGR and newly T2DM by multiple logistical regression analyses (Wang et al., 2018). In newly diagnosed DM, circulating asprosin is significantly suppressed, after 24-weeks treatment, as well as HbA1c, FPG, and BMI, by sodium-glucose co-transporter-2 (SGLT2) inhibitors, a hypoglycemic agent, which selectively inhibit glucose reabsorption (Jiang et al., 2021). Asprosin therefore appears to be a potential biomarker for DM diagnosis.
As diabetes progresses, various complications gradually emerge. In DM patients with kidney disease, circulating asprosin is higher than that in individuals without diabetic nephropathy. Furthermore, patients with macroalbuminuria and microalbuminuria have a significantly elevated asprosin level. Moreover, asprosin level is positively associated with disease duration, urinary albumin-to-creatinine ratio (UACR), creatinine, blood urea nitrogen (BUN), and negatively correlated with estimated glomerular filtration rate (eGFR), and the treatment of metformin, and acarbose, especially in the early stage of nephropathy. Furthermore, asprosin is independently related to UACR, BUN, low-density lipoprotein (LDL)-C, and the development of albuminuria in T2DM patients by regression analyses (Deng et al., 2020; Wang et al., 2021; Zhang H. et al., 2020). Similarly, in diabetic retinopathy (DRP) individuals, the asprosin level is higher compared with non-DRP patients in blood and aqueous humor (Oruc et al., 2020). Collectively, asprosin appears to play a role in the onset and progression of DM and might be a potential therapeutic target for the treatment of this illness.
The researchers have explored the pathogenic mechanism of asprosin in DM. In vivo experiments suggest that asprosin could only elevate BG level in normoglycemic mice but not in diabetic ones. Further, intraperitoneal injection of asprosin was found to decrease excessive hepatic TG, cholesterol, and LDL of diabetic animals (Hekim et al., 2021). In vitro findings indicated that asprosin could affect the physiological function of insulin-releasing pancreatic β-cells. For example, Lee et al. (2019) examined the knockdown effects of asprosin and its downstream toll-like receptor (TLR) 4 or JNK expressions in a pancreatic β-cell line MIN6. The results demonstrate that asprosin increases the level of inflammatory cytokines TNF and MCP-1, and phosphorylation of nuclear factor-kappa B (NF-κB) to exacerbate inflammation. Analogously, cell viability and insulin secretion are all attenuated via asprosin treatment, through the TLR4/JNK signal pathway. Similarly, research on MIN6 cells reports that recombinant asprosin inhibits the expression of autophagy marker LC3-II/LC3-I and beclin 1, and promotes apoptosis through an AMP-activated protein kinase (AMPK)-mTOR pathway in β-cells (Wang and Hu, 2021).
In addition to disrupting β-cells secretion, asprosin also contributes to insulin resistance. The maintenance of blood glucose homeostasis depends on the insulin sensitivity of tissues such as muscle, liver, and fat. When the extracellular environment is disturbed, increased cellular stress in these tissues hinders the metabolic activity of insulin, resulting in insulin resistance, which is a major determinant of T2DM progression (DeFronzo and Tripathy, 2009; Petersen and Shulman, 2018; Fazakerley et al., 2019). In a normoglycemic hyperinsulinemic model, skeletal muscle takes up about 80% of the total glucose metabolism (DeFronzo et al., 1981), while in a study of leg muscle glucose uptake, insulin-stimulated glucose uptake was reduced by approximately 50% in T2DM. This suggested that skeletal muscle insulin resistance is considered the incipient metabolic disorder of T2DM (DeFronzo et al., 1981; DeFronzo and Tripathy, 2009). Specifically, impaired glycogen synthesis is a major mechanism leading to muscle insulin resistance. During the process of insulin-mediated glucose uptake, it has been suggested that the IRS-1/PI-3 kinase/Akt pathway is critical (Krook et al., 2000; DeFronzo and Tripathy, 2009). However, the regulatory role of asprosin in this pathway remains to be elucidated. An intervention with asprosin in myocyte cell line C2C12 and soleus skeletal muscle reveals that asprosin accelerates insulin resistance by stimulating the ER stress/inflammation-mediated pathway. In addition, asprosin suppresses the expression of protein kinase C-δ (PKCδ) phosphorylation by high-affinity binding and inhibits sarcoplasmic reticulum Ca2+ ATPase 2b level, resulting in impaired myocyte insulin sensitivity (Jung et al., 2019).
