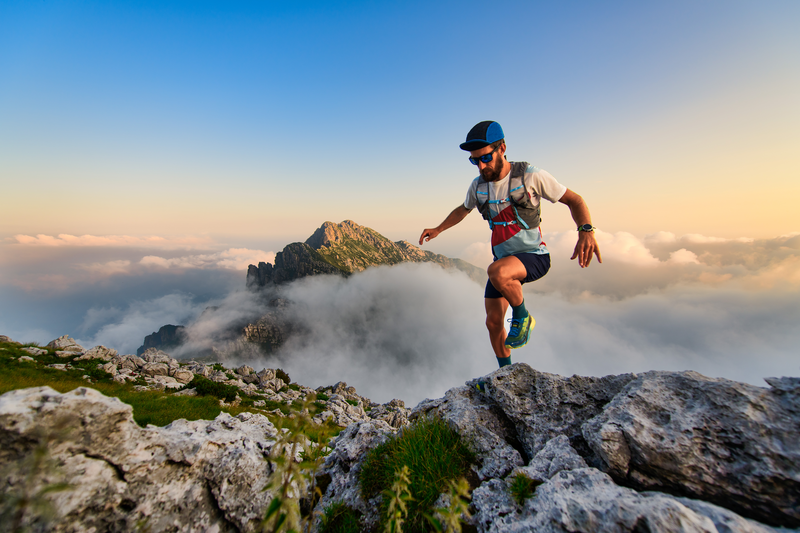
95% of researchers rate our articles as excellent or good
Learn more about the work of our research integrity team to safeguard the quality of each article we publish.
Find out more
ORIGINAL RESEARCH article
Front. Physiol. , 24 May 2022
Sec. Red Blood Cell Physiology
Volume 13 - 2022 | https://doi.org/10.3389/fphys.2022.904280
This article is part of the Research Topic Comparative Biology of Red Blood Cells View all 5 articles
Red cells from LK sheep represent an important paradigm for control of KCl cotransport activity, as well as being important to sheep erythroid function. A previous report (Godart et al., 1997) suggested that autologous plasma markedly inhibits red cell KCC activity and identified the presence of the bicarbonate/CO2 buffer system as the probable cause. Findings were restricted, however, to red cells from patients with sickle cell disease (SCD) swollen anisotonically and carried out at a very high O2 tension (c.700 mmHg). It was therefore important to investigate the generality of the effect described and whether it was also relevant to the two main stimuli for KCC activity encountered most often by circulating red cells in vivo - low pH in active muscle beds during exercise and high urea concentrations in the renal medulla during antidiuresis. Results confirm that inhibition was significant in response to anisotonic swelling with KCC activity in MOPS-buffered saline (MBS) vs. bicarbonate-buffered saline (BBS) and in MBS vs. plasma both reduced (by about 25 and 50%, respectively). By contrast, however, inhibition was absent at low pH and in high concentrations of urea. These findings suggest therefore that red cell KCC activity represents an important membrane permeability in vivo in red cells suspended in plasma. They are relevant, in particular, to sheep red cells, and may also be important by extension to those of other species and to the abnormal red cells found in human patients with SCD.
It has become increasingly apparent that red cells are not simply quiescent carrier vehicles for haemoglobin (Hb) but rather have the ability to contribute to a rich complexity of responses. Physiologically, they participate in the overall homeostasis of organisms (eg from this journal: Pretini et al., 2019; Bogadnova and Kaestner, 2020), whilst, pathologically, their abnormal behaviour may contribute to a number of disease states (eg from elsewhere: Gallagher, 2017; Bernard, 2021). In humans, much evidence pertaining to the diversity of red cell function has been recently derived from a better appreciation of red cell phenotype reflects genotype in both healthy individuals and in patients with various disease states. By contrast, red cells from other animals have received relatively little attention.
Historically, comparative red cell research has provided an important resource for study. The behaviour of red cells from species across the animal kingdom—from lower vertebrates like Amphiuma to primates—illustrates the differing requirements of various species and how red cell function is adapted to their biology (Nikinmaa, 1990). Notable differences include the absence of band 3/anion exchanger in primitive fishes to the various energy sources for red cell glycolysis, as exemplified in mammals by the role of inosine (rather than glucose) in pig red cells, or by the presence of mitochondria in lower vertebrates, and to the way in which intracellular pH is regulated to modify Hb oxygen affinity.