Obesity
Obesity, especially abdominal obesity, is often accompanied by metabolic disorders such as hyperglycemia and dyslipidemia, and cardiovascular disease morbidity and mortality (Engin, 2017). In obese subjects, circulating asprosin is not only increased compared with normal weight but also elevated to accompany the rising BMI, in both adults and children (Ugur and Aydin, 2019; Sunnetci Silistre and Hatipogl, 2020). Analogously, in underweight subjects and weight reduction after bariatric surgery participants, asprosin levels are also associated with BMI (Ugur and Aydin, 2019; Wang C. Y. et al., 2019). The studies of obesity in children reveal that asprosin level is increased in children with insulin resistance as compared with the non-insulin resistance group. Among obesity-related indicators, waist-to-hip ratio (WHR) and HOMA-IR are positively associated with asprosin, which is considered to be a predictor of obesity by multiple regression analysis (Wang M. et al., 2019; Sunnetci Silistre and Hatipogl, 2020).
Additional research of asprosin regulation in an obesity model of monosodium glutamate (MSG)-induced hypothalamic obesity mice showed that 3-weeks treatment by AM6545, a peripheral cannabinoid receptor 1 (CB1R) blocker, diminishes the level of asprosin while reducing the increased body weight, dyslipidemia and intraperitoneal fat mass (Ma et al., 2018). Irisin, a peptide hormone secreted by skeletal muscle, also regulates asprosin expression. Ozcan et al. (2020) reported that asprosin level is decreased in female obese rats with irisin subcutaneous administration, as well as excessive blood glucose, LDL, and TG.
For obesity, appetite is an effective predictor of reduced energy intake and weight loss (Drapeau et al., 2007). Duerrschmid et al. found that decreasing the plasma asprosin level by monoclonal antibody reduces both appetite and body weight in obese mice to ameliorate BG level. This result is due to the appetite-regulating effect of asprosin, which promotes the activity of orexigenic receptor protein tyrosine phosphatase receptor δ (Ptprd) in AgRP+ neurons in a cAMP-dependent manner; whilst inhibiting anorexic POMC+ neurons in a GABA-dependent pathway, thereby stimulating appetite and promoting bodyweight accumulation similar to ghrelin (Duerrschmid et al., 2017; Donma & Donma, 2018; Mishra et al., 2022).
PCOS
Polycystic ovary syndrome (PCOS) is a gynaecological syndrome with characteristics of hyperandrogenemia, ovulatory dysfunction, and/or ovarian polycystic pathology. PCOS patients have metabolic disorders, such as insulin resistance and dyslipidemia (Deniz et al., 2021). Asprosin levels are found to be higher in PCOS patients, and in addition, individuals with the highest asprosin secretion have the greatest risks of PCOS (Alan et al., 2019). Plasma asprosin concentration is an independent risk factor for PCOS, according to the binary logistic regression analysis (Li et al., 2018). Similar to T2DM, asprosin is reported to be positively associated with FBG, HbA1c, HOMA-IR, and testosterone in PCOS (Deniz et al., 2021; Li et al., 2018). Asprosin could therefore become a novel metabolic marker of PCOS.
In other endocrine diseases, studies have found that asprosin is involved in different pathological processes. Non-alcoholic fatty liver disease (NAFLD) is a type of hepatic disease, which is associated with insulin resistance, metabolic syndrome, and type 2 diabetes (Cobbina and Akhlaghi, 2017). In NAFLD patients, serum asprosin is higher than in healthy individuals, and is independently related to FBG and HOMA-IR by multivariate linear regression analyses (Ke et al., 2020).
Asprosin in Exercise-Regulating Metabolic Diseases
Exercise is essential for human health, especially for the endocrine system. Lack of exercise and obesity are attributed to chronic oxidative stress, which leads to instability of insulin secretion and vascular complications. Physical exercise could induce adaptive responses to maintain the redox balance, thereby controlling disease progression and complications (Poblete-Aro et al., 2018). Meta-analysis shows that structured exercise intervention is effective for glycaemic control in T2DM patients with decreased insulin resistance (Sampath Kumar et al., 2019).
Exercise affects the expression of asprosin, such that untrained women have higher circulating asprosin concentrations during the menstrual cycle especially in the follicular phase, compared with trained women (Leonard et al., 2021). A single short-term anaerobic exercise for a 20s bicycle sprint indicated that both circulating asprosin and BG are elevated after exercise, interestingly, this influence only appears in women, and the mechanism remains unknown (Wiecek et al., 2018). In addition, exercise also plays a different role in diverse metabolic diseases through asprosin.