A major transport function, Cl−-dependent cation transport, is present in the membranes of red cells from many different animals. Evidence for Cl−-cation cotransporters was first suggested in the late 1970s by work in Ehrlich ascites tumour cells (Hoffmann, 1978; Geck et al., 1980), and in low potassium-containing (LK) sheep red cells, and in humans (Dunham et al., 1980; Ellory and Dunham, 1980; Lauf and Theg, 1980). These systems are now established as protein-mediated coupled fluxes, as opposed to ones activated by the presence of Cl−. Three systems predominate: the first two, with Cl− requiring the presence of both K+ and/or Na+, the Na+-K+-2Cl− cotransporter (NKCC) and the Na+-Cl− cotransporter (NCC); and, the third, a system dependent only K+ in addition to Cl−, the K+-Cl− cotransporter (KCC). Their cloning and the establishment of the Cl−-cation cotransport family (SLC12A family including NCC; NKCC; KCC) has established that they function as true cotransport systems in numerous settings and many species (Herbert et al., 2004; Gamba, 2005).
Both KCC and NKCC, but not NCC, are widely present in red cells from different animals. They have a complex regulation which is often reciprocal but which is still incompletely understood. Physiological modulators include cell volume (Ellory and Dunham, 1980; Dunham and Ellory, 1981), intracellular pH (Ellory et al., 1991), intracellular [Cl−] (Voets et al., 1999), extracellular [K+] and [Cl−] (Lauf et al., 1992), urea (Dunham, 1995; Kaji and Gasson, 1995; Speake and Gibson, 1997), temperature (Khan and Ellory, 2000) and O2 tension (Borgese et al., 1991; Nielsen et al., 1992; Campbell and Gibson, 1998; Gibson et al., 1998; Gibson et al., 2000). Using protein kinase and phosphatase inhibitors of differing degrees of specificity (such as okadaic acid, calyculin, staurosporine, genistein, imantanib, etc), pharmacological studies have shown an important role for protein phosphorylation (Jennings and Al-Rohil, 1990; Jennings and Schulz, 1991; Starke and Jennings, 1993; Cossins et al., 1994; Flatman et al., 1996; Bize et al., 1999; Bize et al., 2000; Campbell et al., 2000).
Over the years, the behaviour of KCC in LK sheep red cells has provided an important paradigm for that in human red cells and in other species (eg Ellory and Dunham, 1980; Lauf and Theg, 1980; Lauf et al., 1992; Dunham, 1995; Campbell and Gibson, 1998; Campbell et al., 2000), and remains a valuable model system as well as being important in sheep erythrogenesis (Lauf and Bauer, 1987). It is worth noting that the genes for KCC are well conserved between human and sheep (eg for KCC1/SLC12A4 - https://www.ncbi.nim.ni.gov/gene6560 &/gene443178) and that sheep red cells have a similar low oxygen affinity to sickle cells (Samaja and Gattioni, 1978; Correra et al., 1984), which may be relevant when considering an oxygen-sensitive membrane transport system. This report focuses on the effect of plasma, as almost exclusively, studies investigating red cell KCC have used artificial media—simple salt solutions with synthetic buffers like HEPES, TRIS and MOPS—in which to suspend red cells whilst measuring K+ transport. A paper published towards the end of the 1990s, however, provocatively suggested that more physiological solutions—a bicarbonate-buffered saline (BBS) mimicking plasma, or plasma itself—contain constituents which markedly inhibit red cell KCC activity when compared to that measured in simple artificial saline (Godart et al., 1997). Red cell swelling was used as the stimulus for KCC activity, with cells suspended in MOPS-buffered saline (MBS), BBS or autologous plasma. An inhibition of KCC activity of over 80%, elicited by the presence of bicarbonate/CO2, rather than by that of some other component of plasma. The authors provokingly considered whether high levels of KCC activity may actually occur in circulating red cells in vivo (Godart et al., 1997). Their report, however, focussed on abnormal red cells from patients with sickle cell disease—which have several differences to those from normal individuals, not least a high reticulocyte count - whilst only anisotonic swelling was tested—which is unlikely to occur to a significant extent in vivo—and, in addition, very high O2 tensions (about 700 mmHg O2) were used—which might be problematical in an O2-sensitive system (Gibson et al., 2000). The applicability of their findings more generally therefore warrants further scrutiny.
This report focuses on the role of plasma in modulation of KCC activity in normal red cells from healthy LK sheep. As well as using anisotonic swelling as a stimulus, low pH and high levels of urea were also tested, as these represent the main physiological stimuli in vivo. In addition, a more realistic value for O2 tension (100 mmHg), approximating the maximum normally encountered in the arterial system in vivo, was used. Results suggest that plasma or the bicarbonate/CO2 buffer system is not as inhibitory as proposed and that KCC activity is therefore relevant to the function of red cells in vivo in the circulation.
All chemicals and inhibitors came from Sigma-Aldrich (Poole, Dorset, United Kingdom) unless otherwise stated. 86Rb+ came from Perkin-Elmer (Beaconsfield, Bucks., United Kingdom). Nitrogen, oxygen and carbon dioxide were from BOC Ltd. (Guildford, Surrey, United Kingdom).
Sheep blood samples were obtained freshly from a commercial source (TCS Biosciences Ltd., Botolph Claydon, United Kingdom) using heparin as an anticoagulant. Samples were immediately refrigerated or kept on ice until use, within two days. Whole blood was centrifuged gently at 600 g and the plasma removed and also stored on ice. Red cells were then washed in MOPS-buffered saline (see below) to remove any residual plasma and the buffy coat. Washed red cells and cell-free plasma were then stored separately on ice. Red cell and plasma [K+]s were measured by The Queen’s Veterinary School Hospital Central Diagnostic Services, University of Cambridge. For this purpose, red cell aliquots were lysed in ten-times their own volume of distilled water. [K+] of red cells varied from 7 to 16 mM (12.8 ± 1.6, mean ± S.E.M., n = 11), confirming that they came from LK sheep; that of plasma varied from 4.1 to 5.1 mM (4.2 ± 0.2, mean ± S.E.M., n = 11) and was adjusted through addition of KNO3 (100 mM) to a final value of 7.5 mM. Red cell volume was assessed as water content following the method of Borgese et al. (1987) and expressed as ml water per g dry cell solids (ml.g−1 d.c.s.).
MOPS-buffered saline (MBS) comprised (in mM): NaCl 137.5, KNO3 7.5, MOPS 10, glucose 5, pH 7.4 at 37°C. For bicarbonate-buffered saline (BBS), 24 mM NaCl was replaced with NaHCO3. Wash solution (W-MBS) for removing unincorporated radioisotope was isotonic MgCl2 solution: MgCl2 107, MOPS 10, pH 7.4 at 0°C. All solutions had an osmolality of 290 mOsm.kg−1 and a pH of 7.4, unless the effect of anisotonicity or pH was being studied when media of pH 7.0 and 6.5 were also prepared, and the effect of added hypertonic sucrose and water tested. For MBS, pHs were adjusted through addition of NaOH or HNO3 (in the absence of CO2), whilst for BBS and plasma, the media was equilibrated with the requisite partial pressure of CO2 (see below).
KCC activity in red cells from many species is O2-sensitive (Gibson et al., 2000). It was therefore important to regulate O2 tension during incubation. Cells were gently rotated at 37°C in Eschweiler tonometers, coupled to a Wösthoff gas mixing pump (Speake et al., 1997) to set the O2 tension at the requisite level, either 677 or 100 mmHg O2 by flushing with pre-warmed and humidified air or 100% O2 mixed with N2. When using BBS or plasma, the gas mixture also included CO2 at a partial pressure necessary to take the pH to 7.4, 7.0 or 6.5 (typically 43 mmHg, 100 mmHg or 321 mmHg CO2, respectively). Cell suspensions were placed in the tonometers at ten-fold the haematocrit (Hct) needed for transport assay and equilibrated with the requisite gas mixture. They were then diluted ten-fold into test tubes, also pre-equilibrated at the required O2 and CO2 levels. Tubes were also gassed during incubation, but not bubbled (to prevent red cell lysis). Fully humidified gases at 37°C were used, which is necessary to prevent dehydration of the samples and to prevent condensation. All glassware and tubing was submerged and kept at 37°C.
86Rb+ was used as a K+ congener. After dilution of the red cell samples into the test tubes, the influx was started by addition of 86Rb+ (final activity 0.02–0.05 MBq.ml−1) in distilled water at a 1 in 20 dilution. The duration of uptake was 10 min—control experiments have established that uptake is linear over this time period—and determinations were carried out in triplicates or quadruplicates. Uptake was stopped by diluting aliquots of the cell suspension into ice-cold W-MBS. Previous work has shown that K+ uptake achieves the new steady state within a minute or so of altering media conditions and that this is maintained for the duration of the incubations described here (eg Jennings and Al-Rohil, 1990). Unincorporated 86Rb+ was removed by centrifugation (10 s at 15,000 g), aspiration of supernatant and addition of further wash solution (4 washes and 5 spins in total). After each centrifugation step, cells were resuspended by gentle vortexing. Following the final wash, the cell pellet was lysed with Triton X-100 (0.1%) and protein (mainly haemoglobin in the case of red cells) precipitated with trichloroacetic acid (TCA, 5%). A final centrifugation step was used to harvest the clear, colourless supernatant before counting Čerenkov radiation by liquid scintillation (Packard Tri-carb 2800TR). Ouabain (100 µM) and bumetanide (10 µM) were present during all transport assays to inhibit K+ uptake via the Na+/K+ pump and Na+-K+-2Cl− cotransporter (NKCC), respectively. K+ influx was calculated in mmol K+(l cells.h)−1. Under the conditions used, Cl−-independent K+ influx or K+ influx via NKCC were minimal, < 0.1 mmol K+ (l cells.h)−1, less than 5% of the total influx, so that the values given are taken as measures of KCC activity.