In DM, appropriate exercise ameliorates hyperglycemia by regulating hepatic glucose metabolism. Asprosin is reported to increase the release of hepatocyte glucose via a cAMP-PKA axis, independent of glucagon or epinephrine (Romere et al., 2016). While, an eight-week aerobic exercise decreases the hepatic asprosin level by PKA/TGF-β pathway, and increases the AMPK-related signal pathway to alleviate impaired glucose metabolism and help with DM treatment, in a study of streptozotocin (STZ)-induced diabetic rats (Ko et al., 2019). Additionally, exercise also accelerates insulin sensitivity and glycogen storage of skeletal muscle by increasing glucose transporter 4 (GLUT4) expression (Holloszy, 2005; Richter and Hargreaves, 2013). Conversely, a recent study of skeletal muscle glucose uptake indicates that asprosin has three glycosylation sites and enhances glucose transport by elevating the level of GLUT4 in myotubes. Further, the glucose uptake is promoted by AMPK phosphorylation in skeletal muscle by exogenously administering asprosin (Zhang et al., 2021). These findings contradict other studies considering the differential effects of asprosin on various pathways, which deserves further research. Notably, different forms of exercise have diverse effects on DM improvement. A meta-analysis shows that both aerobic and resistance exercise are effective for the reduction of HbA1c, but combined exercise generates more improvement than either aerobic or resistance exercise (Pan et al., 2018).
In obesity, regular physical activity reduces adipose tissue and weight and improves fat browning by increasing UCP1 (Reisi et al., 2016; Mu et al., 2021). During exercise, triacylglycerols, an adipose tissue energy metabolite, are hydrolyzed into free fatty acids (FA), which are then released into the circulation to empower the muscle to function (Mika et al., 2019). After moderate intensity aerobic exercises, serum asprosin is significantly decreased in overweight and obese participants (Ceylan et al., 2020).
In PCOS, a previous meta-analysis concludes that vigorous-intensity exercise accelerates the indicators such as VO2peak, BMI, and waist circumference in patients, thereby improving cardiopulmonary function, insulin sensitivity, and ovulation function (Hakimi and Cameron, 2017; Patten et al., 2020). Asprosin is reported to downregulate mTOR expression, which inhibits follicular activation in PCOS (Wang and Hu, 2021; Zhang et al., 2022), while exercise decreases asprosin level and improves mTOR concentration (Ko et al., 2019; Stepto et al., 2020), so it is speculated that asprosin might play a role in alleviating PCOS with exercise.
Both NAFLD and thyroid function benefit from exercise (Farzanegi et al., 2019; Masaki et al., 2019). For instance, exercise enhances lipophagy to diminish liver steatosis by stimulating the AMPK/SIRT1 pathway (Li et al., 2021). In prophyltiouracil (PTU) induced hypothyroidism rats, asprosin level is lower than the control group, and after thyroxine treatment, asprosin is elevated (Mogulkoc et al., 2020). However, the role of asprosin in these conditions requires further investigation.
Summary
As a new protein discovered recently, asprosin has attracted great interest for its role in metabolism and diseases. In metabolic disorders such as DM, obesity, and PCOS, asprosin could serve as a biomarker for early diagnosis and a therapeutic target. Exercise, as an effective intervention for regulating metabolism and endocrine activity, is a preferred non-pharmacological therapy in many metabolic diseases. The regulation of asprosin on metabolic abnormalities and the important role of exercise are summarized in Figure 4. Asprosin has a vital regulatory effect on the improvement of metabolic diseases by exercise and is expected to become an important target of exercise regulation in future scientific research and clinical practice.
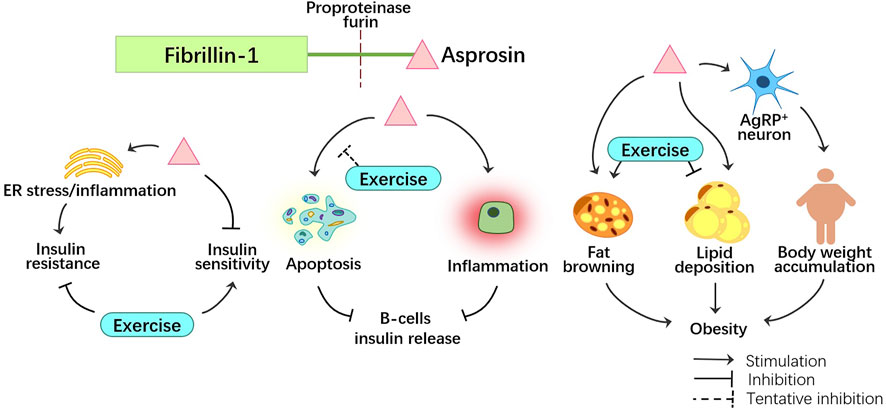
FIGURE 4. The role of asprosin in metabolic disorders, such as insulin resistance, decreased insulin release and obesity. Exercise plays an inhibitory role to asprosin, helping to mitigate metabolic disorders.
Author Contributions
LL, YL and MH wrote the manuscript. YL, MZ and CZ prepared figures. SB and XC revised the paper and assisted paper preparation. JX and JZ critically reviewed and edited the manuscript and supervised the study. All authors read and approved the final manuscript.