Data are given as means ± S.E.M. for samples from n different sheep. K+ influxes at different tonicities, pHs or in the presence/absence of urea were all made simultaneously in paired samples from the same sheep, with red cells incubated in each of the three different media at the same time. Statistical comparisons were made using Student’s t test for paired samples and ANOVA where more than two conditions were compared. A value of p < 0.05 taken as significant.
The activity of KCl cotransport (KCC) in red cells from LK sheep is markedly affected by pH, volume, O2 tension and temperature, as well as by the concentrations of extracellular K+ and Cl−. All of these were therefore controlled carefully. Red cell water contents in MOPS -buffered saline (MBS), bicarbonate-buffered saline (BBS) and plasma were 1.97 ± 0.04, 1.97 ± 0.04 and 1.97 ± 0.04 ml g−1 d.c.s. (N.S.), respectively, showing that cell volume was not affected by the media used. This finding is consistent with modulation by intracellular pH and anisotonicity having the main effects on red cell volume. KCC activity was also compared in the different media equilibrated with an O2 tension of either 100 mmHg O2 or 677 mmHg, the latter value used by Godart et al. (1997). The magnitude of fluxes was reduced by about 25% at the lower O2 tension—for example at pH 7.4 K+ influx reduced from 2.20 ± 0.24 mmol (l cells.h)−1 to 1.62 ± 0.32 (n = 3)—but otherwise the pattern of responses to different modalities of stimulus (volume, pH and urea) and the effect of the incubation in the two other incubation media, BBS or plasma, were similar and unaffected by the two O2 tensions tested. In the following, results are therefore shown only for an O2 of 100 mmHg.
The following series of experiments investigated the effects of incubation media on KCC activity altered through anisotonicity, with LK sheep red cells shrunken or swollen by 15% through addition of water or hypertonic sucrose (Figure 1). Cell volumes were not measured but previous work has established that alterations due to anisotonic media are complete. In all three media, anisotonic swelling significantly increased KCC activity by about 50%, consistent with previous reports (Dunham et al., 1980; Dunham and Ellory, 1981; Lauf and Adragna, 1992). KCC activity was highest in MBS, followed by BBS, and lowest in plasma. Fluxes in swollen red cells was reduced by about 10% comparing BBS with MBS, and by about 25% comparing plasma with MBS. Reduction in the component of KCC activity altered by aniostonicity, defined as the difference in K+ influx in red cells swollen compared with shrunken ones (= volume-sensitive K+ flux), was about 25% comparing BBS with MBS and about 50% comparing plasma with MBS (Figure 1). Although percentage inhibition was more modest in the bicarbonate-buffered media, these findings were similar to those described previously in red cells from SCD patients whose volume was altered similarly by an anisotonicity of 15% but at high O2 tension, about 700 mmHg (Godart et al., 1997), and in which the corresponding inhibition of volume-sensitive K+ fluxes was about 80% (MBS to BBS) and 75% (MBS to plasma). Inhibition was not due to altered red cell volume in the different media (Godart et al., 1997 and see above). In both the present work and that of Godart et al. (1997), K+ fluxes in shrunken red cells were unaffected by the medium used.
FIGURE 1. The effect of incubation media on volume-sensitive KCl cotransport activity in LK sheep red cells. Red cells (50% Hct) from LK sheep were equilibrated in Eschweiler tonometers for 15 min at an O2 tension of 100 mmHg under isotonic conditions (osmolality 290 mOsm.kg−1). Extracellular pH was pH 7.4, adjusted using HNO3/NaOH in MOPS-buffered saline (MBS), or with CO2 (43 mmHg) in the case of bicarbonate-buffered saline (BBS) and plasma. Red cell aliquots were then diluted ten-fold into test tubes pre-equilibrated with the same gas mixtures to alter cell volume anisotonically and for measurement of K+ influx (extracellular [K+] of 7.5 mM) over 10 min. Cell volume was altered through addition of hypertonic sucrose (15% shrunken; SH) or water (15% swollen; SW). All K+ influxes were carried out in the presence of ouabain (100 µM) and bumetanide (10 µM) to inhibit K+ influx through the Na+/K+ pump and Na+-K+-Cl- cotransporter (NKCC), respectively. The volume-sensitive component of the K+ flux (VSF) represents the changes induced by altered (at ± 15% osmolality cf isotonic conditions). Histograms represent means ± S.E.M., n = 4. ∗p ≤ 0.05; ∗∗p ≤ 0.01.
The effect of altered extracellular pH was then examined (Figure 2). The response of LK sheep red cell KCC activity showed a bell-shaped response, as described previously (Lauf and Adragna, 1992), with increased activity as pH fell from pH 7.4 to pH 7.0, with a subsequent decrease as pH was further reduced to pH 6.5. At pH 7.0, KCC activity was not significantly different regardless of which incubation medium was used. At pH 7.4 and 6.5, activity was lowest in MBS, and no difference was observed in that measured in BBS or plasma. As a consequence, the pH-sensitive component of KCC activity (defined as the increase in K+ influx at pH 7.0 compared to that at pH 7.4) declined by about 40 and 50%, comparing MBS with BBS or plasma, respectively. The magnitude of the pH-sensitive component was not significantly altered, however, when comparing BBS and plasma (Figure 2). Overall, the reduction in pH-sensitive components of KCC activity in LK sheep red cells, by incubation in BBS or plasma compared with those measured in MBS, was due to the reduced activity measured in MBS at pH 7.4, and not due to decreased fluxes in BBS and plasma when measured at pH 7.0 or 6.5. In fact, the magnitude of KCC activity at these lower pHs was the same as in MBS or higher.
FIGURE 2. The effect of incubation media on pH-sensitive KCl cotransport activity in LK sheep red cells. Red cells (50% Hct) from LK sheep were equilibrated in Eschweiler tonometers for 15 min at an O2 tension of 100 mmHg under isotonic conditions (osmolality 290 mOsm.kg−1). The pHs of the incubation media were pH 7.4, pH 7.0 and pH 6.5, adjusted using HNO3/NaOH in MBS, but with CO2 in the case of BBS and plasma (with a CO2 of 43 mmHg at pH 7.4, 100 mmHg at pH 7.0 and 321 mmHg at pH 6). RBC aliquots were then diluted ten-fold into test tubes pre-equilibrated with the same gas mixtures for measurement of K+ influx (extracellular [K+] of 7.5 mM) over 10 min. All influxes were carried out in the presence of ouabain (100 µM) and bumetanide (10 µM) to inhibit K+ influx through the Na+/K+ pump and NKCC, respectively. Histograms represent means ± S.E.M., n = 3. ∗p ≤ 0.05.
In the last series of experiments, the stimulatory action of urea was investigated. Incubation at high concentrations of urea (750 mM) significantly increased KCC activity in all three media (Figure 3). In this case, the elevated values observed in the presence of urea were not significantly different in any of the media. Values measured in its absence at pH 7.4 were also similar across the three media, although the trend was for lower fluxes in MBS compared to BBS and plasma, similar to that observed in the previous experiment (Figure 2). The urea-stimulated component (defined as the increase in KCC activity in the presence of urea compared with that measured in its absence) was therefore also unaffected by the incubation media (Figure 3).
FIGURE 3. The effect of incubation media on urea-stimulated KCl cotransport activity in LK sheep red cells. Red cells (50% Hct) from LK sheep were equilibrated in Eschweiler tonometers for 15 min at an O2 tension of 100 mmHg under isotonic conditions (osmolality 290 mOsm.kg−1). Extracellular pH was pH 7.4, adjusted using HNO3/NaOH in MBS, or with CO2 (43 mmHg) in the case of BBS and plasma. Red cell aliquots were then diluted ten-fold into test tubes pre-equilibrated with the same gas mixtures for measurement of K+ influx (extracellular [K+] of 7.5 mM) over 10 min, in the continued absence of urea (-) or in its presence (+) at 750 mM. All influxes were carried out in the presence of ouabain (100 µM) and bumetanide (10 µM) to inhibit K+ influx through the Na+/K+ pump and NKCC, respectively. The urea-sensitive K+ flux (USF) represents the changes of flux induced by the presence of high urea concentrations (750 mM) compared with that measured in its absence. Histograms represent means ± S.E.M., n = 4. ∗p ≤ 0.05; n.s. indicates “not significant”.