Funding
The work in the authors’ laboratory is supported by funding from National Natural Science Foundation of China (NO. 81572242) and Shanghai Key Laboratory of Human Sport Competence Development and Maintenance (Shanghai University of Sport, No.11DZ2261100) to JZ, and National Natural Science Foundation of China (Grant No. 82104883) and Science Technology Planning Project of Guangzhou (Grant No. 202102020930) to YL. YL was a visiting scholar to the University of Western Australia. It is partly supported by NHMRC APP1163933 to JX.
Conflict of Interest
The authors declare that the research was conducted in the absence of any commercial or financial relationships that could be construed as a potential conflict of interest.
Publisher’s Note
All claims expressed in this article are solely those of the authors and do not necessarily represent those of their affiliated organizations, or those of the publisher, the editors and the reviewers. Any product that may be evaluated in this article, or claim that may be made by its manufacturer, is not guaranteed or endorsed by the publisher.
References
Alan M., Gurlek B., Yilmaz A., Aksit M., Aslanipour B., Gulhan I., et al. (2019). Asprosin: a Novel Peptide Hormone Related to Insulin Resistance in Women with Polycystic Ovary Syndrome. Gynecol. Endocrinol. 35 (3), 220–223. doi:10.1080/09513590.2018.1512967
Alberti K. G. M. M., Zimmet P. Z. (1998). Definition, Diagnosis and Classification of Diabetes Mellitus and its Complications. Part 1: Diagnosis and Classification of Diabetes Mellitus. Provisional Report of a WHO Consultation. Diabet. Med. 15 (7), 539–553. doi:10.1002/(sici)1096-9136(199807)15:7<539::aid-dia668>3.0.co;2-s
Baykus Y., Yavuzkir S., Ustebay S., Ugur K., Deniz R., Aydin S. (2019). Asprosin in Umbilical Cord of Newborns and Maternal Blood of Gestational Diabetes, Preeclampsia, Severe Preeclampsia, Intrauterine Growth Retardation and Macrosemic Fetus. Peptides 120, 170132. doi:10.1016/j.peptides.2019.170132
Ceylan H. İ., Saygın Ö., Özel Türkcü Ü. (2020). Assessment of Acute Aerobic Exercise in the Morning versus Evening on Asprosin, Spexin, Lipocalin-2, and Insulin Level in Overweight/obese versus Normal Weight Adult Men. Chronobiology Int. 37 (8), 1252–1268. doi:10.1080/07420528.2020.1792482
Cobbina E., Akhlaghi F. (2017). Non-alcoholic Fatty Liver Disease (NAFLD) - Pathogenesis, Classification, and Effect on Drug Metabolizing Enzymes and Transporters. Drug Metab. Rev. 49 (2), 197–211. doi:10.1080/03602532.2017.1293683
DeFronzo R. A., Jacot E., Jequier E., Maeder E., Wahren J., Felber J. P. (1981). The Effect of Insulin on the Disposal of Intravenous Glucose: Results from Indirect Calorimetry and Hepatic and Femoral Venous Catheterization. Diabetes 30 (12), 1000–1007. doi:10.2337/diab.30.12.1000
DeFronzo R. A., Tripathy D. (2009). Skeletal Muscle Insulin Resistance Is the Primary Defect in Type 2 Diabetes. Diabetes Care 32 (Suppl. 2), S157–S163. doi:10.2337/dc09-S302
Deng X., Zhao L., Guo C., Yang L., Wang D., Li Y., et al. (2020). Higher Serum Asprosin Level Is Associated with Urinary Albumin Excretion and Renal Function in Type 2 Diabetes. Dmso Vol. 13, 4341–4351. doi:10.2147/DMSO.S283413
Deniz R., Yavuzkir S., Ugur K., Ustebay D. U., Baykus Y., Ustebay S., et al. (2021). Subfatin and Asprosin, Two New Metabolic Players of Polycystic Ovary Syndrome. J. Obstetrics Gynaecol. 41 (2), 279–284. doi:10.1080/01443615.2020.1758926
Donma M. M., Donma O. (2018). Asprosin: Possible Target in Connection with Ghrelin and Cytokine Network Expression in the Post-burn Treatment. Med. Hypotheses 118, 163–168. doi:10.1016/j.mehy.2018.07.008