In human red cells from patients with SCD, plasma markedly reduced KCC activity (Godart et al., 1997). This effect, however, was only determined in anisotonically swollen sickle cells. A careful analysis showed that inhibition was due predominantly to the presence of the physiological bicarbonate/CO2 buffer system, and not to physiological levels of Ca2+, Mg2+, phosphate or any alteration in cell volume between the different media. Both Vmax and affinity (ie increased Km) were reduced. There was no such inhibitory action of plasma in red cells whose KCC activity was pharmacologically activated with protein kinase inhibitors, staurosporine and N-ethylmaleimide, indicating that the effect was likely via the regulatory phosphorylation cascade. The present results using red cells from LK sheep confirmed inhibition by bicarbonate/CO2 and plasma under anisotonic swelling. However, it was noticeable that stimulation by the two modalities most commonly encountered by circulating red cells in vivo, low pH and urea, was unaffected. We conclude that a significant inhibitory effect of the constituents of plasma may not be universal. This may be relevant to a role for red cell KCC in sheep and other species. For example, altered red cell pH is important for maternofetal oxygen transfer (Bunn, 1981; Nikinmaa, 1990). It may also be especially significant in human sickle cells in which hyperactivity of KCC is proposed to contribute significantly to red cell dehydration (Brugnara et al., 1986) and hence represents an important mechanism in pathogenesis of the disease. Finally, as the roles of other mechanosensitive transport systems notably PIEZO1 become clearer in healthy red cells and various disease states (eg Gallagher, 2017), it would be interesting to investigate some of the multiple modalities of stimulus to which KCC has been shown to respond (oxygen, pH, urea), and to also include any effect of incubation media on these other transporters (Bernhardt and Ellory, 2003)1.
The raw data supporting the conclusion of this article will be made available by the authors, without undue reservation.
JG planned the study; DL and AH carried out the experiments and analysed the data; DL, AH, and JG wrote the manuscript.
The authors declare that the research was conducted in the absence of any commercial or financial relationships that could be construed as a potential conflict of interest.
All claims expressed in this article are solely those of the authors and do not necessarily represent those of their affiliated organizations, or those of the publisher, the editors and the reviewers. Any product that may be evaluated in this article, or claim that may be made by its manufacturer, is not guaranteed or endorsed by the publisher.
We thank J. Clive Ellory for helpful discussions.
1Ncbi. https://www.ncbi.nim.ni.gov/gene6560 &/gene443178.
Bernard N. J. (2021). Another Function for Red Blood Cells. Nat. Immunol. 22, 1469. doi:10.1038/s41590-021-01081-2
Bernhardt I., Ellory J. C. (2003). Red Cell Membrane Transport in Health and Disease. Berlin Heidelberg New York: Springer.
Bize I., Güvenç B., Buchbinder G., Brugnara C. (2000). Stimulation of Human Erythrocyte K-Cl Cotransport and Protein Phosphatase Type 2A by N-Ethylmaleimide: Role of Intracellular Mg ++. J. Membr. Biol. 177 (2), 159–168. doi:10.1007/s002320001109
Bize I., Güvenç B., Robb A., Buchbinder G., Brugnara C. (1999). Serine/threonine Protein Phosphatases and Regulation of K-Cl Cotransport in Human Erythrocytes. Am. J. Physiology-Cell Physiology 277 (5), C926–C936. doi:10.1152/ajpcell.1999.277.5.c926
Bogdanova A., Kaestner L. (2020). Early Career Scientists' Guide to the Red Blood Cell - Don't Panic!. Front. Physiol. 11, 588. doi:10.3389/fphys.2020.00588
Borgese F., Garcia-Romeu F., Motais R. (1987). Control of Cell Volume and Ion Transport by Beta-Adrenergic Catecholamines in Erythrocytes of Rainbow Trout, Salmo Gairdneri. J. Physiology 382, 123–144. doi:10.1113/jphysiol.1987.sp016359
Borgese F., Motais R., García-Romeu F. (1991). Regulation of Cl-dependent K Transport by Oxy-Deoxyhemoglobin Transitions in Trout Red Cells. Biochimica Biophysica Acta (BBA) - Biomembr. 1066, 252–256. doi:10.1016/0005-2736(91)90194-d
Brugnara C., Bunn H. F., Tosteson D. C. (1986). Regulation of Erythrocyte Cation and Water Content in Sickle Cell Anemia. Science 232 (4748), 388–390. doi:10.1126/science.3961486
Bunn H. (1981). Evolution of Mammalian Hemoglobin Function. Blood 58, 189–197. doi:10.1182/blood.v58.2.189.bloodjournal582189
Campbell E. H., Ellory J. C., Gibson J. S. (2000). Effects of Protein Kinase and Phosphatase Inhibitors and Anti-L Antisera on K+ Transport in LK Sheep Red Cells. Bioelectrochemistry 52, 151–159. doi:10.1016/s0302-4598(00)00097-0
Campbell E. H., Gibson J. S. (1998). Oxygen-dependent K+fluxes in Sheep Red Cells. J. Physiology 506 (3), 679–688. doi:10.1111/j.1469-7793.1998.679bv.x
Correra A., Graziano J. H., Seaman C., Piomelli S. (1984). Inappropriately Low Red Cell 2,3-diphosphoglycerate and P50 in Transfused Beta-Thalassemia. Blood 63, 803–806.
Cossins A. R., Weaver Y. R., Lykkeboe G., Nielsen O. B. (1994). Role of Protein Phosphorylation in Control of K Flux Pathways of Trout Red Blood Cells. Am. J. Physiology-Cell Physiology 267 (6), C1641–C1650. doi:10.1152/ajpcell.1994.267.6.c1641
Dunham P. B. (1995). Effects of Urea on K-Cl Cotransport in Sheep Red Blood Cells: Evidence for Two Signals of Swelling. Am. J. Physiology-Cell Physiology 268 (4), C1026–C1032. doi:10.1152/ajpcell.1995.268.4.c1026
Dunham P. B., Ellory J. C. (1981). Passive Potassium Transport in Low Potassium Sheep Red Cells: Dependence upon Cell Volume and Chloride. J. Physiology 318 (1), 511–530. doi:10.1113/jphysiol.1981.sp013881
Dunham P. B., Stewart G. W., Ellory J. C. (1980). Chloride-activated Passive Potassium Transport in Human Erythrocytes. Proc. Natl. Acad. Sci. U.S.A. 77, 1711–1715. doi:10.1073/pnas.77.3.1711
Ellory J. C., Dunham P. B. (1980). “Volume-dependent Passive Potassium Transport in LK Sheep Red Cells,” in Membrane Transport in Erythrocytes. Editors U. V. Lassen, H. H. Ussing, and J. O. Wieth (Copenhagen: Munksgaard), 406–427.
Ellory J. C., Hall A. C., Ody S. A., de Figueiredos C. E. P., Chalder S., Stuart J. (1991). KCl Cotransport in HbAA and HbSS Red Cells: Activation by Intracellular Acidity and Disappearance during Maturation. Adv. Exp. Med. Biol. 307, 47–57. doi:10.1007/978-1-4684-5985-2_5
Flatman P. W., Adragna N. C., Lauf P. K. (1996). Role of Protein Kinases in Regulating Sheep Erythrocyte K-Cl Cotransport. Am. J. Physiology-Cell Physiology 271 (1), C255–C263. doi:10.1152/ajpcell.1996.271.1.c255
Gallagher P. G. (2017). Disorders of Erythrocyte Hydration. Blood 130, 2699–2708. doi:10.1182/blood-2017-04-590810
Gamba G. (2005). Molecular Physiology and Pathophysiology of Electroneutral Cation-Chloride Cotransporters. Physiol. Rev. 85, 423–493. doi:10.1152/physrev.00011.2004
Geck P., Pietrzyk C., Burckhardt B.-C., Pfeifferl B., Heinz E. (1980). Electrically Silent Cotransport of Na+, K+ and Cl− in Ehrlich Cells. Biochimica Biophysica Acta (BBA) - Biomembr. 600, 432–447. doi:10.1016/0005-2736(80)90446-0
Gibson J. S., Cossins A. R., Ellory J. C. (2000). Oxygen-sensitive Membrane Transporters in Vertebrate Red Cells. J. Exp. Biol. 203, 1395–1407. doi:10.1242/jeb.203.9.1395
Gibson J. S., Speake P. F., Ellory J. C. (1998). Differential Oxygen Sensitivity of the K+-Cl−cotransporter in Normal and Sickle Human Red Blood Cells. J. Physiology 511, 225–234. doi:10.1111/j.1469-7793.1998.225bi.x
Godart H., Dormandy A., Ellory J. C. (1997). Do HbSS Erythrocytes Lose KCl in Physiological Conditions? Br. J. Haematol. 98 (1), 25–31. doi:10.1046/j.1365-2141.1997.1762994.x
Herbert S. C., Mount D. B., Gamba G. (2004). Molecular Physiology of Cation-Coupled Cl- Cotransport: the SLC12 Family. Pflugers Archiv – Eur. J. Physiology 447, 580–593.
Hoffmann E. K. (1978). “Regulation of Cell Volume by Selective Changes in the Leak Permeabilities of Ehrlich Ascites Tumour Cells,” in Alfred Benson Symposium XI. Copehagen: Munsgaard, 397–417.