Drapeau V., King N., Hetherington M., Doucet E., Blundell J., Tremblay A. (2007). Appetite Sensations and Satiety Quotient: Predictors of Energy Intake and Weight Loss. Appetite 48 (2), 159–166. doi:10.1016/j.appet.2006.08.002
Duerrschmid C., He Y., Wang C., Li C., Bournat J. C., Romere C., et al. (2017). Asprosin Is a Centrally Acting Orexigenic Hormone. Nat. Med. 23 (12), 1444–1453. doi:10.1038/nm.4432
Engin A. (2017). The Definition and Prevalence of Obesity and Metabolic Syndrome. Adv. Exp. Med. Biol. 960, 1–17. doi:10.1007/978-3-319-48382-5_1
Farzanegi P., Dana A., Ebrahimpoor Z., Asadi M., Azarbayjani M. A. (2019). Mechanisms of Beneficial Effects of Exercise Training on Non-alcoholic Fatty Liver Disease (NAFLD): Roles of Oxidative Stress and Inflammation. Eur. J. Sport Sci. 19 (7), 994–1003. doi:10.1080/17461391.2019.1571114
Fazakerley D. J., Krycer J. R., Kearney A. L., Hocking S. L., James D. E. (2019). Muscle and Adipose Tissue Insulin Resistance: Malady without Mechanism? J. Lipid Res. 60 (10), 1720–1732. doi:10.1194/jlr.R087510
Gonzalez-Gil A. M., Elizondo-Montemayor L. (2020). The Role of Exercise in the Interplay between Myokines, Hepatokines, Osteokines, Adipokines, and Modulation of Inflammation for Energy Substrate Redistribution and Fat Mass Loss: A Review. Nutrients 12 (6), 1899. doi:10.3390/nu12061899
Greenhill C. (2016). Asprosin - New Hormone Involved in Hepatic Glucose Release. Nat. Rev. Endocrinol. 12 (6), 312. doi:10.1038/nrendo.2016.66
Hakimi O., Cameron L.-C. (2017). Effect of Exercise on Ovulation: A Systematic Review. Sports Med. 47 (8), 1555–1567. doi:10.1007/s40279-016-0669-8
Hekim M. G., Kelestemur M. M., Bulmus F. G., Bilgin B., Bulut F., Gokdere E., et al. (2021). Asprosin, a Novel Glucogenic Adipokine: a Potential Therapeutic Implication in Diabetes Mellitus. Archives Physiology Biochem., 1–7. doi:10.1080/13813455.2021.1894178
Holloszy J. O. (20051985). Exercise-induced Increase in Muscle Insulin Sensitivity. J. Appl. Physiology 99 (1), 338–343. doi:10.1152/japplphysiol.00123.2005
Hruz T., Laule O., Szabo G., Wessendorp F., Bleuler S., Oertle L., et al. (20082008). Genevestigator V3: a Reference Expression Database for the Meta-Analysis of Transcriptomes. Adv. Bioinforma. 2008, 1–5. doi:10.1155/2008/420747
Jensen S. A., Aspinall G., Handford P. A. (2014). C-terminal Propeptide Is Required for Fibrillin-1 Secretion and Blocks Premature Assembly through Linkage to Domains cbEGF41-43. Proc. Natl. Acad. Sci. U.S.A. 111 (28), 10155–10160. doi:10.1073/pnas.1401697111
Jiang A., Feng Z., Yuan L., Zhang Y., Li Q., She Y. (2021). Effect of Sodium-Glucose Co-transporter-2 Inhibitors on the Levels of Serum Asprosin in Patients with Newly Diagnosed Type 2 Diabetes Mellitus. Diabetol. Metab. Syndr. 13 (1), 34. doi:10.1186/s13098-021-00652-5
Jung T. W., Kim H. C., Kim H. U., Park T., Park J., Kim U., et al. (2019). Asprosin Attenuates Insulin Signaling Pathway through PKCδ‐activated ER Stress and Inflammation in Skeletal Muscle. J. Cell. Physiology 234 (11), 20888–20899. doi:10.1002/jcp.28694
Ke F., Xue G., Jiang X., Li F., Lai X., Zhang M., et al. (2020). Combination of Asprosin and Adiponectin as a Novel Marker for Diagnosing Non-alcoholic Fatty Liver Disease. Cytokine 134, 155184. doi:10.1016/j.cyto.2020.155184
Ko J. R., Seo D. Y., Kim T. N., Park S. H., Kwak H.-B., Ko K. S., et al. (2019). Aerobic Exercise Training Decreases Hepatic Asprosin in Diabetic Rats. Jcm 8 (5), 666. doi:10.3390/jcm8050666
Kocaman N., Kuloğlu T. (2020). Expression of Asprosin in Rat Hepatic, Renal, Heart, Gastric, Testicular and Brain Tissues and its Changes in a Streptozotocin-Induced Diabetes Mellitus Model. Tissue Cell. 66, 101397. doi:10.1016/j.tice.2020.101397