Jennings M. L., al-Rohil N. (1990). Kinetics of Activation and Inactivation of Swelling-Stimulated K+/Cl- Transport. The Volume-Sensitive Parameter Is the Rate Constant for Inactivation. J. General Physiology 95 (6), 1021–1040. doi:10.1085/jgp.95.6.1021
Jennings M. L., Schulz R. K. (1991). Okadaic Acid Inhibition of KCl Cotransport. Evidence that Protein Dephosphorylation Is Necessary for Activation of Transport by Either Cell Swelling or N-Ethylmaleimide. J. General Physiology 97 (4), 799–817. doi:10.1085/jgp.97.4.799
Kaji D. M., Gasson C. (1995). Urea Activation of K-Cl Transport in Human Erythrocytes. Am. J. Physiology-Cell Physiology 268, C1018–C1025. doi:10.1152/ajpcell.1995.268.4.c1018
Khan A., Ellory J. C. (2000). Elevated Temperatures Enhance KCCl Activity in Sickle Cells. Bioelectrochemistry 52 (2), 127–131. doi:10.1016/s0302-4598(00)00111-2
Lauf P. K., Bauer J., Adragna N. C., Fujise H., Zade-Oppen A. M., Ryu K. H., et al. (1992). Erythrocyte K-Cl Cotransport: Properties and Regulation. Am. J. Physiology-Cell Physiology 263 (5), C917–C932. doi:10.1152/ajpcell.1992.263.5.c917
Lauf P. K., Bauer J. (1987). Direct Evidence for Chloride-dependent Volume Reduction in Macrocytic Sheep Reticulocytes. Biochem. Biophysical Res. Commun. 144, 849–855. doi:10.1016/s0006-291x(87)80042-6
Lauf P. K., Theg B. E. (1980). A Chloride Dependent K+ Flux Induced by N-Ethylmaleimide in Genetically Low K+ Sheep and Goat Erythrocytes. Biochem. Biophysical Res. Commun. 92 (4), 1422–1428. doi:10.1016/0006-291x(80)90445-3
Nielsen O. B., Lykkeboe G., Cossins A. R. (1992). Oxygenation-activated K Fluxes in Trout Red Blood Cells. Am. J. Physiology-Cell Physiology 263, C1057–C1064. doi:10.1152/ajpcell.1992.263.5.c1057
Nikinimaa M. (1990). Vertebrate Red Blood Cells: Adaptations of Function to Respiratory Requirements. Berlin Heidelberg: Springer-Verlag.
Pretini V., Koenen M. H., Kaestner L., Fens M. H. A., Schiffelers R. M., Bartels M., et al. (2019). Red Blood Cells: Chasing Interactions. Front. Physiology. doi:10.3389/fphys.2019.00945
Samaja M., Gattinoni L. (1978). Oxygen Affinity in the Blood of Sheep. Respir. Physiol. 34, 385–392. doi:10.1016/0034-5687(78)90136-6
Speake P. F., Gibson J. S. (1997). Urea-stimulated K-Cl Cotransport in Equine Red Blood Cells. Pflugers Arch. 434, 104–112. doi:10.1007/s004240050369
Speake P. F., Roberts C. A., Gibson J. S. (1997). Effect of Changes in Respiratory Blood Parameters on Equine Red Blood Cell K-Cl Cotransporter. Am. J. Physiology-Cell Physiology 273 (6), C1811–C1818. doi:10.1152/ajpcell.1997.273.6.c1811
Starke L. C., Jennings M. L. (1993). K-cl Cotransport in Rabbit Red Cells: Further Evidence for Regulation by Protein Phosphatase Type 1. Am. J. Physiology-Cell Physiology 264 (1), C118–C124. doi:10.1152/ajpcell.1993.264.1.c118
Keywords: KCl cotransport, LK sheep, incubation media, volume, pH, urea
Citation: Lu DC-Y, Hannemann A and Gibson JS (2022) Does Plasma Inhibit the Activity of KCl Cotransport in Red Cells From LK Sheep?. Front. Physiol. 13:904280. doi: 10.3389/fphys.2022.904280
Received: 25 March 2022; Accepted: 28 April 2022;
Published: 24 May 2022.
Edited by:
Mikko Juhani Nikinmaa, University of Turku, FinlandReviewed by:
Helene Guizouarn, Centre National de la Recherche Scientifique (CNRS), FranceCopyright © 2022 Lu, Hannemann and Gibson. This is an open-access article distributed under the terms of the Creative Commons Attribution License (CC BY). The use, distribution or reproduction in other forums is permitted, provided the original author(s) and the copyright owner(s) are credited and that the original publication in this journal is cited, in accordance with accepted academic practice. No use, distribution or reproduction is permitted which does not comply with these terms.
*Correspondence: John S. Gibson, anNnMTAwMUBjYW0uYWMudWs=
Disclaimer: All claims expressed in this article are solely those of the authors and do not necessarily represent those of their affiliated organizations, or those of the publisher, the editors and the reviewers. Any product that may be evaluated in this article or claim that may be made by its manufacturer is not guaranteed or endorsed by the publisher.
Research integrity at Frontiers
Learn more about the work of our research integrity team to safeguard the quality of each article we publish.