Krook A., Björnholm M., Galuska D., Jiang X. J., Fahlman R., Myers M. G., et al. (2000). Characterization of Signal Transduction and Glucose Transport in Skeletal Muscle from Type 2 Diabetic Patients. Diabetes 49 (2), 284–292. doi:10.2337/diabetes.49.2.284
Lee T., Yun S., Jeong J. H., Jung T. W. (2019). Asprosin Impairs Insulin Secretion in Response to Glucose and Viability through TLR4/JNK-Mediated Inflammation. Mol. Cell. Endocrinol. 486, 96–104. doi:10.1016/j.mce.2019.03.001
Leonard A. N., Shill A. L., Thackray A. E., Stensel D. J., Bishop N. C. (2021). Fasted Plasma Asprosin Concentrations Are Associated with Menstrual Cycle Phase, Oral Contraceptive Use and Training Status in Healthy Women. Eur. J. Appl. Physiol. 121 (3), 793–801. doi:10.1007/s00421-020-04570-8
Li E., Shan H., Chen L., Long A., Zhang Y., Liu Y., et al. (2019). OLFR734 Mediates Glucose Metabolism as a Receptor of Asprosin. Cell. Metab. 30 (2), 319–328 e318. doi:10.1016/j.cmet.2019.05.022
Li H., Dun Y., Zhang W., You B., Liu Y., Fu S., et al. (2021). Exercise Improves Lipid Droplet Metabolism Disorder through Activation of AMPK-Mediated Lipophagy in NAFLD. Life Sci. 273, 119314. doi:10.1016/j.lfs.2021.119314
Li X., Liao M., Shen R., Zhang L., Hu H., Wu J., et al. (20182018). Plasma Asprosin Levels Are Associated with Glucose Metabolism, Lipid, and Sex Hormone Profiles in Females with Metabolic-Related Diseases. Mediat. Inflamm. 2018, 1–12. doi:10.1155/2018/7375294
Ma H., Zhang G., Mou C., Fu X., Chen Y. (2018). Peripheral CB1 Receptor Neutral Antagonist, AM6545, Ameliorates Hypometabolic Obesity and Improves Adipokine Secretion in Monosodium Glutamate Induced Obese Mice. Front. Pharmacol. 9, 156. doi:10.3389/fphar.2018.00156
Masaki M., Koide K., Goda A., Miyazaki A., Masuyama T., Koshiba M. (2019). Effect of Acute Aerobic Exercise on Arterial Stiffness and Thyroid-Stimulating Hormone in Subclinical Hypothyroidism. Heart Vessels 34 (8), 1309–1316. doi:10.1007/s00380-019-01355-8
Miao Y., Qin H., Zhong Y., Huang K., Rao C. (2021). Novel Adipokine Asprosin Modulates Browning and Adipogenesis in White Adipose Tissue. J. Endocrinol. 249 (2), 83–93. doi:10.1530/JOE-20-0503
Mika A., Macaluso F., Barone R., Di Felice V., Sledzinski T. (2019). Effect of Exercise on Fatty Acid Metabolism and Adipokine Secretion in Adipose Tissue. Front. Physiol. 10, 26. doi:10.3389/fphys.2019.00026
Mishra I., Xie W. R., Bournat J. C., He Y., Wang C., Silva E. S., et al. (2022). Protein Tyrosine Phosphatase Receptor δ Serves as the Orexigenic Asprosin Receptor. Cell. Metab. 34 (4), 549–563. doi:10.1016/j.cmet.2022.02.012
Mogulkoc R., Dasdelen D., Baltaci S. B., Baltaci A. K., Sivrikaya A. (2020). The Effect of Thyroid Dysfunction and Treatment on Adropin, Asprosin and Preptin Levels in Rats. Horm. Mol. Biol. Clin. Investig. 42 (1), 37–42. doi:10.1515/hmbci-2020-0058
Mu W.-J., Zhu J.-Y., Chen M., Guo L. (2021). Exercise-Mediated Browning of White Adipose Tissue: Its Significance, Mechanism and Effectiveness. Ijms 22 (21), 11512. doi:10.3390/ijms222111512
Naiemian S., Naeemipour M., Zarei M., Lari Najafi M., Gohari A., Behroozikhah M. R., et al. (2020). Serum Concentration of Asprosin in New-Onset Type 2 Diabetes. Diabetol. Metab. Syndr. 12, 65. doi:10.1186/s13098-020-00564-w
Oruc Y., Celik F., Ozgur G., Beyazyildiz E., Ugur K., Yardim M., et al. (2020). Altered Blood and Aqueous Humor Levels of Asprosin, 4-Hydroxynonenal, and 8-Hydroxy-Deoxyguanosine in Patients with Diabetes Mellitus and Cataract with and without Diabetic Retinopathy. Retina 40 (12), 2410–2416. doi:10.1097/IAE.0000000000002776
Ozcan S., Ulker N., Bulmus O., Yardimci A., Ozcan M., Canpolat S. (2020). The Modulatory Effects of Irisin on Asprosin, Leptin, Glucose Levels and Lipid Profile in Healthy and Obese Male and Female Rats. Archives Physiology Biochem. 128, 724–731. doi:10.1080/13813455.2020.1722706
Pan B., Ge L., Xun Y.-q., Chen Y.-j., Gao C.-y., Han X., et al. (2018). Exercise Training Modalities in Patients with Type 2 Diabetes Mellitus: a Systematic Review and Network Meta-Analysis. Int. J. Behav. Nutr. Phys. Act. 15 (1), 72. doi:10.1186/s12966-018-0703-3
Patten R. K., Boyle R. A., Moholdt T., Kiel I., Hopkins W. G., Harrison C. L., et al. (2020). Exercise Interventions in Polycystic Ovary Syndrome: A Systematic Review and Meta-Analysis. Front. Physiol. 11, 606. doi:10.3389/fphys.2020.00606
Petersen M. C., Shulman G. I. (2018). Mechanisms of Insulin Action and Insulin Resistance. Physiol. Rev. 98 (4), 2133–2223. doi:10.1152/physrev.00063.2017
Poblete-Aro C., Russell-Guzmán J., Parra P., Soto-Muñoz M., Villegas-González B., Cofré-Bola-Dos C., et al. (2018). Efecto del ejercicio físico sobre marcadores de estrés oxidativo en pacientes con diabetes mellitus tipo 2. Rev. Méd. Chile 146 (3), 362–372. doi:10.4067/s0034-98872018000300362
Reisi J., Ghaedi K., Rajabi H., Marandi S. M. (2016). Can Resistance Exercise Alter Irisin Levels and Expression Profiles of FNDC5 and UCP1 in Rats? Asian J. Sports Med. 7 (4), e35205. doi:10.5812/asjsm.35205
Richter E. A., Hargreaves M. (2013). Exercise, GLUT4, and Skeletal Muscle Glucose Uptake. Physiol. Rev. 93 (3), 993–1017. doi:10.1152/physrev.00038.2012
Romere C., Duerrschmid C., Bournat J., Constable P., Jain M., Xia F., et al. (2016). Asprosin, a Fasting-Induced Glucogenic Protein Hormone. Cell. 165 (3), 566–579. doi:10.1016/j.cell.2016.02.063
Sampath Kumar A., Maiya A. G., Shastry B. A., Vaishali K., Ravishankar N., Hazari A., et al. (2019). Exercise and Insulin Resistance in Type 2 Diabetes Mellitus: A Systematic Review and Meta-Analysis. Ann. Phys. Rehabilitation Med. 62 (2), 98–103. doi:10.1016/j.rehab.2018.11.001
Stepto N. K., Hiam D., Gibson-Helm M., Cassar S., Harrison C. L., Hutchison S. K., et al. (2020). Exercise and Insulin Resistance in PCOS: Muscle Insulin Signalling and Fibrosis. Endocr. Connect. 9 (4), 346–359. doi:10.1530/EC-19-0551
Sünnetçi Silistre E., Hatipoğl H. U. (2020). Increased Serum Circulating Asprosin Levels in Children with Obesity. Pediatr. Int. 62 (4), 467–476. doi:10.1111/ped.14176
Tabák A. G., Herder C., Rathmann W., Brunner E. J., Kivimäki M. (2012). Prediabetes: a High-Risk State for Diabetes Development. Lancet 379 (9833), 2279–2290. doi:10.1016/S0140-6736(12)60283-9
Ugur K., Aydin S. (20192019). Saliva and Blood Asprosin Hormone Concentration Associated with Obesity. Int. J. Endocrinol. 2019, 1–8. doi:10.1155/2019/2521096
Wang C.-Y., Lin T.-A., Liu K.-H., Liao C.-H., Liu Y.-Y., Wu V. C.-C., et al. (2019). Serum Asprosin Levels and Bariatric Surgery Outcomes in Obese Adults. Int. J. Obes. 43 (5), 1019–1025. doi:10.1038/s41366-018-0248-1
Wang M., Yin C., Wang L., Liu Y., Li H., Li M., et al. (2019). Serum Asprosin Concentrations Are Increased and Associated with Insulin Resistance in Children with Obesity. Ann. Nutr. Metab. 75 (4), 205–212. doi:10.1159/000503808
Wang R., Hu W. (2021). Asprosin Promotes β‐cell Apoptosis by Inhibiting the Autophagy of β‐cell via AMPK‐mTOR Pathway. J. Cell. Physiol. 236 (1), 215–221. doi:10.1002/jcp.29835
Wang R., Lin P., Sun H., Hu W. (2021). Increased Serum Asprosin Is Correlated with Diabetic Nephropathy. Diabetol. Metab. Syndr. 13 (1), 51. doi:10.1186/s13098-021-00668-x
Wang Y., Qu H., Xiong X., Qiu Y., Liao Y., Chen Y., et al. (2018). Plasma Asprosin Concentrations Are Increased in Individuals with Glucose Dysregulation and Correlated with Insulin Resistance and First-phase Insulin Secretion. Mediat. Inflamm. 2018, 1–7. doi:10.1155/2018/9471583
Wiecek M., Szymura J., Maciejczyk M., Kantorowicz M., Szygula Z. (2018). Acute Anaerobic Exercise Affects the Secretion of Asprosin, Irisin, and Other Cytokines - A Comparison between Sexes. Front. Physiol. 9, 1782. doi:10.3389/fphys.2018.01782
Wiecek M., Szymura J., Sproull J., Szygula Z. (2019). Decreased Blood Asprosin in Hyperglycemic Menopausal Women as a Result of Whole-Body Cryotherapy Regardless of Metabolic Syndrome. Jcm 8 (9), 1428. doi:10.3390/jcm8091428
Williamson D. A. (2018). Prevention of Type 2 Diabetes: what Is the Right Target Population? Expert Rev. Endocrinol. Metabolism 13 (6), 295–305. doi:10.1080/17446651.2018.1535896
Zhang H., Hu W., Zhang G. (2020). Circulating Asprosin Levels Are Increased in Patients with Type 2 Diabetes and Associated with Early-Stage Diabetic Kidney Disease. Int. Urol. Nephrol. 52 (8), 1517–1522. doi:10.1007/s11255-020-02509-8
Zhang L., Chen C., Zhou N., Fu Y., Cheng X. (2019). Circulating Asprosin Concentrations Are Increased in Type 2 Diabetes Mellitus and Independently Associated with Fasting Glucose and Triglyceride. Clin. Chim. Acta 489, 183–188. doi:10.1016/j.cca.2017.10.034
Zhang X., Jiang H., Ma X., Wu H. (2020). Increased Serum Level and Impaired Response to Glucose Fluctuation of Asprosin Is Associated with Type 2 Diabetes Mellitus. J. Diabetes Investig. 11 (2), 349–355. doi:10.1111/jdi.13148
Zhang X., Zhang W., Wang Z., Zheng N., Yuan F., Li B., et al. (2022). Enhanced Glycolysis in Granulosa Cells Promotes the Activation of Primordial Follicles through mTOR Signaling. Cell. Death Dis. 13 (1), 87. doi:10.1038/s41419-022-04541-1
Zhang Y., Zhu Z., Zhai W., Bi Y., Yin Y., Zhang W. (2021). Expression and Purification of Asprosin in Pichia pastoris and Investigation of its Increase Glucose Uptake Activity in Skeletal Muscle through Activation of AMPK. Enzyme Microb. Technol. 144, 109737. doi:10.1016/j.enzmictec.2020.109737
Zhang Z., Tan Y., Zhu L., Zhang B., Feng P., Gao E., et al. (2019). Asprosin Improves the Survival of Mesenchymal Stromal Cells in Myocardial Infarction by Inhibiting Apoptosis via the Activated ERK1/2-SOD2 Pathway. Life Sci. 231, 116554. doi:10.1016/j.lfs.2019.116554
Keywords: asprosin, exercise, metabolism disease, T2DM, obesity, pcos
Citation: Liu L, Liu Y, Huang M, Zhang M, Zhu C, Chen X, Bennett S, Xu J and Zou J (2022) The Effects of Asprosin on Exercise-Intervention in Metabolic Diseases. Front. Physiol. 13:907358. doi: 10.3389/fphys.2022.907358
Received: 30 March 2022; Accepted: 07 June 2022;
Published: 11 July 2022.
Edited by:
Tania Romacho, University of Almeria, SpainReviewed by:
Nevena Jeremic, University of Kragujevac, SerbiaCopyright © 2022 Liu, Liu, Huang, Zhang, Zhu, Chen, Bennett, Xu and Zou. This is an open-access article distributed under the terms of the Creative Commons Attribution License (CC BY). The use, distribution or reproduction in other forums is permitted, provided the original author(s) and the copyright owner(s) are credited and that the original publication in this journal is cited, in accordance with accepted academic practice. No use, distribution or reproduction is permitted which does not comply with these terms.
*Correspondence: Jun Zou, anVuem91QHN1cy5lZHUuY24=; Jiake Xu, amlha2UueHVAdXdhLmVkdS5hdQ==
†These authors have contributed equally to this work